DOI:
10.1039/C9RA10463A
(Paper)
RSC Adv., 2020,
10, 10263-10276
Regio- and stereoselective synthesis of spiropyrrolidine-oxindole and bis-spiropyrrolizidine-oxindole grafted macrocycles through [3 + 2] cycloaddition of azomethine ylides†
Received
12th December 2019
, Accepted 21st February 2020
First published on 10th March 2020
Abstract
A convenient and efficient method for the regioselective macrocyclization of triazole bridged spiropyrrolidine-oxindole, and bis-spiropyrrolizidine-oxindole derivatives was accomplished through intra and self-intermolecular [3 + 2] cycloaddition of azomethine ylides. The chalcone isatin precursors 9a–i required for the click reaction were obtained from the reaction of N-alkylazidoisatin 4 and propargyloxy chalcone 8a–i which in turn were obtained by the aldol condensation of propargyloxy salicylaldehyde 6 and substituted methyl ketones 7a–i. The regio- and stereochemical outcome of the cycloadducts were assigned based on 2D NMR and confirmed by single crystal XRD analysis. High efficiency, mild reaction conditions, high regio- and stereoselectivity, atom economy and operational simplicity are the exemplary advantages of the employed macrocyclization procedure.
Introduction
Macrocyclic molecules have received significant attention because of varied ring size and chemical constitution commonly found in natural products and pharmaceutical molecules, and such molecules have potential application in chemistry, biology, nanotechnology and medical fields.1,2 Further, heteroatom-containing macrocyclic molecules have privileged structural units that are essential in the area of drug discovery.3 The druglike “rule of five” properties are not obeyed by the macromolecules and the improved binding properties, unique stereochemical and structural feature and conformational flexibility make macrocycle molecules function as important biological targets.4,5 Moreover, proteins with various functional groups in preorganized three-dimensional constellation can increase the catalytic efficiency in enzyme active sites and molecular recognition in protein binding sites. Therefore, there is acute necessity for the development of synthetic macrocyclic scaffolds that can be used for the preorganized constellation of functional groups for catalytic and molecular recognition applications.6 Hence, macrocyclic compounds of the following type such as calixarenes,7,8 cyclodextrin,9,10 cucurbiturils,11 resorcinarenes,12,13 conjugated aromatic systems,14 and Schiff base macrocycles,15–17 are important from the synthetic view point. Noncovalent self-assembly18 of coordination bonds in organometallics19,20 functioning as sensors for the recognition of selective ions in host gest chemistry21 make macrocyclic molecules an important class of supramolecules.
Spiro compounds comprise two rings fused at a central spiro atom, and possess a unique three dimensional architecture. Synthetic chemists have been fascinated by spirocycles for more than hundred years and the first spiran was synthesized by Baeyer in 1900.22 Spirocyclic oxindoles occupy a privileged position in organic and diversity-oriented synthesis,23 and attract a wide range of natural and synthetic products and display a variety of bioactivities and present in pharmaceutically active compounds24,25 In particular multifunctional polycyclic spiropyrrolidine-oxindole and spiropyrrolizidine-oxindole are the privileged heterocyclic skeleton which contain spiro stereocenter at the C3 position of the oxindole frequently encountered in natural alkaloids and in important structural moieties exhibiting versatile bioactivities,26 such as antibacterial, anticancer, antimicrobial, antimycobacterial, antitumor, antifungal, acetylcholinesterase (AChE) inhibitory properties and cholinesterase inhibitory activity.27–29 The spirooxindoles unit occurs in several natural products, such as (−)-horsfiline, coerulescene, elacomine, spirotryprostatin A, peteropodine, rhynchophylline, and also in spirooxindole alkaloids (Fig. 1). Spiro pyrrolidine-3,3-oxindole alkaloids also shows anticancer activity and inhibition of p53-MDM2 protein–protein interaction with rapid regression of tumor cells.30 Thus, the anticancer effect of spirooxindole natural products allow more significant non-natural analogues design. Presently, MI-77301 (SAR405838) is yet another promising anticancer drug under clinical trials.31
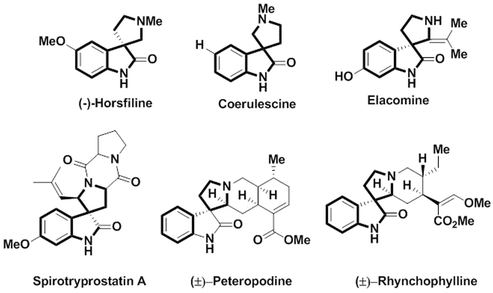 |
| Fig. 1 Representative examples of naturally occurring bioactive spiropyrrolidine-oxindole alkaloids. | |
1,3-Dipolar cycloaddition32 of azomethine ylide is a powerful method for the synthesis of biologically significant five-membered heterocyclic compounds. Electron-deficient alkenes are employed as dipolarophiles in the presence of either transition metals or organo catalysts to achieve highly functionalized and stereochemically enriched pyrroldine, pyrrolizidine and spirocyclic oxindole molecules.33 The simplicity, atomic economy, mild reaction conditions and extension of the scope of substrates make such cycloaddition as the current direction in combinatorial chemistry. Driven thorough literature survey, we are encouraged to construct hybrid macrocyclic systems. However, the extensive applications of 1,3-dipolar cycloaddition for the synthesis of such macrocycles still remains a challenge.34 To the best of our knowledge there is no report in the literature on a one pot self-intermolecular [3 + 2] cycloaddition to give bis-spiropyrrolizidine-oxindole grafted macrocyles.
We disclose a novel method for the synthesis and electrochemical property and biological activity35 of 1,2,3-triazole bridged pyrrolidine grafted macrocycles with high regio- and stereoselectivity via [3 + 2] cycloaddition approach. We wish to report herein the synthesis of novel spiropyrrolidine oxindole grafted macrocyles 12a–i (Fig. 2) and bis-spiropyrrolizidine oxindole grafted macrocycles 16a–i (Fig. 3) through intra and self-intermolecular [3 + 2] cycloaddition of azomethine ylides (AMY) generated in situ by the decarboxylative condensation of cyclic ketones and secondary amino acids.
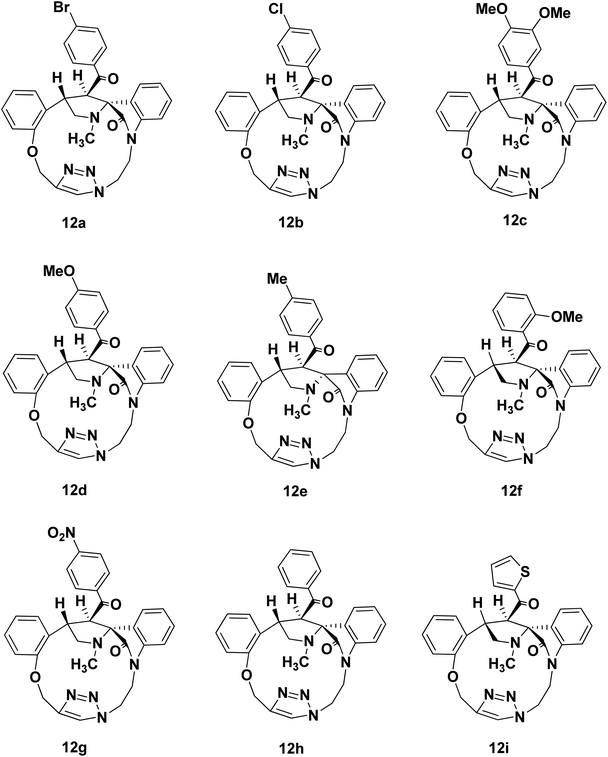 |
| Fig. 2 Molecular structure of spiropyrrolidine-oxindole grafted macrocyles 12a–i. | |
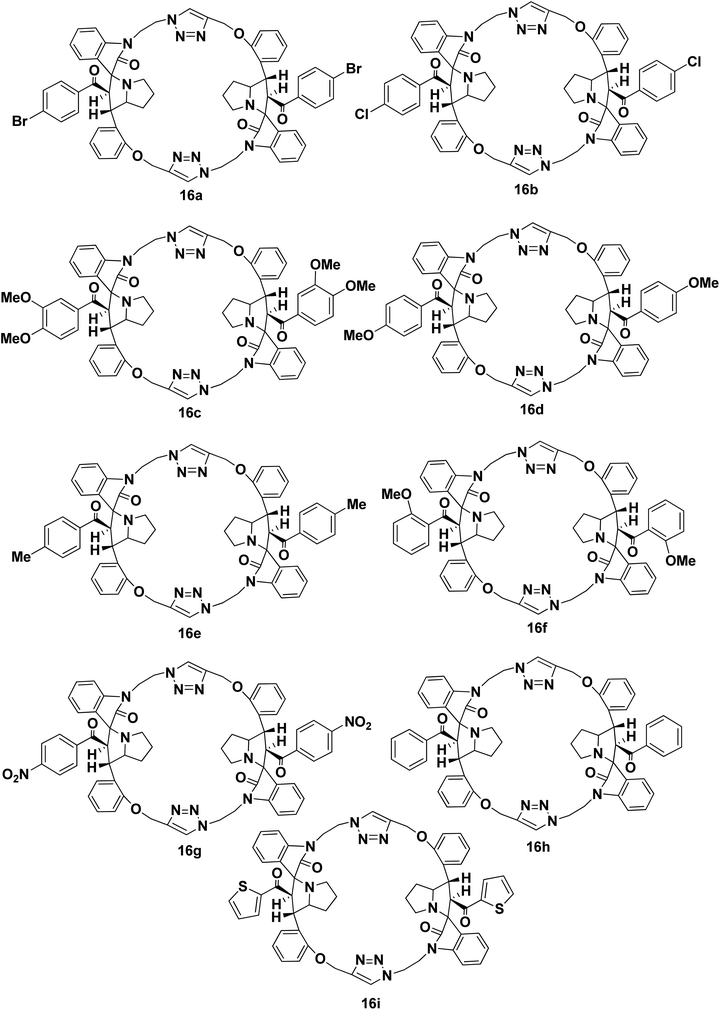 |
| Fig. 3 Molecular structure of bis-spiropyrrolizidine-oxindole grafted macrocyles 16a–i. | |
Results and discussion
The synthetic plan for achieving the spiropyrrolidine-oxindole grafted macrocycles 12a–i is shown in Scheme 1. The macrocyclic spiropyrrolidine-oxindole 12a–i can be obtained by the intramolecular (3 + 2) cycloaddition of chalcone isatins 9a–i with the azomethine ylide generated from the corresponding amino acids. The chalcone isatins 9a–i can be derived from the click reaction of the propargyloxy chalcone 8a–i with the azido fragment derived from isatin. The propargyloxy chalcone 8a–i can be prepared by the O-propargylation of salicylaldehyde with propargyl bromide followed by the aldol condensation using suitable methyl aryl ketone (Scheme 1).
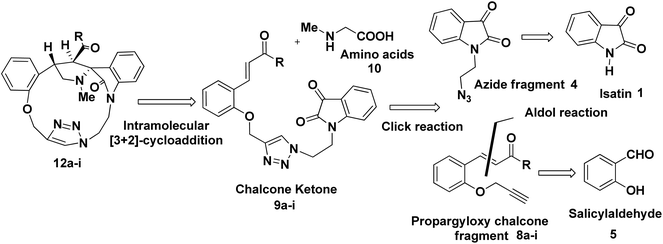 |
| Scheme 1 Synthetic plan for spiropyrrolidine-oxindole grafted macrocycles 12a–i. | |
Hence we began the synthesis of the desired azido compound 4 from isatin (1). Reaction of isatin (1) with one equivalent of 1,2-dibromoethane in the presence of K2CO3 in DMF at room temperature gave the N-alkylbromo ketone 3 in 58% yield along with the bisalkylated tetraketone 2 in 24% yield. The monobromoketone 3 was smoothly converted to the desired azide 4 in 93% by NaN3 in DMF at room temperature (Scheme 2). The structure of the N-alkylazidoketone 4 was confirmed from 1H and 13C NMR spectra. Synthesis of O-propargylated chalcone fragments 8a–i was achieved from salicylaldehyde (5) by adopting a reported procedure35 (Scheme 2). Reaction of salicylaldehyde (5) with 1.2 equiv. of propargyl bromide in the presence of potassium carbonate in DMF at room temperature gave propargyloxy salicylaldehyde 6 in 92% yield, which on further aldol condensation with 1.2 equiv. of each of the various substituted aromatic and heteroaromatic methyl ketones viz., 4-bromoacetophenone 7a, 4-chloroacetophenone 7b, 3,4-dimethoxyacetophenone 7c, 4-methoxyacetophenone 7d, 4-methylacetophenone 7e, 2-methoxyacetophenone 7f, 4-nitroacetophenone 7g, acetophenone 7h and 2-acetylthiophene 7i in the presence of 20% aqueous NaOH solution in EtOH gave the substituted chalcone derivatives 8a–i in 75–85% yields.
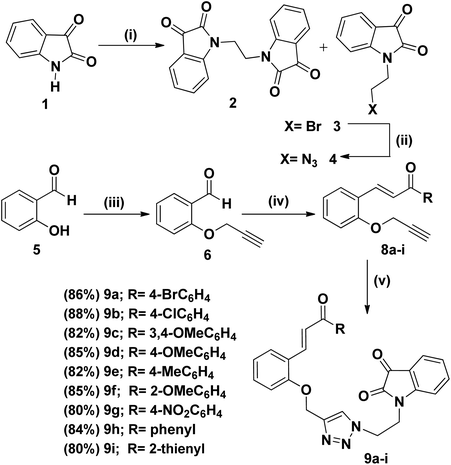 |
| Scheme 2 Synthesis of 1,2,3-triazole bridged chalcone isatin 9a–i. Reagent and conditions: (i) 1,2-dibromoethane, K2CO3, DMF, rt, 2 h, 2 (24%), 3 (58%); (ii) NaN3, DMF, rt, 8 h, 4 (93%); (iii) propargyl bromide, K2CO3, DMF, rt, 12 h, 6 (92%); (iv) methyl ketones 7a–i, NaOH, EtOH, rt, 6 h 8a–i (75–85%). (v) N-Alkylazidoketone 4, CuSO4·5H2O, sodium ascorbate, THF : water (1 : 1), rt, 9a–i (80–90%). | |
In convergent approach, the 1,3-dipolar cycloaddition of propargyloxychalcone derivatives 8a–i with 1.0 equiv. of N-alkylazidoketone 4 under click reaction conditions using CuSO4·5H2O and sodium ascorbate in THF
:
H2O as solvent gave the 1,4-triazole bridged chalcone isatin 9a–i in 80–90% yields (Scheme 2). The structure of the 1,4-triazole bridged chalcone isatin 9a–i was characterized from spectroscopic methods. In the 1H NMR spectrum of 9a the triazole –CH– proton resonated at δ 7.75 as a singlet. The geometry of the olefinic double bond showed the E configuration as evidenced from 1H NMR spectrum. The olefinic protons of the chalcone isatin 9a appeared as a doublet at 7.99 with a coupling constant of 15.9 Hz which confirms the E configuration of the double bond. In the 13C NMR spectrum of 9a, the carbonyl carbons exhibited peaks at 182.2 and 189.7 ppm.
To optimize the conditions for 1,3-dipolar cycloaddition, the cycloaddition of the chalcone isatin 9a and sarcosine was chosen as the model reaction (Table 1). The reaction mixture with equimolar amounts of chacone isatin 9a and sarcosine (10) was tested under various reaction conditions. The effects of solvent and temperature on the 1,3-dipolar cycloaddition was evaluated and from the optimization study the best results were obtained by refluxing the reaction mixture in toluene for 6 h in Dean–Stark apparatus to give the regio- and stereoselective cycloadduct 12a in 74% yields. Having established suitable reaction conditions (Table 1, entry 4), we tried to extend the scope of the reaction, using a series of various substituted chalcone isatin 9b–i. Regio- and stereoselective [3 + 2] cycloaddition of azomethine ylide from sarcosine (10) with the substituted chalcone isatin 9b–i gave the cycloadducts 12b–i in 65–75% yields (Scheme 3). The intramolecular dipolarophile O-alkyl enone regioselectively reacts with the azomethine ylide (dipole) in toluene under refluxing conditions for 6 h in Dean–Stark apparatus to give the regio- and stereoselective macrocyclic adducts 12a–i in 65–75% yields.
Table 1 Optimizing the reaction conditions for the synthesis of cycloadduct 12a
Entry |
Solvent |
Temperature (°C) |
Time (h) |
Isolated yield (%) |
1 |
Benzene |
Reflux |
6 |
Trace |
2 |
MeOH |
Reflux |
6 |
34 |
3 |
CH3CN |
Reflux |
6 |
58 |
4 |
Toluene |
Reflux |
6 |
74 |
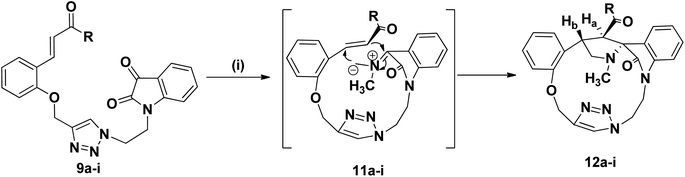 |
| Scheme 3 Synthesis of spiropyrrolidine-oxindole grafted macrocycles 12a–i. Reagent and conditions: (i) sarcosine 10, toluene, N2 atm, Dean– Stark water separator, 6 h, 12a (74%), 12b (75%), 12c (68%), 12d (70%), 12e (68%), 12f (65%), 12g (72%), 12h (70%), 12i (65%). | |
The structure and the regiochemistry of the spiropyrrolidine oxindole grafted macrocyles 12a–i was unambiguously established from spectroscopic data. In the 1H NMR spectrum, the cycloadduct 12a displayed the N-methyl protons of the pyrrolidine ring as a singlet at δ 2.14 and the two diastereotopic N-methylene protons of the pyrrolidine moiety resonated as triplets at δ 3.38 and δ 3.92 (J = 8.1, J = 9.6 Hz). The two O-methylene protons appeared as two doublets at δ 5.09 and 5.37 (J = 10.2 Hz, J = 10.5 Hz). The benzylic proton Hb of the pyrrolidine ring appeared as a multiplet in the region of δ 3.57–3.61. The pyrrolidine Hb proton adjacent to the carbonyl group appeared as a doublet at δ 5.21 (J = 6.9 Hz), which clearly proved the regiochemistry of the cycloaddition. Further, the triazolyl –CH– proton appeared at δ 8.12 as a singlet.
In the 13C NMR spectrum, the cycloadduct 12a showed the N-methyl, N-methylene and carbonyl carbons at δ 34.5, 60.1 and 199.3 ppm respectively. In DEPT-135 13C NMR spectrum, the cycloadduct 12a exhibited the four peaks in the negative region at δ 39.5, 48.7, 60.1 and 62.3 ppm which confirm the presence of four methylene carbons in 12a. Moreover, 1H–1H COSY and 1H–13C COSY experiments were carried out to confirm the structure of regio- and stereoselective isomer 12a (ESI†). The macrocyclic compound 12a showed a peak at m/z 584.1271 (M + H)+ in HRMS (TOF MS ES+ 3.35 × 107). Similarly, the structure of the pyrrolidine grafted macrocycles 12b–i was confirmed from the spectral and analytical data.
The electron withdrawing or electron donating substituents on the phenyl ring of the dipolarophiles tolerated the reaction conditions, leading to the spirooxindolo pyrrolidine macrocycles in good yields. The regioselectivity of the macrocyclization can be demonstrated by the intramolecular secondary orbital interaction (SOI)36 between the orbital of the dipolarophile carbonyl group and dipole azomethine ylide, as shown in Fig. 4. Formation of the observed regioisomer 12a–i through path A is favorable because of the SOI, which is not possible in the transitions state 11′ which could have given the regioisomer 13a–i (path B). The relative stereochemistry at the positions of spiro center of the macrocycles was observed as highly stereocontroled cycloaddition and the favorable SOI results in the formation of the regioisomer 12a–i only.
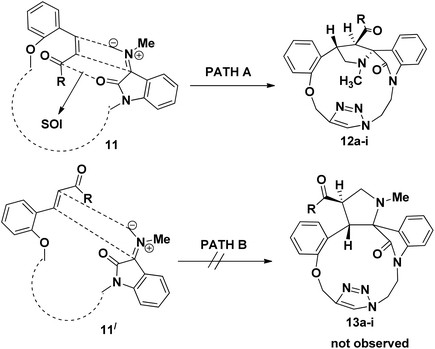 |
| Fig. 4 Regioselectivity due to SOI in the transitions state that leads to the cycloadduct 12a–i. | |
As a logical extension of the methodology, synthesis of bis-spiropyrrolizidine-oxindole grafted macrocycles 16a–i was then focused. Reaction of the triazole bridged chalcone isatin 9a–i with L-proline (14) generated the 1,3-dipolar intermediate which could undergo self-intermolecular [3 + 2] cycloaddition. The azomethine ylides 15a–i underwent smooth self-intermolecular 1,3-dipolar cycloaddition to give the bis-spiropyrrolizidine oxindole grafted macrocyles 16a–i in 55–65% yields (Scheme 4).
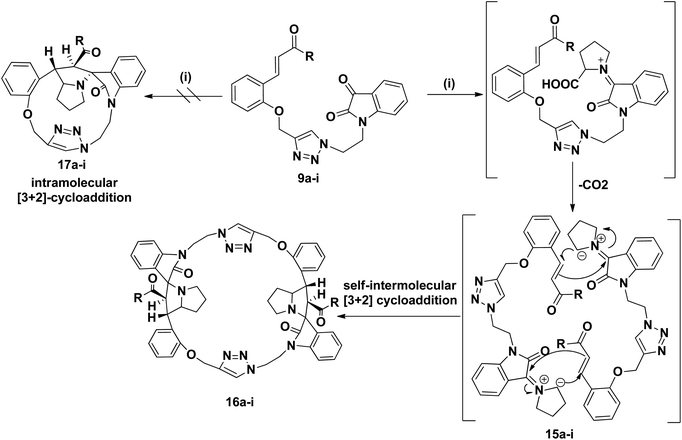 |
| Scheme 4 Synthesis of bis-spiropyrrolizidine-oxindole grafted macrocycles 16a–i. Reagents and conditions: (i) proline (14), toluene, N2 atm, Dean–Stark water separator, 6 h, 16a (64%), 16b (65%), 16c (58%), 16d (60%), 16e (56%), 16f (55%), 16g (62%), 16h (60%), 16i (56%). | |
Optimization of the reaction condition for the formation of the cycloadduct 16a was then focused. Variation with respect to solvent, temperature and reaction time for the formation of 16a was carried out and the resulting yield of 16a is show in Table 2. The best optimized yield of 64% is show in entry 4.
Table 2 Optimizing the reaction conditions for the synthesis of cycloadduct 16a
Entry |
Solvent |
Temperature (°C) |
Time (h) |
Isolated yield (%) |
1 |
Benzene |
Reflux |
6 |
Trace |
2 |
MeOH |
Reflux |
6 |
18 |
3 |
CH3CN |
Reflux |
6 |
32 |
4 |
Toluene |
Reflux |
6 |
64 |
The structure and regioselective of self-intermolecular cycloadducts 16a–i were established from spectroscopic data. The 1H NMR spectrum of the cycloadduct 16b displayed a multiplet at δ 1.68–2.69 for the pyrrolizidine ring methylene protons. The O–CH2– protons appeared as a ABq at δ 5.24, 5.49 (J = 11.7 Hz). A doublet at δ 5.60 (J = 11.7 Hz) was observed for the benzoyl protons. The benzylic proton of the pyrrolidine ring appeared as a multiplet in the region of δ 4.47–4.58, which clearly showed the stereo- and regiochemistry of the self-intermolecular cycloaddition of azomethine ylides. A neat singlet appeared at δ 9.85 corresponding to triazole –CH– proton which proved the presence of a triazole unit. The stereochemistry of the cycloadduct 16b was also deduced on the basis of 2D 1H–1H COSY and 1H–13C COSY (ESI†) experiments. In DEPT-135 13C NMR spectrum, the methylene carbons exhibited six peaks in the negative region at δ 28.4, 32.6, 40.0, 46.6, 47.4 and 64.2 ppm which confirms the presence of six methylene carbons in the macrocycle 16b. Furthermore, the presence of molecular ion peak at m/z 1131.3840 (M + 1) in HRMS, also confirmed the structure of the cycloadduct 16b. The carbonyl carbon exhibited a peak at 196.6 ppm in the 13C NMR spectrum. The remaining carbons resonated at the corresponding ppm values (ESI†). Finally, the structure of the macrocyclic compound 16b was again established from the single crystal XRD analysis (Fig. 5) However, the structure of the bis-spiropyrrolizidine-oxindole grafted macrocycles 16a and 16c–i was confirmed from the spectral and analytical data.
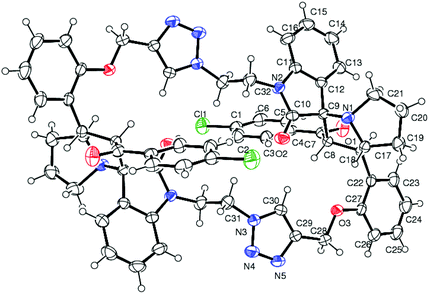 |
| Fig. 5 ORTEP diagram of bis-spiropyrrolizidine-oxindole grafted macrocycle 16b. | |
In conclusion, for the first time we have developed an efficient protocol for the macrocyclizations of spiropyrrolidine oxindole and bis-spiropyrrolizidine oxindole with triazole as spacer unit via regioselective click reaction, intra and self-intermolecular 1,3-dipolar cycloaddition of azomethine ylide derived from sarcosine and proline under thermal decarboxylative condensation by relatively simple procedure. The [3 + 2] cycloaddition methodology was found to be highly regioselective and the structure of the regio- and stereo isomer was determined from 2D NMR and confirmed by XRD studies.
Experimental
General considerations
All reagents and solvents were of analytical grade and used without further purification as obtained from commercial suppliers. Ethanol, N,N-dimethylformamide (DMF), tetrahydrofuran (THF), and toluene were retrieved from a solvent purification system. Routine monitoring of the reaction was done by thin-layer chromatography (TLC) using hexane/ethyl acetate mixtures as eluent. Column chromatography was carried out on Silica gel (100–200 meshes) by using increasing polarity. All melting points are uncorrected. 1H NMR, 13C NMR and DEPT 135 spectra were recorded in CDCl3 using TMS as an internal standard on 300 MHz Bruker instrument. 2D NMR spectra were recorded at 500 MHz spectrometer on Bruker instrument. Chemical shifts are reported in ppm relative to deuterated solvent peaks as internal standards (δH = CDCl3 7.26 ppm, and DMSO-d6 2.50 ppm; δC = CDCl3 77.16 ppm, and DMSO-d6 39.5 ppm). Coupling constants (J) are quoted in Hertz (Hz). The HRMS spectra were recorded on Xevo G2s Qtof (ESI) instruments.
Synthesis of 1,2,3-triazole bridged chalcone isatin 9a–i
(i) General procedure. To solution of propargyloxy chalcones 8a–i (1 eq.) and N-alkylazidoketones 4 (1 eq.) in a mixture of THF (20 mL), and H2O (20 mL), was added CuSO4·5H2O (0.5 eq.) and sodium ascorbate (0.5 eq.). The resulting solution was then stirred for 12 h at room temperature. The solvent was evaporated under vacuum and the residue was dissolved in chloroform (150 mL) and washed with water (50 mL) and brine (50 mL) dried over Na2SO4 and concentrated. The crude product was purified by column chromatography using hexane
:
EtOAc (3
:
2) as eluent to give the chalcone isatin 9a–i.
Chalcone isatin 9a. Yield 86%, orange-yellow solid. Mp: 174–176 °C. 1H NMR (300 MHz, CDCl3): δ 4.23 (t, J = 6.0 Hz, 2H); 4.73 (t, J = 6.0 Hz, 2H); 5.20 (s, 2H); 6.55 (d, J = 7.8 Hz, 1H); 6.97–7.05 (m, 3H); 7.31–7.41 (m, 2H); 7.47–7.51 (m, 1H); 7.56–7.61 (m, 4H); 7.75 (s, 1H); 7.83 (d, J = 8.1 Hz, 2H); 7.99 (d, J = 15.9 Hz, 1H). 13C NMR (75 MHz, CDCl3): δ 40.6, 47.8, 62.4, 109.4, 113.0, 117.5, 121.6, 122.6, 123.9, 124.0, 124.3, 125.5, 127.6, 129.4, 130.1, 131.8, 131.9137.1, 138.4, 140.5, 144.1, 149.9, 157.5, 158.5, 182.2, 189.7.
Chalcone isatin 9b. Yield 88%. Yellow solid. Mp: 162–164 °C. 1H NMR (300 MHz, CDCl3): δ 4.24 (t, J = 5.7 Hz, 2H); 4.72 (t, J = 5.7 Hz, 2H); 5.2 (s, 2H); 6.53 (d, J = 8.1 Hz, 1H); 7.00–7.05 (m, 3H); 7.26–7.41 (m, 2H); 7.43–7.54 (m, 3H); 7.60–7.63 (m, 2H); 7.80 (s, 1H); 7.91 (d, J = 9.9 Hz, 2H); 8.01 (d, J = 15.6 Hz, 1H). 13C NMR (75 MHz, CDCl3): δ 40.6, 47.9, 62.2, 109.4, 112.8, 117.4, 121.5, 122.4, 124.1, 124.2, 125.6, 128.8, 129.3, 129.6, 129.9, 131.9, 136.6, 138.6, 139.1, 140.5, 144.1, 149.9, 157.4, 158.5, 182.3, 189.4.
Chalcone isatin 9c. Yield 82%, orange-yellow solid. Mp: 198–200 °C. 1H NMR (300 MHz, CDCl3): δ 3.95 (s, 3H); 3.96 (s, 3H); 4.23 (t, J = 6 Hz, 2H); 4.73 (t, J = 6 Hz, 2H); 5.22 (s, 2H); 6.50 (d, J = 7.8 Hz, 1H); 6.92 (d, J = 8.4 Hz, 1H); 6.99–7.05 (m, 3H); 7.31–7.40 (m, 2H); 7.49 (d, J = 7.2 Hz, 1H); 7.57–7.65 (m, 4H); 7.80 (s, 1H); 8.01 (d, J = 15.6 Hz, 1H). 13C NMR (75 MHz, CDCl3): δ 40.6, 47.9, 56.09, 56.1, 62.4, 109.3, 110.1, 110.9, 112.9, 117.4, 121.5, 122.5, 123.1, 124.6, 125.6, 128.9, 131.6, 138.5, 139.0, 149.2, 149.9, 153.2, 157.2, 158.5, 182.3, 188.9.
Chalcone isatin 9d. Yield 85%, orange solid. Mp: 158–160 °C. 1H NMR (300 MHz, CDCl3): δ 3.82 (s, 3H); 4.17 (t, J = 5.4 Hz, 2H); 4.72 (t, J = 5.4 Hz, 2H); 5.13 (s, 2H); 6.47 (d, J = 8.1 Hz, 1H); 6.88–7.00 (m, 5H); 7.27–7.34 (m, 3H); 7.39 (t, J = 7.2 Hz, 1H); 7.55–7.60 (m, 2H); 7.90–7.98 (m, 3H). 13C NMR (75 MHz, CDCl3): δ 40.6, 47.8, 55.4, 62.1, 109.5, 112.7, 113.7, 117.3, 121.4, 122.5, 123.9, 124.2, 124.5, 125.3, 129.1, 130.7, 131.0, 131.6, 131.6, 138.5, 138.9, 143.9, 150.0, 157.2, 158.5, 163.3, 182.6, 188.9.
Chalcone isatin 9e. Yield 82%. Orange-yellow solid. Mp: 182–184 °C. 1H NMR (300 MHz, CDCl3): δ 2.38 (s, 3H); 4.19 (t, J = 5.4 Hz, 2H); 4.73 (t, J = 5.4 Hz, 2H); 5.16 (s, 2H); 6.47 (d, J = 7.8 Hz, 1H); 6.91–7.02 (m, 3H). 7.24 (d, J = 7.8 Hz, 2H); 7.28–7.32 (m, 2H); 7.42 (d, J = 7.5 Hz, 1H); 7.55–7.61 (m, 2H); 7.84 (d, J = 8.1 Hz, 2H); 7.94 (s, 1H); 7.99 (d, J = 15.6 Hz, 1H) ppm. 13C NMR (75 MHz, CDCl3): δ 21.6, 40.6, 47.9, 62.2, 109.4, 112.7, 117.3, 121.4, 122.6, 124.0, 124.1, 124.5, 125.4, 128.6, 129.0, 129.3, 131.7, 135.6, 138.5, 139.4, 143.6, 150.0, 157.3, 158.6, 182.5, 189.9.
Chalcone isatin 9f. Yield 85%, orange-yellow solid. Mp: 128–130 °C. 1H NMR (300 MHz, CDCl3): δ 3.86, (s, 3H); 4.23 (t, J = 6.0 Hz, 2H); 4.69 (t, J = 6.0 Hz, 2H); 5.20 (s, 2H); 6.56 (d, J = 8.1 Hz, 1H); 6.97–7.04 (m, 5H); 7.29–7.33 (m, 1H); 7.36–7.43 (m, 2H); 7.45–7.48 (m, 1H); 7.53 (d, J = 7.5 Hz, 1H); 7.56–7.59 (m, 2H); 7.63 (s, 1H). 7.89 (d, J = 15.9 Hz, 1H); 13C NMR (75 MHz, CDCl3): δ 40.6, 47.8, 55.8, 62.7, 109.4, 111.9, 113.1, 117.5, 120.7, 121.6, 123.7, 124.1, 124.8, 125.5, 127.7, 128.4, 129.7, 130.3, 131.5, 132.6, 138.1, 138.5, 149.9, 157.2, 158.5, 186.4, 193.1.
Chalcone isatin 9g. Yield 80%. Orange-yellow solid. Mp: 180–182 °C. 1H NMR (300, MHz, CDCl3): δ 4.26 (t, J = 4.7 Hz, 2H); 4.75 (t, J = 6.0 Hz, 2H); 5.22 (s, 2H); 6.60 (d, J = 8.1 Hz, 1H); 7.00–7.06 (m, 3H); 7.36–7.43 (m, 2H); 7.51 (d, J = 7.2 Hz, 1H); 7.60–7.63 (m, 2H); 7.78 (s, 1H); 8.03 (d, J = 15.6 Hz, 1H); 8.11–8.14 (m, 2H); 8.31 (d, J = 8.7 Hz, 2H). 13C NMR (75 MHz, CDCl3): δ 40.5, 47.7, 62.0, 109.5, 112.7, 117.9, 121.6, 122.4, 124.1, 124.2, 123.7, 125.6, 129.6, 130.1, 132.4, 138.6, 142.1, 143.9, 149.9, 150.1, 157.6, 158.5, 182.2, 189.4.
Chalcone isatin 9h. Yield 84%. Orange-yellow solid. Mp: 82–84 °C. 1H NMR (300 MHz, CDCl3): δ 4.21 (t, J = 6.0 Hz, 2H); 4.72 (t, J = 6.0 Hz, 2H); 5.18 (s, 2H); 6.49 (d, J = 7.8 Hz, 1H); 6.94–7.04 (m, 3H); 7.31–7.37 (m, 2H); 7.40–7.48 (m, 4H); 7.52–7.56 (m, 1H); 7.61–7.63 (m, 1H); 7.86 (s, 1H); 7.93–7.95 (m, 2H); 8.02 (d, J = 15.9 Hz, 1H). 13C NMR (75 MHz, CDCl3): δ 40.6, 47.92, 62.3, 109.4, 112.8, 117.4, 121.5, 122.7, 124.1, 124.2, 124.3, 125.5, 128.1, 128.5, 128.6, 129.1, 131.8, 132.7, 138.3, 138.5, 139.8, 144.1, 150.0, 157.3, 158.6, 182.4, 190.7.
Chalcone isatin 9i. Yield 80%, yellow solid. Mp: 110–112 °C. 1H NMR (300 MHz, CDCl3): δ 4.24 (t, J = 6.0 Hz, 2H); 4.71 (t, J = 6.0 Hz, 2H); 5.23 (s, 2H); 6.55 (d, J = 8.1 Hz, 1H); 6.98–7.06 (m, 2H); 7.14–7.17 (m, 1H); 7.34–7.49 (m, 3H); 7.49–7.53 (m, 2H); 7.60–7.65 (m, 2H); 7.72 (s, 1H); 7.82 (d, J = 3.6 Hz, 1H); 8.05 (J = 15.6 Hz, 1H). 13C NMR (75 MHz, CDCl3): δ 31.6, 40.6, 47.9, 60.4, 62.3, 69.7, 69.9, 70.1, 72.7, 80.7, 109.5, 112.8, 117.4, 121.5, 124.0, 124.1, 124.3, 124.5, 125.6, 128.8, 131.3, 135.8, 138.6, 144.2, 150.0, 157.2, 158.6, 182.4, 193.5.
Synthesis of 1,2,3-triazole bridged spiropyrrolidine-oxindole grafted macrocycles 12a–i/bis-spiropyrrolizidne-oxindole grafted macrocycles 16a–i
(ii) General procedure. A solution of the chalcone isatins 9a–i (1 mmol) and sarcosine (10)/proline (14) (2.5 mmol) was refluxed in dry toluene under N2 atmosphere for 12 h at 120 °C using Dean–Stark apparatus. After the completion of reaction as indicated by TLC, toluene was evaporated under reduced pressure. The crude product was washed with water and extracted with dichloromethane (4 × 20 mL). The combined organic layer was dried (MgSO4) and filtered, concentrated in vacuum. The crude product was purified by column chromatography using hexane/ethyl acetate (1
:
1) as eluent to give the spiropyrrolidine-oxindole grafted macrocycles 12a–i and bis-spiropyrrolizidine-oxindole grafted macrocylcles 16a–i.
Spiropyrrolidine-oxindole grafted macrocycle 12a. Yield 74%. White solid. Mp 206–208 °C. 1H NMR (300 MHz, CDCl3): δ 2.14 (s, 3H); 3.38 (t, J = 8.1 Hz, 1H); 3.56–3.60 (m, 1H); 3.92 (t, J = 9.1 Hz, 1H); 4.44–4.52 (m, 1H); 4.56–4.72 (m, 2H); 4.81–4.88 (m, 1H); 5.09 (d, J = 10.2 Hz, 1H); 5.21 (d, J = 6.9 Hz, 1H); 5.37 (d, J = 10.5 Hz, 1H); 6.58 (d, J = 7.8 Hz, 1H); 6.77 (t, J = 7.2 Hz, 1H); 6.86–6.93 (m, 2H); 6.99 (t, J = 8.7 Hz, 1H); 7.03–707 (m, 3H); 7.20–7.27 (m, 2H); 7.39 (d, J = 8.4 Hz, 2H); 8.12 (s, 1H). 13C NMR (70 MHz, CDCl3): δ 34.5, 39.5, 44.8, 48.7, 52.1, 60.1, 62.3, 73.8, 107.0, 112.6, 121.1, 122.8, 125.5, 126.2, 126.6, 126.8, 127.5, 128.3, 129.2, 129.4, 131.3, 133.0, 136.6, 142.9, 144.3, 156.9, 178.2, 199.3. DEPT135: 34.5, 39.5, 44.8, 48.7, 52.1, 60.1, 62.3, 107.0, 112.6, 121.1, 122.8, 126.6, 126.8, 128.3, 129.3, 129.4, 131.3, 133.0. HRMS (TOF MS ES+ 3.35 × 107) m/z calcd for C30H26BrN5O5 + H+ [M + H]+ 584.1297 found 584.1271.
Spiropyrrolidine-oxindole grafted macrocycle 12b. Yield: 75%; white solid; mp; 146–148 °C. 1H NMR (300 MHz, CDCl3): δ 2.14 (S, 3H); 3.38 (t, J = 7.8 Hz, 1H); 3.57–3.61 (m, 1H); 3.92 (t, J = 7.5 Hz, 1H); 4.45–4.53 (m, 1H); 4.57–4.72 (m, 2H); 4.81–4.91 (m, 1H); 5.10, (d, J = 10.5 Hz, 1H); 5.22 (d, J = 6.6 Hz, 1H); 5.38 (d, J = 10.5 Hz, 1H); 6.58 (d, J = 7.8 Hz, 1H); 6.77 (t, J = 7.5 Hz, 1H); 6.87–6.93 (m, 2H); 6.96–7.04 (m, 2H); 7.13 (d, J = 8.4 Hz, 2H); 7.21–7.26 (m, 4H); 8.13 (s, 1H). 13C NMR (75 MHz,CDCl3): δ 34.5, 39.5, 44.8, 48.7, 52.1, 60.1, 62.3, 73.8, 107.0, 112.6, 121.1, 122.8, 125.5, 126.2, 126.6, 126.8, 128.3, 129.2, 129.3, 133.0, 136.2, 138.7, 142.9, 144.3, 156.9, 178.2, 199.1 DEPT135: 34.5, 39.5, 44.8, 48.7, 52.1, 60.1, 62.3, 107.0, 112.6, 121.1, 122.8, 126.6, 126.8, 128.3, 129.2, 129.3, 133.0 ppm. HRMS (TOF MS ES+ 3.36 × 107) m/z calcd for C30H26ClN5O5 + H2 [M + 2]+ 541.1881 found 541.1859.
Spiropyrrolidine-oxindole grafted macrocycle 12c. 68%; white solid; mp; 218–220 °C. 1H NMR (300 MHz, CDCl3): δ 2.15 (s, 3H); 3.36 (t, J = 8.1 Hz, 1H); 3.55–3.59 (m, 1H); 3.78 (s, 3H); 3.87 (s, 3H); 3.90–3.96 (m, 1H); 4.41–4.52 (m, 3H); 4.88–4.92 (m, 1H); 5.12 (d, J = 10.5 Hz, 1H); 5.31 (d, J = 6.9 Hz, 1H); 5.43 (d, J = 10.5 Hz, 1H); 6.57 (d, J = 8.1 Hz, 1H); 6.67–6.76 (m, 2H); 6.82–6.91 (m, 2H); 6.96–6.98 (m, 2H); 7.01–7.07 (m, 2H); 7.21–7.27 (m, 2H); 8.08 (s, 1H). 13C NMR (75 MHz, CDCl3): δ 34.6, 39.9, 45.5, 49.0, 52.6, 55.9, 56.0, 60.0, 62.3, 73.9, 106.7, 109.3, 110.3, 112.5, 121.1, 122.2, 122.6, 125.9, 126.3, 126.5, 127.2, 128.3, 129.0, 131.9, 133.1, 142.9, 148.6, 152.7, 157.0, 178.3, 199.4. DEPT135: δ 34.6, 39.9, 45.5, 49.0, 52.6, 55.9, 56.0, 60.0, 62.3, 106.7, 109.3, 110.3, 112.5, 121.1, 122.2, 122.6, 126.5, 127.2, 128.3, 129.1, 133.1. HRMS (TOF MS ES+ 9.65 × 108) m/z calcd for C32H31N5O5 + H+ [M + H]+ 566.2403 found 566.2404.
Spiropyrrolidine-oxindole grafted macrocycle 12d. Yield: 70%; white solid; mp; 232–234 °C. 1H NMR (300 MHz, CDCl3): δ 2.14 (s, 3H); 3.36 (t, J = 8.1 Hz, 1H); 3.58 (t, J = 6.3 Hz, 1H); 3.77 (s, 3H); 3.89–3.96 (m, 1H); 4.43–4.41 (m, 1H); 4.52–4.61 (m, 2H); 4.87–4.92 (m, 1H); 5.12 (d, J = 10.2 Hz, 1H); 5.27 (d, J = 6.9 Hz, 1H); 5.41 (d, J = 10.2 Hz, 1H); 6.57 (d, J = 7.8 Hz, 1H); 6.72 (d, J = 8.7 Hz, 2H); 6.81 (t, J = 8.1 Hz, 1H); 6.86–6.91 (m, 1H); 6.97–7.04 (m, 3H); 7.21–7.27 (m, 4H); 8.09 (s, 1H). 13C NMR (75 MHz, CDCl3): δ 34.6, 39.8, 45.3, 48.9, 52.2, 55.4, 60.0, 62.3, 73.9, 106.7, 112.5, 113.7, 121.1, 122.7, 125.8, 126.4, 126.5, 127.1, 128.2, 129.0, 130.1, 131.4, 133.1, 142.9, 144.4, 157.1, 157.0, 162.8, 178.3, 198.7. DEPT135: δ 34.6, 39.8, 45.3, 49.0, 52.2, 55.4, 60.0, 62.3, 106.7, 112.5, 113.7, 121.1, 126.5, 127.1, 128.3, 129.1, 130.1, 133.1. HRMS (TOF MS ES+ 2.50 × 108) m/z calcd for C31H29N5O4 + H+ [M + H]+ 536.2298 found 536.2377.
Spiropyrrolidine-oxindole grafted macrocycle 12e. Yield: 68%. White solid; mp 184–186 °C. 1H NMR (300 MHz, CDCl3): δ 2.14 (s, 3H); 2.26 (s, 3H); 3.34 (t, J = 7.8 Hz, 1H); 3.51–3.61 (m, 1H); 3.90–3.96 (m, 1H); 4.41–4.52 (m, 3H); 4.91 (d, J = 10.2 Hz, 1H); 5.09 (d, J = 10.5 Hz, 1H); 5.34 (d, J = 6.9 Hz, 1H); 5.48 (d, J = 10.5 Hz, 1H); 6.56 (d, J = 8.1 Hz, 1H); 6.81–6.90 (m, 2H); 6.98–7.12 (m, 5H); 7.10 (d, J = 8.1 Hz, 2H); 7.22–7.26 (m, 3H); 7.99 (s, 1H). 13C NMR (75 MHz, CDCl3): 21.5, 34.55, 40.1, 45.7, 49.2, 52.8, 59.9, 62.4, 73.8, 106.7, 112.5, 121.1, 122.7, 125.9, 126.2, 126.4, 127.3, 127.7, 128.3, 128.7, 129.0, 133.1, 136.1, 142.9, 143.0, 144.5, 157.1, 178.2, 210.4. DEPT135: δ 21.5, 34.6, 40.1, 45.7, 49.2, 52.8, 59.9, 62.4, 106.7, 112.5, 121.1, 122.3, 126.4, 127.3, 128.4, 128.7, 129.0, 133.2. HRMS (TOF MS ES+ 9.22 × 108) m/z calcd for C31H29N5O3 + H2 [M + 2]+ 521.2427 found 521.2437.
Spiropyrrolidine-oxindole grafted macrocycle 12f. Yield: 65%; white solid; mp; 196–198 °C. 1H NMR (300 MHz, CDCl3): δ 2.11 (s, 3H); 3.14 (s, 3H); 3.38–3.52 (m, 1H); 3.52–3.61 (m, 1H); 3.75–3.88 (m, 1H); 4.01–4.11 (m, 1H); 4.20–4.29 (m, 1H); 4.76–4.80 (m, 1H); 4.89 (d, J = 10.5, Hz, 1H); 5.45–5.53 (m, 3H); 6.57–6.63 (m, 2H); 6.69 (d, J = 8.4 Hz, 1H); 6.78 (s, 1H); 6.89–6.94 (m, 1H); 7.02 (d, J = 7.8 Hz, 1H); 7.14–7.19 (m, 2H); 7.21–7.24 (m, 3H); 7.24–7.28 (m, 1H); 7.31–7.33 (m, 2H). 13C NMR (75 MHz, CDCl3): δ 34.3, 40.8, 47.8, 50.0, 56.4, 58.9, 59.3, 63.3, 73.3, 106.4, 112.0, 112.7, 121.1, 121.2, 123.1, 126.0, 126.4, 126.5, 126.8, 127.1, 128.3, 128.4, 129.1, 131.2, 133.2, 134.1, 143.8, 144.7, 156.2, 158.4, 178.0, 205.2. DEPT135: δ 34.3, 40.8, 47.7, 50.0, 56.4, 58.9, 59.3, 63.3, 106.4, 112.0, 112.7, 121.1, 121.2, 123.1, 126.0, 126.3, 128.3, 128.4, 129.1, 131.2, 133.2.
Spiropyrrolidine-oxindole grafted macrocycle 12g. Yield: 72%; white solid; mp; 228–230 °C. 1H NMR (300 MHz, CDCl3): δ 2.14 (s, 3H); 3.41 (t, J = 8.1 Hz, 1H); 3.58–3.62 (m, 1H); 3.88–3.95 (m, 1H); 4.52–4.55 (m, 1H); 4.75–4.83 (m, 3H); 5.12 (d, J = 10.5 Hz, 1H); 5.19 (d, J = 6.6 Hz, 1H); 5.35 (d, J = 10.5 Hz, 1H); 6.57 (d, J = 7.8 Hz, 1H); 6.74 (t, J = 7.5 Hz, 1H); 6.85–6.87 (m, 1H); 6.91–6.97 (m, 3H); 7.01–7.26 (m, 2H); 7.35 (d, J = 8.4 Hz, 2H); 8.10 (d, J = 8.4 Hz, 2H); 8.22 (s, 1H). 13C NMR (75 MHz, CDCl3): δ 34.4, 39.2, 44.3, 48.3, 52.1, 60.2, 62.3, 73.8, 107.2, 112.7, 121.2, 122.9, 123.2, 125.2, 125.9, 126.7, 126.8, 128.4, 129.2, 129.5, 133.0, 142.9, 144.3, 149.6, 157.0, 178.1, 198.7 ppm. DEPT135: δ 34.4, 39.2, 44.3, 48.3, 52.1, 60.2, 62.3, 107.2, 112.7, 121.14, 122.8, 123.2.126.7, 126.8, 128.4, 129.2, 129.5, 133.0. HRMS (TOF MS ES+ 4.14 × 107) m/z calcd for C30H26N6O5 + H2 [M + 2]+ 552.2122 found 552.2100.
Spiropyrrolidine-oxindole grafted macrocycle 12h. Yield: 73%; white solid; mp; 216–218 °C. 1H NMR (300 MHz, CDCl3): δ 2.14 (s, 3H); 3.34 (t, J = 8.1 Hz, 1H); 3.52–3.56 (m, 1H); 3.90–3.96 (m, 1H); 4.39–4.50 (m, 3H); 4.87–4.91 (m, 1H); 5.09, (d, J = 10.5 Hz, 1H); 5.38 (d; J = 7.2 Hz, 1H); 5.43 (d, J = 10.5 Hz, 1H); 6.54 (d, J = 7.8 Hz, 1H); 6.82–6.91 (m, 2H); 6.98–7.05 (m, 3H); 7.15 (d, J = 7.2 Hz, 2H); 7.20–7.26 (m, 4H); 7.29–7.34 (m, 1H); 7.94 (s, 1H). 13C NMR (70 MHz, CDCl3): δ 34.5, 40.1, 45.6, 49.2, 53.2, 59.9, 62.4, 73.8, 106.7, 112.6, 121.6, 122.8, 125.9, 126.2, 126.4, 127.2, 127.5, 128.0, 128.4, 129.1, 132.1, 133.1, 138.9, 143.0, 144.5, 157.1, 178.2, 200.9. DEPT135: δ 34.5, 40.1, 45.6, 49.2, 53.2, 59.8, 62.4, 106.7, 112.5, 121.1, 122.8, 126.4, 127.2, 127.4, 128.0, 128.4, 129.1, 132.2, 133.2. HRMS (TOF MS ES+ 3.97 × 107) m/z calcd for C30H27N5O3 + H2 [M + 2]+ 507.2270 found 507.2260.
Spiropyrrolidine-oxindole grafted macrocycle 12i. Yield: 70%; white solid; mp; 238–240 °C. 1H NMR (300 MHz, CDCl3): δ 2.14 (s, 3H); 3.33 (t, J = 8.1 Hz, 1H); 3.58–3.71 (m, 1H); 3.92–3.98 (m, 1H); 4.45–4.51 (m, 1H); 4.56–4.62 (m, 1H); 4.85–4.95 (m, 1H); 4.99 (d, J = 6.6 Hz, 1H); 5.11 (d, J = 10.5 Hz, 1H); 5.40 (d, J = 10.5 Hz, 1H); 6.61 (d, J = 7.5 Hz, 1H); 6.81 (t, J = 7.5 Hz, 1H); 6.86–6.89 (m, 1H); 6.92–6.98 (m, 1H); 7.00–7.21 (m, 2H); 7.21–7.26 (m, 4H); 7.40 (d, J = 4.8 Hz, 1H) 8.23 (s, 1H). 13C NMR (75 MHz,CDCl3): δ 34.6, 40.1, 44.9, 49.0, 54.7, 62.2, 73.9, 106.8, 112.5, 121.1, 122.8, 126.8, 127.1, 127.9, 128.4, 129.4, 132.3, 133.0, 133.1, 142.6, 144.8, 157.0, 178.2, 199.3. DEPT 135: δ 34.6, 44.9, 49.0, 54.7, 59.7, 62.2, 106.8, 112.5, 121.1, 121.5, 122.8, 126.8, 127.1, 127.9, 128.4, 129.2, 132.3, 133.1, 133.3.
Bis-spiropyrrolizidine-oxindole grafted macrocycle 16a. Yield: 64%; white solid; mp; 220–222 °C. 1H NMR (300 MHz, CDCl3): δ 1.63–1.77 (m, 4H); 1.79–1.94 (m, 4H); 2.43–2.65 (m, 4H); 3.77–3.83 (m, 2H); 3.86–3.97 (m, 4H); 4.38–4.40 (m, 2H); 4.41–4.53 (m, 2H); 4.59–4.67 (m, 2H); 5.24, 5.49 (ABq, J = 11.7 Hz, 4H); 5.60 (d; J = 11.7 Hz, 2H); 6.79 (d, J = 7.5 Hz, 2H); 6.95–7.02 (m, 8H); 7.14 (t, J = 7.5 Hz, 2H); 7.23–7.25 (m, 8H); 7.32–7.35 (m, 2H); 7.47 (d, J = 6.9 Hz, 2H); 9.85 (s, 2H). 13C NMR (75 MHz, CDCl3): δ 28.4, 32.6, 40.0, 46.6, 47.4, 53.3, 61.6, 64.3, 67.8, 74.1, 108.6, 112.5, 121.7, 122.8, 124.9, 125.4, 126.3, 127.3, 127.6, 128.8, 129.3, 129.9, 131.1, 133.5, 136.4, 141.9, 145.2, 156.8, 179.2, 196.8. DEPT135: δ 28.4, 32.6, 40.0, 46.6, 47.4, 53.3, 61.6, 64.2, 67.8, 108.7, 112.5, 121.7, 122.9, 125.4, 127.3, 128.8, 129.3, 129.9, 131.1, 133.5. HRMS (TOF MS ES+ 2.29 × 108) m/z calcd for C64H56Br2N10O6 + H+ [M + H]+ 1219.2829 found 1219.2828.
Bis-spiropyrrolizidine-oxindole grafted macrocycle 16b. Yield: 65%; white solid; mp; 236–238 °C. 1H NMR (300 MHz, CDCl3): δ 1.68–1.78 (m, 4H); 1.90–2.0 (m, 4H); 2.43–2.5 (m, 2H); 2.67–2.69 (m, 2H); 3.79–3.85 (m, 2H); 3.87–3.97 (m, 4H); 4.35–4.44 (m, 2H); 4.47–4.58 (m, 2H); 4.62–4.67 (m, 2H); 5.24, 5.49 (AB q, J = 11.7 Hz, 4H); 5.60 (d, J = 11.7 Hz, 2H); 6.58 (d, J = 7.8 Hz, 2H); 6.94–7.01 (m, 4H); 7.05–7.09 (m, 8H); 7.14 (t, J = 7.8 Hz, 2H); 7.26–7.30 (m, 4H); 7.31–7.35 (m, 2H); 7.47 (d, J = 7.5 Hz, 2H); 9.85 (s, 2H). 13C NMR (75 MHz, CDCl3): δ 28.4, 31.6, 32.6, 40.0, 46.6, 47.4, 53.3, 61.7, 64.3, 67.8, 74.1, 108.6, 112.5, 121.7, 122.8, 125.0, 125.4, 126.3, 127.3, 128.1, 128.8, 129.2, 129.9, 133.5, 135.9, 138.9, 141.9, 145.2, 156.8, 179.3, 196.6. DEPT135: δ 28.4, 31.6, 32.6, 40.0, 46.6, 47.4, 53.3, 61.7, 64.3, 67.8, 108.6, 112.5, 121.7, 122.9, 125.4, 127.3, 128.2, 128.8, 129.2, 129.9, 133.5. HRMS (TOF MS ES+ 4.22 × 108) m/z calcd for C64H56Cl2N10O6 + H+ [M + H]+ 1131.3838 found 1131.3840.
Bis-spiropyrrolizidine-oxindole grafted macrocycle 16c. Yield: 58%; white solid; mp; 270–272 °C. 1H NMR (300 MHz, CDCl3): δ 1.61–1.75 (m, 4H); 1.79–1.90 (m, 4H); 2.50 (t, J = 6.3 Hz, 4H); 3.54 (s, 6H); 3.74 (s, 6H); 3.79–3.83 (m, 4H); 4.17–4.27 (m, 2H); 4.33–4.44 (m, 4H); 4.46–4.52 (m, 2H); 5.21, 5.35 (AB q, J = 12 Hz, 4H); 5.67 (d, J = 11.7 Hz, 2H); 6.58 (d, J = 7.2 Hz, 2H); 6.71–6.72 (m, 2H); 6.80 (d, J = 7.8 Hz, 2H); 6.86–6.89 (m, 2H); 6.93 (d, J = 7.5 Hz, 4H); 7.09 (t, J = 7.5 Hz, 2H); 7.19–7.24 (m, 4H); 7.33 (d, J = 7.2 Hz, 2H); 7.40–7.43 (m, 2H); 9.65 (s, 2H). 13C NMR (75 MHz, CDCl3): δ 27.6, 31.6, 39.2, 45.6, 48.1, 54.5, 55.5, 56.1, 60.1, 63.8, 67.5, 74.2, 108.3, 109.8, 110.4, 111.8, 121.7, 121.9, 122.8, 124.7, 125.3, 126.0, 127.6, 128.7, 129.7, 130.5, 133.8, 141.5, 144.8, 148.4, 153.1, 156.7, 179.4, 195.7. DEPT135: 27.6, 31.6, 39.2, 45.6, 48.2, 54.4, 55.5, 56.1, 60.2, 63.8, 67.5, 108.3, 109.7, 110.4, 111.8, 121.7, 121.9, 122.8, 124.7, 127.6, 128.7, 129.7, 133.8. HRMS (TOF MS ES+ 5.86 × 107) m/z calcd for C68H66N10O10 + H+ [M + H]+ 1183.5041 found 1183.5045.
Bis-spiropyrrolizidine-oxindole grafted macrocycle 16d. Yield: 60%. White solid; mp 250–252 °C. 1H NMR (300 MHz, CDCl3): δ 1.73–1.83 (m, 4H); 1.86–1.98 (m, 4H); 2.59 (t, J = 6.3 Hz, 4H); 3.73 (s, 6H); 3.74–3.76 (m, 2H); 3.86 (t, J = 8.1 Hz, 2H); 4.39–4.48 (m, 4H); 4.54–4.60 (m, 4H); 5.33, 5.42 (ABq J = 11.7 Hz, 4H); 5.75 (d, J = 12 Hz, 2H); 6.67 (d, J = 8.7 Hz, 4H); 6.89 (d, J = 7.5 Hz, 2H); 6.98–7.02 (m, 4H); 7.17–7.23 (m, 6H); 7.28–7.35 (m, 4H); 7.41 (d, J = 7.5 Hz, 2H); 7.49–7.52 (m, 2H); 9.73 (s, 2H). 13C NMR (75 MHz, CDCl3): 27.7, 29.7, 31.6, 39.4, 45.6, 48.2, 54.3, 55.4, 60.4, 63.7, 67.6, 74.3, 108.4, 111.7, 113.1, 121.7, 122.8, 124.6, 125.3, 126.0, 127.5, 128.6, 129.8, 130.1, 130.4, 133.8, 141.4, 145.0, 156.6, 163.4, 179.2, 195.7. DEPT135: δ 27.7, 29.7, 31.6, 39.4, 45.6, 48.2, 54.3, 55.4, 60.4, 63.7, 67.6, 108.4, 111.7, 113.1, 121.7, 122.8, 124.6, 127.5, 128.6, 129.8, 130.1, 133.8. HRMS (TOF MS ES+ 1.24 × 109) m/z calcd for C66H62N10O8 + H+ [M + H]+ 1123.4830 found 1123.4847.
Bis-spiropyrrolizidine-oxindole grafted macrocycle 16e. Yield 56%. White Solid. Mp 238–240 °C. 1H NMR (300 MHz, CDCl3): δ 1.69–1.75 (m, 4H); 1.92–1.94 (m, 4H); 2.22 (s, 6H); 2.49 (t, J = 3 Hz, 2H); 2.62 (t, J = 2.4 Hz, 2H); 3.72–3.80 (m, 2H); 3.90–3.97 (m, 4H); 4.26–4.33 (m, 2H); 4.52–4.57 (m, 4H); 5.25, 5.49 (ABq, J = 11.7 Hz, 4H); 5.67 (d, J = 11.4 Hz, 2H); 6.76 (d, J = 7.8 Hz, 2H); 6.90–6.97 (m, 4H); 6.99–7.02 (m, 4H); 7.01–7.10 (m, 4H); 7.13–7.16 (m, 2H); 7.25–7.30 (m, 4H); 7.34–7.36 (m, 2H); 7.49 (d, J = 7.2 Hz, 2H); 9.87 (s, 2H). 13C NMR (70 MHz, CDCl3): δ 21.5, 28.1, 32.4, 40.2, 46.4, 47.6, 53.6, 61.3, 64.1, 67.7, 74.1, 108.5, 112.3, 121.7, 122.7, 125.2, 125.3, 126.5, 127.3, 127.8, 128.6, 128.7, 129.7, 133.7, 135.2, 141.9, 143.4, 152.3, 156.8, 179.4, 197.3. DEPT135: 21.5, 28.1, 32.4, 40.2, 46.4, 47.6, 53.6, 61.3, 64.1, 67.7, 108.5, 112.3, 121.7, 122.7, 125.3, 127.3, 127.8, 128.5, 128.6, 128.7, 129.7, 133.7. HRMS (TOF MS ES+ 1.21 × 109) m/z calcd for C66H62N10O6 + H+ [M + H]+ 1091.4932 found 1091.4944.
Bis-spiropyrrolizidine-oxindole grafted macrocycle 16f. Yield: 55%; white solid; mp; 228–230 °C. 1H NMR (300 MHz, CDCl3): δ 1.58–1.75 (m, 4H); 1.71–1.94 (m, 4H); 2.32–2.40 (m, 2H); 2.50–2.55 (m, 2H); 3.33 (s, 6H); 3.44–3.53 (m, 2H); 3.78–3.89 (m, 4H); 4.21–4.28 (m, 2H); 4.41–4.51 (m, 4H); 5.27, 5.47 (ABq, J = 12.0 Hz, 4H); 5.93 (d, J = 11.7 Hz, 2H); 6.14 (d, J = 6.6 Hz, 2H); 6.52–6.57 (m, 4H); 6.84 (d, J = 7.8 Hz, 2H); 6.96 (t, J = 7.2 Hz, 2H); 7.02 (d, J = 8.4 Hz, 2H); 7.12–7.16 (m, 4H); 7.28 (d, J = 9.3 Hz, 2H); 7.35 (d, J = 7.8 Hz, 4H); 7.49 (d, J = 7.2 Hz, 2H); 9.81 (s, 2H). 13C NMR (75 MHz, CDCl3): δ 28.1, 32.5, 40.1, 46.7, 47.2, 53.0, 55.3, 64.0, 64.2, 67.8, 73.6, 108.5, 110.8, 112.0, 119.8, 121.5, 122.6, 125.7, 127.0, 127.2, 128.5, 128.9, 129.1, 129.7, 132.2, 133.7, 142.7, 145.1, 156.8, 157.5, 178.8, 199.6 ppm. DEPT135: δ 28.1, 32.4, 40.1, 46.7, 47.2, 53.0, 55.3, 64.0, 64.2, 67.8, 108.4, 110.8, 112.0, 119.8, 121.5, 122.6, 125.7, 127.2, 128.5, 128.9, 129.7, 132.2, 133.7.
Bis-spiropyrrolizidine-oxindole grafted macrocycle 16g. Yield: 62%; white solid; mp; 196–198 °C. 1H NMR (300 MHz, CDCl3): δ 1.69–1.81 (m, 4H); 1.94–2.01 (m, 4H); 2.45–2.53 (m, 2H); 2.65–2.72 (m, 2H); 3.64–3.77 (m, 4H); 3.92–3.97 (m, 2H); 4.39–4.55 (m, 4H); 4.61–4.71 (m, 2H); 5.22, 5.50 (ABq, J = 12 Hz, 4H); 5.63 (d, J = 11.7 Hz, 2H); 6.76 (d, J = 7.8 Hz, 2H); 6.95–7.02 (m, 4H); 7.16 (t, J = 7.5 Hz, 2H); 7.25–7.31 (m, 8H); 7.33–7.35 (m, 2H); 7.46–7.49 (m, 2H); 7.94 (d, J = 8.7 Hz, 4H); 9.81 (s, 2H). 13C NMR (75 MHz, CDCl3): δ 28.4, 29.7, 32.8, 39.8, 46.7, 47.3, 53.2, 62.2, 64.4, 67.8, 73.9, 108.7, 112.6, 121.8, 122.9, 123.1, 124.8, 125.5, 126.0, 127.3, 128.8, 129.0, 130.2, 133.5, 141.8, 142.2, 145.1, 149.8, 156.8, 179.1, 196.5. DEPT135: δ 28.4, 29.7, 32.8, 39.8, 46.7, 47.3, 53.2, 62.3, 64.4, 67.8, 108.7, 112.6, 121.8, 122.9, 123.1, 125.5, 127.3, 128.8, 129.1, 130.2, 133.5. HRMS (TOF MS ES+ 9.19 × 108) m/z calcd for C64H56N12O10 + H+ [M + H ]+ 1153.4320 found 1153.4332.
Bis-spiropyrrolizidine-oxindole grafted macrocycle 16h. 60%; white solid; mp; 208–210 °C. 1H NMR (300 MHz, CDCl3): δ 1.79–1.76 (m, 8H); 2.59 (t, J = 6.0 Hz, 4H); 3.47–3.54 (m, 2H); 3.79–3.86 (m, 2H); 4.37–4.44 (m, 4H); 4.50–4.59 (m, 4H); 5.29, 5.43 (AB q, J = 11.7 Hz, 4H); 5.80 (d, J = 11.7 Hz, 2H); 6.86 (d, J = 7.8 Hz, 2H); 6.9–7.03 (m, 4H); 7.10–7.14 (m, 8H); 7.16–7.20 (m, 2H); 7.29–7.34 (m, 4H); 7.37–7.40 (m, 4H); 7.53 (d, J = 6 Hz, 2H); 9.69 (s, 2H). 13C NMR (75 MHz, CDCl3): δ 27.6, 31.5, 39.4, 45.6, 48.2, 54.1, 60.9, 63.7, 67.7, 74.1, 108.5, 111.8, 121.7, 122.9, 124.7, 125.1, 125.9, 127.3, 127.7, 127.8, 127.9, 128.7, 129.9, 132.6, 133.8, 137.7, 141.5, 144.9, 156.6, 178.9, 197.9. DEPT135: δ 27.6, 31.5, 39.4, 45.6, 48.2, 54.1, 60.9, 63.7, 67.7, 108.5, 111.8, 121.7, 122.9, 124.6, 127.3, 127.7, 127.8, 127.9, 128.7, 129.9, 132.6, 133.8. HRMS (TOF MS ES+ 4.89 × 107) m/z calcd for C29H27N5O5 + H+ [M + H ]+ 1063.4619 found 1063.4640.
Bis-spiropyrrolizidine-oxindole grafted macrocycle 16i. Yield: 56%; white solid; mp; 202–204 °C. 1H NMR (300 MHz, CDCl3): δ 1.67–1.83 (m, 4H); 1.81–2.04 (m, 4H); 2.61 (t, J = 6 Hz, 4H); 3.84–3.93 (m, 4H); 4.49–4.65 (m, 8H); 5.27, 5.39 (AB q, J = 11.7 Hz, 4H); 5.62 (d, J = 12 Hz, 2H); 6.84 (t, J = 7.8 Hz, 2H); 6.97 (t, J = 7.5 Hz, 6H); 7.18 (t, J = 7.5 Hz, 2H); 7.25–7.36 (m, 8H); 7.40–7.45 (m, 4H); 9.70 (s, 2H). 13C NMR (75 MHz, CDCl3): δ 27.8, 31.7, 39.8, 45.8, 48.1, 54.3, 61.9, 63.9, 67.7, 74.8, 108.6, 111.9, 121.8, 123.1, 124.7, 125.3, 125.8, 127.0, 127.7, 128.8, 130.0, 131.5, 133.9, 134.2, 141.5, 144.6, 145.1, 156.8, 179.5, 188.7. DEPT135: δ 27.8, 31.7, 39.8, 45.8, 48.1, 54.3, 61.9, 63.9, 67.7, 108.6, 111.9, 121.8, 123.1, 124.7, 127.0, 127.8, 128.8, 130.0, 131.5, 133.8, 134.2.
Conflicts of interest
The authors have no conflicts of interest.
Acknowledgements
The authors thank DST-FIST for providing NMR and HRMS facility to the Department of Organic Chemistry, University of Madras, Chennai, India, and the authors also thank SAIF, IIT Madras for single crystal XRD analysis. PP thanks Council of Scientific and Industrial Research (CSIR) New Delhi, India for SRF (grant number: 09/115(0775)/2014 EMR-I).
References
-
(a) A. K. Yudin, Chem. Sci., 2015, 6, 30–49 RSC;
(b) Practical Medicinal Chemistry with Macrocycles: Design, Synthesis, and Studies, ed. E. Marsault and M. L. Peterson, Wiley & Sons, Hoboken, 2017 Search PubMed;
(c) F. Davis and S. Higson, Macrocycles: Construction, Chemistry and Nanotechnology Applications, Wiley & Sons, Chichester, U.K., 2011 CrossRef;
(d) E. M. Driggers, S. P. Hale, J. Lee and N. K. Terrett, Nat. Rev. Drug Discovery, 2008, 7, 608–624 CrossRef PubMed;
(e) J. Mallinson and I. Collins, Future Med. Chem., 2012, 4, 1409–1438 CrossRef PubMed;
(f) D. Krahn, C. Ottmann and M. Kaiser, Curr. Med. Chem., 2011, 18, 5052–5060 CrossRef PubMed.
-
(a) V. Balzani, A. Credi, F. M. Raymo and J. F. Stoddart, Angew. Chem., Int. Ed., 2000, 39, 3349–3391 CrossRef;
(b) C. P. Collier, G. Mattersteig, E. W. Wong, Y. Luo, K. Beverley, J. Sampaio, F. M. Raymo, J. F. Stoddart and J. R. Heath, Science, 2000, 289, 1172–1175 CrossRef PubMed;
(c) X. –J. Wanga, J. Liu, C.-X. Zhang, D.-L. Gong, H. Zhang, J.-D. Wang, Y.-J. Yan and W.-S. Xiang, Bioorg. Med. Chem. Lett., 2011, 21, 5145–5148 CrossRef PubMed;
(d) T. Berg, Angew. Chem., Int. Ed., 2003, 42, 2462–2481 CrossRef PubMed;
(e) S. Purushothaman, R. Prasanna and R. Raghunathan, Tetrahedron Lett., 2013, 54, 6450–6454 CrossRef.
-
(a) S. Purushothaman, R. Prasanna, P. Niranjana, R. Raghunathan, S. Nagaraj and R. Rengasamy, Bioorg. Med. Chem. Lett., 2010, 20, 7288–7291 CrossRef PubMed;
(b) J. R. Liddell, Nat. Prod. Rep., 1996, 13, 187 RSC;
(c) J. P. Michael, Nat. Prod. Rep., 1995, 12, 535–552 RSC;
(d) D. J. Robins, Nat. Prod. Rep., 1995, 12, 413–418 RSC;
(e) W. Glassco, J. Suchocki, C. George, B. R. Martin and E. L. May, J. Med. Chem., 1993, 36, 3381–3385 CrossRef PubMed;
(f) B. B. Snider, Y. Ahn and B. M. Foxman, Tetrahedron Lett., 1999, 40, 3339–3342 CrossRef;
(g) J. A. Nieman and M. D. Ennis, Org. Lett., 2000, 2, 1395–1397 CrossRef;
(h) S. R. Angle, D. Bensa and D. S. Belanger, J. Org. Chem., 2007, 72, 5592–5597 CrossRef.
-
(a) K. Zheng and R. Hong, Nat. Prod. Rep., 2019, 36, 1546–1575 RSC;
(b) L.-S. You, R. An, K. Liang, B. Cui and X.-H. Wang, Curr. Pharm. Des., 2016, 22, 4086–4093 CrossRef;
(c) F. Giordanetto and J. Kihlberg, J. Med. Chem., 2014, 57, 278–295 CrossRef;
(d) C. Heinis, Nat. Chem. Biol., 2014, 10, 696–698 CrossRef;
(e) J. Mallinson and I. Collins, Future Med. Chem., 2012, 4, 1409–1438 CrossRef.
-
(a) X. Yu and D. Sun, Molecules, 2013, 18, 6230–6268 CrossRef;
(b) C. A. Lipinski, F. Lombardo, B. W. Dominy and P. J. Feeney, Adv. Drug Deliv. Rev., 2001, 46, 3–26 CrossRef PubMed;
(c) E. M. Driggers, S. P. Hale, J. Lee and N. K. Terrett, Nat. Rev. Drug Discovery, 2008, 7, 608–624 CrossRef;
(d) E. Marsault and M. L. Peterson, J. Med. Chem., 2011, 54, 1961–2004 CrossRef.
-
(a) Q. Zhao and C. E. Schafmeister, J. Org. Chem., 2015, 80, 8968–8978 CrossRef;
(b) P. T. Quinn, P. D. Atwood, J. M. Tanski, T. F. Moore and F. Folmer-Andersen, J. Org. Chem., 2011, 76, 10020–10030 CrossRef;
(c) S. Guo, G. Wang and L. Ai, Tetrahedron: Asymmetry, 2013, 24, 480–491 CrossRef.
-
(a) C. D. Gutsche, A. E. Rowan, S. J. Rowan and T. Aida, Calixarenes: An Introduction, The Royal Society of Chemistry, Cambridge, U.K., 2008 Search PubMed;
(b) D. M. Homden and C. Redshaw, Chem. Rev., 2008, 108, 5086–5130 CrossRef;
(c) F. Yang, H. Guo and J. Vicens, J. Inclusion Phenom. Macrocyclic Chem., 2014, 80, 177–186 CrossRef;
(d) E. V. Ukhatskaya, S. V. Kurkov, S. E. Matthews and T. J. Loftsson, J. Pharmaceut. Sci., 2013, 102, 3485–3512 CrossRef PubMed;
(e) J. S. Kim and D. T. Quang, Chem. Rev., 2007, 107, 3780–3799 CrossRef PubMed.
-
(a) A. Dondoni and A. Marra, Chem. Rev., 2010, 110, 4949 CrossRef PubMed;
(b) J. S. Kim and D. T. Quang, Chem. Rev., 2007, 107, 3780–3799 CrossRef CAS PubMed;
(c) V. M. Centelles, M. D. Pandey, M. Isabel Burguete and S. V. Luis, Chem. Rev., 2015, 115, 8736–8834 CrossRef.
-
(a) S. Hu, J. Li, J. Xiang, J. Pan, S. Luo and J.-P. Cheng, J. Am. Chem. Soc., 2010, 132, 7216–7228 CrossRef CAS;
(b) R. Breslow, J. B. Doherty, G. Guillot and C. Lipsey, J. Am. Chem. Soc., 1978, 100, 3227–3322 CrossRef CAS;
(c) E. Anslyn and R. Breslow, J. Am. Chem. Soc., 1989, 111, 8931–8932 CrossRef CAS;
(d) A. Douhal, Chem. Rev., 2004, 104, 1955–1976 CrossRef CAS.
-
(a) J. Li and X. J. Loh, Cyclodextrin- Based Supramolecular Architectures: Syntheses, Structures, and Applications for Drug and Gene Delivery, Adv. Drug Delivery Rev., 2008, 60, 1000–1017 CrossRef CAS;
(b) G. Crini, Review: A History of Cyclodextrins, Chem. Rev., 2014, 114, 10940–10975 CrossRef CAS;
(c) E. M. Martin Del Valle, Cyclodextrins and Their Uses: a Review, Process Biochem., 2004, 39, 1033–1046 CrossRef.
-
(a) J. W. Lee, S. Samal, N. Selvapalam, H. Kim and K. Kim, Acc. Chem. Res., 2003, 36, 621–630 CrossRef CAS;
(b) J. Lagona, P. Mukhopadhyay, S. Chakrabarti and L. Isaacs, Angew. Chem., Int. Ed., 2005, 44, 4844–4870 CrossRef CAS;
(c) K. I. Assaf and W. M. Nau, Chem. Soc. Rev., 2015, 44, 394–418 RSC;
(d) F. Seidi, A. A. Shamsabadi, M. Amini, M. Shabanian and D. Crespy, Polym. Chem., 2019, 10, 3674–3711 RSC.
-
(a) J. L. Atwood and L. R. MacGillivray, Nature, 1997, 389, 469–472 CrossRef;
(b) J. R. Moran, S. Karbach and D. J. Cram, J. Am. Chem. Soc., 1982, 104, 5826–5828 CrossRef CAS;
(c) F. R. Pinacho Crisóstomo, A. Lledó, S. R. Shenoy, T. Iwasawa and J. Rebek, J. Am. Chem. Soc., 2009, 131, 7402–7410 CrossRef;
(d) M. M. D. Cominetti, D. L. Hughesb and S. E. Matthews, Org. Biomol. Chem., 2016, 14, 10161–10164 RSC.
-
(a) N. Natarajan, E. Brenner, D. Sémeril, D. Matt and J. Harrowfield, Eur. J. Org. Chem., 2017, 6100–6113 CrossRef CAS;
(b) N. Li, F. Yang, H. A. Stock, D. V. Dearden, J. D. Lamb and R. G. Harrison, Org. Biomol. Chem., 2012, 10, 7392–7401 RSC;
(c) B. A. Roberts, G. W. V. Cave, C. L. Rastonb and J. L. Scott, Green Chem., 2001, 3, 280–284 RSC.
-
(a) M. Li, F.-G. Klärner, J. Sakamoto and A. D. Schlüter, Chem.–Eur. J., 2013, 19, 13348–13354 CrossRef CAS;
(b) S. Lee, C.-H. Chen and A. H. Flood, Nat. Chem., 2013, 5, 704–710 CrossRef CAS PubMed;
(c) M. Fritzsche, A. Bohle, D. Dudenko, U. Baumeister, D. Sebastiani, G. Richardt, H. W. Spiess, M. R. Hansen and S. Höger, Angew. Chem., Int. Ed., 2011, 50, 3030–3033 CrossRef CAS PubMed;
(d) L.-l. Tian, C. Wang, S. Dawn, M. D. Smith, J. A. Krause and L. S. Shimizu, J. Am. Chem. Soc., 2009, 131, 17620–17629 CrossRef CAS PubMed.
-
(a) M. Bru, I. Alfonso, M. Bolte, M. I. Burguete and S. V. Luis, Chem. Commun., 2011, 47, 283–285 RSC;
(b) A. J. Gallant, M. Yun, M. Sauer, C. S. Yeung and M. J. MacLachlan, Org. Lett., 2005, 7, 4827–4830 CrossRef CAS PubMed;
(c) J. Gawronski, K. Gawronska, J. Grajewski, M. Kwit, A. Plutecka and U. Rychlewska, Chem.–Eur. J., 2006, 12, 1807–1817 CrossRef CAS PubMed;
(d) S. Guieu, A. K. Crane and M. J. MacLachlan, Chem. Commun., 2011, 47, 1169–1171 RSC.
-
(a) A.-M. Stadler, J.-J. Jiang, H.-P. Wang and C. Bailly, Chem. Commun., 2013, 49, 3784–3786 RSC;
(b) J. Jiang and M. J. MacLachlan, Org. Lett., 2010, 12, 1020–1023 CrossRef CAS PubMed;
(c) M. Kwit, A. Plutecka, U. Rychlewska, J. Gawroński, A. F. Khlebnikov, S. I. Kozhushkov, K. Rauch and A. de Meijere, Chem.–Eur. J., 2007, 13, 8688–8695 CrossRef CAS PubMed.
-
(a) A. M. Lopez-Periago, C. A. Garcia-Gonzalez and C. Domingo, Chem. Commun., 2010, 46, 4315–4317 RSC;
(b) A. Sarnicka, P. Starynowicz and J. Lisowski, Chem. Commun., 2012, 48, 2237–2239 RSC;
(c) J. W. Leeland, F. J. White and J. B. Love, J. Am. Chem. Soc., 2011, 133, 7320–7323 CrossRef CAS PubMed.
-
(a) Y. Yamauchi, D. Ajami, J.-Y. Lee and J. Rebek, Angew. Chem., Int. Ed., 2011, 50, 9150–9153 CrossRef CAS PubMed;
(b) M. Chas and P. Ballester, Chem. Sci., 2012, 3, 186–191 RSC;
(c) A. Asadi, D. Ajami and J. Rebek, J. Am. Chem. Soc., 2011, 133, 10682–10684 CrossRef CAS PubMed;
(d) H. Dube and J. Rebek, Angew. Chem., Int. Ed., 2012, 51, 3207–3210 CrossRef CAS PubMed;
(e) K. Tiefenbacher and J. Rebek, J. Am. Chem. Soc., 2012, 134, 2914–2917 CrossRef CAS PubMed.
-
(a) P. Ballester, Chem. Soc. Rev., 2010, 39, 3810–3830 RSC;
(b) P. Mal, D. Schultz, K. Beyeh, K. Rissanen and J. R. Nitschke, Angew. Chem., Int. Ed., 2008, 47, 8297–8301 CrossRef CAS PubMed;
(c) J.-F. Ayme, J. E. Beves, D. A. Leigh, R. T. McBurney, K. Rissanen and D. Schultz, Nat. Chem., 2011, 4, 15–20 CrossRef PubMed;
(d) Y. Wang, J. Xiang and H. Jiang, Chem.–Eur. J., 2011, 17, 613–619 CrossRef CAS PubMed.
-
(a) H. Li, R. Aneja and I. Chaiken, Molecules, 2013, 18, 9797–9817 CrossRef CAS PubMed;
(b) C. Chu and R. Liu, Chem. Soc. Rev., 2011, 40, 2177–2188 RSC;
(c) D. Astruc, L. Liang, A. Rapakousiou and J. Ruiz, Acc. Chem. Res., 2012, 45, 630–640 CrossRef CAS PubMed;
(d) J. Shang, N. M. Gallagher, F. Bie, Q. Li, Y. Che, Y. Wang and H. Jiang, J. Org. Chem., 2014, 79, 5134–5144 CrossRef CAS PubMed.
-
(a) C. NfflÇez, A. Aldrey, V. Garca, R. Bastida, A. Macas and C. Lodeiro, Inorg. Chim. Acta, 2012, 381, 85–94 CrossRef;
(b) C. Bazzicalupi, A. Bencini, I. Matera, S. Puccioni and B. Valtancoli, Inorg. Chim. Acta, 2012, 381, 162–169 CrossRef CAS;
(c) J. Beckmann, A. Duthie, G. Reeske and M. Schürmann, Organometallics, 2005, 24, 3629–3633 CrossRef CAS;
(d) T. Berg, Angew. Chem., Int. Ed., 2003, 42, 2462–2481 CrossRef CAS PubMed;
(e) J. A. Wells and L. McClendon, Nature, 2007, 450, 1001–1009 CrossRef CAS PubMed;
(f) Ahsanullah and J. Rademann, Angew. Chem., Int. Ed., 2010, 49, 5378–5382 CrossRef CAS PubMed.
-
(a) A. Von Baeyer, Ber. Dtsch. Chem. Ges., 1900, 33, 3771–3775 CrossRef CAS;
(b) J. Pospíšilová, V. Krchňák and E. Schütznerová, ACS Comb. Sci., 2019, 21, 1–5 CrossRef PubMed.
-
(a) S.-S. Li, S. Zhu, C. Chen, K. Duan, Q. Liu and J. Xiao, Org. Lett., 2019, 21, 1058–1062 CrossRef CAS PubMed;
(b) Y.-B. Shen, S.-S. Li, X. Liu, L. Yu, Y.-M. Sun, Q. Liu and J. Xiao, J. Org. Chem., 2019, 84, 3990–3999 CrossRef CAS PubMed;
(c) Y.-B. Shen, S.-S. Li, X. Liu, L. Yu, Q. Liu and J. Xiao, Adv. Synth. Catal., 2019, 361, 1453–1458 CrossRef CAS.
-
(a) J. S. Bindra, Oxindole Alkaloids, in Alkaloid Chemistry and Physiology, ed. R. H. F. Manske, Academic Press, New York, 1973, p. 14 Search PubMed;
(b) A. Padwa and W. H. Pearson, Synthetic Applications of 1,3 Dipolar Cycloaddition Chemistry Toward Heterocycles and Natural Products, Wiley, New York, 2002 CrossRef;
(c) G. S. Singh and Z. Y. Desta, Chem. Rev., 2012, 112, 6104–6155 CrossRef CAS PubMed;
(d) N. Lashgari and G. M. Ziarani, Arkivoc, 2012, 277–320 Search PubMed.
-
(a) X. Fang and C.-J. Wang, Org. Biomol. Chem., 2018, 16, 2591–2601 RSC;
(b) I. J. Enyedy, W. A. Zaman, S. Sakamuri, A. P. Kozikowski, K. M. Johnson and S. Wang, Bioorg. Med. Chem. Lett., 2001, 11, 1113 CrossRef CAS PubMed;
(c) C. V. Galliford and K. A. Scheidt, Angew. Chem., Int. Ed., 2007, 46, 8748–8758 CrossRef CAS PubMed;
(d) J. P. Michael, Nat. Prod. Rep., 2008, 25, 139–165 RSC;
(e) N. Deppermann, H. Thomanek, A. H. G. P. Prenzel and W. Maison, J. Org. Chem., 2010, 75, 5994–6000 CrossRef CAS PubMed.
-
(a) M. Ochi, K. Kawasaki, H. Kataoka, Y. Uchio and H. Nishi, Biochem. Biophys. Res. Commun., 2001, 283, 1118–1123 CrossRef CAS PubMed;
(b) A. Czarna, B. Beck, S. Srivastava, G. M. Popowicz, S. Wolf, Y. Huang, M. Bista, T. A. Holak and A. Dömling, Angew. Chem., Int. Ed., 2010, 49, 5352–5356 CrossRef CAS PubMed;
(c) S. Crosignani, C. Jorand-Lebrun, P. Page, G. Campbell, V. Colovray, M. Missotten, Y. Humbert, C. Cleva, J.-F. Arrighi, M. Gaudet, Z. Johnson, P. Ferro and A. Chollet, ACS Med. Chem. Lett., 2011, 2, 644–649 CrossRef CAS PubMed;
(d) J. I. Jimenez, U. Huber, R. E. Moore and G. M. L. J. Patterson, Nat. Prod., 1999, 62, 569–572 CrossRef CAS PubMed;
(e) S. Rana and A. Natarajan, Org. Biomol. Chem., 2013, 11, 244–247 RSC;
(f) B.-D. Cui, J. Zuo, J.-Q. Zhao, M.-Q. Zhou, Z.-J. Wu, X.-M. Zhang and W.-C. Yuan, J. Org. Chem., 2014, 79, 5305–5314 CrossRef CAS PubMed.
-
(a) P. C. Mhaske, S. H. Shelke, R. P. Jadhav, H. N. Raundal, S. V. Patil, A. A. Patil and V. D Bobade, J. Heterocycl. Chem., 2010, 47, 1415–1420 CrossRef CAS;
(b) P. Prasanna, K. Balamurugan, S. Perumal, P. Yogeeswari and D. Sriram, Eur. J. Med. Chem., 2010, 45, 5653–5661 CrossRef CAS PubMed;
(c) S. Purushothaman, R. Prasanna, S. Lavanya and R. Raghunathan, Tetrahedron Lett., 2013, 54, 5744–5747 CrossRef CAS;
(d) T. Usui, M. Kondoh, C. B. Cui, T. Mayumi and H. Osada, Biochem. J., 1998, 333, 543–548 CrossRef CAS PubMed.
-
(a) G. Periyasami, R. Raghunathan, G. Surendiran and N. Mathivanan, Bioorg. Med. Chem. Lett., 2008, 18, 2342–23425 CrossRef CAS PubMed;
(b) G. Bhaskar, Y. Arun, C. Balachandran, C. Saikumar and P. T Perumal, Eur. J. Med. Chem., 2012, 51, 79–91 CrossRef CAS PubMed;
(c) S. Haddad, S. Boudriga, F. Porzio, A. Soldera, M. Askri, M. Knorr, Y. Rousselin, M. M. Kubicki, C. Golz and C. Strohmann, J. Org. Chem., 2015, 80, 9064–9075 CrossRef CAS PubMed.
-
(a) M. A. Ali, R. Ismail, T. S. Choon, Y. K. Yoon, A. C. Wei, S. Pandian, R. S. Kumar, H. Osman and E. Manogaran, Bioorg. Med. Chem. Lett., 2010, 20, 7064–7066 CrossRef CAS PubMed;
(b) Y. Kia, H. Osman, R. S. Kumar, V. Murugaiyah, A. Basiri, S. Perumal and I. A. Razak, Bioorg. Med. Chem. Lett., 2013, 23, 2979–2983 CrossRef CAS PubMed;
(c) A. S. Filatov, N. A. Knyazev, A. P. Molchanov, T. L. Panikorovsky, R. R. Kostikov, A. G. Larina, V. M. Boitsov and A. V. Stepakov, J. Org. Chem., 2017, 82, 959–975 CrossRef CAS PubMed.
-
(a) C. J. A. Ribeiro, C. M. P. Rodrigues, R. Moreira and M. M. M. Santos, Pharmaceuticals, 2016, 9, 25–57 CrossRef PubMed;
(b) S. Nag, X. Zhang, K. S. Srivenugopal, M.-H. Wang, W. Wang and R. Zhang, Curr. Med. Chem., 2014, 21, 553–574 CrossRef CAS PubMed.
-
(a) A. A. Akaev, E. V. Villemson, N. S. Vorobyeva, A. G. Majouga, E. M. Budynina and M. Y. Melnikov, J. Org. Chem., 2017, 82, 5689–5701 CrossRef CAS PubMed;
(b) Y. Zhao, A. Aguilar, D. Bernard and S. Wang, J. Med. Chem., 2015, 58, 1038–1052 CrossRef CAS PubMed;
(c) Y. Zhao, S. Yu, W. Sun, L. Liu, J. Lu, D. McEachern, S. Shargary, D. Bernard, X. Li, T. Zhao, P. Zou, D. Sun and S. Wang, J. Med. Chem., 2013, 56, 5553–5561 CrossRef CAS PubMed.
-
(a) F. Shi, R. Mancuso and R. T. Larock, Tetrahedron Lett., 2009, 50, 4067–4070 CrossRef CAS PubMed;
(b) C. E. P. Galvis and V. V. Kouznetsov, Org. Biomol. Chem., 2013, 11, 7372–7386 RSC;
(c) N. Lashgari and G. M. Ziarani, ARKIVOC, 2012, 277–320 Search PubMed;
(d) S. Kamalraj and J. Muthumary, Eur. J. Med. Chem., 2011, 46, 600–607 CrossRef PubMed;
(e) I. Coldham and R. Hufton, Chem. Rev., 2005, 105, 2765–2810 CrossRef CAS PubMed;
(f) G. Pandey, P. Banerjee and S. R. Gadre, Chem. Rev., 2006, 106, 4484–4517 CrossRef CAS PubMed;
(g) M. Naodovic and H. Yamamoto, Chem. Rev., 2008, 108, 3132–3148 CrossRef CAS PubMed;
(h) J. Adrio and J. C. Carretero, Chem. Commun., 2011, 47, 6784–6794 RSC.
-
(a) J. Nájera and M. Sansano, Angew. Chem., Int. Ed., 2005, 44, 6272–6276 CrossRef PubMed;
(b) V. Nair and D. T. Suja, Tetrahedron, 2007, 63, 12247–12275 CrossRef CAS;
(c) H. Pellissier, Tetrahedron, 2007, 63, 3235–3285 CrossRef CAS;
(d) K. Anita and R. M. Marie, Chem. Soc. Rev., 2010, 39, 845–883 RSC;
(e) L. M. Stanley and M. P. Sibi, Chem. Rev., 2008, 108, 2887–2902 CrossRef CAS PubMed;
(f) A. Moyano and R. Rios, Chem. Rev., 2011, 111, 4703–4832 CrossRef CAS PubMed;
(g) C.-S. Wang, R.-Y. Zhu, J. Zheng, F. Shi and S.-J. Tu, J. Org. Chem., 2015, 80, 512–520 CrossRef CAS PubMed.
-
(a) R. Prasanna, S. Purushothaman and R. Raghunathan, Org. Biomol. Chem., 2014, 12, 9375–9383 RSC;
(b) G. Venkataramana, P. Dongare, L. N. Dawe, D. W. Thompson, Y. Zhao and G. J. Bodwell, Org. Lett., 2011, 13, 2240–2243 CrossRef CAS PubMed;
(c) I.-S. Tamgho, J. T. Engle and C. J. Ziegler, J. Org. Chem., 2012, 77, 11372–11376 CrossRef CAS PubMed;
(d) B. Lewandowski and S. Jarosz, Org. Lett., 2010, 12, 2532–2535 CrossRef CAS PubMed.
- P. Prabhakaran, M. Subaraja and P. Rajakumar, ChemistrySelect, 2018, 3, 4687–4693 CrossRef CAS.
-
(a) H. Ardill, M. J. R. Dorrity, R. Grigg, M. S. Leon-Ling, J. F. Malone, V. Sridharan and S. Thianpatanagul, Tetrahedron, 1990, 46, 6433–6448 CrossRef CAS;
(b) G. Subramaniyan, R. Raghunathan and M. Nethaji, Tetrahedron, 2002, 58, 9075–9079 CrossRef CAS;
(c) J.-M. Yang, Y. Hu, Q. Li, F. Yu, J. Cao, D. Fang, Z.-B. Huang and D.-Q. Shi, ACS Comb. Sci., 2014, 16, 139–145 CrossRef CAS PubMed.
Footnote |
† Electronic supplementary information (ESI) available. CCDC 1938425. For ESI and crystallographic data in CIF or other electronic format see DOI: 10.1039/c9ra10463a |
|
This journal is © The Royal Society of Chemistry 2020 |
Click here to see how this site uses Cookies. View our privacy policy here.