DOI:
10.1039/D0RA00698J
(Paper)
RSC Adv., 2020,
10, 6395-6404
Facile fabrication of porous BiVO4 hollow spheres with improved visible-light photocatalytic properties†
Received
22nd January 2020
, Accepted 4th February 2020
First published on 11th February 2020
Abstract
Bismuth vanadate (BiVO4) hollow spheres with porous structure have been successfully fabricated by a one-step wet solution method with no surfactant and template. The structure, morphologies, and composition of the as-prepared products were studied with X-ray powder diffraction (XRD), transmission electron morphology (TEM), Brunauer–Emmett–Teller (BET), X-ray photoelectron spectroscopy (XPS) and UV-vis spectroscopy. Based upon the time-dependent experimental results, BiVO4 nanospheres with hollow and solid structures can be controlled effectively through the reaction time, and a reasonable formation process was suggested in this work. Moreover, the experiment of degrading methyl orange (MO) under visible-light illumination was conducted to evaluate the photocatalytic performance of the obtained BiVO4 samples. The porous BiVO4 hollow spheres exhibit superior visible-light photocatalytic properties for MO degradation than other photocatalysts under irradiation, and could be reused for up to five times without significant reduction in the photocatalytic activity. In addition, based on active group trapping experiments, ˙OH radicals as the main active species from H2O2 molecules play a vital role in the photocatalytic degradation of MO, and a photocatalytic mechanism for the BiVO4 system was proposed. High photocatalytic activity, universality and stability suggest that the porous BiVO4 hollow spheres may have potential applications in wastewater treatment.
1. Introduction
The rapid growth of the economy will inevitably give rise to environmental pollution and energy shortages. The large discharge of colored wastewater from textile, paper, rubber and other industries has posed a threat to people's health. Semiconductor photocatalysis utilizes free and inexhaustible solar energy to convert these pollutants to carbonaceous products, which provides an effective avenue for environmental purification and solar energy utilization.1,2 As a green technology, photocatalysis has been an attractive alternative approach to treat wastewater containing colorants in the last few years.3–5 The widely used semiconductor-based photocatalysts include zinc oxide (ZnO) and titanium dioxide (TiO2).6,7 However, only ultraviolet or near ultraviolet fractions of sunlight can be absorbed by ZnO and TiO2 because of their broad bandgaps (3.0–3.2 eV). So, the development of photocatalysts with enhanced solar energy utilization efficiency is urgent.8
Among various kinds of visible-light photocatalysts, bismuth vanadate (BiVO4) with a bandgap of about 2.4 eV was recognized as a promising visible-light-driven photocatalyst to degrade colorants.9,10 The morphology, particle size and crystal structure of the samples significantly affect the photocatalytic properties. In the past few years, BiVO4 with various micro- and nano-structures, such as fibers,9 quantum dots,11 nanosheets,12 olive-like structures,13 microtubes,14 and hollow spheres,15 have been prepared by some controlled methods to enhance the photocatalytic activity. And some remarkable achievements have been obtained. Compared with the solid materials, the porous and hollow structures have been extensively investigated because of their widespread promising application scenarios in multiple areas, for example catalysis,16 molecular separation,17 photonics,18 and gas sensors.19 In general, the preparation of hollow structure utilizes the removable or sacrificial hard and soft templates,20,21 which limits the crystallization, the grain size-distribution and the purity of products because of the high temperature heat treatment. Therefore, it is scientific and practical essential to develop a template-free facile synthesis approach of porous BiVO4 with hollow structure.
In this paper, bismuth vanadate (BiVO4) nanospheres with porous and hollow structures were prepared with a facile and effective template-free method, which differed from other synthesis approaches of BiVO4 hollow spheres13,22,23 in the utilization of template. To reveal the impact of morphologies on the photocatalytic activity of BiVO4 samples, solid spheres were also fabricated for comparison. The results indicated the as-synthesized porous BiVO4 hollow spheres presented better photocatalytic activity and stability in degrading dyes under visible-light illumination.
2. Experiment section
2.1 Reagents
Deionized water and as-received analytical grade chemical reagents were used without further purification throughout the experiment. Reactive dyes, such as reactive black R-2BG and reactive orange R-2RLN, were purchased from Zhejiang Runtu Co., Ltd, China.
2.2 Preparation of BiVO4 samples
Typically, NH4VO3 (1.0 mmol) and Bi(NO3)3·5H2O (1.0 mmol) were dispersed in 1 mL concentrated nitric acid and 15 mL deionized water under vigorous stirring. After stirring for 10 min, NaHCO3 (1.56 g) was slowly added into the obtained solution with constant stirring. Another 0.5 h stirring was performed before the above suspension solution was sealed in a stainless-steel autoclave (volume 25 mL) with Teflon liner. Then, the autoclave was placed in the oven at 120 °C for 2 h, and then naturally cooled to ambient air temperature. Finally, the light yellow samples obtained by centrifugation were washed repeatedly with ethanol and distilled water for five times, and dried at 60 °C for 12 h in vacuum. The BiVO4 solid spheres were prepared with the similar method to that of the porous BiVO4 hollow spheres but at the reaction time of 10 min.
2.3 Characterization
The morphologies of the samples were characterized by scanning electron microscopy (SEM, Sigma300) and transmission electron microscope (TEM, JEM-1011). X-ray powder diffraction (XRD) patterns were collected by an Empyrean X-ray diffract meter with Cu Kα radiation source (λ = 0.15406 nm) for revealing the crystal phase of the samples. A X-ray photoelectron spectroscopy (XPS) in a Physical Electronics PHI 1600 spectrometer with Al Kα X-ray source (E = 1486.6 eV) was used, and the XPS analysis was carried out at a pass energy of 187.85 eV, and the C 1s peak at 284.6 eV was used to calibrate all the binding energies of the composing elements. A Micromeritics TriStar 3020 instrument was used to measure N2 adsorption–desorption isotherms. The Brunauer–Emmett–Teller (BET) surface areas and the pore size distribution of the samples were determined by the BET method and the Barrett–Joyner–Halenda (BJH) method. A spectrophotometer (UV-2550, Shimadzu, Japan) was employed to measure the UV-vis diffused reflectance spectra of the samples with BaSO4 as a reference. A Fluoromax-3 spectrometer (Horiba Scientific) was employed to measure the photoluminescence (PL) emission spectrum of samples at room temperature.
For photoelectrochemical characterizations, a CHI760E electrochemical workstation (Chenhua, Shanghai, China) was utilized to perform conventional three-electrode tests. The working electrode was prepared by ultrasound dispersion of the powder sample (1 mg) in ethanol (1 mL) for 30 min. The slurry was suspended on an F-doped tin oxide (FTO) glass substrate with 0.196 cm2 active area drop by drop. The uniform electrode film was dried by air and then transferred to oven at 300 °C for 3 h. And a platinum wire and Ag/AgCl (saturated KCl) acted as counter electrode and reference electrode, respectively. The photocurrent properties of the photocatalysts were measured in a Na2SO4 aqueous solution (0.5 mol L−1) at 0.6 V (vs. Ag/AgCl). The light source was a 300 W xenon arc lamp and placed at 10 cm distance from the electrochemical cell. All light wavelengths below 420 nm were eliminated with glass filter.
2.4 Photocatalytic activity
Typically, 10 mg of photocatalysts were suspended in the dye aqueous solution (20 mg L−1, 20 mL), such as methyl orange (MO), rhodamin B (RhB), methylene blue (MB), reactive black R-2BG (RB) and reactive orange R-2RLN (RO), with the pH value of about 2.0 adjusted by concentrate hydrochloride under ultrasonication for 5 min. To reach the adsorption–desorption equilibrium on catalyst particles, the suspensions were well magnetically stirred at 300 rpm for 0.5 h in the dark before irradiation. 0.05 mL of H2O2 solution (30 wt%) was added into the above suspension. Then a 250 W xenon arc lamp as the light source was switched on to initiate the photocatalytic reaction and a glass filter was adopted to eliminate UV wavelengths below 420 nm. The degradation experiments were conducted at room temperature with a continuous magnetic stirring. The samples were taken from the suspensions at different time intervals, and then analyzed after the photocatalyst particles were removed by centrifugation immediately. The absorption of the centrifuged aqueous solution was measured with a UV-vis spectrophotometer to monitor the MO concentration. The photodegradation ratio (η) was obtained according to the following equation (eqn (1)). |
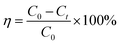 | (1) |
where C0 and Ct are the concentrations of MO solution at initial time and certain irradiation time (t).
2.5 Active species trapping experiments and recycle measurements
In order to detect the active species in the photocatalytic reaction, tert-butyl alcohol (TBA, 1 mmol L−1), benzoquinone (BQ, 1 mmol L−1), or disodium ethylenediaminetetraacetate dehydrate (EDTA-2Na, 1 mmol L−1) were added to the photocatalytic system to make clear the function of hydroxyl radical (˙OH), superoxideradiacal (˙O2−) and hole (h+), respectively. The tests for the stability of BiVO4 photocatalysts were performed according to the photocatalytic degradation experiments of MO. The recycling experiments were performed 5 times with the same experimental procedure and conditions as above. After every photocatalytic cycle test, the catalysts obtained by centrifugation for the test were dried with a vacuum oven.
3. Results and discussion
3.1 Morphology and structure
SEM and TEM were adopted to observe the morphologies of the as-prepared samples, and the results are shown in Fig. S1† and 1. As shown in Fig. S1,† the sphere-like nanoparticles of about 50 nm are fabricated on a large scale. The above results are further proved by TEM image in Fig. 1a that the BiVO4 particles are almost spherical nanoparticles and are intertwined with each other. Interestingly, the further enlarged TEM image in Fig. 1b shows that BiVO4 nanoparticles are hollow structures with the diameter and wall thickness of about 20 nm and 5 nm. The aggregates of hollow structures with small size in Fig. 1b are favorable for the entry and exit of chemical species, and the recovery and reuse of catalyst. At the same time a big specific surface area will be created in the inner space. Therefore, it is a reasonable candidate for photocatalysts.
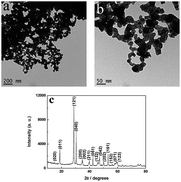 |
| Fig. 1 TEM images (a and b) and XRD pattern (c) of the as-synthesized product. | |
Fig. 1c presents the XRD pattern of the as-prepared BiVO4 hollow spheres. All measured diffraction peaks in the XRD spectrum can be referred to monoclinic BiVO4 according to the standard data of JCPDS file number 14-0688. The strong and sharp peaks imply that the as-prepared BiVO4 hollow spheres are well crystallized. The absence of peak from other phases suggests the successful preparation of pure and single phase BiVO4 crystal.
In order to further confirm the chemical composition and the surface chemical status of elements, the prepared BiVO4 hollow spheres were further characterized by XPS, as shown in Fig. S2.† The typical XPS survey spectrum of BiVO4 hollow spheres with distinct peaks of Bi 4f, V 2p and O 1s is displayed in Fig. S1a.† The characteristic peaks of Bi 4f7/2 and Bi 4f5/2 located at 158.9 eV and 164.5 eV prove the presence of Bi3+ in BiVO4 hollow spheres.24 The peaks of V 2p3/2 and V 2p1/2 at 516.5 eV and 524.0 eV are ascribed to the V5+ species, confirming that the element V in the sample was in pentavalent state.24 Also, the sample showed similar XPS signals of O 1s at 529.8 eV and 532.2 eV from the O2− anions in BiVO4 hollow spheres.24
The formation process of BiVO4 hollow spheres was investigated with time-dependent experiments, and the evolution process was clearly presented by the TEM images of the intermediate products, as shown in Fig. 2. At the beginning, the nanoparticles with sphere-like shape were formed after reacting for 10 min in Fig. S3.† Further observation showed that the spherical nanoparticles with about 50 nm were solid structure (Fig. 2a). These nanoparticles were intertwined with each other, which leads to a large number of pores in the product. With the increasing reaction time to 30 min (Fig. 2b), the nanoparticles with similar morphology has no changes in size. At the same time close observation shows that some small pores appear inside a small amount of nanoparticles. As shown in Fig. 2c and d, this feature became more manifest with the increase of reaction time. The inner parts of spherical nanoparticles have more and larger pores. The formation of spherical nanoparticles with uniform hollow structures was finally completed 2 h after the reaction (Fig. 1a and b). The crystalline structure of the products prepared at the reaction time of 10 min, 30 min, 60 min and 90 min were determined by XRD measurements presented in Fig. S4.† As depicted in Fig. S4,† all diffractions peaks of the as-prepared samples at the different reaction time can be well assigned to monoclinic BiVO4, which is in accordance to BiVO4 hollow spheres (JCPDS 14-0688). Based on the further observation of Fig. S4a and b,† it is found that the position and intensity of the diffraction peaks are almost unchanged with the extension of reaction time, indicating that the crystal structure of the products can be independent of reaction time.
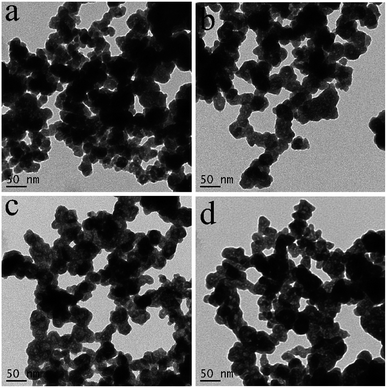 |
| Fig. 2 TEM images of the as-prepared products at the different reaction times, (a) 10 min, (b) 30 min, (c) 60 min and (d) 90 min. | |
The formation mechanism of BiVO4 hollow spheres can be deduced as follows. When NaHCO3 was slowly added in the reaction system, a large amount of CO2 gas was generated. The gas molecules would congregate at the BiVO4 nuclei and limit the further growth of crystal nucleus at the early stage. With the increasing reaction temperature, the expansion of gas molecules leaded to the appearance of holes in the interior of the product, which eventually forms the BiVO4 hollow nanospheres. The evolution process of the morphologies of the as-synthesized products is described in Scheme 1. During the formation process of hollow structures, the internal and external pores are intertwined with one another so that BiVO4 hollow spheres have a larger specific surface area, indicating the potential application in the photocatalytic field. And BiVO4 hollow spheres and solid spheres can be controllably synthesized by adjusting the reaction time.
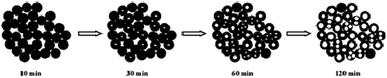 |
| Scheme 1 Formation mechanism of the as-prepared BiVO4 hollow spheres. | |
3.2 Nitrogen adsorption–desorption isotherms
The porous properties of the as-prepared BiVO4 hollow spheres and solid spheres clearly revealed by the TEM images are further investigated by the nitrogen adsorption–desorption isotherms and the distribution of pore size. The BiVO4 samples showed the presence of porous structure and gave type IV isotherms according to the BET classification, as shown in Fig. 3.25 The BET specific surface area of the obtained product is 17.46 m2 g−1 and 4.42 m2 g−1 for BiVO4 hollow spheres and BiVO4 solid spheres, respectively. The increased surface area of BiVO4 hollow spheres could be attributed to the porous and hollow structure. The presence of macropores and mesopores in the as-synthesized samples is indicated by the hysteresis loop in the relative pressure (p/p0) range of 0.7 to 1 and proved by the pore size distribution (inset of Fig. 3). The calculated pore size of the BiVO4 hollow spheres with BJH method ranges very widely and about at 3.8 nm, 31 nm and 117 nm, according to the adsorption branch. However, the pore size of the BiVO4 solid spheres is mainly at about 70 nm. In addition, a very small number of pores are observed at about 2.5 nm. It is worth to mention here that the presence of a large number of pores will lead to a high specific surface area. A transport pathway to the interior of photocatalyst for reactants would be provided by the porous properties of the sample, and so its catalytic performance in wastewater treatment could be enhanced at the same time.
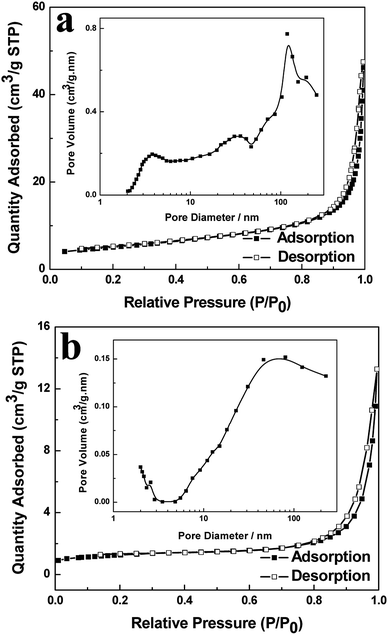 |
| Fig. 3 Nitrogen adsorption–desorption isotherms and pore size distribution curve (inset) of BiVO4 hollow spheres (a) and BiVO4 solid spheres (b). | |
3.3 UV-vis diffuse reflection spectra
The UV-vis absorbance spectra of the obtained BiVO4 samples can be found in Fig. 4. Both BiVO4 hollow spheres and BiVO4 solid spheres exhibit a strong photoabsorption from UV light to visible light. The steep shape of the spectra proves that the visible-light absorption was contributed to the band gap transition instead of the impurity level transition.23 The significant visible light absorption of BiVO4 hollow spheres indicates that hollow structure is a better candidate than solid material in the visible-light photocatalysis. From inset of Fig. 4, the band gap energies of BiVO4 hollow spheres and solid spheres could be estimated from the linear fitting of the linear part of (αhν)2 vs. (hν) plot,22 and are about 2.39 eV and 2.62 eV, respectively. The absorption of UV- and visible-light for exciting valence band electrons to the conduction band due to the narrow bandgap of BiVO4 samples will improve the effective solar energy utilization. The band gap of BiVO4 hollow spheres is narrower than that of solid ones, so the absorption and utilization of visible light energy will be stronger for BiVO4 hollow spheres, thus, the higher photocatalytic activity BiVO4 hollow spheres will be expected.
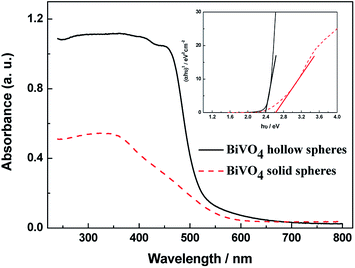 |
| Fig. 4 UV-vis diffuse reflection spectra and plots of (αhν)2 versus the photon energy (hν) curves (inset) of the as-prepared BiVO4 samples. | |
3.4 Photocatalytic properties
The MO degradation experiments under the visible-light irradiation were conducted to evaluate the photocatalytic activity of the as-prepared photocatalyst, as shown in Fig. 5. To demonstrate the photodegradation of MO, UV-vis spectra were taken over certain time in the presence of BiVO4 hollow spheres (Fig. 5a). The photocatalytic degradation process was monitored through the intensity of characteristic absorption peak around 500 nm. With the increasing irradiation time, the characteristic peak decreases continuously in intensity and blue shifts in wavelength, indicating the decomposition of MO. And the characteristic absorption peak at about 500 nm disappears at the irritation time of 240 min, indicating the complete degradation of MO.
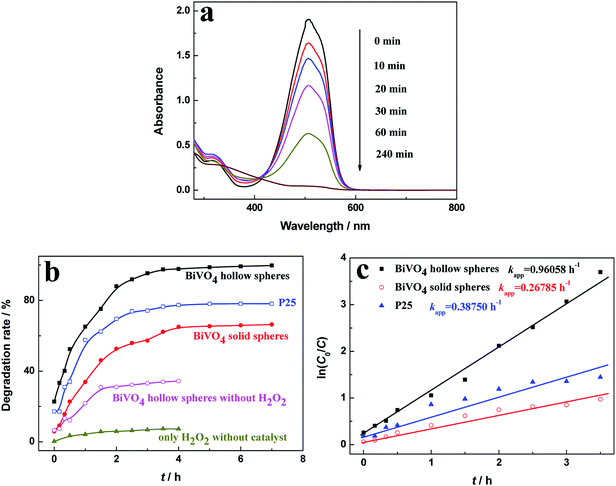 |
| Fig. 5 (a) Absorption spectra of MO aqueous solutions in the presence of BiVO4 hollow spheres, (b) degradation rate of MO at different intervals and (c) pseudo-first-order kinetic plots of MO in the presence of various photocatalysts. | |
Fig. 5b shows the photocatalytic performance of MO in the presence of various photocatalysts. The degradation rate of 20 mg L−1 MO solution with 0.05 mL of H2O2 (30 wt%) after 4 h reaction was only about 7.27% in the absence of various photocatalyst, indicating that the photocatalyst is crucial in the photocatalytic reaction. In the presence of H2O2 and photocatalysts, the degradation rate of MO increases rapidly at first, then increases gently, and keeps stable finally as illumination time increased. The degradation rates of MO for as-prepared BiVO4 hollow and solid spheres after 3.0 h reaction were 95.3% and 57.2%, respectively. For comparison, degrading MO experiment is conducted with the photocatalyst of P25. And about 74.2% MO was degraded under visible-light irradiation for 3 h, which is lower than BiVO4 hollow spheres and slightly higher than BiVO4 solid spheres. But, the degradation of MO for BiVO4 hollow spheres without H2O2 was only 33.1% at the same irradiation conditions. The effect of H2O2 amount on the photocatalytic performance of MO in the presence of BiVO4 hollow spheres is investigated in Fig. S5.† It can be found that with the increasing amount of H2O2, the photocatalytic degradation rate of MO first goes up rapidly and then tends to stabilize gradually. The further increase of H2O2 over 0.075 mL inhibits the photocatalytic activity, which may mainly due to the quenching of ˙OH group by the excess H2O2.26,27 The degradation of organic molecules with the BiVO4 photocatalysts can be greatly accelerated by the radical precursor H2O2,28 because H2O2 molecules can react with photogenerated electrons as an electron trapping reagent and the produced ˙OH radicals will further react with MO and increase degradation of MO. Thus, the recombination of photogenerated electrons and holes will be effectively suppressed assisted by H2O2, leading to a high photocatalytic efficiency. In addition, the photogenerated charges must migrate to the surface of photocatalyst for the photocatalytic degradation of dyes, so large specific surface area will enhance the catalytic efficiency. Compared with BiVO4 solid spheres, the significantly superior performance in the degradation of MO with BiVO4 hollow spheres could be contributed to the large specific surface area due to the unique hollow and porous structures of the samples.
The pseudo first-order Langmuir–Hinshelwood (L–H) model for heterogeneous photocatalysis under low concentration dye is also used to investigate the photocatalytic degradation kinetic,29,30 and the degradation rate (r) is given by the following equation (eqn (2)).
|
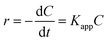 | (2) |
where
Kapp is the apparent first-order rate constant (h
−1),
C0 and
C are the concentrations of MO at adsorption–desorption equilibrium and various intervals of time (
t).
Fig. 5c shows
Kapp obtained from the gradient of the curve of ln(
C0/
C)
versus time (
t), and the calculated
Kapp values are 0.9606, 0.2679 and 0.3875 h
−1 for BiVO
4 hollow spheres, BiVO
4 solid spheres and P25, respectively. The BiVO
4 hollow spheres exhibit the largest
Kapp value that is approximately 3.6 and 2.5 times higher than these of BiVO
4 solid spheres and P25, respectively. The higher photocatalytic performance of BiVO
4 hollow spheres over BiVO
4 solid spheres and P25 is agreement with that of
Fig. 5b. The photocatalytic performances of BiVO
4 hollow spheres are further compared with other BiVO
4 hollow structures as summarized in
Table 1. Various BiVO
4 samples with hollow structures can effectively degrade pollutants under their own photocatalytic reaction conditions. As compared to other BiVO
4 hollow catalysts, the as-prepared BiVO
4 hollow spheres can be highly beneficial for pollutants removal at the low concentration of photocatalyst and low light power, indicating superior performance in the visible-light photocatalysis.
Table 1 Comparison of photocatalytic performances of BiVO4 hollow structures
Synthesis method |
Morphology |
Photocatalytic activity |
Ref. |
Method: wet solution method |
Hollow nanospheres |
Pollutant: MO, RhB, MB, RB, RO |
This study |
10 mg photocatalyst, 20 mL 20 mg L−1 dye solutions |
Additive: no additives |
Light source: 250 W Xe lamp (λ > 420 nm) |
Degradation: 97.8% for MO, 98.6% for RhB, 98.0% for MB, 96.5% for RB and 95.7% for RO in 4 h |
Method: hydrothermal route |
Hollow microspheres |
Pollutant: MB |
31 |
Additive: urea |
0.1 g photocatalyst, 20 mL 3 × 10−5 M dye solution |
Light source: 350 W Xe lamp (λ > 420 nm) |
Degradation: color disappeared in 180 min |
Method: a precursor solution mediated growth method |
Hollow spheres |
Pollutant: RhB |
32 |
0.5 g photocatalyst, 100 mL 10−4 M dye solution |
Light source: 120 W compact fluorescent lamp (λ > 420 nm) |
Additive: polyvinyl alcohol (PVA) |
Degradation: characteristic peak disappears in 50 min |
Method: solvothermal process under microwave irradiation |
Hollow spheres with hierarchical microstructures |
Pollutant: MO |
33 |
0.2 g photocatalyst, 100 mL 0.01 g L−1 dye solution |
Additive: EDTA |
Light source: 300 W Xe lamp (λ > 420 nm) |
Degradation: characteristic absorption disappears in 180 min |
Method: sacrificial template growth technique (calcination) |
Multi-shell hollow spheres |
Pollutant: MB |
34 |
0.1 g photocatalyst, 100 mL 10−5 M dye solution |
Light source: 300 W Xe lamp (λ > 420 nm) |
Additive: carbonaceous microspheres |
Degradation: 100% in 80 min |
Method: anchoring method (calcination) |
Hollow nanospheres |
Pollutant: RhB |
23 |
0.1 g photocatalyst, 100 mL 10−5 M dye solution |
Light source: 500 W Xe lamp (λ > 420 nm) |
Additive: colloidal carbon spheres |
Degradation: completely degraded in 70 min |
Method: hydrothermal method |
Core-shell structured hollow spheres |
Pollutant: RhB |
35 |
0.3 g photocatalyst, 600 mL 10−5 M dye solution |
Light source: 500 W Xe lamp (λ > 400 nm) |
Additive: no additives |
Degradation: 99% in 4.5 h |
Method: hydrothermal method |
Hollow polygon |
Pollutant: MB |
36 |
0.1 g photocatalyst, 100 mL 5 mg L−1 dye solution |
Light source: 500 W Xe lamp |
Additive: ethylenediamine tetraacetic acid (EDTA) |
Degradation: 90.84% in 5 h |
Method: reflux method |
Microtubes with flower-like morphology |
Pollutant: RhB |
14 |
0.5 mmol photocatalyst, 100 mL 10−5 M dye solution |
Additive: no additives |
Light source: 500 W Xe lamp (λ > 400 nm) |
Degradation: 96% in 180 min |
Method: ultrasound irradiation |
Spindle particles with hollow interior |
Pollutant: RhB |
37 |
0.2 g photocatalyst, 100 mL 10−5 M dye solution |
Light source: 500 W Xe lamp (λ > 400 nm) |
Additive: no additives |
Degradation: 99% in 240 min |
In order to investigate the universality of photocatalytic performance of BiVO4 samples, the photocatalytic activities of other dye aqueous solutions assisted by BiVO4 hollow or solid spheres are shown in Fig. 6. At the similar experimental conditions, the degradation rates of RhB, MB, RB and RO for BiVO4 hollow spheres under visible light irradiation of 4 h are more than 95%, which is obviously higher than those in the presence of BiVO4 solid spheres. Based on the comparison with the BiVO4 solid spheres, the BiVO4 hollow spheres exhibit the outstanding photocatalytic properties for various dyes, indicating that the BiVO4 nanospheres with porous and hollow structure have better universality and potential applications in visible light photocatalytic field.
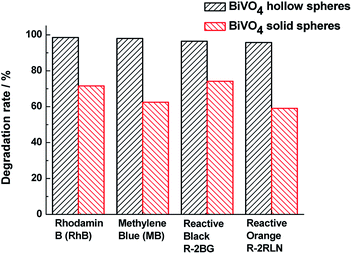 |
| Fig. 6 Degradation rate of various dyes in the presence of BiVO4 hollow or solid spheres. | |
To estimate the reproducibility and stability of the BiVO4 samples, Fig. 7 presents the recycling test of catalyst for the visible light photocatalytic degradation of MO. The catalytic activities of BiVO4 samples are almost unchanged after 5 test recycles of degradation of MO. It is found in Fig. 7 that after 5 cycles, the degradation rates of MO solution are 97.0% for BiVO4 hollow spheres and 64.0% for BiVO4 solid spheres, which are 99.2% and 98.5% of the first degradation rate of MO solution, respectively. This suggests that BiVO4 hollow spheres have slightly better cyclic stability than BiVO4 solid spheres.
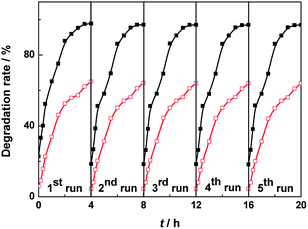 |
| Fig. 7 Recycle curves of the BiVO4 hollow spheres (black and solid) and BiVO4 solid spheres (red and hollow) for the visible light photocatalytic degradation of MO. | |
3.5 Photoelectrochemical analyses
To reveal the high efficiency separation of photogenerated electrons and holes, the transient photocurrent responses of BiVO4 hollow spheres and solid spheres are recorded for a few on-off illumination cycles. The higher photocurrent density implies the efficient photogenerated charge separation and their transfer across interface.38 The I–t curves for BiVO4 samples are shown in Fig. 8a for comparison. Reproducible increase under irradiation and quick recovery in the dark are observed from the photocurrent response of the BiVO4 samples. Moreover, the increase of the photocurrent response of BiVO4 hollow spheres is more significant in comparison with that of BiVO4 solid spheres, suggesting the higher electron–hole pair separation efficiency and a smaller recombination possibility under visible-light illumination. Besides, PL spectra are used to disclose the migration, transfer, and recombination process of the photogenerated charges in the semiconductor.22 PL spectra of BiVO4 samples were recorded with a pump of 325 nm wavelength, as shown in Fig. S6.† The emission intensity of BiVO4 hollow spheres with similar emission peaks is weaker than that of BiVO4 solid spheres, indicating the high efficiency separation of photogenerated electron–hole pairs.
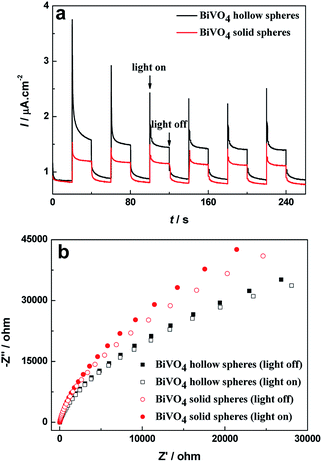 |
| Fig. 8 (a) Transient photocurrent curves and (b) Nyquist plots of BiVO4 hollow spheres and BiVO4 solid spheres under dark and visible light irradiation. | |
Electrochemical impedance spectroscopy (EIS) measurement has been used to reveal the efficient charge transfer process in the traditional three-electrode system39 and corresponding Nyquist plots of BiVO4 samples with and without visible light irradiation can be found in Fig. 8b. The BiVO4 hollow spheres show smaller semicircle as compared to the BiVO4 solid spheres with and without visible light irradiation. And it can be seen clearly that for the same sample, the radii of semicircle at illumination is smaller in size than that at darkness, implying the generation of charge carriers and low resistance as compared to dark condition. The minimum semicircle of BiVO4 hollow spheres under visible light irradiation indicates the easier interfacial charge transfer between solution and electrode, and excellent conductivity, which suppresses recombination of photogenerated charge and decreases resistance. Therefore BiVO4 hollow spheres could exhibit superior photocatalytic activity.
3.6 Mechanism of photocatalytic activity
Studies on active group trapping experiments give further verification of the photocatalytic activities of BiVO4 hollow spheres. During the photocatalytic degradation of organic pollutants, hydroxyl radiacals (˙OH), superoxide radical (˙O2−) and hole (h+) are generally considered as the main active species. So the reactive species trapping experiments on the photocatalytic degradation of MO are conducted to further investigate the photocatalytic mechanism over BiVO4 hollow spheres. Tert-butyl alcohol (TBA), benzoquinone (BQ) and disodium ethylenediaminetetraacetate dehydrate (EDTA-2Na) are introduced as quenchers of ˙OH, ˙O2− and h+ to make clear the effective active species in the photocatalytic process. The photocatalytic degradation rates of MO over BiVO4 hollow spheres without and with the help of H2O2 are shown in Fig. 9. As presented in Fig. 9a, experiments involving EDTA-2Na show a significant decrease in the degradation rate in comparison to the blank experiment of no quencher, implying h+ is the predominant active species in the absence of H2O2. Besides, the addition of BQ into the photocatalytic system could also decrease the photocatalytic degradation MO, suggesting ˙O2− also plays an important role in the photocatalytic process. In order to make clear the importance of O2 molecules, the photocatalytic reaction of MO is carried out under N2 without any quencher. A fast deactivation of degradation rate under nitrogen suggests that O2 molecules are involved in the photocatalytic reaction and promote the formation of ˙O2−. Therefore h+ and ˙O2− are the mainly active groups for the photodegradation of MO without the help of H2O2. Compared with Fig. 9a, the photocatalytic activity of MO is significantly inhibited with TBA and decreases to 14.6% from 95.3% with the help of H2O2, revealing the active role played by ˙OH mainly from H2O2. Similarly, the addition of BQ also reduces the activity and it drops to 52.4%, showing the significant impact of ˙O2− radicals, further proved by the N2 experiments in Fig. 9b. Based on the above analysis, the proposed photodegradation mechanism of MO over BiVO4 hollow spheres is depicted in Scheme 2. ˙OH, ˙O2− and h+ are all active groups for the photocatalytic degradation of MO. Among them, ˙OH radiaclas are predominantly active species with the help of H2O2. H2O2 plays an important role in the photocatalytic degradation of MO due to the presence of a large amount of ˙OH radicals from H2O2.
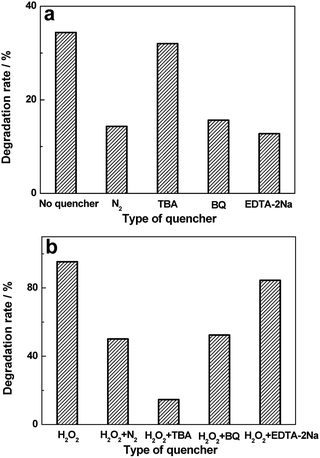 |
| Fig. 9 Effects of a series of quenchers on the degradation rates of MO over BiVO4 hollow spheres without (a) and with (b) the help of H2O2. | |
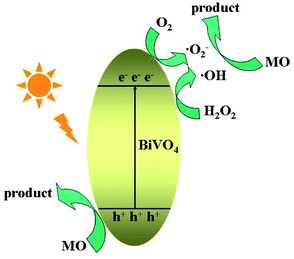 |
| Scheme 2 The schematic diagram of the proposed photocatalytic mechanism of MO for BiVO4 hollow spheres. | |
4. Conclusions
Bismuth vanadate (BiVO4) hollow spheres with porous structures have been fabricated by a facile template- and surfactant-free one-step wet solution method, which could be adopted to prepare hollow structures in a large scale. Based on the time-dependent experiments, BiVO4 spheres with hollow and solid structures are controlled through the reaction time and the formation process behind the synthesis of BiVO4 hollow spheres is proposed. In addition, due to the distinctive morphology and narrower bandgap, BiVO4 hollow spheres exhibit the outstanding visible-light photocatalytic activities and stability in the MO degradation comparing with BiVO4 solid spheres. In addition, the active species trapping experiments reveal the ˙OH radicals are predominantly active groups for the photocatalytic degradation of MO at the presence of H2O2. Overall, the obtained porous BiVO4 hollow spheres with high photocatalytic activities exhibit a promising application in wastewater treatment.
Conflicts of interest
There are no conflicts to declare.
Acknowledgements
We gratefully thank the financial supports by National Natural Science Foundation of China (20901051, 51372154), Science Foundation of Ministry of Housing and Urban-Rural Development of China (2015-K4-003) and Project of Science Technology Department of Zhejiang Province of China (2017C33209).
Notes and references
- M. Pirhashemi, S. Elhag, A. Habibi-Yangjeh, G. Pozina, M. Willander and O. Nur, Polyethylene glycol-doped BiZn2VO6 as a high-efficiency solar-light-activated photocatalyst with substantial durability toward photodegradation of organic contaminations, RSC Adv., 2018, 8(65), 37480–37491 RSC.
- L. K. Dhandole, M. A. Mahadik, S.-G. Kim, H.-S. Chung, Y.-S. Seo, M. Cho, J. H. Ryu and J. S. Jang, Boosting photocatalytic performance of inactive rutile TiO2 nanorods under solar light irradiation: synergistic effect of acid treatment and metal oxide co-catalysts, ACS Appl. Mater. Interfaces, 2017, 9, 23602–23613 CrossRef CAS.
- Y. Cui, J. Briscoe, Y. Wang, N. V. Tarakina and S. Dunn, Enhanced photocatalytic activity of heterostructured ferroelectric BaTiO3/α-Fe2O3 and the significance of interface morphology control, ACS Appl. Mater. Interfaces, 2017, 9, 24518–24526 CrossRef CAS PubMed.
- E. Prabakaran and K. Pillay, Synthesis of N-doped ZnO nanoparticles with cabbage morphology as a catalyst for the efficient photocatalytic degradation of methylene blue under UV and visible light, RSC Adv., 2019, 9(13), 7509–7535 RSC.
- N. Pugazhenthiran, R. V. Mangalaraja, P. Sathishkumar, S. Murugesan, T. Muneeswaran, T. Pandiyarajan, S. Naveenraj, D. Contreras and S. Anandan, Green synthesis of porous Au-Nx-TiO2 nanospheres for solar light induced photocatalytic degradation of diazo and triazo dyes and their eco-toxic effects, New J. Chem., 2018, 42(23), 18717–18728 RSC.
- M.-C. Wu, K.-C. Hsiao, Y.-H. Chang and K. Kordás, Core-shell heterostructures of rutile and anatase TiO2 nanofibers for photocatalytic solar energy conversion, ACS Appl. Nano Mater., 2019, 2(4), 1970–1979 CrossRef CAS.
- J. Kegel, V. Z. Zubialevich, M. Schmidt, I. M. Povey and M. E. Pemble, Effect of surface and defect chemistry on the photocatalytic properties of intentionally defect-rich ZnO nanorod arrays, ACS Appl. Mater. Interfaces, 2018, 10(21), 17994–18004 CrossRef CAS.
- H. Huang, F. Li, H. Wang and X. Zheng, The size controlled synthesis of Cu2S/P25 hetero junction solar-energy-materials and their application in photocatalytic degradation of dyes, RSC Adv., 2017, 7(79), 50056–50063 RSC.
- H. Liu, H. Hou, F. Gao, X. Yao and W. Yang, Tailored fabrication of thoroughly mesoporous BiVO4 nanofibers and their visible-light photocatalytic activities, ACS Appl. Mater. Interfaces, 2016, 8, 1929–1936 CrossRef CAS.
- T. Das, X. Rocquefelte, R. Laskowski, L. Lajaunie, S. Jobic, P. Blaha and K. Schwarz, Investigation of the optical and excitonic properties of the visible light-driven photocatalytic BiVO4 material, Chem. Mater., 2017, 29, 3380–3386 CrossRef CAS.
- M. Xie, Z. Zhang, W. Han, X. Cheng, X. Li and E. Xie, Efficient hydrogen evolution under visible light irradiation over BiVO4 quantum dot decorated screw-like SnO2 nanostructures, J. Mater. Chem. A, 2017, 5, 10338–10346 RSC.
- C. Dong, S. Lu, S. Yao, R. Ge, Z. Wang, Z. Wang, P. An, Y. Liu, B. Yang and H. Zhang, Colloidal synthesis of ultrathin monoclinic BiVO4 nanosheets for Z-scheme overall water splitting under visible light, ACS Catal., 2018, 8(9), 8649–8658 CrossRef CAS.
- B. Wang, L. Guo and T. He, Fabrication of an olive-like BiVO4 hierarchical architecture with enhanced visible-light photocatalytic activity, RSC Adv., 2016, 6(36), 30115–30124 RSC.
- L. Zhou, W. Wang, L. Zhang, H. Xu and W. Zhu, Single-crystalline BiVO4 microtubes with square cross-sections: microstructure, growth mechanism, and photocatalytic property, J. Phys. Chem. C, 2007, 111, 13659–13664 CrossRef CAS.
- J. Sun, G. Chen, J. Wu, H. Dong and G. Xiong, Bismuth vanadate hollow spheres: bubble template synthesis and enhanced photocatalytic properties for photodegradation, Appl. Catal., B, 2013, 132–133, 304–314 CrossRef CAS.
- Z. Wang, Q. Wang, W. Liu, H. Ran, C. Zhang, X. Han, X. He, X. Wang and C. Hu, Optical porous hollow-boxes assembled by SrSO4/TiO2/Pt nanoparticles for high performance of photocatalytic H2 evolution, Nano Energy, 2019, 59, 129–137 CrossRef CAS.
- A. Ovcharova, V. Vasilevsky, I. Borisov, S. Bazhenov, A. Volkov, A. Bildyukevich and V. Volkov, Polysulfone porous hollow fiber membranes for ethylene-ethane separation in gas-liquid membrane contactor, Sep. Purif. Technol., 2017, 183, 162–172 CrossRef CAS.
- Y. Liu, C. Li, H. Zhang, X. Fan, Y. Liu and Q. Zhang, One-pot hydrothermal synthesis of highly monodisperse water-dispersible hollow magnetic microspheres and construction of photonic crystals, Chem. Eng. J., 2015, 259, 779–786 CrossRef CAS.
- K. Tao, X. Han, Q. Yin, D. Wang, L. Han and L. Chen, Metal–organic frameworks-derived porous In2O3 hollow nanorod for high-performance ethanol gas sensor, ChemistrySelect, 2017, 2(33), 10918–10925 CrossRef CAS.
- X. Wang, J. Feng, Y. Bai, Q. Zhang and Y. Yin, Synthesis, properties, and applications of hollow micro-/nanostructures, Chem. Rev., 2016, 116, 10983–11060 CrossRef CAS PubMed.
- H. Zhang, H. Xu, M. Wu, Y. Zhong, D. Wang and Z. Jiao, A soft-hard template approach towards hollow mesoporous silica nanoparticles with rough surfaces for controlled drug delivery and protein adsorption, J. Mater. Chem. B, 2015, 3, 6480–6489 RSC.
- J.-Q. Li, M.-M. Cui, Z.-X. Liu, J. Du and Z.-F. Zhu, BiVO4 hollow spheres with hierarchical microstructures and enhanced photocatalytic performance under visible-light illumination, Phys. Status Solidi A, 2013, 210, 1881–1887 CAS.
- W. Yin, W. Wang, M. Shang, L. Zhou, S. Sun and L. Wang, BiVO4 hollow nanospheres: anchoring synthesis, growth mechanism, and their application in photocatalysis, Eur. J. Inorg. Chem., 2009,(29–30), 4379–4384 CrossRef CAS.
- C. Li, P. Zhang, R. Lv, J. Lu, T. Wang, S. Wang, H. Wang and J. Gong, Selective deposition of Ag3PO4 on monoclinic BiVO4 (040) for highly efficient photocatalysis, Small, 2013, 9, 3951–3956 CrossRef CAS PubMed.
- K. S. W. Sing, D. H. Everett, R. A. W. Haul, L. Moscou, R. A. Pierotti, J. Rouquérol and T. Siemieniewska, Reporting physisorption data for gas/solid systems with special reference to the determination of surface area and porosity (Recommendations 1984), Pure Appl. Chem., 1985, 57, 603–619 CAS.
- Y. Wang and C.-S. Hong, Effect of hydrogen peroxide, periodate and persulfate on photocatalysis of 2-chlorobiphenyl in aqueous TiO2 suspensions, Water Res., 1999, 33, 2031–2036 CrossRef CAS.
- H. M. Coleman, V. Vimonses, G. Leslie and R. Amal, Degradation of 1,4-dioxane in water using TiO2 based photocatalytic and H2O2/UV processes, J. Hazard. Mater., 2007, 146, 496–501 CrossRef CAS PubMed.
- M. Ge, L. Liu, W. Chen and Z. Zhou, Sunlight-driven degradation of rhodamine B by peanut-shaped porous BiVO4 nanostructures in the H2O2-containing system, CrystEngComm, 2012, 14, 1038–1044 RSC.
- W. Z. Tang and H. An, UV/TiO2 photocatalytic oxidation of commercial dyes in aqueous solutions, Chemosphere, 1995, 31, 4157–4170 CrossRef CAS.
- I. M. Arabatzis, T. Stergiopoulos, D. Andreeva, S. Kitova, S. G. Neophytides and P. Falaras, Characterization and photocatalytic activity of Au/TiO2 thin films for azo-dye degradation, J. Catal., 2003, 220, 127–135 CrossRef CAS.
- B. Cheng, W. Wang, L. Shi, J. Zhang, J. Ran and H. Yu, One-pot template-free hydrothermal synthesis of monoclinic BiVO4 hollow microspheres and their enhanced visible-light photocatalytic activity, Int. J. Photoenergy, 2012, 797968 Search PubMed.
- S. Sarkar and K. K. Chattopadhyay, Visible light photocatalysis and electron emission from porous hollow spherical BiVO4 nanostructures synthesized by a novel route, Phys. E, 2014, 58, 52–58 CrossRef CAS.
- J.-Q. Li, M.-M. Cui, Z.-X. Liu, J. Du and Z.-F. Zhu, BiVO4 hollow spheres with hierarchical microstructures and enhanced photocatalytic performance under visible-light illumination, Phys. Status Solidi A, 2013, 210(9), 1881–1887 CAS.
- L. Zong, P. Cui, F. Qin, K. Zhao, Z. Wang and R. Yu, Heterostructured bismuth vanadate multi-shell hollow spheres with high visible-light-driven photocatalytic activity, Mater. Res. Bull., 2017, 86, 44–50 CrossRef CAS.
- Y. Lu, Y.-S. Luo, H.-M. Xiao and S.-Y. Fu, Novel core-shell structured BiVO4 hollow spheres with an ultra-high surface area as visible-light-driven catalyst, CrystEngComm, 2014, 16(27), 6059–6065 RSC.
- W. Ma, Z. Li and W. Liu, Hydrothermal preparation of BiVO4 photocatalyst with perforated hollow morphology and its performance on methylene blue degradation, Ceram. Int., 2015, 41(3), 4340–4347 CrossRef CAS.
- W. Liu, L. Cao, G. Su, H. Liu, X. Wang and L. Zhang, Ultrasound assisted synthesis of monoclinic structured spindle BiVO4 particles with hollow structure and its photocatalytic property, Ultrason. Sonochem., 2010, 17(4), 669–674 CrossRef CAS PubMed.
- Y. Liu, Y.-X. Yu and W.-D. Zhang, MoS2/CdS heterojuction with high photoelectrochemical activity for H2 evolution under visible light: the role of MoS2, J. Phys. Chem. C, 2013, 117(25), 12949–12957 CrossRef CAS.
- S. Kumar, A. Kumar, A. Kumar, R. Balaji and V. Krishnan, Highly efficient visible light active 2D-2D nanocomposites of N-ZnO-g-C3N4 for photocatalytic degradation of diverse industrial pollutants, ChemistrySelect, 2018, 3(6), 1919–1932 CrossRef CAS.
Footnotes |
† Electronic supplementary information (ESI) available: (1) SEM images of BiVO4 hollow spheres; (2) XPS spectra; (3) SEM images of BiVO4 solid spheres; (4) XRD patterns; (5) effect of H2O2 amount on photocatalytic degradation rate of MO in the presence of BiVO4 hollow spheres; (6) PL spectra. See DOI: 10.1039/d0ra00698j |
‡ J. Mao and Q. Wu contributed equally. |
|
This journal is © The Royal Society of Chemistry 2020 |
Click here to see how this site uses Cookies. View our privacy policy here.