DOI:
10.1039/D0RA00127A
(Paper)
RSC Adv., 2020,
10, 12485-12491
Extracellular histones play a pathogenic role in primary graft dysfunction after human lung transplantation
Received
6th January 2020
, Accepted 22nd March 2020
First published on 27th March 2020
Abstract
Primary graft dysfunction (PGD) causes early mortality and late graft failure after lung transplantation. The mechanisms of PGD are not fully understood but ischemia/reperfusion (I/R) injury may be involved. Extracellular histones have recently been identified as major contributors to I/R injury. Hence, we investigated whether extracellular histones are associated with PGD after lung transplantation. In total, 65 lung transplant patients were enrolled into this study. Blood samples were collected from patients before and serially after transplantation (24 h, 48 h, and 72 h) and measured for extracellular histones, myeloperoxidase (MPO), lactate dehydrogenase (LDH), and multiple cytokines. Besides, the patients' sera were cultured with human pulmonary artery endothelial cells (HPAEC) and human monocyte cell line (THP1) cells, respectively, and cellular viability and cytokine production were determined. Heparin or anti-histone antibody were used to study the effects of histone-neutralized interventions. The results showed that extracellular histones increased markedly after lung transplantation, peaked by 24 h and tended to decrease thereafter, but still retained high levels up to 72 h. Extracellular histones were more abundant in patients with PGD (n = 8) than patients without PGD (n = 57) and linearly correlated with MPO, LDH, and most detected cytokines. Ex vivo studies showed that the patients' sera collected within 24 h after transplantation were very damaging to HPAEC cells and promoted cytokine production in cultured THP1 cells, which could be largely prevented by heparin or anti-histone antibodies. These data suggested a pathogenic role for extracellular histones in PGD after lung transplantation. Targeting extracellular histones may serve as a preventive and therapeutic strategy for PGD following lung transplantation.
1. Introduction
Lung transplantation is now considered the most effective treatment for end-stage lung diseases.1 With the improvement of preservation methods and surgical techniques, as well as the use of immunosuppressants, lung transplantation outcomes have significantly improved in the past decades.2 However, because of the special anatomical structure and physiological functions of the lung, several complications such as primary graft dysfunction (PGD) are commonly seen after lung transplantation, which may be the reason that survival after lung transplantation is relatively low compared with other organ transplants.3,4 PGD is a form of acute lung injury (ALI) that develops within the first 72 hours after lung transplantation.5 It is defined by the presence of hypoxemia and radiographic infiltrates, and is a major cause of early mortality and morbidity after transplant.6 The incidence of PGD after lung transplantation is estimated to be 10 to 30%.3 PGD is also associated with the development of bronchiolitis obliterans syndrome (BOS), a form of chronic lung allograft dysfunction.7 Patients with PGD usually had prolonged hospitalization, and increased short- and long-term mortality when compared to non-PGD patients.8 So far, no specific therapies are available for PGD.
The pathogenesis of PGD is multifactorial but ischemia-reperfusion (I/R) injury is commonly implicated, which may play a central role resulting in transplant lung dysfunction.9 Lung I/R injury is a complex pathophysiologic process involving many types of cells and mediators. Of these, extracellular histones have been considered as key mediators implicated in I/R injuries of many organs including the lung, liver, kidney, heart, and brain.10–13 Extracellular histones increase abundantly after I/R injury, which are not only correlated with disease severity and poor outcomes, but also may act as a potential therapeutic target.14–16 It has been confirmed that targeting extracellular histones ameliorates the I/R injuries of many organs.17 In view of the critical role of extracellular histones in mediating I/R injury, we aimed to investigate whether extracellular histones may play a pathological and targetable role in PGD after human lung transplantation.
2. Patients and methods
2.1. Patients
Totally 65 patients undergoing lung transplantation between August 2015 and October 2019 were included in this study. The study was approved by the Institutional Review Board of Shanghai Pulmonary Hospital, Shanghai, China and performed with the Declaration of Helsinki. All patients or their nominated next of kin provided written informed consent. Baseline characteristics of these patients were recorded. PGD is defined by radiographic presence of diffuse pulmonary infiltrates within 72 hours after lung transplantation.5 Clinical manifestations of PGD are similar to non-cardiogenic pulmonary edema, featured as hypoxemia, hypotension and low cardiac output caused by decreased lung compliance and increased pulmonary capillary resistance.18
2.2. Blood sample collection
The peripheral blood samples from patients were collected before and serially after lung transplantation. A total of 4 time points was defined: before graft, within 24 hours, 48 hours, and 72 hours after graft. Blood samples were collected into citrated vacutainers and centrifuged at 2000 × g for 20 min and the generated plasma was separated, aliquoted and stored at −80 °C. The matched plasma was also produced for another set of ex vivo experiments.
2.3. Measurement of extracellular histones and multiple cytokines
The levels of extracellular histones (nucleosomes) in the plasma samples were determined using a Cell Death Detection ELISA kit (Roche, Germany).19 The purified calf thymus histones were used to generate standard curves. For detection of various cytokines (IL-1β, IL-2, IL-4, IL-5, IL-6, IL-8, IL-10, IL-12p70, IL-17A, IL-18, IL-22, IL-23, IL-27, GM-CSF, TNF-α), a panel of multiplex immunoassay from Affymetrix eBioscience (USA) was used on a Luminex/Bioplex-200 system.
2.4. Measurement of MPO and LDH
Plasma MPO, a classical marker for neutrophil and monocyte/macrophage activation, and LDH, a cytoplasmic enzyme indicating cellular damage, were measured using commercial kits (Roche, Germany), according to the manufacturer's protocols.20
2.5. Ex vivo experiments
Human pulmonary artery endothelial cells (HPAEC) and human monocyte cell line (THP1) were obtained from American Type Culture Collection (ATCC). 2 × 105 cells were seeded in 24-well plates and cultured in RPMI-1640 (10% fetal bovine serum, 100 U ml−1 penicillin/streptomycin, 2 mM glutamine) in a 5% CO2 humidified atmosphere at 37 °C. After the cells reached 80–90% confluence, they were incubated with 50% of the sera samples (approximately 400 μl) for 24 h, which were pooled from the patients before or within 24 h after transplantation, respectively. To verify the impact of extracellular histones in the sera, 20 μg ml−1 of anti-histone H4 antibody or 200 U ml−1 of heparin (Sigma-Aldrich, USA) was added to the cultured cells, respectively.
For the cytotoxicity assay, the cultured HPAEC cells were detached and washed with PBS and incubated with PI dye solution (10 μg ml−1, Sigma-Aldrich, USA) in the dark for 10 min at room temperature. The cells were then subjected to flow cytometry analysis. In addition, the supernatants were collected and analyzed for LDH levels. To test the influence of extracellular histones in sera samples on THP1 cells, the supernatants (approximately 100 μl) were collected and analyzed for the production of multiple cytokines (IL-1β, IL-6, IL-10, IL-17A, IL-18, TNF-α) using a Multiplex Immunoassay kit from Affymetrix eBioscience.
2.6. Statistical analysis
For human data, values were presented as medians and interquartile ranges. For ex vivo data, values were presented as mean ± standard deviation (SD). The results were analyzed using unpaired Student's t test or Mann–Whitney test (for two groups), one-way ANOVA with Turkey post-tests (for more than two groups). The correlations were analyzed using Spearman's correlation or Pearson correlation analysis. Differences were considered statistically significant when p < 0.05. All data were analyzed using GraphPad Prism (GraphPad Software, Inc., San Diego, CA, USA).
3. Results
3.1. Patient characteristics
A total of 65 patients received lung transplantation. The baseline characteristics of the patients were indicated in Table 1. Main reasons for transplantation were COPD (n = 23, 35.38%), interstitial lung disease (n = 20, 30.67%), idiopathic pulmonary fibrosis (n = 12, 18.46%), pulmonary hypertension (n = 2, 3.07%) and others (n = 8, 12.3%). Donor lungs were obtained from deceased cardiac failure patients.
Table 1 Baseline characteristics in patients undergoing lung transplantationa
Characteristics |
Values |
Data were expressed as mean (SD) or number of patients (%). |
Age (years) |
60.60 ± 11.17 |
Gender (male/female) |
58/7 |
BMI |
21.37 ± 3.76 |
Operation time (min) |
239.1 ± 71.79 |
![[thin space (1/6-em)]](https://www.rsc.org/images/entities/char_2009.gif) |
Etiology of lung diseases |
Chronic obstructive pulmonary disease |
23 (35.38%) |
Idiopathic pulmonary fibrosis |
20 (30. 67%) |
Interstitial lung disease |
12 (18.46%) |
Pulmonary hypertension |
2 (3.07%) |
Others |
8 (12.3%) |
Hospital stay (day) |
36 (28–60) |
![[thin space (1/6-em)]](https://www.rsc.org/images/entities/char_2009.gif) |
Type of transplant |
Double lung transplant |
5 (7.69%) |
Single lung transplant |
60 (92.31%) |
3.2. Extracellular histones increased significantly after lung transplantation
Extracellular histone levels in the patients following lung transplantation were 4–6 times higher than the levels measured before transplantation. Extracellular histones reached a peak by 24 h after graft [5.368 (3.686, 7.103) μg ml−1] and then gradually decreased within 72 h [2.756 (1.961, 4.713) μg ml−1] but were still higher than the levels before transplantation [1.251 (0.896, 1.398) μg ml−1] (Fig. 1A). Based on an early evaluation (within 72 h after graft), the patients were divided into the groups of with PGD (n = 8) and without PGD (n = 57). Extracellular histones in patients with PGD were significantly higher than that in the patients without PGD [5.882 (4.664, 7.755) vs. 2.478 (1.789, 4.073) μg ml−1] (Fig. 1B).
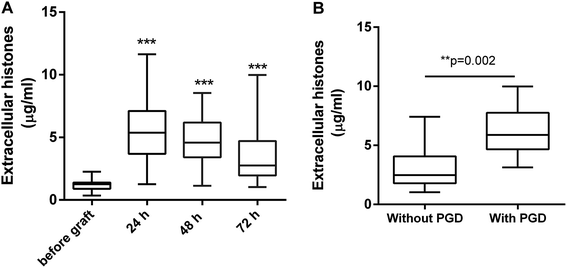 |
| Fig. 1 The levels of extracellular histones in the blood of patients undergoing lung transplantation. (A) Extracellular histone levels were increased significantly in patients undergoing lung transplantation at different time points. (B) Median extracellular histones at 72 h after graft were significantly higher in patients with PGD than those in patient without PGD. Values were presented as median (interquartile range). | |
3.3. Extracellular histones correlated with increased MPO, LDH, and systemic inflammation
When compared with the levels measured before graft, both MPO and LDH were significantly elevated by 24 h after graft. They showed a decreasing trend in the following time points (48 h, 72 h) but were still higher than the levels before graft (Fig. 2). The changes in extracellular histones correlated with the changes in MPO and LDH (Table 2).
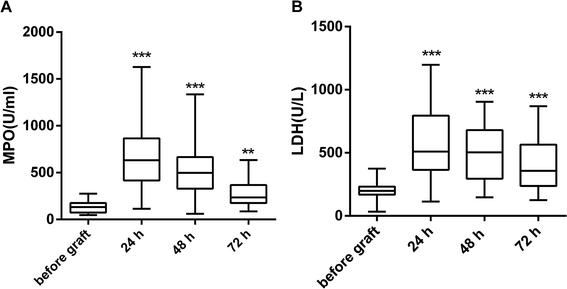 |
| Fig. 2 The levels of MPO and LDH in the blood of patients undergoing lung transplantation. (A) MPO (B) LDH levels were increased markedly at 24 hours after graft and showed a decreasing trend at 48 h and 72 h after graft but were still significantly higher as compared with the levels before graft. Values were presented as median (interquartile range). | |
Table 2 Correlation of extracellular histones with various variables in patients undergoing lung transplantationa
|
r |
p |
*p < 0.05 was considered to be statistically significant. |
MPO |
0.4369 |
0.011* |
LDH |
0.5292 |
0.007* |
IL-1β |
0.3023 |
0.064 |
IL-6 |
0.5047 |
0.007* |
IL-10 |
0.6915 |
0.001* |
IL-17A |
0.3899 |
0.024* |
IL-18 |
0.5176 |
0.008* |
TNF-α |
0.3792 |
0.012* |
A panel of multiple cytokines was measured as well to assess the degree of systemic inflammation. Of these, a total of 6 cytokines (IL-1β, IL-6, IL-10, IL-17A, IL-18, TNF-α) were significantly increased in the patients by 24 h after lung transplantation in contrast to their levels measured before graft. Subsequently, most cytokines exhibited a decreasing trend but remained high levels up to 72 h. Extracellular histone levels were positively correlated with most detected cytokines (Table 2). Taken together, these data suggested that the increased extracellular histones might play a pivotal role in promoting innate immune cell activation, cellular damage, and the enhanced systemic inflammation after lung transplantation (Fig. 3).
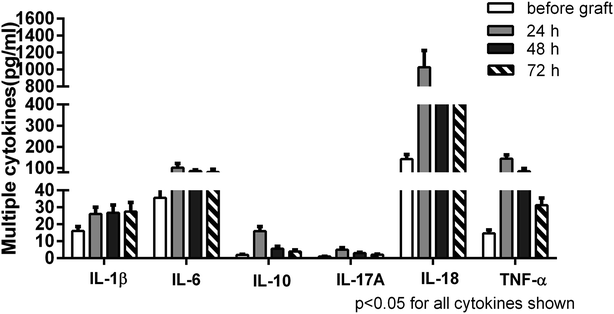 |
| Fig. 3 Detection of systemic inflammation in lung transplantation patients. Multiplex immunoassay for a panel of various cytokines (IL-1β, IL-2, IL-4, IL-5, IL-6, IL-8, IL-10, IL-12p70, IL-17A, IL-18, IL-22, IL-23, IL-27, GM-CSF, TNF-α) was performed. Only 6 cytokines with significant differences (*p < 0.05 in contrast to before graft) were shown. Values were presented as median (interquartile range). | |
3.4. Extracellular histones provoked cytotoxicity and cytokine production in vitro
We next asked whether the increased extracellular histones in patients' blood may have adverse effects contributing to lung injury in PGD or other complications. The patients' sera collected at 24 h after graft were found to contain the highest levels of extracellular histones (median value, 5.368 μg ml−1) and thereby pooled and incubated with HPAEC and THP1 cells, respectively. The sera before graft (with histone median value of 1.251 μg ml−1) were also included with the cells as the controls. As shown in Fig. 4, the patients' sera at 24 h after graft significantly reduced HPAEC cell viability and promoted production of various inflammatory cytokines in the supernatant of cultured THP1 cells.
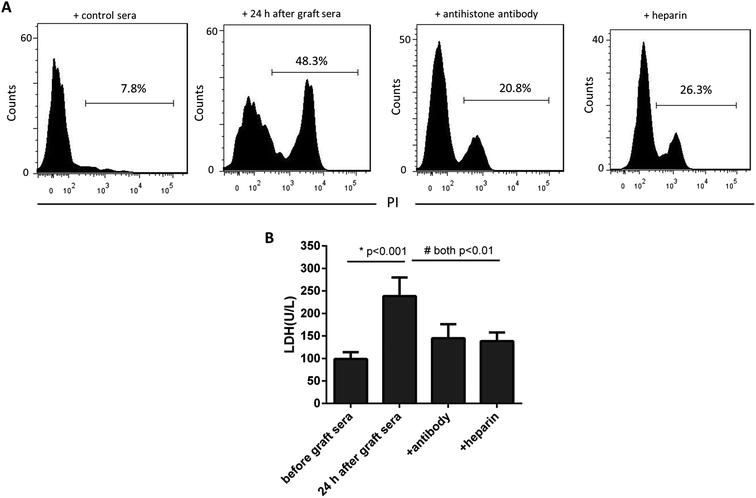 |
| Fig. 4 Lung transplantation patients' sera induced direct cytotoxicity towards HPAEC cells. (A) Flow cytometric analysis of PI staining showed that the patients' sera collected at 24 h after graft that contained high levels of extracellular histones were injurious to cultured HPAEC cells. (B) The LDH levels in the supernatants of cultured HPAEC cells treated with the sera pooled at 24 h after graft were significantly increased. Administration of anti-histone H4 antibody or heparin equally improved cell damage. Variables were expressed as mean ± standard deviation (SD). | |
Neutralization of extracellular histones has been reported to be protective in various inflammatory conditions.21,22 Here we investigated whether heparin, which is able to bind histones,23 would diminish the observed effects of histones on the cells. Anti-histone H4 antibody was applied as the control. The results showed that administration of heparin or anti-histone antibody equally inhibited the patients' sera-induced HPAEC damage and cytokine production from THP1 cells, as evidenced by improved cell viability and decreased inflammatory cytokine levels (Fig. 4 and 5). Collectively these data suggest a direct relationship between extracellular histones and cellular damage and systemic inflammation, and provide a possible therapeutic target in clinical management of PGD after lung transplantation.
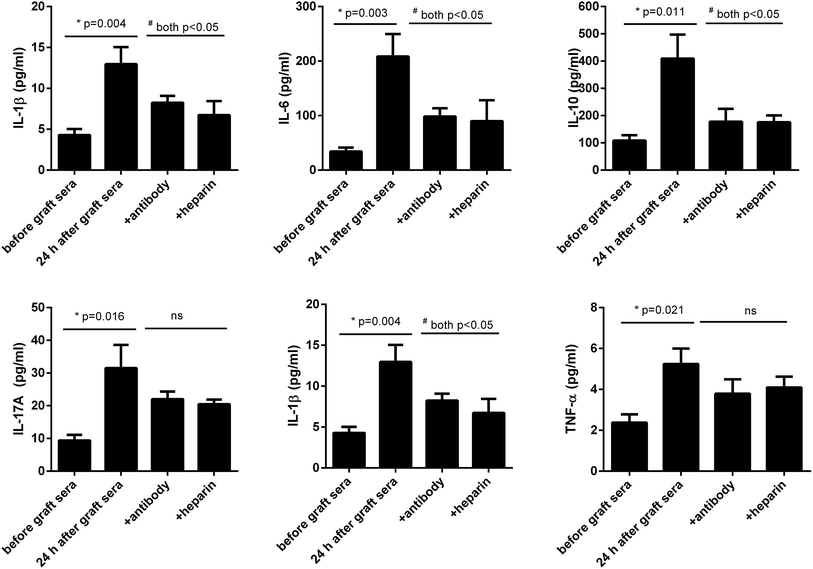 |
| Fig. 5 Lung transplantation patients' sera provoked human monocytic cells. It showed that 6 histone-related cytokines were all increased in the supernatants of human monocytic THP1 cells treated by lung transplantation patients' sera collected at 24 h after graft, whereas addition of anti-histone H4 antibody or heparin largely decreased these cytokine levels. Variables were expressed as mean ± standard deviation (SD). | |
4. Discussion
Lung transplantation has been proved to be the most effective life-saving therapy for the end-stage lung disease patients such as chronic obstructive pulmonary disease (COPD), interstitial lung disease, idiopathic pulmonary fibrosis, and pulmonary hypertension.1 Currently, the prognosis of patients undergoing lung transplantation has significantly improved because of the development of surgical techniques and wide administration of immunosuppressants.2 However, PGD is still one of the most common and life-threatening complications affecting lung transplantation outcome. PGD occurs in up to 30% of lung transplant patients within the first 72 hours after graft, characterized by the development of bilateral pulmonary infiltrates and noncardiogenic pulmonary edema.24 Discouragingly, there are no certified effective methods for the prevention and treatment of PGD. There were many risk factors for PGD including surgical risk factors, donor-related factors and recipient-related factors.2 Among these factors, I/R injury is considered to be the most common and critical.25 In the process of I/R injury, various inflammatory mediators and cytokines released abundantly, which further trigger inflammatory response cascade and lead to the damage of vascular endothelium and alveolar epithelial cells.25–27 Notably, extracellular histones are discovered to be key mediators of I/R injury and targeting extracellular histones may attenuate I/R-related cell and tissue damage.28 Hence, we intended to investigate whether extracellular histones may contribute to the occurrence of PGD in human lung transplantation.
Histones, including H1, H2A, H2B, H3, and H4, have been a traditional research focus in the context of regulating nuclear architecture.29 Under conditions of cellular stress or injury such as trauma, ischemic or chemical injury, or infection, histones can be released from both dying cells (e.g., apoptotic or necrotic cells) and activated immune cells (e.g., neutrophils) into the extracellular space.11 It has revealed that extracellular histones have the ability to cause endothelial cytotoxicity, platelet activation and aggregation, and most importantly act as danger/damage-associated molecular patterns (DAMPs) to initiate and promote systemic inflammation.11,30 The link between extracellular histones and I/R injury has been recognized;14 however, it is uncertain whether extracellular histones are associated with PGD in human lung transplantation. Our hypothesis was that extracellular histones are a danger signal contributing to PGD pathogenesis.
In this study, we determined extracellular histone levels in patients undergoing lung transplantation and found that extracellular histones were elevated abundantly after transplantation and peaked by 24 h with high levels still measurable through 72 h. Previous studies showed that I/R injury of various organs consistently caused an elevation of extracellular histones.31 Thus, the observed increase in the extracellular histones in this study is likely derived from I/R injury during the process of transplantation. It is noticeable that, compared with patients without PGD, patients with PGD had significantly higher levels of extracellular histones after graft, thereby suggesting that extracellular histones may act as an important cause for PGD. In addition, we detected an evident cellular damage and pronounced immune cell activation, along with a significant inflammatory response after lung transplantation. Extracellular histones correlated with cellular damage, immune cell activation, and systemic inflammation, all of these are in line with previous studies that extracellular histones may induce cytotoxicity and act as a DAMP molecule to enhance systemic inflammation.32,33
To further confirm these findings, we incubated the patients' sera collected after graft and contained higher levels of extracellular histones with HPAEC and THP1 cells and observed that the sera induced massive HPAEC damage as well as caused significant production of inflammatory cytokines from cultured THP1 cells. We further confirmed that those effects on cell death and cytokine production were caused from histones, since addition of specific anti-histone H4 antibody and heparin into the cells significantly prevented HPAEC death and production of cytokines from THP1. These data suggest that the elevated extracellular histones is an important determinant for the development of PGD after transplantation and blockade of extracellular histones may serve as a preventive and therapeutic strategy for PGD following lung transplantation.
In summary, our data provide a plausible explanation for the mechanisms related to PGD after lung transplantation. Lung transplantation-related I/R injury causes damage of cells and tissues, and leads to the release of histones from these dying cells into extracellular space such as the circulation. High concentrations of extracellular histones induce direct cytotoxicity, as well as act as DAMP molecules to aggravate systemic inflammation by stimulating immune cells to produce multiple cytokines, which eventually contribute to PGD or other complications. Therefore, determining extracellular histone levels may predict the occurrence of early graft dysfunction in human lung transplantation. More importantly, extracellular histone-targeted therapy appears to be potentially effective for clinical management of PGD, which requires more investigations in the future.
Conflicts of interest
The authors declare that they have no conflict of interests.
Acknowledgements
This study was supported by the Science and Technology Commission of Shanghai Municipality (19ZR1443000) and the Innovation Team of Shanghai Pulmonary Hospital, Tongji University of Medicine (fkcx1906). This study was conducted by Shanghai Pulmonary Hospital, Tongji University School of Medicine, Shanghai 200433, P. R. China. We thank all the patients for their participation.
References
- M. Hartert, O. Senbaklavacin, B. Gohrbandt, B. M. Fischer, R. Buhl and C. F. Vahld, Lung transplantation: a treatment option in end-stage lung disease, Dtsch. Arztebl. Int., 2014, 111(7), 107–116 Search PubMed.
- C. M. Shaver and L. B. Ware, Primary graft dysfunction: pathophysiology to guide new preventive therapies, Expet Rev. Respir. Med., 2017, 11(2), 119–128 CrossRef CAS PubMed.
- R. J. Shah and J. M. Diamond, Primary Graft Dysfunction (PGD) Following Lung Transplantation, Semin. Respir. Crit. Care Med., 2018, 39(2), 148–154 CrossRef PubMed.
- V. E. Laubach and A. K. Sharma, Mechanisms of lung ischemia-reperfusion injury, Curr. Opin. Organ Transplant., 2016, 21(3), 246–252 CrossRef CAS PubMed.
- M. I. Morrison, T. L. Pither and A. J. Fisher, Pathophysiology and classification of primary graft dysfunction after lung transplantation, J. Thorac. Dis., 2017, 9(10), 4084–4097 CrossRef PubMed.
- R. J. Shah, J. M. Diamond and E. Cantu, et al., Objective Estimates Improve Risk Stratification for Primary Graft Dysfunction after Lung Transplantation, Am. J. Transplant., 2015, 15(8), 2188–2196 CrossRef CAS PubMed.
- A. DerHovanessian, S. S. Weigt and V. Palchevskiy, et al., The Role of TGF-beta in the Association Between Primary Graft Dysfunction and Bronchiolitis Obliterans Syndrome, Am. J. Transplant., 2016, 16(2), 640–649 CrossRef CAS PubMed.
- M. K. Porteous and J. C. Lee, Primary Graft Dysfunction After Lung Transplantation, Clin. Chest Med., 2017, 38(4), 641–654 CrossRef PubMed.
- H. Kim, R. Zamel and X. H. Bai, et al., Ischemia-reperfusion induces death receptor-independent necroptosis via calpain-STAT3 activation in a lung transplant setting, Am. J. Physiol. Lung Cell Mol. Physiol., 2018, 315(4), L595–l608 CrossRef CAS PubMed.
- D. Nakazawa, S. V. Kumar and J. Marschner, et al., Histones and Neutrophil Extracellular Traps Enhance Tubular Necrosis and Remote Organ Injury in Ischemic AKI, J. Am. Soc. Nephrol., 2017, 28(6), 1753–1768 CrossRef CAS PubMed.
- E. Silk, H. Zhao, H. Weng and D. Ma, The role of extracellular histone in organ injury, Cell Death Dis., 2017, 8(5), e2812 CrossRef CAS PubMed.
- H. Zhao, H. Huang and A. Alam, et al., VEGF mitigates histone-induced pyroptosis in the remote liver injury associated with renal allograft ischemia-reperfusion injury in rats, Am. J. Transplant., 2018, 18(8), 1890–1903 CrossRef CAS PubMed.
- A. S. Savchenko, J. I. Borissoff and K. Martinod, et al., VWF-mediated leukocyte recruitment with chromatin decondensation by PAD4 increases myocardial ischemia/reperfusion injury in mice, Blood, 2014, 123(1), 141–148 CrossRef CAS PubMed.
- H. Huang, J. Evankovich and W. Yan, et al., Endogenous histones function as alarmins in sterile inflammatory liver injury through toll-like receptor 9 in mice, Hepatology, 2011, 54(3), 999–1008 CrossRef CAS PubMed.
- T. C. van Smaalen, D. M. Beurskens and E. R. Hoogland, et al., Presence of Cytotoxic Extracellular Histones in Machine Perfusate of Donation After Circulatory Death Kidneys, Transplantation, 2017, 101(4), e93–e101 CrossRef CAS PubMed.
- S. Arumugam, K. Girish Subbiah, K. Kemparaju and C. Thirunavukkarasu, Neutrophil extracellular traps in acrolein promoted hepatic ischemia reperfusion injury: therapeutic potential of NOX2 and p38MAPK inhibitors, J. Cell. Physiol., 2018, 233(4), 3244–3261 CrossRef CAS PubMed.
- N. Hayase, K. Doi and T. Hiruma, et al., Recombinant Thrombomodulin on Neutrophil Extracellular Traps in Murine Intestinal Ischemia-Reperfusion, Anesthesiology, 2019, 131(4), 866–882 CrossRef CAS PubMed.
- Y. Suzuki, E. Cantu and J. D. Christie, Primary graft dysfunction, Semin. Respir. Crit. Care Med., 2013, 34(3), 305–319 CrossRef PubMed.
- X. Lv, T. Wen and J. Song, et al., Extracellular histones are clinically relevant mediators in the pathogenesis of acute respiratory distress syndrome, Respir. Res., 2017, 18(1), 165 CrossRef PubMed.
- Y. Zhang, Z. Wen and L. Guan, et al., Extracellular histones play an inflammatory role in acid aspiration-induced acute respiratory distress syndrome, Anesthesiology, 2015, 122(1), 127–139 CrossRef CAS PubMed.
- K. Daigo, Y. Takamatsu and T. Hamakubo, The Protective Effect against Extracellular Histones Afforded by Long-Pentraxin PTX3 as a Regulator of NETs, Front. Immunol., 2016, 7, 344 Search PubMed.
- R. Yang, X. Zou, J. Tenhunen and T. I. Tonnessen, HMGB1 and Extracellular Histones Significantly Contribute to Systemic Inflammation and Multiple Organ Failure in Acute Liver Failure, Mediat. Inflamm., 2017, 2017, 5928078 Search PubMed.
- K. C. Wildhagen, P. Garcia de Frutos and C. P. Reutelingsperger, et al., Nonanticoagulant heparin prevents histone-mediated cytotoxicity in vitro and improves survival in sepsis, Blood, 2014, 123(7), 1098–1101 CrossRef CAS PubMed.
- M. K. Porteous, J. M. Diamond and J. D. Christie, Primary graft dysfunction: lessons learned about the first 72 h after lung transplantation, Curr. Opin. Organ Transplant., 2015, 20(5), 506–514 CrossRef CAS PubMed.
- W. A. den Hengst, J. F. Gielis, J. Y. Lin, P. E. Van Schil, L. J. De Windt and A. L. Moens, Lung ischemia-reperfusion injury: a molecular and clinical view on a complex pathophysiological process, Am. J. Physiol. Heart Circ. Physiol., 2010, 299(5), H1283–H1299 CrossRef CAS PubMed.
- G. Y. Chen and G. Nunez, Sterile inflammation: sensing and reacting to damage, Nat. Rev. Immunol., 2010, 10(12), 826–837 CrossRef CAS PubMed.
- Z. G. Li, M. J. Scott and T. Brzoska, et al., Lung epithelial cell-derived IL-25 negatively regulates LPS-induced exosome release from macrophages, Military Medical Research, 2018, 5(1), 24 CrossRef PubMed.
- H. Huang, S. Tohme and A. B. Al-Khafaji, et al., Damage-associated molecular pattern-activated neutrophil extracellular trap exacerbates sterile inflammatory liver injury, Hepatology, 2015, 62(2), 600–614 CrossRef CAS PubMed.
- M. Hoeksema, M. van Eijk, H. P. Haagsman and K. L. Hartshorn, Histones as mediators of host defense, inflammation and thrombosis, Future Microbiol., 2016, 11(3), 441–453 CrossRef CAS PubMed.
- J. Xu, X. Zhang and R. Pelayo, et al., Extracellular histones are major mediators of death in sepsis, Nat. Med., 2009, 15(11), 1318–1321 CrossRef CAS PubMed.
- Z. Cheng, S. T. Abrams and Y. Alhamdi, et al., Circulating Histones Are Major Mediators of Multiple Organ Dysfunction Syndrome in Acute Critical Illnesses, Crit. Care Med., 2019, 47(8), e677–e684 CrossRef PubMed.
- C. Zhu, Y. Liang, X. Li, N. Chen and X. Ma, Unfractionated heparin attenuates histone-mediated cytotoxicity in vitro and prevents intestinal microcirculatory dysfunction in histone-infused rats, J. Trauma Acute Care Surg., 2019, 87(3), 614–622 CrossRef CAS PubMed.
- Y. Zhang, X. Li and J. J. Grailer, et al., Melatonin alleviates acute lung injury through inhibiting the NLRP3 inflammasome, J. Pineal Res., 2016, 60(4), 405–414 CrossRef CAS PubMed.
Footnote |
† Yang Jin and Meng Sun contributed equally to this work. |
|
This journal is © The Royal Society of Chemistry 2020 |
Click here to see how this site uses Cookies. View our privacy policy here.