DOI:
10.1039/C9RA10020B
(Paper)
RSC Adv., 2020,
10, 10254-10262
Nobiletin, a citrus polymethoxyflavone, enhances the effects of bicalutamide on prostate cancer cells via down regulation of NF-κB, STAT3, and ERK activation
Received
29th November 2019
, Accepted 26th February 2020
First published on 10th March 2020
Abstract
Natural products have shown potential to be combined with current cancer therapies to improve patient outcomes. Nobiletin (NBT) is a citrus polymethoxyflavone and has been shown to exert an anticancer effect in various cancer cells. We investigated the effects and mechanisms of NBT in combination with bicalutamide (BCT), a commonly used anti-androgen drug in prostate cancer therapy, on prostate cancer cells. Our results demonstrate that the combined treatment with NBT and BCT produces an enhanced inhibitory effect on the growth of prostate cancer cells compared to either compound alone. The synergistic action of NBT and BCT was confirmed using isobologram analysis. Moreover, this study has shown that NBT and BCT synergistically inhibited colony formation and migration as well as induced apoptosis. Mechanistic studies demonstrate that NBT and BCT combination reduced key cellular signaling regulators including: p-Erk/Erk, p-STAT3/STAT3 and NF-κB. Overall, these results suggest that NBT combination with BCT may be an effective treatment for prostate cancer.
Introduction
Prostate cancer is the most common non-skin malignancy in males in developed countries and is the second most frequent cause of male cancer-related death.1 Despite recent diagnostic and therapeutic advances, prostate cancer is quite deadly due to its metastatic propensity. Prostate cancer therapies have shown toxic side effects and eventual resistance.2 Currently, androgen deprivation/castration is the principal option for treating prostate cancer. Bicalutamide (BCT, Fig. 1B) is a non-steroidal competitive androgen receptor inhibitor approved by the FDA for the treatment of stage D2 metastatic prostate carcinoma. Although BCT treatment initially exhibits favorable responses, prostate cancers eventually develop resistance and progress to castration-resistant prostate cancer (CRPC).3 Thus, CRPC presents a major obstacle during the management of advanced prostate cancer. In addition, long-term administration of BCT may lead to a series of side effects including breast pain, hot flushes, gynecomastia, and/or decreased libido and impotence, which decreases the quality of life of patients.4 Therefore, it is urgent to develop a novel and efficacious treatment for prostate cancer. Previous reports have indicated that combining anticancer drugs with natural products may help minimize drug resistance and enhance therapeutic efficacy.5,6
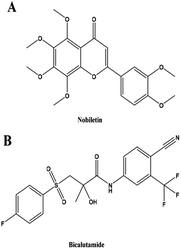 |
| Fig. 1 Chemical structures of NBT and BCT. | |
Polymethoxyflavones (PMFs) are a group of flavonoids whose bioactivity has just been discovered. These flavonoids are mainly derived from the citrus plant of Rutaceae, especially from Citrus sinensis and Citrus reticulata. Nobiletin (NBT, Fig. 1A) is one of the more widely known citrus polymethoxyflavones and has been shown to exert a potential pharmacological effect in various groups of diseases, such as cardiac,7 vascular,8 and neurodegenerative diseases.9 NBT, as a promising natural product, has exhibited anti-proliferative, anti-angiogenesis and apoptotic effects in various cancer vis inhibited NF-κB, STAT3 and ERK.10–13 Many studies have shown that NBT enhances the drug sensitivity of cancer cells to some clinical drugs, such as atorvastatin5 and paclitaxel.14 Moreover, NBT also shown synergistic anti-prostate cancer cells when combined with sorafenib.15 So NBT may be a great adjuvant to use in anti-cancer.
Due to the complexity of cancers, there is an increasing interest in using a combination of anticancer agents at low doses to target multiple pathways rather than using a high dose single agent to target one pathway only. Based on a series of studies looking at the bioactivity of NBT and its application to androgen-dependent and androgen-independent prostate cancer in conjunction with BCT, and previous research on our group,16–18 we hypothesize that together NBT and BCT may synergistically and more efficaciously inhibit prostate cancer cells. Our study is the first to provide evidence that shows NBT's ability to enhances the anti-cancer effects of BCT in prostate cancer cells, especially those which are androgen-independent.
Experimental
Cell culture and reagents
Prostate cancer VCaP and PC-3 cells, and non-tumorigenic epithelial HaCaT cells were obtained from the American Type Culture Collection (ATCC, Rockville, MD, USA). NBT (MB6570-S) was acquired from Dalian Meilun Biotechnology Co, Ltd (Dalian, China), and BCT was purchased from Med Chem Express (China). RPMI-1640 tissue and DMEM culture medium, penicillin–streptomycin, L-glutamine and fetal bovine serum (FBS) were obtained from Gibco (Grand Island, NY). VCaP and PC-3 cells were maintained in a RPMI-1640 culture medium, HaCaT cells were maintained in a DMEM culture medium. Both RPMI-1640 and DMEM culture medium were supplemented with 10% FBS, penicillin (100 units per mL)–streptomycin (100 μg mL−1) and L-glutamine (300 μg mL−1). Cultured cells were grown at 37 °C in a humidified atmosphere of 5% CO2 and were passaged twice a week.
MTT assay
MTT assay is one of the common methods for cell viability assay, which based on the activity of mitochondrial enzyme succinate dehydrogenase.19 In the present assay, PC-3, VCaP and HaCaT cells were seeded on 96-well plates at a density of 4 × 103 per well and incubated at 37 °C for 24 h. The cells were then treated with various concentrations of NBT and/or BCT for 24 h, 48 h and 72 h. After treatment, 100 μL of MTT solution (0.5 mg mL−1 in free-FBS medium) was added to each of the wells of the plate and incubated at 37 °C for 4 h. After careful removal of the medium, 100 μL DMSO was added to each well. The absorbance was recorded on a microplate reader at 490 nm.
Trypan blue exclusion assays
Trypan blue exclusion assay is used to determine the number of viable cells by measuring the cell membrane integrity.20 PC-3 and VCaP cells were seeded at a density of 0.2 × 105 cells per mL in 35 mm dishes and incubated for 24 h. The cells were then treated with NBT and BCT alone or in combination for 72 h. The number of viable cells after each treatment was determined using a hemocytometer under a light microscope (Nikon Optiphot, Tokyo, Japan). Cell viability was determined by the trypan blue exclusion assay, which was done by mixing 80 μL of cell suspension and 20 μL of 0.4% trypan blue solution for 2 min. Blue cells were counted as dead cells and the cells that did not absorb dye were counted as live cells.
Colony formation assay
Cells were seeded at 1 × 103 cells per well in 6-well plates and treated with NBT, BCT or a combination of the two for 24 h and replaced with fresh media. Cells were grown for 5 days. In order to visualize the colonies, cells were fixed in methanol for 15 min and then stained with Giemsa for an additional 10 min.
Assessment of apoptotic cells by morphology
Apoptosis was determined by morphological assessment of cells stained with PI.21 PC-3 and VCaP cells were seeded at a density of 2 × 105 cells per mL and the cells were treated with NBT (30 μM) or BCT (30 μM) alone or in combination for 48 h. Cytospin slides were prepared after each trypan blue exclusion experiment and cells were fixed with acetone/methanol (1
:
1, v/v) for 10 min at room temperature, followed by 10 min with PI staining (1 μg mL−1 in PBS) and analyzed using a fluorescence microscope (Nikon Eclipse TE200). Apoptotic cells were identified by classic morphological features, including nuclear condensation, cell shrinkage, and formation of apoptotic bodies.
Cell migration assays
The cell migration ability was assessed using a scratch wound-healing assay. In brief, cells were seeded at 5 × 105 cells per well in 12-well plates. After 24 h, when the cells were 95–100% confluent, media was removed and the cell surface was scratched using a sterile 200 μL pipette tip. Floating cells were removed by washing twice with PBS. Cultivation was subsequently continued for 24 h in 1% FBS medium. The cells were treated with NBT or BCT alone or in combination.
NF-κB-dependent reporter gene expression assay
According to a previously published protocol, NF-κB transcriptional activity was measured using the NF-κB-luciferase reporter gene expression assay using PC-3/N and VCaP/N cells.21,22 PC-3/N and VCaP/N cells were seeded at a density of 2 × 104 cells per mL in 12-well plates and treated with NBT and/or BCT for 24 h, and then the cells were washed with ice-cold PBS and harvested in 1× reporter lysis buffer (Promega Madison WI, USA). After centrifugation, 10 μL aliquots of the supernatants were mixed with 10 μL luciferase substrate (Promega) and measured for luciferase activity using a Luminometer from Turner Designs Instrument (Sunnyvale, CA, USA).
Western blot analysis
PC-3 cells were seeded at a density of 1 × 106 cells in 100 mm culture dishes and treated with NBT and/or BCT for 24 h. After treatment, the cell lysates were prepared as described earlier. Proteins were subjected to sodium dodecyl sulfate polyacrylamide gel electrophoresis (SDS-PAGE) and transferred to a nitrocellulose membrane. After blocking nonspecific binding sites with blocking buffer, the membrane was incubated overnight at 4 °C with primary antibodies. The β-actin was used as a loading control. Following removal of the primary antibody, the membrane was washed three times with TBS (PBS containing 0.05% tween 20) buffer at room temperature and then incubated with fluorochrome conjugated secondary antibody. The membrane was then washed with TBS three times. The final detection was carried out using Pierce™ ECL Western Blotting Substrate (Thermo, USA).
Statistical analysis
The interaction between BCT and NBT was determined using a combination index (CI), which was calculated according to the median-effect principle, based on a previous report.23 The equation for the isobologram was shown as CI = A1/A2 + B1/B2. A1 and B1 represent the doses of NBT and BCT necessary to produce the same effect in combination, and A2 and B2 represent the individual doses of drug NBT and BCT required to inhibit a given level of cell viability respectively. If CI is <1, then the drugs are considered to act synergistically. If the CI is >1 or =1, then the drugs act in an antagonistic or additive manner, respectively. All the data is represented as mean ± SEM. Experimental data analysis was carried out using a one-way ANOVA. A p-value < 0.05 was considered statistically significant.
Results
Treatment with NBT or BCT reduces the viability of both PC-3 and VCaP cells
The effects of NBT and BCT alone on the growth of human prostate cancer cells were determined using MTT assays. VCaP (androgen-dependent) and PC-3 (androgen-independent) prostate cancer cells were treated with different concentrations of NBT or BCT alone for 24 h, 48 h and 72 h. As shown in Fig. 2, treatment of PC-3 and VCaP cells with BCT or NBT alone resulted in the inhibition of the viability of the cancer cells in a dose-dependent and time-dependent manner. In this result, BCT showed greater inhibitory effect on cell viability in VCaP cells than that in PC-3 cells, while the inhibitory effect of NBT were stronger in PC-3 cells than that in VcaP cells. It was worth noting that BCT, as an androgen receptor, shown inhibiting activity on PC-3 cells. These results agree with previous studies.6,24,25 NBT or BCT at 20–50 μM concentrations had small to moderate effects on prostate cancer cells, which allow us to determine the potential synergistic effect of combinations of the two compounds. Therefore we chose 20–50 μM for combination studies.
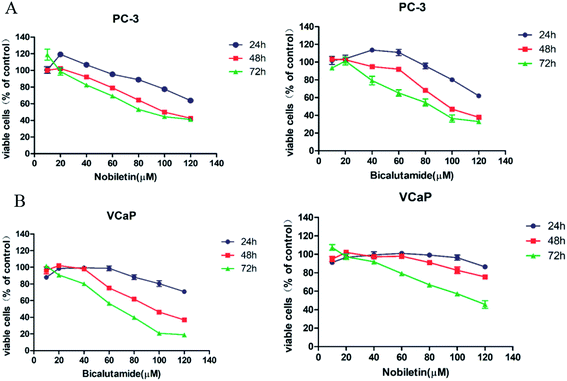 |
| Fig. 2 Inhibitory effect of NBT and BCT on the growth of prostate cancer cell lines. Cells were treated with NBT and BCT for 24 h, 48 h, 72 h, and cell viability was measured using MTT assay. Figure showing reduced viability of PC-3 (A) and VCaP (B) upon NBT or BCT exposure. Data shown are mean ± SEM; n = 5. | |
Combination treatment of NBT and BCT synergistically inhibit the viability of both PC-3 and VCaP cells
For the combination studies, we chose NBT and BCT to incubate PC-3 cells and VCaP cells at concentrations ranging from 20 to 50 μM with a 1
:
1 ratio using MTT assay. Fig. 3A shows that a greater inhibit viability effect was observed with NBT and BCT used together to treat PC-3 and VCaP cells than NBT or BCT alone. The fraction affected versus combination index (FA–CI) curve shown in Fig. 3B demonstrates a synergistic (CI < 1) cytotoxic effect of NBT combined with BCT, with CI values in PC-3 cells ranging from 0.602 to 0.87 and in VCaP cells ranging from 0.81 to 1.15 (Table 1). Moreover, we selected lower concentrations (20 and 30 μM) in the trypan blue exclusion assay to determine the effect of NBT/BCT combination treatment on PC-3 and VCaP cell viability. As shown in Fig. 3C, combinations of NBT and BCT had more potent inhibitory effect on cell viability than either compound used alone. According to the trypan blue exclusion assay, the CI value in PC-3 cells was 0.67 (20 μM) and 0.64 (30 μM), VCaP cells was 0.81 (20 μM) and 0.69 (30 μM). For this result, the combination effect of NBT and BCT on viable cells in PC-3 cells was greater than that in VCaP cells. The combination group of 30 μM show more effective of inhibited viability and had a lower CI than 20 μM. Cell morphology observed under the microscope is shown Fig. 3D. In additional experiments, we determined the effect of the combination on viability of non-tumorigenic epithelial HaCaT cells and found that the combination treatment only had a small inhibitory effect on the cell viability of HaCaT cells (Fig. 3E). Based on this result, we used 30 μM concentration of NBT and BCT alone or in combination for the following experiment.
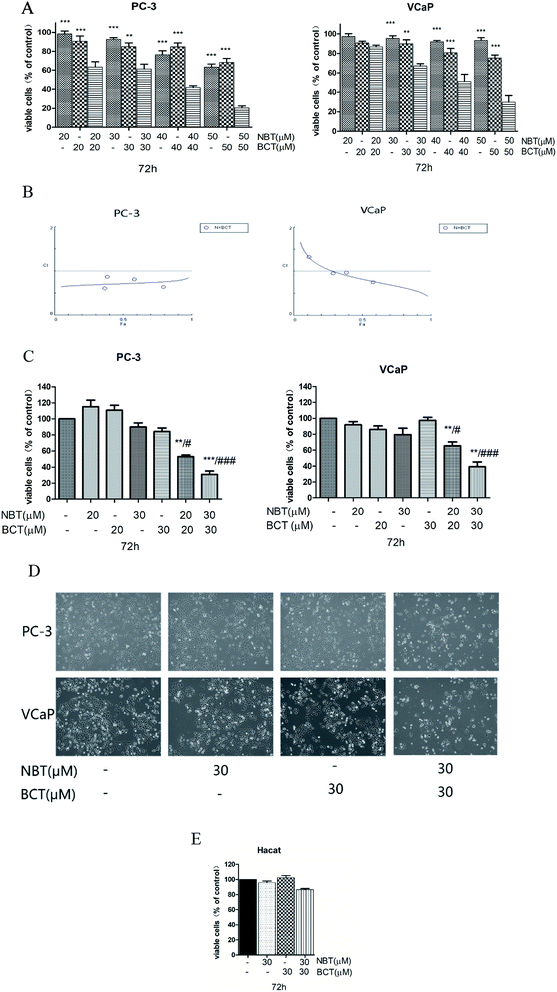 |
| Fig. 3 NBT and BCT alone or in combination showing their effect on cell viability in prostate cancer cells. Cell viability was assessed using MTT after treating cells with different concentrations of NBT and/or BCT for 72 h (A). Data shown are mean ± SEM; n = 5. **p < 0.01, ***p < 0.001, significantly different from combination group. Combination index analysis for the cell viability in PC-3 and VCaP cells treated with the combination of NBT and BCT (B). (C) Cell viability in the various cell lines treated with NBT and/or BCT for 72 h by trypan blue exclusion assay. Data shown are mean ± SEM; n = 3. **p < 0.01, ***p < 0.001, significantly different from NBT-treated group, #p < 0.05, ###p < 0.001 significantly different from BCT-treated group. (D) Cell morphology under a microscope treated with NBT and BCT alone or combination. (E) Cell viability of HaCaT cells after treating cells with NBT and/or BCT for 72 h. | |
Table 1 Combination index (CI) analysis of the viable in PC-3 and VCaP cells treated with the combination of NBT and BCT
NBT (μM) |
BCT (μM) |
CI |
PC-3 |
VCaP |
20 |
20 |
0.602 |
1.15 |
30 |
30 |
0.87 |
1.00 |
40 |
40 |
0.80 |
0.98 |
50 |
50 |
0.63 |
0.81 |
Combination treatment of NBT and BCT induced PC-3 and VCaP cell apoptosis and inhibited colony formation
We assessed the effects of NBT and BCT alone or in combination on the apoptosis of two prostate cancer cells by morphologically assessing these cells, which were stained with PI. Apoptotic cells were identified according to classic morphological features such as nuclear condensation, cell shrinkage, and the formation of apoptotic bodies.26 Morphologically distinct apoptotic cells from the representative samples are shown in Fig. 4A. In both cell lines, individual treatment with BCT and NBT led to a minor enhancement of apoptosis; however, there was a stronger increase of apoptotic cells treated with the combination. In addition, colony formation by PC-3 and VCaP was significantly reduced when the cells were treated with both NBT and BCT, as shown in Fig. 4B.
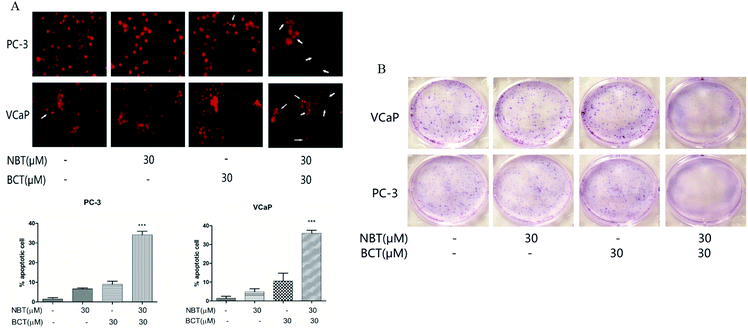 |
| Fig. 4 Effects of NBT and BCT alone or in combination on apoptosis and colony formation of prostate cancer cells. Apoptotic cells were determined by propidium iodide staining (A). Data shown are mean ± SEM; n = 3. ***p < 0.001 between the combination group and the NBT or BCT-treated group. Effect of combined treatment on colony formation. Cell were treated for 24 h and stained with Giemsa on day 5 (B). | |
Combination treatment of NBT and BCT inhibited cell migration of both PC-3 and VCaP cells
The effects of NBT and BCT alone or in combination on the migration of VCaP and PC-3 cells was determined using a scratch wound-healing assay. We treated PC-3 and VCaP cells with NBT/BCT 30 μM alone or in combination for 24 h. As shown in Fig. 5, we observed that treatment with NBT or BCT alone in 30 μM slightly reduced cell motility. However, combination treatment with NBT and BCT significantly inhibited the migration of VCaP and PC-3 cells.
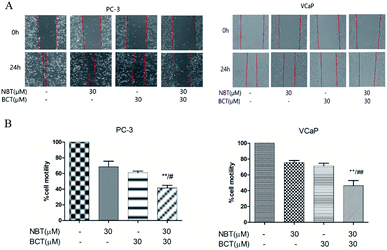 |
| Fig. 5 Effects of NBT and BCT in combination on the migration of prostate cancer cells. For cell migration assays, micrographs were obtained at 0 h and 24 h after scratch. Representative micrographs of cells treated with NBT and/or BCT. Migration of cells was determined and expressed as percentages of the original scratch area. Data shown are mean ± SEM; n = 3. **p < 0.01 between the combination group and the NBT-treated group, #p < 0.05, ##p < 0.05 between the combination group and BCT-treated group. | |
Combination treatment of NBT and BCT inhibited NF-κB transcriptional activity and expression of related proteins
A luciferase reporter gene expression assay was used to determine the effect of NBT and BCT when used alone or in combination on the activation of NF-κB. Since growth inhibition and apoptosis usually occur at later time point after inhibition of NF-κB signaling pathway, we determined the NF-κB activity at 24 h after the cells were treated with NBT and BCT. In the experiments, PC-3/N and VCaP/N cells were treated with NBT and BCT individually or in combination for 24 h, and the activity of NF-κB in prostate cancer cells was reflected by luciferase activity. As shown in Fig. 6A, treatment of PC-3/N and VCaP/N cells with NBT or BCT alone caused a modest decrease in luciferase activity in PC-3/N and VCaP/N cells, and the combinations of NBT and BCT had a stronger effect than either agent alone. It is worth noting that the combination treatment had a greater effect on the PC-3 cells than the VCaP cells. We further detected the levels of phospho-p65 (p-p65; a protein of the NF-κB family), and the NF-κB downstream targets Bcl-2 and survivin27,28 in PC-3 cells by western blot analysis. As depicted in Fig. 6B, the results show that combination treatment with NBT and BCT had even stronger effect on decreasing p-p65 and survivin levels than either alone; however, the combination treatment seemed to have no significant effect on the expression of Bcl-2.
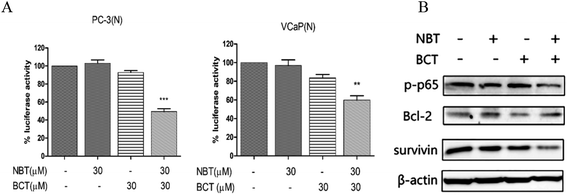 |
| Fig. 6 Effect of NBT and BCT in combination on NF-κB activity and the levels of p-p65, Bcl-2 and survivin. For the assay of NF-κB activity, the luciferase activity was measured and expressed as a percentage relative to the control (A). Data shown are mean ± SEM; n = 3. ***p < 0.001 between the combination group and the NBT-treated group, ##p < 0.01, ###p < 0.001 between the combination group and BCT-treated group. Western blotting was performed to determine the levels of p-p65, Bcl-2 and survivin (B). | |
Combination treatment of NBT and BCT inhibited the levels of p-STAT3/STAT3 and p-Erk/Erk in PC-3 cells
The levels of p-STAT3/STAT3 and p-Erk/Erk in PC-3 cells were determined using western blotting. As shown in Fig. 7A, treatment of PC-3 cells with NBT or BCT alone resulted in a moderate to strong decrease in the level of p-Erk1/2, while the combination treatment with NBT and BCT showed a stronger effect on reducing the expression of p-Erk1/2. Furthermore, the cells showed a much stronger inhibition on the expression of c-myc, a downstream target of Erk1/2, when treated with NBT and BCT, while CyclinD1 seemed to show no significant difference. We also detected the expression of p-STAT3/STAT3 in PC-3 cells. As shown in Fig. 7B, the combination of NBT and BCT had a strong inhibitory effect on the expression of p-STAT3/STAT3 than either drug alone.
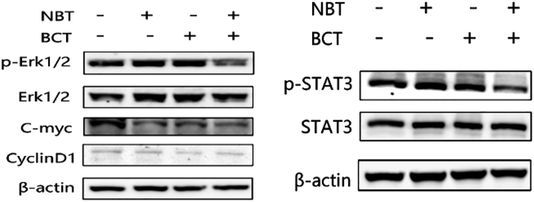 |
| Fig. 7 Effect of NBT and BCT on the level of p-Erk1/2, Erk1/2, c-myc, CyclinD1, p-STAT3, STAT3 in PC-3 cell line. Western blotting was used to check the levels of p-Erk1/2, Erk1/2, c-myc, CyclinD1, p-STAT3, STAT3. | |
Discussion
Majority of advanced prostate cancers are sensitive to androgen deprivation therapy initially, but subsequently progress to CRPC. Due to the limitations of current therapeutic approaches, many patients die of recurrent and metastatic diseases. Natural products are very promising compounds that may be developed as anticancer agents or as adjuvants to one's diet. NBT is one of the main compounds extracted from the plants of Citrus sinensis and Citrus reticulata, which has displayed antitumor activities by inhibiting cell growth, migration, inducing cell-cycle arrest and apoptosis.10,29,30 In this study we demonstrated that NBT enhanced the efficacy of BCT against prostate cancer cells, especially in androgen-independent prostate cancer cells.
BCT is widely accepted as an androgen receptor antagonist and shows efficacious pharmacological activity in androgen-dependent prostate cancer cells. Our result showed that BCT had greater inhibitory effect on viability in VCaP than in PC-3 cells. Interestingly, our study shows that BCT exhibits anticancer activity not only in androgen-dependent prostate cancer cells, but also in androgen-independent prostate cancer cells. These findings agree with earlier studies reported.6,25 One of studies reveals that the mechanisms of BCT-induced apoptosis in androgen-independent prostate cancer cells is partially reliant on calpain activity and pan-caspase inhibition.31 However, the detailed molecular mechanisms of how bicalutamide inhibits the growth of androgen-independent prostate cancer cells remains unclear. In addition, NBT inhibited the cell viability of both VCaP and PC-3 cells, and NBT showed greater inhibitory effect in PC-3 than in VCaP cells. This result implies that androgen-independent prostate cancer cells were more sensitive with NBT.
Furthermore, NBT has been studied due to it low cytotoxicity and enhances the efficacy of chemotherapeutic agents.5,14 In the present study, we showed that combination treatment of NBT and BCT (at 30 μM group) on normal epithelial cells had a lower cytotoxicity (viable cell inhibition rate was 13% in HaCaT cells, Fig. 3E) than that in prostate cancer cells (viable cell inhibition rate was 31% and 33% in PC-3 and VCaP cell, respectively, Fig. 3A). Reduction of toxicity to normal cells may be achieved by reducing the doses or changing the ratio of the drugs in combination. Future studies on combinations of NBT and BCT with different dose ratios may help find a combination that have even lower toxicity to normal cells and in the meantime retain strong inhibitory effect on prostate cancer cells. In addition, our result shown that NBT enhances the anticancer effects of BCT by inhibiting cell growth and migration as well as inducing apoptosis, especially in androgen-independent prostate cancer cells. These results were similar to those of previous studies in which curcumin enhanced the inhibitory effect of BCT on the growth of androgen-independent prostate cancer cells.6 Our findings imply that the combination of NBT and BCT is a potentially new mechanism for this synergy that is AR-unrelated.
Moreover, we used two different assays to analyze the cell viability and synergistic effect. Although the results of cell viability measured by trypan blue exclusion assay were slightly stronger than that by the MTT assay, they had the same trend. This finding suggests that the trypan blue assay may be more sensitive than the MTT assay in our experiments. In addition, the CI value of trypan blue assay is lower than MTT assay. It is possible that the combination of NBT and BCT had a stronger effect on decreasing cell membrane integrity than decreasing mitochondrial enzyme activity. The mechanisms of action for NBT and BCT on mitochondrial and cell membrane in prostate cancer cell need further study. Both of MTT assay and trypan blue exclusion assay have shown that NBT and BCT synergistically inhibited the viability of prostate cancer cells.
The NF-κB transcription factor has been recognized as a major effector of initiation and progression of prostate cancer as well as regulates the inflammatory response, apoptosis, and metastasis seen in prostate cancer.27,28 The activation of inflammatory response can promote the occurrence and development of tumor.28,32 Studies shown that NBT exhibited great anti-inflammatory activity via down-regulating NF-κB activity.33,34 Moreover, NBT shown inhibits cancer activity via mediating NF-κB signaling.11,35 Upregulation of NF-κB occurs in castrate-resistant patients and inhibition of NF-κB activity leads to a decrease in cellular proliferation and induction of apoptosis.36 In addition, targeting NF-κB signaling could restores sensitivity of prostate cancer cells to BCT, especially in CRPC cells.6,37 Since inhibition of the NF-κB signaling pathway by NBT and BCT may occur before the onset of growth inhibition and apoptosis, we determined activation of NF-κB and expression of its downstream anti-apoptosis protein Bcl-2 and survivin at 24 h after the cells were treated with NBT and BCT. We found that the combination of NBT and BCT strongly inhibited NF-κB activation as evidenced by decreases in NF-κB luciferase reporter activity and decreased level of p-p65 protein. Furthermore, the expression of survivin was inhibited by the combination but the combination did not have any effect on the level of Bcl-2. One study found that inhibiting survivin expression will enhance sensitivity to antiandrogen therapy.38 These results imply that the combination of NBT and BCT exerts its anti-prostate cancer cell activity by inhibiting NF-κB and survivin.
The STAT family play an important role in cell proliferation, survival, and differentiation especially STAT3.32 Study show that STAT3 becomes persistently activated in a high percentage of solid and hematopoietic malignancies.39 NBT exhibited activity of inhibit cancer cells viability, migration and angiogenesis via regulating STAT3 expression.40,41 Besides, the interaction between STAT3 and NF-κB plays a crucial role in the tumorigenesis,32 targeting STAT3 and NF-κB offer new chemotherapeutic approaches. In our study, the combination treated with NBT and BCT not only show great inhibit in NF-κB but also in the expression of p-STAT3/STAT3.
ERK is one of the three key members of the MAPK family and plays a central role in normal prostatic epithelial proliferation. In addition, ERK is associated with the proliferation of cancer cells and tumor invasion via degradation of extracellular matrix proteins.42 Review studies documented the ERK can activate transcription factors, such as c-myc and NF-κB.43 This makes ERK1/2 an important anti-prostate cancer target. One study shown that ERK1/2 involved in the activation of AR mutation induced by treatment with BCT in prostate cancer cells.44 Inhibited the activity of ERK1/2 may enhanced the anti-prostate cancer effect of BCT. Some studies have shown that NBT induces cell-cycle arrest and apoptosis by suppressing MAPK pathways.12,45 In the present study, we showed that there was no significant change in the inhibition of ERK expression at low concentrations of NBT and that combination treatment with BCT significantly inhibits the expression of p-ERK/ERK and the downstream targets of c-myc. Our results indicate that the combination of NBT and BCT inhibit cell viability and induce apoptosis involved in a down modulation of NF-κB, STAT3, and ERK in prostate cancer cells.
In conclusion, the results of the current study demonstrate that the combination of NBT and BCT strongly inhibits cell viability, migration and induces apoptosis in human prostate cancer cells. Moreover, mechanism studies have shown that the anti-prostate cancer activity of NBT and BCT together are involved in inhibition of multi-targets, such as NF-κB, STAT3 and ERK. The clearer molecular mechanism of NBT combined with BCT against prostate cancer needs to be further studied. This study suggests that the combination of NBT and BCT may be a novel strategy in the treatment of prostate cancer.
Conflicts of interest
The authors declare no conflict of interest.
Acknowledgements
The present study was supported by grants from the Rutgers Cancer Institute of New Jersey (CCSG P30-CA072720), the Department of Education of Guangdong Province (2016KCXTD005, 2017KZDXM084, 2017KSYS010) and the Jiangmen Program for Innovative Research Team (2017TD02). Dr Xi Zheng is the Unilever Chair in Nutrition and Disease Prevention Research at Rutgers University.
References
- R. L. Siegel, K. D. Miller and A. Jemal, Ca-Cancer J. Clin., 2019, 69, 7–34 CrossRef PubMed.
- K. Mokbel, U. Wazir and K. Mokbel, Anticancer Res., 2019, 39, 5231–5259 CrossRef PubMed.
- M. C. Hodgson, I. Astapova, A. N. Hollenberg and S. P. Balk, Cancer Res., 2007, 67, 8388–8395 CrossRef PubMed.
- E. D. Crawford, P. F. Schellhammer, D. G. McLeod, J. W. Moul, C. S. Higano, N. Shore, L. Denis, P. Iversen, M. A. Eisenberger and F. Labrie, J. Urol., 2018, 200, 956–966 CrossRef PubMed.
- X. Wu, M. Song, P. Qiu, K. Rakariyatham, F. Li, Z. Gao, X. Cai, M. Wang, F. Xu, J. Zheng and H. Xiao, Carcinogenesis, 2017, 38, 455–464 CrossRef PubMed.
- J. Li, S. Xiang, Q. Zhang, J. Wu, Q. Tang, J. Zhou, L. Yang, Z. Chen and S. S. Hann, J. Exp. Clin. Cancer Res., 2015, 34, 46 CrossRef PubMed.
- N. Zhang, Z. Yang, S. Z. Xiang, Y. G. Jin, W. Y. Wei, Z. Y. Bian, W. Deng and Q. Z. Tang, Mol. Cell. Biochem., 2016, 417, 87–96 CrossRef PubMed.
- G. Yang, C. C. Lin, Y. Yang, L. Yuan, P. Wang, X. Wen, M. H. Pan, H. Zhao, C. T. Ho and S. Li, J. Agric. Food Chem., 2019, 67, 6169–6176 CrossRef PubMed.
- A. Nakajima and Y. Ohizumi, Int. J. Mol. Sci., 2019, 20(3380), 1–14 Search PubMed.
- J. X. H. Goh, L. T. Tan, J. K. Goh, K. G. Chan, P. Pusparajah, L. H. Lee and B. H. Goh, Cancers, 2019, 11 Search PubMed.
- N. Sp, D. Y. Kang, D. H. Kim, J. H. Park, H. G. Lee, H. J. Kim, P. Darvin, Y. M. Park and Y. M. Yang, Nutrients, 2018, 10(772), 1–15 Search PubMed.
- P. C. Hsiao, W. J. Lee, S. F. Yang, P. Tan, H. Y. Chen, L. M. Lee, J. L. Chang, G. M. Lai, J. M. Chow and M. H. Chien, Tumor Biol., 2014, 35, 11903–11911 CrossRef PubMed.
- J. Y. Moon and S. K. Cho, Molecules, 2016, 21(914), 1–13 Search PubMed.
- W. Ma, S. Feng, X. Yao, Z. Yuan, L. Liu and Y. Xie, Sci. Rep., 2015, 5, 18789 CrossRef PubMed.
- G. Guney Eskiler, A. O. Deveci, C. Bilir and S. Kaleli, Nutr. Cancer, 2019, 71, 1299–1312 CrossRef PubMed.
- X. T. Xu, X. Y. Deng, J. Chen, Q. M. Liang, K. Zhang, D. L. Li, P. P. Wu, X. Zheng, R. P. Zhou, Z. Y. Jiang, A. J. Ma, W. H. Chen and S. H. Wang, Eur. J. Med. Chem., 2019, 189, 112013 CrossRef PubMed.
- Y. Zhong, L. Yu, Q. He, Q. Zhu, C. Zhang, X. Cui, J. Zheng and S. Zhao, ACS Appl. Mater. Interfaces, 2019, 11, 32769–32777 CrossRef.
- S. Jiang, K. Zhang, Y. He, X. Xu, D. Li, S. Cheng and X. Zheng, RSC Adv., 2018, 8, 2768–2776 RSC.
- P. Wang, S. M. Henning and D. Heber, PLoS One, 2010, 5, e10202 CrossRef.
- W. Strober, Curr. Protoc. Immunol., 2001, A.3B.1–A.3B.2 Search PubMed.
- H. Huang, Y. He, L. Zhang, H. Xiang, D. Li, W. Liu, X. T. Xu, S. Goodin, K. Zhang and X. Zheng, Food Funct., 2018, 9, 2398–2408 RSC.
- H. Huang, X. Chen, D. Li, Y. He, Y. Li, Z. Du, K. Zhang, R. DiPaola, S. Goodin and X. Zheng, PLoS One, 2015, 10, e0144293 CrossRef.
- T. C. Chou, Cancer Res., 2010, 70, 440–446 CrossRef.
- N. A. Colabufo, V. Pagliarulo, F. Berardi, M. Contino, C. Inglese, M. Niso, P. Ancona, G. Albo, A. Pagliarulo and R. Perrone, Eur. J. Pharmacol., 2008, 601, 38–42 CrossRef.
- K. Kavya, M. N. Kumar, R. H. Patil, S. M. Hegde, K. M. Kiran Kumar, R. Nagesh, R. L. Babu, G. T. Ramesh and S. Chidananda Sharma, Mol. Cell. Biochem., 2017, 433, 13–26 CrossRef.
- L. Yu, X. Wu, M. Chen, H. Huang, Y. He, H. Wang, D. Li, Z. Du, K. Zhang, S. Goodin and X. Zheng, Int. J. Med. Sci., 2017, 14, 356–366 CrossRef.
- G. Jain, M. V. Cronauer, M. Schrader, P. Moller and R. B. Marienfeld, World J. Urol., 2012, 30, 303–310 CrossRef.
- D. P. Nguyen, J. Li, S. S. Yadav and A. K. Tewari, BJU Int., 2014, 114, 168–176 CrossRef.
- J. Chen, A. Creed, A. Y. Chen, H. Huang, Z. Li, G. O. Rankin, X. Ye, G. Xu and Y. C. Chen, BMC Pharmacol. Toxicol., 2014, 15, 59 CrossRef.
- P. Y. Chen, Y. T. Chen, W. Y. Gao, M. J. Wu and J. H. Yen, J. Agric. Food Chem., 2018, 66, 13423–13434 CrossRef.
- M. S. Floyd, Jr, S. J. Teahan, J. M. Fitzpatrick and R. W. Watson, Prostate Cancer Prostatic Dis., 2009, 12, 25–33 CrossRef PubMed.
- Y. Fan, R. Mao and J. Yang, Protein Cell, 2013, 4, 176–185 CrossRef CAS PubMed.
- Z. Lin, D. Wu, L. Huang, C. Jiang, T. Pan, X. Kang and J. Pan, Front. Pharmacol., 2019, 10, 570 CrossRef.
- G. Qi, Y. Mi, R. Fan, R. Li, Z. Liu and X. Liu, J. Agric. Food Chem., 2019, 67, 5122–5134 CrossRef CAS.
- J. Liu, S. Wang, S. Tian, Y. He, H. Lou, Z. Yang, Y. Kong and X. Cao, Food Nutr. Res., 2018, 62(323), 1–10 Search PubMed.
- P. McCall, L. Bennett, I. Ahmad, L. M. Mackenzie, I. W. Forbes, H. Y. Leung, O. J. Sansom, C. Orange, M. Seywright, M. A. Underwood and J. Edwards, Br. J. Cancer, 2012, 107, 1554–1563 CrossRef CAS.
- J. J. Nunes, S. K. Pandey, A. Yadav, S. Goel and B. Ateeq, Neoplasia, 2017, 19, 333–345 CrossRef CAS.
- M. Zhang, D. E. Latham, M. A. Delaney and A. Chakravarti, Oncogene, 2005, 24, 2474–2482 CrossRef CAS.
- F. Laudisi, F. Cherubini, G. Monteleone and C. Stolfi, Int. J. Mol. Sci., 2018, 19(1787), 1–21 Search PubMed.
- D. Wei, G. Zhang, Z. Zhu, Y. Zheng, F. Yan, C. Pan, Z. Wang, X. Li, F. Wang, P. Meng, W. Zheng, Z. Yan, D. Zhai, Z. Lu and J. Yuan, Front. Pharmacol., 2019, 10, 690 CrossRef.
- N. Sp, D. Y. Kang, Y. H. Joung, J. H. Park, W. S. Kim, H. K. Lee, K. D. Song, Y. M. Park and Y. M. Yang, Int. J. Mol. Sci., 2017, 18(935), 1–13 Search PubMed.
- W. Zhu, Y. Xue, C. Liang, R. Zhang, Z. Zhang, H. Li, D. Su, X. Liang, Y. Zhang, Q. Huang, M. Liu, L. Li, D. Li, A. Z. Zhao and Y. Liu, Tumor Biol., 2016, 37, 12241–12250 CrossRef CAS.
- C. Braicu, M. Buse, C. Busuioc, R. Drula, D. Gulei, L. Raduly, A. Rusu, A. Irimie, A. G. Atanasov, O. Slaby, C. Ionescu and I. Berindan-Neagoe, Cancers, 2019, 11 Search PubMed.
- T. Terakawa, H. Miyake, M. Kumano, I. Sakai and M. Fujisawa, Oncol. Rep., 2010, 24, 1395–1399 CAS.
- H. L. Cheng, M. J. Hsieh, J. S. Yang, C. W. Lin, K. H. Lue, K. H. Lu and S. F. Yang, Oncotarget, 2016, 7, 35208–35223 Search PubMed.
|
This journal is © The Royal Society of Chemistry 2020 |
Click here to see how this site uses Cookies. View our privacy policy here.