DOI:
10.1039/D0RA00684J
(Paper)
RSC Adv., 2020,
10, 16101-16109
Ring-opening cyclization of activated spiro-aziridine oxindoles with heteroarenes: a facile synthetic approach to spiro-oxindole-fused pyrroloindolines†
Received
22nd January 2020
, Accepted 30th March 2020
First published on 24th April 2020
Abstract
Herein, we report a facile tandem approach for the synthesis of both spiro-oxindole-fused pyrroloindolines and benzofurano-pyrrolidines via a Lewis acid-catalyzed domino ring-opening with concomitant ring annulation using activated spiro-aziridines and heteroarenes. This method offers a new class of novel spiro-fused polycyclic pyrrolidines in a one-pot and sustainable manner with good yields and high diastereoselectivity. In addition, the structure of 3d was confirmed by single X-ray crystallography analysis.
Introduction
The use of natural products as drug leads has resulted in great demand for the synthetic community to develop effective strategies for the single-step synthesis of rare complicated heterocycles. Spiro-fused polycyclic pyrrolidine frameworks are the core skeletons of various architecturally complex molecules and natural product-like compounds as potential drug candidates.1 Accordingly, direct access to spiro-fused polycyclic pyrrolidine derivatives in the minimum number of steps is an easily expanded approach for very quick optimization of their biological properties. Thus, versatile synthetic strategies have been developed, e.g., [3 + 2] cycloaddition,2 Pictet–Spengler,3 Morita–Baylis–Hillman,4 and Michael/Mannich [3 + 2] cycloaddition reactions.5 The Lewis acid-catalyzed cascade annulation of heteroarenes has gained considerable attention for the development of fused pyrrolidines based on the tethered built-in nucleophilicity (ring-opening of aziridine) on the C-3 position and electrophilicity (intramolecular annulation) on the C-2 position of heteroarenes, thereby providing considerable synthetic benefits from the viewpoint of easy availability and accessibility to react with distinct reaction partners.6
Indole and benzofuran are the most important class of heteroarenes, exhibiting a broad spectrum of biological activities such as anti-tumor, analgesic, anti-microbial, anti-malarial, anti-diabetic, anti-tubercular, anti-HIV, and anti-oxidant activity, and thus are considered important templates for drug discovery.7 Simultaneously, (hetero)arene-annulated tricyclic pyrrolidine frameworks are frequently encountered in numerous natural products and biologically significant molecules such as physostigmine and physovenine as acetyl cholinesterase inhibitors, and (−)-flustramine B as an anticancer agent (Fig. 1).8 In addition, spiro-fused pyrrolidine functionalization at the C-3 position of oxindole has occupied a remarkable position in synthetic chemistry. A large group of diverse skeletons of spiro-fused pyrrolidines exists in natural products such as spirotryprostatine A and B, elacomine, and horsifiline, with various types of bioactivities as anti-tumor, anti-microbial and anti-malarial agents.9
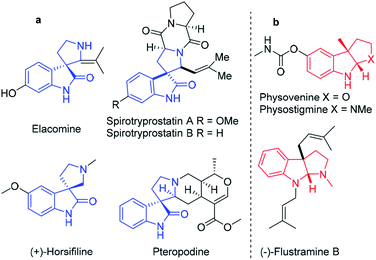 |
| Fig. 1 (a) Spiro-oxindole-fused mono and tricyclic pyrrolidine alkaloid natural products and (b) heteroarene-fused tricyclic pyrrolidine natural products. | |
Although the abovementioned reactions have made a significant contribution, the domino ring-opening and dearomative cyclization of activated aziridine with heteroarenes in the presence of a Lewis acid is fascinating. Owing to the rapid access to stereoselective heteroarene-annulated polycyclic derivatives and advances in the synthesis of natural products, this specific transformation has attracted attention from synthetic chemists.10 In 2014, Wang and co-workers reported an asymmetric [3 + 2] cycloaddition for the construction of pyrroloindolines mediated by the in situ generation of a magnesium catalyst.11 Subsequently, in 2015, Chai and co-workers established a copper-catalyzed [3 + 2] annulation of indoles with 2-arylaziridines, which could concisely furnish pyrroloindolines bearing multiple contiguous stereogenic centers with excellent regio-, diastereo- and enantioselectivity in one synthetic operation (Scheme 1a).12 Recently, the catalyst-free “on-water” regio- and stereospecific ring-opening of spiro-aziridine oxindole was described by Hajra and co-workers to give enantiopure unsymmetrical 3,3′-bisindoles (Scheme 1b).13
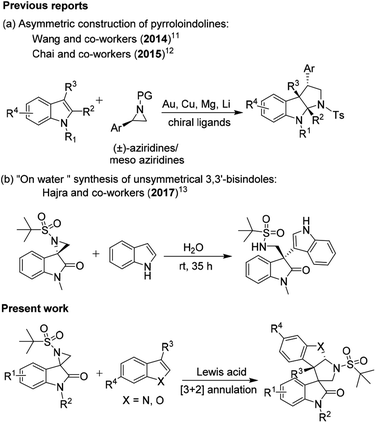 |
| Scheme 1 Lewis acid-catalyzed ring-opening of spiro-oxindole aziridines with heteroarenes. | |
Based on these established methods, in continuation of our research interest in the synthesis of spiro-oxindole derivatives,14 herein, we report the Lewis acid-catalyzed domino ring-opening (Friedel–Craft-type C–C bond formation) of activated spiro-aziridine oxindole with heteroarenes followed by intramolecular C-2 annulation. Although the ring-opening version of this reaction was promoted by copper and scandium triflates with moderate yields, C2 annulation was promoted using BF3·OEt2 as a Lewis acid with good control of the diastereoselectivity. Irrespective of C3 substitution on heteroarenes, the reactions progressed smoothly with excellent regio- and diastereoselectivity.
Results and discussion
As illustrated in Table 1, the feasibility of the proposed domino reaction was first evaluated between activated spiro-aziridineoxindole 1a and indole 2a with Sc(OTf)3 as a catalyst; however, the corresponding tetrahydropyrrolo[2,3-b]indole 3a was not obtained, instead it gave 3,3′-bisindoles at room temperature (entries 1 and 5, Table 1). Lewis acids such as Bi(OTf)3, Yb(OTf)3 and the less acidic Cu(OTf)2 also failed to afford the desired product 3a (entries 2–4, Table 2). To check the impact of Sc(OTf)3 on the intramolecular cyclization, the reaction was performed for different reaction times at varying temperature, but we were unsuccessful in obtaining the preferred product 3a (entries 6–9, Table 2). We then investigated the reaction by employing BF3·OEt2 as a Lewis acid for different reaction times at varying temperature (entries 10–14, Table 2). Next, by lowering the temperature to 0 °C, the reaction proceeded smoothly to afford the corresponding product 3a with good yield (82%) and enhanced diastereoselectivity (dr: 9
:
1) (entry 9, Table 2). An increase in the catalyst loading up to 20 mol%, did not affect the reaction yield to a great extent. Thus, the use of 10 mol% of BF3·OEt2 in CH2Cl2 at 0 °C (entry 9, Table 1) was found to be the optimum reaction conditions for this transformation. The strength of the Lewis acid critically influenced the formation of 3′a and 3a. Co-ordination of the Lewis acid on the nitrogen atom of the heteroarene was promoted by BF3·OEt2 because of its binding nature towards the weak bases.
Table 1 Optimization of the reaction conditionsa
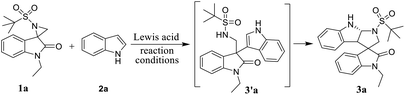
|
Entry |
Catalyst (10 mol%) |
Solvent |
Temp. (°C) |
Time (h) |
Yieldb (%) |
3′a |
3a |
All reactions were performed with 1.0 mmol of 1a and 1.0 mmol of 2a in (5 mL) of solvent in the presence of a Lewis acid catalyst (10 mol%) at room temperature. Isolated yields. The reaction was performed at 0 °C. TLC was not clear. The reaction was carried out at 80 °C. Nr: no reaction. |
1 |
Sc(OTf)3 |
CH3CN |
25 |
4 |
46 |
0 |
2 |
Bi(OTf)3 |
CH3CN |
25 |
12 |
25 |
0 |
3 |
Yb(OTf)3 |
CH3CN |
25 |
10 |
Nr |
Nr |
4 |
Cu(OTf)2 |
CH3CN |
25 |
6 |
35 |
0 |
5 |
Sc(OTf)3 |
CH2Cl2 |
25 |
7 |
40 |
Trace |
6 |
Sc(OTf)3 |
CH2Cl2 |
0 |
30 min |
0 |
0 |
7 |
Sc(OTf)3 |
CH3CN |
80 |
30 min |
20 |
0 |
8 |
Sc(OTf)3 |
CH3CN |
80 |
4 |
0d |
|
9 |
Sc(OTf)3 |
CH2Cl2 |
40 |
4 |
30 |
0 |
10 |
BF3·OEt2 |
CH3CN |
25 |
3 |
0 |
35 |
11e |
BF3·OEt2 |
CH3CN |
80 |
3 |
0 |
Trace |
12 |
BF3·OEt2 |
CH2Cl2 |
25 |
30 min |
0 |
45 |
13c |
BF3·OEt2 |
CH2Cl2 |
0 |
5 min |
0 |
82 |
14c |
BF3·OEt2 (20) |
CH2Cl2 |
0 |
5 min |
0 |
80 |
Table 2 Lewis acid-catalyzed domino ring-opening and annulation reaction of spiro-oxindole aziridines with indolesa
Reactions were performed with 1.0 mmol of 1a and 1.0 mmol of 2a in CH2Cl2 (5 mL) in the presence of BF3·OEt2 (10 mol%) at 0 °C for 5–10 min. |
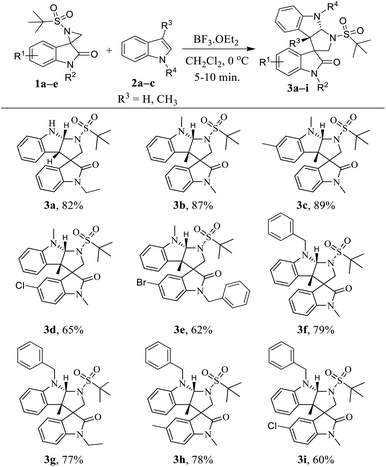 |
With the optimized reaction conditions in hand, we next generalized the protocol with regard to different spiro-aziridine oxindole derivatives and 3-methyl indole, and the corresponding substituted tetrahydropyrrolo[2,3-b]indole products were obtained in moderate to good yields (Table 2). Spiro-aziridines 1a–e, derived from substituted isatins, were prepared according to the previous literature methods.15 The results showed that both the electron-donating and electron-withdrawing functional groups were well tolerated to give the desired products 3a–i. For example, the electron-neutral and donating substituents (R1, e.g. H and CH3 in 3a–c and 3f–h, respectively) on the C5 position of oxindole reacted much faster with better yields (77–89%) than that with electron with-drawing groups (R1, e.g. Cl and Br in 3d, 3e and 3i) (60–65%). Subsequently, for the spiro-aziridines bearing different substituents on the N-atom of oxindole, that with benzyl groups were generally more sluggish (3e, 62% yield) in the reaction than that with ethyl and methyl groups, which is certainly due to bulky effect of benzyl group. Then, we explored the reaction scope with regard to different N-substituted indoles. Notably, the reaction of indole with a free N–H group was more time-consuming compared to that for the N-protected indole.
Then, the same set of reaction parameters were studied to extend the scope of various substituted spiro-aziridine oxindoles with benzofurans, and the results are compiled in Table 3. Under the optimized conditions, the transformation proceeded more smoothly using benzofurans than indole with respect to yield (5a–m, 55–89%) and diastereoselectivity. The reactions furnished the desired tetrahydropyrrolo[2,3-b]benzofurans in moderate to good yield and high diastereoselectivity with different substituents at the C5- and N1- positions of spiro-aziridine oxindoles 5a–i. However, electron-donating substituents (5c and 5k) at the C5-position of oxindole proved to be more efficient in this transformation, proceeding with higher yields (86% and 77%, respectively) than that with electron with-drawing groups (5d–f, 5h, 5i and 5m, 79–55% yield). Particularly, spiro-aziridine bearing a fluoro substituent at the C5-position reacted very slowly, and even after a prolonged reaction time resulted in a low conversion (5d, 59% yield). Interestingly, the oxindole bearing an N-benzyl group was also tolerable in the reaction to afford the corresponding cyclized adducts in comparatively moderate yields (5g–i and 5l, 79–68%) with different electronic nature at the C5-position of oxindole. Furthermore, C5-bromine-substituted benzofurans were tested, and the stereochemical integrity was uniformly maintained regardless of the substituent on the C5 and N1-positions of oxindole, but the yields were altered depending on their electronic nature (5j–l, 81–68%). Unfortunately, C5-brominated N-benzyl oxindole required a higher catalyst loading and longer reaction time to react with C6-bromo benzofuran, and the analogous cyclized product 5m was obtained in poor yield (55%).
Table 3 Lewis acid-catalyzed dearomative domino ring-opening and annulation of spiro-oxindole aziridine with benzofuransa
Reactions were performed with 1.0 mmol of 1a and 1.0 mmol of 4a in CH2Cl2 (5 mL) in the presence of BF3·OEt2 (10 mol%) at 0 °C for 5–10 min. |
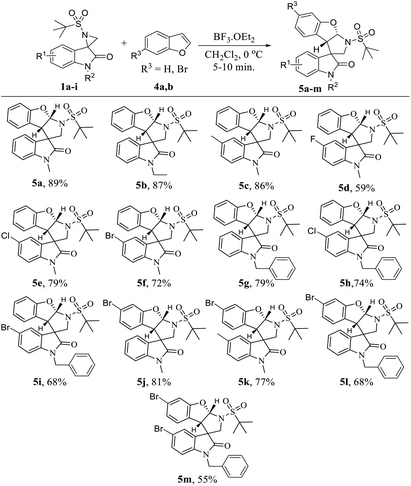 |
To determine the scalability of this method, a gram-scale reaction was performed under the optimized conditions. Satisfyingly, the reaction proceeded smoothly and afforded the desired product 5a in 78% yield (Scheme 2).
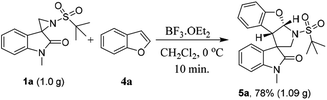 |
| Scheme 2 Gram-scale reaction. | |
The plausible mechanism for the synthesis of tetrahydropyrrolo [2,3-b] indoles 3a–i, and tetrahydropyrrolo [2,3-b] benzofurans 5a–m is depicted in Scheme 3. Specifically, highly reactive aziridine intermediate A is generated via the delocalization of a lone pair of electrons followed by the weakening of the C–N bond of the spiro-aziridine. In this process, the nucleophilic centre (C3) of the heteroarene attacks intermediate A via a Friedel–Crafts-type C–C bond formation, providing iminium/carbonium species B. The co-ordination of the Lewis acid on the heteroatom of the arene ring promotes the intramolecular nucleophilic attack of the nitrogen of the aziridine ring leading, to the formation of the corresponding dearomative cyclized spiro-fused tricyclic pyrrolidine 3 with the dissociation of BF3·OEt2. The stereochemical outcome of one of the cyclized compounds, 3d, was confirmed by single X-ray diffraction analysis (Fig. 2).
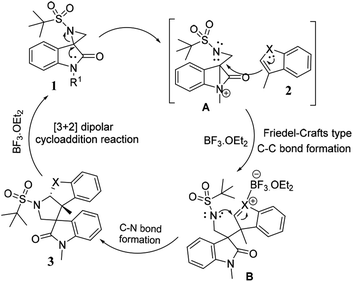 |
| Scheme 3 Plausible reaction mechanism. | |
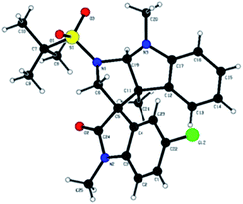 |
| Fig. 2 Single X-ray crystal analysis of compound 3d. | |
In silico DFT calculations
For further insight into mechanistic investigations and defining the different transition states, in silico density functional theory (DFT) calculations were performed using Schrödinger.16 Full geometry optimizations were carried out using the B3LYP method and 6-31G** as the basis set. Single point energy for all the structures including reactants, probable transition states13 (favored and disfavored), intermediates and products were calculated using Jaguar. According to the ΔG values, it was observed that the activation barrier for the formation of the favored and disfavored transition state is 351.38 and 428.38 kcal mol−1 respectively. Fig. 3 clearly presents the energy barrier for the formation of the acyclic intermediate and product via two transition states (TS). Moreover, the energy barrier through route A necessitates additional energy in comparison to route B, which supports the formation of a favourable TS in this reaction.
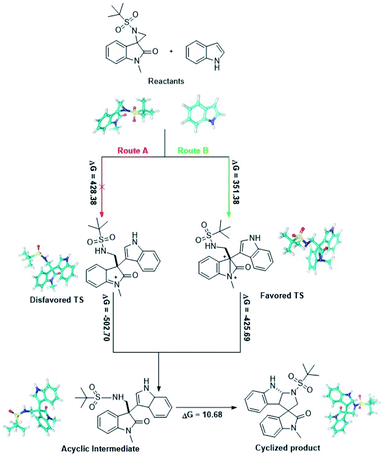 |
| Fig. 3 Optimized B3LYP/6-31G** structures of the reaction; 3D structures represented in cyan color. | |
Conclusion
In summary, we developed a Lewis acid-mediated domino ring-opening with a concomitant annulation strategy for the synthesis of biologically significant spiro-fused tricyclic pyrrolidines. In particular, a variety of heterocyclic nucleophiles was investigated with different electronic nature on the aromatic ring of oxindole, which offered a one-step protocol for the synthesis spirocyclic scaffolds. The present protocol enables facile access to a variety of spiro-oxindole-fused pyrrolidines with distinct substitutions in a highly convergent and diastereoselective manner.
Experimental section
General information
All reagents and solvents were obtained from commercial suppliers and used without further purification. Analytical thin layer chromatography (TLC) was performed on MERCK precoated silica gel 60-F254 (0.5 mm) aluminum plates. Visualization of the spots on the TLC plates was achieved using UV light. 1H and 13C NMR spectra were recorded on a Bruker 500 MHz spectrometer using tetramethylsilane (TMS) as the internal standard. Chemical shifts for 1H and 13C are reported in parts per million (ppm) downfield from tetramethylsilane. Spin multiplicities are described as s (singlet), bs (broad singlet), d (doublet), dd (double doublet), t (triplet), q (quartet), and m (multiplet). Coupling constant (J) values are reported in hertz (Hz). HRMS was performed using an Agilent QTOF 6540 series mass spectrometer. Wherever required, column chromatography was performed using silica gel (60–120 or 100–200) or neutral alumina.
General procedure for the synthesis of dihydrospiro[benzo [e]indole-1,3′-indolin]-2′-one (3a–i) and (5a–m)
A solution of indole (1.0 equiv.) and spiro-oxindole aziridine (1.0 equiv.) was added to 5 mL of dry DCM under an argon atmosphere at 0 °C. Then, a catalytic amount of BF3·OEt2 (10 mol%) was added and the progress of the reaction was monitored by TLC. After completion of the reaction, the suspension was extracted with ethyl acetate (3 × 5.0 mL), and washed with a 1
:
1 mixture of brine. The combined organic extracts were dried over anhydrous sodium sulphate. After removal of the solvent under reduced pressure, the crude product was purified by column chromatography on silica gel to afford the pure product.
1′-(tert-Butylsulfonyl)-1-ethyl-1′,3a′,8′,8a′-tetrahydro-2′H-spiro[indoline-3,3′-pyrrolo[2,3b]indol]-2-one (3a)
White solid; yield: 82%; mp: 204–207 °C; FT-IR (cm−1): 3245, 2925, 1677, 1609, 1462, 1319, 740; 1H NMR (500 MHz, DMSO-d6): δ 11.09 (s, 1H), 7.35 (d, J = 2.2 Hz, 1H), 7.32 (dd, J = 7.7, 3.8 Hz, 1H), 7.19 (d, J = 7.3 Hz, 1H), 7.14 (d, J = 7.8 Hz, 1H), 6.99 (t, J = 7.4 Hz, 1H), 6.85 (d, J = 8.0 Hz, 1H), 6.67 (t, J = 7.5 Hz, 1H), 6.63 (t, J = 6.3 Hz, 1H), 3.99 (d, J = 6.3 Hz, 2H), 3.80–3.77 (m, 2H), 2.89 (s, 1H), 2.73 (s, 1H), 1.22 (t, J = 7.2 Hz, 3H), 1.18 (s, 9H); 13C NMR (125 MHz, DMSO-d6): δ 176.4, 143.6, 137.0, 131.1, 128.7, 125.8, 125.5, 124.1, 122.3, 121.5, 119.6, 119.0, 112.1, 108.7, 59.5, 53.3, 48.6, 34.8, 31.2, 24.5, 13.0; HRMS (ESI): m/z calc. for C23H27N3O3S 426.1851, found 426.1861 [M + H]+.
1′-(tert-Butylsulfonyl)-1,3a′,8′-trimethyl-1′,3a′,8′,8a′-tetrahydro-2′H-spiro[indoline-3,3′-pyrrolo[2,3b]indol]-2-one (3b)
Cream solid; yield: 87%; mp: 201–204 °C; FT-IR (cm−1): 2922, 2852, 1709, 1610, 1308, 1122, 741; 1H NMR (500 MHz, DMSO-d6): δ 7.48 (d, J = 7.1 Hz, 1H), 7.38 (t, J = 8.3 Hz, 1H), 7.13–7.08 (m, 3H), 6.77 (d, J = 7.5 Hz, 1H), 6.62 (t, J = 7.0 Hz, 1H), 6.58 (d, J = 7.8 Hz, 1H), 5.45 (s, 1H), 3.76 (d, J = 10.4 Hz, 1H), 3.44 (d, J = 10.4 Hz, 1H), 3.10 (s, 3H), 3.03 (s, 3H), 1.34 (s, 9H), 0.96 (s, 3H); 13C NMR (125 MHz, DMSO-d6): δ 174.0, 151.4, 144.0, 131.5, 129.2, 129.1, 128.9, 125.4, 125.2, 122.1, 118.0, 109.0, 108.2, 92.2, 61.1, 59.7, 58.7, 56.4, 36.7, 26.7, 24.8, 24.1; HRMS (ESI): m/z calc. for C24H29N3O3S 440.2008, found 440.2000 [M + H]+.
1′-(tert-Butylsulfonyl)-1,3a′,5,8′-tetramethyl-1′,3a′,8′,8a′-tetrahydro-2′H-spiro[indoline-3,3′-pyrrolo[2,3b]indol]-2-one (3c)
White solid; yield: 89%; mp: 205–208 °C; FT-IR (cm−1): 2922, 1709, 1609, 1308, 742; 1H NMR (500 MHz, DMSO-d6): δ 7.05 (t, J = 7.5 Hz, 1H), 7.01 (d, J = 7.7 Hz, 1H), 6.84 (d, J = 7.9 Hz, 1H), 6.60 (t, J = 12.1 Hz, 2H), 6.45 (t, J = 7.3 Hz, 1H), 6.39 (d, J = 7.1 Hz, 1H), 5.34 (s, 1H), 3.79 (d, J = 10.7 Hz, 1H), 3.46 (d, J = 10.7 Hz, 1H), 3.14 (s, 3H), 3.03 (s, 3H), 2.06 (s, 3H), 1.39 (s, 9H), 1.31 (s, 3H); 13C NMR (125 MHz, DMSO-d6): δ 176.3, 151.5, 141.7, 131.7, 130.5, 129.1, 129.0, 127.4, 126.8, 124.4, 117.9, 108.2, 108.2, 93.3, 61.5, 59.0, 56.5, 35.9, 26.6, 24.3, 23.4, 21.0; HRMS (ESI): m/z calc. for C25H31N3O3S 454.2164, found 454.2163 [M + H]+.
1′-(tert-Butylsulfonyl)-5-chloro-1,3a′,8′-trimethyl-1′,3a′,8′,8a′-tetrahydro-2′H-spiro[indole ne-3,3′-pyrrolo[2,3b]indol]-2-one (3d)
White solid; yield: 65%; mp: 205–208 °C; FT-IR (cm−1): 2922, 2852, 1709, 1610, 1308, 1122, 787, 741; 1H NMR (500 MHz, DMSO-d6): δ 7.22 (d, J = 8.1 Hz, 1H), 7.05 (d, J = 9.8 Hz, 1H), 6.97 (d, J = 10.5 Hz, 1H), 6.88 (s, 1H), 6.63 (d, J = 9.2 Hz, 1H), 6.55–6.27 (m, 2H), 5.33 (s, 1H), 3.88 (d, J = 10.1 Hz, 1H), 3.53 (d, J = 8.1 Hz, 1H), 3.18 (s, 3H), 3.04 (s, 3H), 1.43 (s, 9H), 1.25 (s, 3H); 13C NMR (125 MHz, DMSO-d6): δ 151.6, 142.7, 131.4, 130.5, 129.3, 128.4, 125.9, 125.5, 123.2, 118.4, 109.8, 108.6, 93.6, 61.5, 59.2, 58.9, 56.5, 36.1, 26.8, 24.3, 23.2; HRMS (ESI): m/z calc. for C24H28ClN3O3S 474.1618, found 474.1616 [M + H]+.
1-Benzyl-5-bromo-1′-(tert-butylsulfonyl)-3a′,8′-dimethyl-1′,3a′,8′,8a′-tetrahydro-2′H-spiro[indoline-3,3′-pyrrolo[2,3b]indol]-2-one (3e)
White solid; yield: 62%; mp: 206–208 °C; FT-IR (cm−1): 2977, 1713, 1609, 1487, 1308, 994, 752; 1H NMR (500 MHz, DMSO-d6): δ 7.35 (s, 1H), 7.34 (d, J = 9.6 Hz, 1H), 7.32–7.29 (m, 3H), 7.24 (t, J = 9.3 Hz, 2H), 7.11 (t, J = 7.6 Hz, 1H), 6.92 (d, J = 8.4 Hz, 1H), 6.71 (d, J = 7.4 Hz, 1H), 6.59 (t, J = 8.7 Hz, 2H), 5.48 (s, 1H), 4.96 (d, J = 15.9 Hz, 1H), 4.75 (d, J = 16.0 Hz, 1H), 3.88 (d, J = 10.6 Hz, 1H), 3.50 (d, J = 4.6 Hz, 1H), 3.02 (s, 3H), 1.35 (s, 9H), 1.03 (s, 3H); 13C NMR (125 MHz, DMSO-d6): δ 173.7, 151.5, 142.5, 136.4, 131.7, 131.0, 129.1, 128.6, 127.9, 127.6, 125.2, 118.2, 114.4, 111.5, 108.3, 92.2, 61.2, 60.0, 59.2, 56.5, 43.7, 36.8, 25.0, 24.2, 21.3, 14.5; HRMS (ESI): m/z calc. for C30H32BrN3O3S 596.1406, found 596.1401 [M + 2H]+.
8′-Benzyl-1′-(tert-butylsulfonyl)-1,3a′-dimethyl-1′,3a′,8′,8a′-tetrahydro-2′H-spiro[indoline-3,3′-pyrrolo[2,3b]indol]-2-one (3f)
Cream solid; yield: 79%; mp: 251–254 °C; FT-IR (cm−1): 2983, 1711, 1611, 1306, 748; 1H NMR (500 MHz, DMSO-d6): δ 7.35 (d, J = 7.4 Hz, 1H), 7.24 (t, J = 7.7 Hz, 1H), 7.18 (d, J = 4.3, 4H), 7.11–7.09 (m, 1H), 7.08 (dd, J = 13.6, 7.8 Hz, 3H), 6.82 (d, J = 7.3 Hz, 1H), 6.75 (d, J = 7.9 Hz, 1H), 6.59 (t, J = 7.4 Hz, 1H), 5.67 (s, 1H), 4.78 (d, J = 15.7 Hz, 1H), 4.62 (d, J = 15.8 Hz, 1H), 3.72 (d, J = 10.4 Hz, 1H), 3.43 (d, J = 10.5 Hz, 1H), 3.12 (s, 3H), 1.35 (s, 9H), 0.58 (s, 3H); 13C NMR (125 MHz, DMSO-d6): δ 173.7, 149.4, 143.8, 138.7, 131.4, 129.4, 129.0, 128.8, 128.3, 127.7, 126.0, 125.1, 122.3, 118.0, 109.1, 108.8, 89.0, 61.1, 59.8, 58.7, 56.7, 50.4, 26.8, 24.2, 23.3; HRMS (ESI): m/z calc. for C30H33N3O3S 516.2321, found 516.2325 [M + H]+.
8′-Benzyl-1′-(tert-butylsulfonyl)-1-ethyl-3a′-methyl-1′,3a′,8′,8a′-tetrahydro-2′H-spiro[indoline-3,3′-pyrrolo[2,3b]indol]-2-one (3g)
White solid; yield: 77%; mp: 185–188 °C; FT-IR (cm−1): 2973, 1721, 1610, 1484, 1305, 731; 1H NMR (500 MHz, DMSO-d6): δ 7.44 (d, J = 7.3 Hz, 1H), 7.35 (t, J = 7.5 Hz, 1H), 7.25 (d, J = 3.7 Hz, 4H), 7.19 (s, 1H), 7.14–7.05 (m, 3H), 6.77 (d, J = 7.3 Hz, 1H), 6.73 (d, J = 7.8 Hz, 1H), 6.59 (t, J = 7.2 Hz, 1H), 5.67 (s, 1H), 4.77 (d, J = 15.8 Hz, 1H), 4.60 (d, J = 15.8 Hz, 1H), 3.75 (d, J = 10.0 Hz, 1H), 3.62–3.57 (m, 2H), 3.46 (d, J = 10.4 Hz, 1H), 1.36 (s, 9H), 1.12 (t, J = 6.8 Hz, 3H), 0.62 (s, 3H); 13C NMR (125 MHz, DMSO-d6): δ 173.5, 149.5, 142.8, 138.7, 131.4, 129.4, 129.1, 128.8, 128.7, 128.2, 127.6, 125.7, 125.5, 122.1, 118.0, 109.1, 108.8, 89.2, 61.1, 59.6, 58.7, 56.6, 50.5, 34.7, 24.2, 23.3, 12.9; HRMS (ESI): m/z calc. for C31H35N3O3S 530.2477, found 530.2492 [M + H]+.
8′-Benzyl-1′-(tert-butylsulfonyl)-1,3a′,5-trimethyl-1′,3a′,8′,8a′-tetrahydro-2′H-spiro[indoline-3,3′-pyrrolo[2,3b]indol]-2-one (3h)
White solid; yield: 78%; mp: 195–198 °C; FT-IR (cm−1): 2923, 2853, 1712, 1600, 1465, 1307, 1102, 709; 1H NMR (500 MHz, DMSO-d6): δ 7.25 (t, J = 4.1 Hz, 5H), 7.20–7.16 (m, 2H), 7.06 (t, J = 7.7 Hz 1H), 6.96 (d, J = 7.9 Hz, 1H), 6.80 (d, J = 7.5 Hz 1H), 6.69 (d, J = 7.9 Hz, 1H), 6.58 (t, J = 7.8 Hz, 1H), 5.71 (s, 1H), 4.76 (d, J = 15.9 Hz, 1H), 4.64 (d, J = 15.9 Hz, 1H), 3.69 (d, J = 10.4 Hz, 1H), 3.44 (d, J = 10.4 Hz, 1H), 3.09 (s, 3H), 2.31 (s, 3H), 1.35 (s, 9H), 0.65 (s, 3H); 13C NMR (125 MHz, DMSO-d6): δ 173.7, 149.2, 141.5, 138.7, 131.4, 131.1, 129.4, 129.1, 128.7, 128.7, 128.2, 127.6, 126.1, 125.9, 117.9, 108.7, 108.6, 89.2, 61.1, 59.6, 58.8, 56.7, 50.3, 26.8, 24.2, 23.5, 21.4; HRMS (ESI): m/z calc. for C31H35N3O3S 530.2477, found 530.2472 [M + H]+.
8′-Benzyl-1′-(tert-butylsulfonyl)-5-chloro-1,3a′-dimethyl-1′,3a′,8′,8a′-tetrahydro-2′H-spiro[indoline-3,3′-pyrrolo[2,3b]indol]-2-one (3i)
White solid; yield: 60%; mp: 184–187 °C; FT-IR (cm−1): 2980, 2928, 1719, 1605, 1488, 1308, 748; 1H NMR (500 MHz, DMSO-d6): δ 7.31–7.26 (m, 5H), 7.24 (t, J = 6.7 Hz, 1H), 7.04 (t, J = 7.3 Hz, 1H), 7.00 (d, J = 8.4 Hz, 1H), 6.92 (s, 1H), 6.68 (d, J = 7.9 Hz, 1H), 6.49 (t, J = 7.3 Hz, 1H), 6.45 (d, J = 7.1 Hz, 1H), 5.60 (s, 1H), 4.90 (d, J = 15.8 Hz, 1H), 4.54 (d, J = 15.9 Hz, 1H), 3.83 (d, J = 10.9 Hz, 1H), 3.58 (d, J = 10.9 Hz, 1H), 3.16 (s, 3H), 1.40 (s, 9H), 1.09 (s, 3H); 13C NMR (125 MHz, DMSO-d6): δ 175.8, 150.3, 143.1, 139.0, 131.4, 129.2, 128.8, 128.7, 128.0, 127.5, 126.1, 125.9, 124.5, 118.2, 110.0, 109.2, 90.4, 61.4, 59.1, 59.0, 56.1, 51.0, 26.7, 24.3, 22.6; HRMS (ESI): m/z calc. for C30H32ClN3O3S 550.1931, found 550.1929 [M + H]+.
1-(tert-Butylsulfonyl)-1′-methyl-1,2,3a,8a-tetrahydrospiro[benzofuro[2,3b]pyrrole-3,3′-indolin]-2′-one (5a)
White solid; yield: 89%; mp: 233–236 °C; FT-IR (cm−1): 2981, 2920, 1721, 1609, 1471, 1315, 753; 1H NMR (500 MHz, DMSO-d6): δ 7.52 (d, J = 7.1 Hz, 1H), 7.45–7.36 (m, 2H), 7.28 (t, J = 7.6 Hz, 1H), 7.16–7.06 (m, 2H), 6.94 (t, J = 7.4 Hz, 1H), 6.86 (d, J = 8.0 Hz, 1H), 5.97 (d, J = 6.9 Hz, 1H), 5.21 (d, J = 6.9 Hz, 1H), 3.84 (d, J = 10.9 Hz, 1H), 3.60 (d, J = 10.9 Hz, 1H), 3.17 (s, 3H), 1.24 (d, 9H); 13C NMR (125 MHz, DMSO-d6): δ 172.2, 160.5, 143.6, 130.9, 129.4, 129.2, 127.6, 125.9, 123.8, 122.7, 121.0, 110.4, 109.1, 90.9, 66.5, 60.7, 56.7, 56.1, 26.8, 24.4; HRMS (ESI): m/z calc. for C22H24N3O3S 413.1535, found 413.1533 [M + H]+.
1-(tert-Butylsulfonyl)-1′-ethyl-1,2,3a,8a-tetrahydrospiro[benzofuro[2,3b]pyrrole-3,3′-indolin]-2′-one (5b)
White solid; yield: 87%; mp: 185–188 °C; FT-IR (cm−1): 3245, 2925, 1677, 1609, 1462, 1319, 1034, 740; 1H NMR (500 MHz, DMSO-d6): δ 7.53 (d, J = 7.4 Hz, 1H), 7.43 (d, J = 7.4 Hz, 1H), 7.38 (t, J = 7.7 Hz, 1H), 7.29 (t, J = 7.7 Hz, 1H), 7.13 (dd, J = 17.4, 7.7 Hz, 2H), 6.95 (t, J = 7.4 Hz, 1H), 6.87 (d, J = 8.1 Hz, 1H), 5.97 (d, J = 6.9 Hz, 1H), 5.21 (d, J = 6.9 Hz, 1H), 3.84 (d, J = 10.0 Hz, 1H), 3.80–3.68 (m, 2H), 3.61 (d, J = 10.9 Hz, 1H), 1.24 (s, 9H), 1.18 (t, J = 7.1 Hz, 3H); 13C NMR (125 MHz, DMSO-d6): δ 171.8, 160.5, 142.5, 130.9, 129.4, 129.2, 127.6, 126.0, 124.0, 122.6, 121.0, 110.3, 109.1, 91.0, 66.3, 60.6, 56.6, 55.9, 34.8, 24.4, 12.9; HRMS (ESI): m/z calc. for C23H26N2O4S427.1692, found 427.1688 [M + H]+.
1-(tert-Butylsulfonyl)-1′,5′-dimethyl-1,2,3a,8a-tetrahydrospiro[benzofuro[2,3b]pyrrole-3,3′-indolin]-2′-one (5c)
Cream solid; yield: 86%; mp: 210–214 °C; FT-IR (cm−1): 2923, 2858, 1704, 1620, 1499, 1308, 1129, 750; 1H NMR (500 MHz, DMSO-d6): δ 7.52 (d, J = 7.1 Hz, 1H), 7.33–7.22 (m, 2H), 7.19 (d, J = 8.5 Hz, 1H), 7.01–6.90 (m, 2H), 6.85 (t, J = 8.1 Hz, 1H), 5.96 (d, J = 6.9 Hz, 1H), 5.19 (d, J = 6.9 Hz, 1H), 3.80 (d, J = 10.8 Hz, 1H), 3.59 (d, J = 10.8 Hz, 1H), 3.14 (s, 3H), 2.31 (s, 3H), 1.23 (s, 9H); 13CNMR (125 MHz, DMSO-d6): δ 172.2, 160.5, 141.2, 131.7, 130.9, 129.4, 129.3, 127.6, 126.0, 124.6, 121.0, 110.3, 108.8, 90.9, 66.4, 60.7, 56.7, 56.0, 26.8, 24.4, 21.5; HRMS (ESI): m/z calc. for C23H26N2O4S is 427.1692, found 427.1682 [M + H]+.
1-(tert-Butylsulfonyl)-5′-fluoro-1′-methyl-1,2,3a,8a-tetrahydrospiro[benzofuro[2,3b]pyrrole-3,3′-indolin]-2′-one (5d)
White solid; yield: 59%; mp: 234–236 °C; FT-IR (cm−1): 2923, 2858, 1704, 1620, 1499, 1308, 1263, 750; 1H NMR (500 MHz, DMSO-d6): δ 7.52 (d, J = 7.3 Hz, 1H), 7.31–7.24 (m, 3H), 7.10–7.08 (m, 1H), 6.95 (t, J = 7.3 Hz, 1H), 6.86 (d, J = 8.0 Hz, 1H), 6.00 (d, J = 7.0 Hz, 1H), 5.25 (d, J = 7.0 Hz, 1H), 3.87 (d, J = 11.0 Hz, 1H), 3.60 (d, J = 11.0 Hz, 1H), 3.16 (s, 3H), 1.25 (s, 9H); 13C NMR (125 MHz, DMSO-d6): δ 172.1, 160.5, 159.6, 157.7 (d, JC–F = 237.2 Hz), 140.0, 131.0, 130.7 (d, JC–F = 8.4 Hz), 127.6, 125.8, 121.2, 115.5 (d, JC–F = 23.1 Hz), 112.2 (d, JC–F = 25.8 Hz), 110.4, 109.9 (d, JC–F = 8.0 Hz), 90.6, 66.3, 60.7, 57.1, 55.9, 27.0, 24.4; HRMS (ESI): m/z calc. for C22H23FN2O4S 431.1441, found 431.1438 [M + H]+.
1-(tert-Butylsulfonyl)-5′-chloro-1′-methyl-1,2-dihydrospiro[benzofuro[2,3b]pyrrole-3,3′-indolin]-2′-one (5e)
Off-white solid; yield: 79%; mp: 227–230 °C; FT-IR, (cm−1): 3340, 2923, 1726, 1477, 1114, 763; 1H NMR (500 MHz, DMSO-d6): δ 7.52 (d, J = 7.3 Hz, 1H), 7.49 (d, J = 1.9 Hz, 1H), 7.47–7.45 (m, 1H), 7.28 (t, J = 8.2 Hz, 1H), 7.12 (d, J = 8.3 Hz, 1H), 6.96 (t, J = 7.3 Hz, 1H), 6.86 (d, J = 8.1 Hz, 1H), 6.00 (d, J = 7.0 Hz, 1H), 5.27 (d, J = 7.0 Hz, 1H), 3.88 (d, J = 11.0 Hz, 1H), 3.60 (d, J = 11.1 Hz, 1H), 3.16 (s, 3H), 1.25 (s, 9H); 13C NMR (125 MHz, DMSO-d6): δ 171.9, 160.3, 142.7, 131.1, 130.9, 129.1, 127.6, 126.7, 125.7, 124.2, 121.2, 110.5, 110.4, 90.6, 66.3, 60.7, 56.9, 55.9, 27.0, 24.4; HRMS (ESI): m/z calc. for C22H23ClN2O4S is 447.1145, found 447.1142 [M + H]+.
5′-Bromo-1-(tert-butylsulfonyl)-1′-methyl-1,2,3a,8a-tetrahydrospiro[benzofuro[2,3b]pyrrole-3,3′-indolin]-2′-one (5f)
White solid; yield: 72%; mp: 231–234 °C; FT-IR (cm−1): 3340, 3245, 2923, 1726, 1477, 1300, 997, 662; 1H NMR (500 MHz, DMSO-d6): δ 7.60–7.57 (m, 2H), 7.52 (d, J = 6.9 Hz, 1H), 7.29 (t, J = 7.1 Hz, 1H), 7.07 (d, J = 8.0 Hz, 1H), 6.95 (t, J = 6.8 Hz, 1H), 6.86 (d, J = 7.7 Hz, 1H), 5.98 (d, J = 6.6 Hz,1H), 5.27 (d, J = 6.6 Hz, 1H), 3.88 (d, J = 11.0 Hz, 1H), 3.60 (d, J = 10.9 Hz, 1H), 3.15 (s, 3H), 1.25 (s, 9H); 13C NMR (125 MHz, DMSO-d6): δ 171.8, 160.4, 143.1, 132.0, 131.5, 130.9, 127.6, 126.9, 125.7, 121.1, 114.4, 111.0, 110.3, 90.5, 66.4, 60.7, 56.9, 55.9, 26.9, 24.4; HRMS (ESI): m/z calc. for C22H23BrN2O4S 493.0620, found 493.0618 [M + 2H]+.
1′-Benzyl-1-(tert-butylsulfonyl)-1,2,3a,8a-tetrahydrospiro[benzofuro[2,3b]pyrrole-3,3′-indolin]-2′-one (5g)
White solid; yield: 79%; mp: 228–231 °C; FT-IR (cm−1): 2965, 2935, 1713, 1729, 1466, 1307, 1128, 745; 1H NMR (500 MHz, DMSO-d6): δ 7.56 (d, J = 7.3 Hz, 1H), 7.49 (d, J = 7.4 Hz, 1H), 7.37–735 (m, 4H), 7.29 (t, J = 7.1 Hz, 3H), 7.11 (t, J = 7.5 Hz, 1H), 6.96 (t, J = 7.9 Hz, 2H), 6.86 (d, J = 8.1 Hz, 1H), 5.99 (d, J = 7.3 Hz, 1H), 5.34 (d, J = 7.3 Hz, 1H), 5.02 (d, J = 15.9 Hz, 1H), 4.85 (d, J = 15.9 Hz, 1H), 3.93 (d, J = 10.8 Hz, 1H), 3.68 (d, J = 10.8 Hz, 1H), 1.27 (s, 9H); 13C NMR (125 MHz, DMSO-d6): δ 172.7, 160.5, 142.6, 136.6, 130.9, 129.4, 129.1, 128.9, 127.8, 127.8, 127.5, 125.9, 124.0, 122.9, 121.0, 110.2, 109.6, 90.8, 66.1, 60.7, 56.9, 55.8, 43.3, 24.4; HRMS (ESI): m/z calc. for C28H28N2O4S 489.1848, found 489.1846 [M + H]+.
1′-Benzyl-1-(tert-butylsulfonyl)-5′-chloro-1,2,3a,8a-tetrahydrospiro[benzofuro[2,3b]pyrrole-3,3′-indolin]-2′-one (5h)
White solid; yield: 74%; mp: 231–234 °C; FT-IR (cm−1): 3044, 2845, 1615, 1588, 1373, 1284, 834, 722, 585; 1H NMR (500 MHz, DMSO-d6): δ 7.58 (d, J = 6.3 Hz, 3H), 7.37 (m, 6H), 6.96 (d, J = 6.9 Hz, 2H), 6.86 (d, J = 7.0 Hz, 1H), 6.01 (d, J = 6.4 Hz, 1H), 5.39 (d, J = 6.0 Hz, 1H), 5.02 (d, J = 15.6 Hz, 1H), 4.84 (d, J = 16.5 Hz, 1H), 3.98 (d, J = 10.6 Hz, 1H), 3.67 (d, J = 11.2 Hz, 1H), 1.29 (s, 9H); 13C NMR (125 MHz, DMSO-d6): δ 172.4, 160.5, 141.6, 136.3, 130.9, 130.8, 129.1, 127.9, 127.7, 127.5, 127.0, 125.7, 124.5, 121.1, 111.0, 110.2, 90.4, 66.0, 60.8, 57.2, 55.6, 43.4, 24.4; HRMS (ESI): m/z calc. for C28H27ClN2O4S 523.1458, found 525.1452 [M + H]+.
1′-Benzyl-5′-bromo-1-(tert-butylsulfonyl)-1,2,3a,8a-tetrahydrospiro[benzofuro[2,3b]pyrrole-3,3′-indolin]-2′-one (5i)
White solid; yield: 68%; mp: 224–227 °C; FT-IR, (cm−1): 2970, 2113, 1737, 1709, 1305, 1119, 758; 1H NMR (500 MHz, DMSO-d6): δ 7.69 (d, J = 1.8 Hz, 1H), 7.55 (d, J = 7.4 Hz, 1H), 7.48 (dd, J = 8.3, 1.9 Hz, 1H), 7.34 (dd, J = 13.4, 6.9 Hz, 4H), 7.31–7.25 (m, 2H), 6.95 (t, J = 7.4 Hz, 1H), 6.90 (d, J = 8.4 Hz, 1H), 6.84 (d, J = 8.0 Hz, 1H), 5.98 (d, J = 7.4 Hz, 1H), 5.38 (d, J = 7.4 Hz, 1H), 5.00 (d, J = 16.0 Hz, 1H), 4.82 (d, J = 15.9 Hz, 1H), 3.97 (d, J = 10.9 Hz, 1H), 3.65 (d, J = 10.9 Hz, 1H), 1.27 (s, 9H); 13C NMR (125 MHz, DMSO-d6): δ 172.3, 160.5, 142.1, 136.3, 131.9, 131.2, 130.9, 129.1, 127.9, 127.7, 127.5, 127.2, 125.7, 121.1, 114.7, 111.5, 110.2, 90.5, 66.1, 60.86, 57.2, 55.9, 43.4, 24.4; HRMS (ESI): m/z calc. for C28H27BrN2O4S 569.0933, found 569.0930 [M + 2H]+.
6-Bromo-1-(tert-butylsulfonyl)-1′-methyl-1,2,3a,8a-tetrahydrospiro[benzofuro[2,3b]pyrrole-3,3′-indolin]-2′-one (5j)
White solid; yield 81%; mp: 231–234 °C; FT-IR (cm−1): 2972, 1713, 1613, 1466, 1315, 1282, 826, 746, 507; 1H NMR (500 MHz, DMSO-d6): δ 7.73 (s, 1H), 7.49 (d, J = 8.1 Hz, 1H), 7.44–7.35 (m, 1H), 7.21 (d, J = 6.8 Hz, 1H), 7.15–7.06 (m, 2H), 6.91 (d, J = 8.2 Hz, 1H), 5.84 (d, J = 6.1 Hz, 1H), 5.35 (d, J = 6.1 Hz, 1H), 3.84 (d, J = 10.9 Hz, 1H), 3.60 (d, J = 10.8 Hz, 1H), 3.19 (s, 3H), 1.32 (s, 9H); 13C NMR (125 MHz, DMSO-d6): δ 175.3, 159.4, 144.6, 133.6, 130.7, 129.6, 129.2, 126.2, 125.0, 122.6, 112.5, 112.4, 109.2, 89.1, 66.6, 61.6, 56.6, 55.6, 26.8, 24.3; HRMS (ESI): m/z calc. for C22H23BrN2O4S 493.0620, found 493.0618 [M + 2H]+.
6-Bromo-1-(tert-butylsulfonyl)-1′,5′-dimethyl-1,2,3a,8a-tetrahydrospiro[benzofuro[2,3b] pyrrole-3,3′-indolin]-2′-one (5k)
White solid; yield: 77%; mp: 228–231 °C; FT-IR (cm−1): 3339, 2970, 2883, 1719, 1467, 1128, 950, 816, 595; 1H NMR (500 MHz, DMSO-d6): δ 7.63 (d, J = 2.0 Hz, 1H), 7.44 (dd, J = 8.6, 2.2 Hz, 1H), 7.29 (s, 1H), 7.20 (d, J = 7.8 Hz, 1H), 6.96 (d, J = 7.9 Hz, 1H), 6.84 (d, J = 8.6 Hz, 1H), 5.94 (d, J = 7.3 Hz, 1H), 5.31 (d, J = 7.3 Hz, 1H), 3.79 (d, J = 10.7 Hz, 1H), 3.59 (d, J = 10.7 Hz, 1H), 3.12 (s, 3H), 2.32 (s, 3H), 1.29 (s, 9H); 13C NMR (125 MHz, DMSO-d6): δ 172.3, 159.9, 141.3, 133.3, 131.8, 130.3, 129.6, 128.9, 128.5, 124.5, 112.4, 111.8, 108.8, 91.2, 65.7, 60.9, 56.7, 56.0, 26.8, 24.4, 21.2; HRMS (ESI): m/z calc. for C23H25BrN2O4S 507.0776, found 507.0774 [M + 2H]+.
1′-Benzyl-6-bromo-1-(tert-butylsulfonyl)-1,2,3a,8a-tetrahydrospiro[benzofuro[2,3b]pyrrole-3,3′-indolin]-2′-one (5l)
White solid; yield: 68%; mp: 245–248 °C; FT-IR (cm−1): 2970, 2113, 1737, 1709, 1305, 1119, 758; 1H NMR (500 MHz, DMSO-d6): δ 7.65 (s, 1H), 7.52 (d, J = 5.3 Hz, 1H), 7.43 (d, J = 6.5 Hz, 1H), 7.31 (m, 6H), 7.10 (s, 1H), 6.93 (d, J = 5.5 Hz, 1H), 6.81 (d, J = 5.9 Hz, 1H), 5.95 (d, J = 4.2 Hz, 1H), 5.44 (d, J = 4.7 Hz, 1H), 5.00 (d, J = 16.1 Hz, 1H), 4.78 (d, J = 14.7 Hz, 1H), 3.90 (d, J = 9.7 Hz, 1H), 3.65 (d, J = 9.4 Hz, 1H), 1.29 (s, 9H); 13C NMR (125 MHz, DMSO-d6): δ 172.9, 160.0, 142.8, 136.6, 133.3, 130.4, 129.5, 129.1, 128.7, 128.3, 127.8, 127.5, 124.0, 123.0, 112.3, 111.9, 109.6, 90.9, 65.3, 60.9, 57.0, 55.7, 43.2, 24.4; HRMS (ESI): m/z calc. for C28H27BrN2O4S 569.0933, found 569.0928 [M + 2H]+.
1′-Benzyl-5′,6-dibromo-1-(tert-butylsulfonyl)-1,2,3a,8a-tetrahydrospiro[benzofuro[2,3b]pyrrole-3,3′-indolin]-2′-one (5m)
White solid; yield: 55%; mp:242–245 °C; FT-IR (cm−1): 3416, 2942, 2821, 2251, 1682, 1023, 758; 1H NMR (500 MHz, DMSO-d6): δ 7.78 (s, 1H), 7.67 (s, 1H), 7.50 (d, J = 7.0 Hz, 1H), 7.46 (d, J = 8.2 Hz, 1H), 7.35–7.29 (m, 5H), 6.92 (d, J = 7.6 Hz, 1H), 6.82 (d, J = 8.6 Hz, 1H), 5.95 (d, J = 7.5 Hz, 1H), 5.51 (d, J = 6.9 Hz, 1H), 5.02 (d, J = 15.6 Hz, 1H), 4.79 (d, J = 15.6 Hz, 1H), 3.96 (d, J = 10.2 Hz, 1H), 3.67 (d, J = 10.3 Hz, 1H), 1.31 (s, 9H); 13C NMR (125 MHz, DMSO-d6): δ 172.7, 160.6, 142.7, 136.7, 130.9, 129.4, 129.1, 128.9, 127.8, 127.8, 127.6, 125.9, 124.0, 122.9, 121.0, 110.2, 109.6, 90.8, 66.1, 60.8, 56.9, 55.8, 43.3, 24.4; HRMS (ESI): m/z calc. for C28H26Br2N2O4S 645.0058, found 645.0051 [M + H]+.
In silico DFT calculations
All structures corresponding to the reactants, probable transition states and products were sketched using a 2D sketcher and prepared by Ligprep. Geometry optimization and single point energy calculation were performed, with DFT methods at the B3LYP level using the 6-311** basis set in Jaguar, Schrödinger. The optimized 3D pose of all the structures was imaged using Schrödinger.
Conflicts of interest
There are no conflicts to declare.
Acknowledgements
Dr N. Shankaraiah and S. B. gratefully acknowledges to SERB, DST, Govt. of India for research grant (YSS-2015-001709) and provided fellowship. Authors are thankful to Dr Balasubramanyam Sridhar, for X-ray crystallography studies. NIPER Research Communication No. NIPER-H/2020/M002.
Notes and references
- For Selected reviews, see
(a) G. M. Cragg, D. J. Newman and K. M. Snader, J. Nat. Prod., 1997, 60, 52 CrossRef CAS PubMed;
(b) G. M. Rishton, Am. J. Cardiol., 2008, 101, 43 CrossRef PubMed;
(c) A. L. Harvey, Drug Discov. Today, 2008, 13, 894 CrossRef CAS PubMed.
-
(a) R. Heesun, S. Jeongseob and M. K. Haye, J. Org. Chem., 2018, 83, 14102 CrossRef PubMed;
(b) C. Gang, Y. Jing, G. Suo, H. Hongping, L. Shunlin, D. Yingtong, C. Ying, L. Yang and H. Xiaojiang, Mol. Divers., 2012, 16, 151 CrossRef PubMed.
-
(a) J. J. Badillo, A. Silva-García, B. H. Shupe, J. C. Fettinger and A. K. Franz, Tetrahedron Lett., 2011, 52, 5550 CrossRef CAS PubMed;
(b) V. A. Nancy, I. Alejandro, R. Angel, E. C. Luis, M. V. B. Unnamatla and G. Rocío, New J. Chem., 2018, 42, 1600 RSC.
-
(a) S. Ponnusamy, V. Baby and M. Suchithra, Org. Lett., 2007, 9, 4095 CrossRef PubMed;
(b) S. Ponnusamy, V. Baby, S. Kodirajan and M. Suchitra, Tetrahedron Lett., 2008, 49, 2611 CrossRef;
(c) L. Ye, S. Yong-Xing and D. Da-Ming, Adv. Synth. Catal., 2018, 361, 1064 Search PubMed.
-
(a) T. Min-Chao, C. Xuan, L. Jun, H. Rong, T. Haiyan and W. Chun-Jiang, Angew. Chem., Int. Ed., 2014, 5, 4680 Search PubMed;
(b) R. Elisabetta, A. Giorgio, D. Monica, N. Marco, P. Andrea and P. Valentina, Org. Biomol. Chem., 2016, 14, 6095 RSC;
(c) H. Wang and S. E. Reisman, Angew. Chem., Int. Ed., 2014, 53, 6206 CrossRef CAS PubMed.
-
(a) Y. Dongxu, W. Linqing, H. Fengxia, L. Dan, Z. Depeng, C. Yiming, M. Yunxia, K. Weidong, S. Quantao and W. Rui, Chem.–Eur. J., 2014, 20, 1 CrossRef;
(b) M. R. Settu, S. Ramakrishnan and K. M. Arasambattu, Org. Lett., 2014, 16, 2720 CrossRef PubMed.
- For Selected reviews, see
(a) T. P. Singh and O. M. Singh, Mini Rev. Med. Chem., 2018, 18, 9 CrossRef CAS PubMed;
(b) C. Navriti and S. Om, Eur. J. Med. Chem., 2017, 134, 159 CrossRef PubMed;
(c) M. D. Kamal, Expert Opin. Ther. Pat., 2013, 23, 1133 CrossRef PubMed;
(d) C. Karam, A. H. Rajeshwaria, S. Mahak, M. S. Amelia and S. K. Rangappa, Pharmacol. Rep., 2017, 69, 281 CrossRef PubMed.
-
(a) A. A. Andrey, V. V. Elena, S. V. Nataliya, G. M. Alexander, M. B. Ekaterina and Y. M. Mikhail, J. Org. Chem., 2017, 82, 5689 CrossRef PubMed;
(b) C. Gang, Y. Jing, G. Suo, H. Hongping, L. Shunlin, D. Yingtong, C. Ying, L. Yang and H. Xiaojiang, Mol. Divers., 2012, 16, 151 CrossRef PubMed.
-
(a) P. R. Sebahar and R. M. Williams, J. Am. Chem. Soc., 2000, 122, 5666 CrossRef CAS;
(b) B. A. Kumar, G. Gupta, S. Srivastava, A. K. Bishnoi, R. Saxena, R. Kant, R. S. Khanna, P. R. Maulik and A. Dwivedi, RSC Adv., 2013, 3, 4731 RSC.
-
(a) U. Loana, K. Philippe and M. Andre, Angew. Chem., Int. Ed., 2000, 39, 4615 CrossRef;
(b) S. Yoshiaki, I. Shinya and N. Juzo, Chem. Commun., 2002, 2, 134 Search PubMed;
(c) S. Masthanvali, M. Abhijit, A. W. Imtiyaz and K. G. Manas, J. Org. Chem., 2016, 81, 6424 CrossRef PubMed.
- W. Linqing, Y. Dongxu, H. Fengxia, L. Dan, Z. Depeng and W. Rui, Org. Lett., 2015, 17, 176 CrossRef PubMed.
- C. Zhuo, Z. You-Min, Y. Pei-Jun, W. Shaoyin, W. Shaowu, L. Zhen and Y. Gaosheng, J. Am. Chem. Soc., 2015, 137, 10088 CrossRef PubMed.
- H. Saumen, S. R. Somnath, S. A. Mohammad and D. Dhiraj, Org. Lett., 2017, 19, 4082 CrossRef PubMed.
-
(a) B. Sonal, S. Sravani, S. Balasubramanyam and N. Shankaraiah, ChemistrySelect, 2019, 4, 1727 CrossRef;
(b) B. Sonal, R. K. Amol, M. B. Deepti, S. Pankaj, T. Venu and N. Shankaraiah, ChemistrySelect, 2019, 3, 6766 Search PubMed;
(c) P. Sharma, N. P. Kumar, N. H. Krishna, D. Prasanna and N. Shankaraiah, Org. Chem. Front., 2016, 3, 1503 RSC;
(d) N. H. Krishna, A. P. Saraswati, M. Sathish, N. Shankaraiah and A. Kamal, Chem. Commun., 2016, 52, 4581 RSC;
(e) K. R. Senwar, P. Sharma, S. Nekkanti, M. Sathish, A. Kamal, B. Sridhar and N. Shankaraiah, New J. Chem., 2015, 39, 3973 RSC.
-
(a) J. Li, T. Du, G. Zhang and Y. Peng, Chem. Commun., 2013, 49, 1330 RSC;
(b) M. A. Marsini, J. T. Reeves, J. N. Desrosiers, M. A. Herbage, J. Savoie, Z. Li, K. R. Fandrick, C. A. Sader, B. McKibben and D. A. Gao, Org. Lett., 2015, 17, 5614 CrossRef CAS PubMed;
(c) S. Hajra, S. M. Aziz, B. Jana, P. Mahish and D. Das, Org. Lett., 2016, 18, 532 CrossRef CAS PubMed.
- Schrödinger Release 2019-1, Jaguar (2019), Schrödinger, LLC, New York, NY, 2019 Search PubMed.
Footnote |
† Electronic supplementary information (ESI) available. CCDC 1970416. For ESI and crystallographic data in CIF or other electronic format see DOI: 10.1039/d0ra00684j |
|
This journal is © The Royal Society of Chemistry 2020 |