DOI:
10.1039/C9RA09227G
(Paper)
RSC Adv., 2020,
10, 4825-4831
Rose bengal as photocatalyst: visible light-mediated Friedel–Crafts alkylation of indoles with nitroalkenes in water†
Received
7th November 2019
, Accepted 21st January 2020
First published on 29th January 2020
Abstract
A novel and facile visible-light-mediated alkylation of indoles and nitroalkenes has been developed. In this protocol, rose bengal acts as a photosensitizer, and environmentally benign water was used as the green and efficient reaction medium. Indoles reacted smoothly with nitroalkenes under the irradiation of visible-light and generated corresponding 3-(2-nitroalkyl)indoles in moderate to good yields (up to 87%).
Introduction
Indole is a versatile building block that is widely used to synthesize bioactive natural products and drugs. The addition of indoles to electron-deficient-olefins, which can be considered as a Friedel–Crafts type alkylation, is a significant reaction in the formation of key C–C bonds.1
Many useful methods for preparing 3-(2-nitroalkyl)indoles have been reported by predecessors. For the past few years, several groups have been devoted to realizing asymmetric Friedel–Crafts alkylation of indoles with nitroalkenes by designing various kinds of new chiral catalysts, such as thiourea, azaindole, sulfonamide2 and organic metal complexes catalysts.3,4 In spite of the efficiency and generality of these reactions, it remains not enough for green and sustainable chemistry because these methods still involve the use of organic solvents, such as toluene and chloroform. Therefore, the development of a facile, efficient, economic and eco-friendly method to construct 3-(2-nitroalkyl)indoles is still highly desirable. Taking into account the ecological and sustainable point of view, water-soluble organic catalytic systems5 have attracted continuously increasing attention for applications in organic synthesis. Nevertheless, as a green alternative to organic solvents, the catalysis of reaction in water is still a major challenge in synthetic chemistry.6 In 2008, water has previously been found to drive the Michael reaction of indoles with nitroalkenes without the assistance of any catalyst, as reported by Jérôme and co-workers,7 but this method has the disadvantages of high reaction temperature (90 °C) and long reaction time (24 h). Besides, although impressive works have been accomplished in various Lewis acid catalytic systems in water, such as InBr3, CeCl3 and Sc(OTf)3,8 little is still known in the aqueous organic catalytic system.
In 2016, Jagdamba and coworkers developed an novel visible-light mediated method on the synthesis of 3-substituted indoles, employing green LED as light source in ethanol. The reaction has mild conditions and good to excellent yields, but only eight substrates were expanded.9 Wu and co-workers further investigated into the direct alkylation of unfunctionalized C–H bonds via photoredox induced radical cation deprotonation.9 Organic photoredox catalyst eosin-Y and 9-mesityl-10-methylacridinium ion (Mes–Acr+) have been employed in C–H bond activation for electron-deficient olefins. Compared with metal-containing photocatalyst, organic dyes such as eosin Y, rose bengal and fluorescein10 are mild in reaction conditions and easier to modify. In recent years, numerous works were reported using organic dyes11 as photoredox catalysts under visible light irradiation from low power source.
According to R. Lambert's research12 in 1997, which reported the triplet state rose bengal's reduction potential was 1.77 eV. That is, the reduction quenching will be thermodynamically favorable for indoles with relatively lower reduction potentials.13 To the best of our knowledge, rose bengal involved, visible light-mediated Friedel–Crafts alkylation of indoles with nitroalkenes in water has not been reported yet. In this process, the reaction was performed under a more gentle conditions in totally green solvent, combining the advantage of the high efficiency of photoinduced electron transfer (PET) process (Scheme 1).14
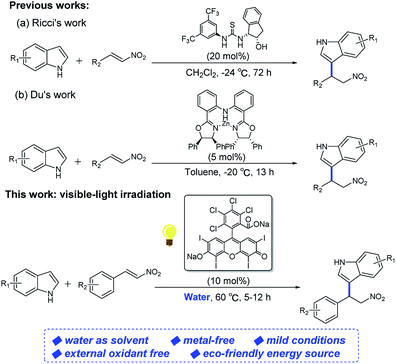 |
| Scheme 1 Comparative approaches for direct synthesis of 3-(2-nitroalkyl)indoles between the reported and this work. | |
Results and discussion
Initially, the visible light mediated alkylation of indoles with nitroalkenes was probed with N-methyl indole (1a) and (E)-2-nitroethenylbenzene (2a) as model substrates. Various kinds of photosensitizers were firstly investigated for the process. The reaction was carried out in tetrahydrofuran under the irradiation of 21 W white LED light (Table 1, entries 1–5). Among the photosensitizers tested, rose bengal showed the best catalytic effect, 3a was obtained in 31% yield. In spite of different kinds of organic solvents were examined for this catalytic system, poor yields were obtained (Table 1, entries 6–12). In contrast, reactions in THF and diethyl ether which have lower solubility to the system achieved relatively high yields (Table 1, entries 1, 9). Therefore, it is reducing the solubility of reaction system that may promote the reaction. To our delight, when water was used as the solvent, 3a was obtained in 54% yield (Table 1, entry 13). Control experiments were carried out in darkness or in the absence rose bengal, the yield decreased dramatically, thus verifying light and rose bengal are pivotal in the reaction (Table 1, entries 14–17).
Table 1 Alkylation of N-methyl indole (1a)a and (E)-2-nitroethenylbenzene (2a)a
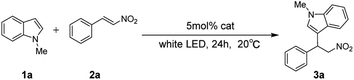
|
Entry |
Catalyst |
Solvent |
Yieldb (%) |
Unless otherwise specified, N-methyl indole 1a (39.4 mg, 0.3 mmol), (E)-2-nitroethenylbenzene 2a (44.7 mg, 0.3 mmol), and catalyst (0.015 mmol, 5 mol%), were added to a test tube equipped with a stirring bar and dissolved in 3 mL solvent. The mixture was stirred in air with exposure to 21 W white LED lamps at 20 °C for 24 h. Isolated yields. Reaction was performed at 60 °C for 6 h. Reaction was performed in darkness. |
1 |
Eosin Y |
THF |
26 |
2 |
Eosin B |
THF |
21 |
3 |
Mes–Acr+ (ClO4−) |
THF |
Trace |
4 |
Rose bengal |
THF |
31 |
5 |
Methylene blue |
THF |
Trace |
6 |
Rose bengal |
Toluene |
Trace |
7 |
Rose bengal |
Paraxylene |
21 |
8 |
Rose bengal |
Cyclohexane |
Trace |
9 |
Rose bengal |
Diethyl ether |
35 |
10 |
Rose bengal |
Ethyl acetate |
20 |
11 |
Rose bengal |
Acetone |
24 |
12 |
Rose bengal |
Methanol |
Trace |
13 |
Rose bengal |
Water |
54 |
14c |
Rose bengal |
Water |
61 |
15c,d |
Rose bengal |
Water |
18 |
16c |
None |
Water |
32 |
17c,d |
None |
Water |
17 |
To further optimize the reaction conditions, the effect of reaction temperature was investigated. To our delight, when reaction temperature raised from 20 °C to 60 °C, yield increased from 54% to 61% (Table 2, entries 1–3). What's more, reaction time reduced from 18 hours to 7 hours. On the contrary, when the reaction temperature continued to rise, side reaction increased, and the yield decreased to 53% (Table 2, entry 4). Taking the effect of the catalyst loading into account, the loading of catalyst was firstly reduced from 5 mol% to 0.5 mol%. However, as the catalyst loading reduced, reaction yield decreased from 61% to 52% (Table 2, entries 4–7), and the reaction time increased from 7 h to 12 h. Increasing catalyst loading to 10 mol%, yield of 3a raised to 64% (Table 2, entry 8). However, yield of 3a did not increase obviously when the catalyst loading raised to 20 mol% (Table 2, entry 9). Therefore, 10 mol% of rose bengal was chosen as the best photosensitizer loading. Subsequently, the molar ratio of substrates was screened, when the ratio of N-methyl indole and (E)-2-nitroethenylbenzene was 1
:
1.5, 3a was generated in the highest yield of 69% (Table 2, entries 10–14).
Table 2 Optimization of reaction conditionsa
Under the optimum reaction conditions, indoles and (E)-2-nitroethenylbenzenes with different kinds of substituents were investigated and the results were summarized in Table 3. (E)-2-Nitroethenylbenzene with divers functional groups at para position can smoothly carry out the Friedel–Crafts alkylation reaction with N-methyl indoles and afforded the desired products (3b–3d) in 75–81% yields, the para-fluoro-substituted (E)-2-nitroethenylbenzene substrate is capable of achieving a yield of up to 82%. Substrates with different functional groups attached to the indole ring were further investigated. It was gratifying to find that the substrates with both electron donating and electron withdrawing groups reacted smoothly and generated corresponding products (3f–3n) in 57–81% yields. In order to further demonstrate the tolerance of functional group, several substrates with substituents on the benzene ring of (E)-2-nitroethenylbenzenes were performed under this protocol. To our delight, (E)-2-nitroethenylbenzenes with electron withdrawing groups generated desired products (3p–3t) in 82–87% yields. What's more, 2-nitrovinylthiophene also reacted with 2-methylindole smoothly, and the corresponding product (3o) was obtained in 81% yield.
Table 3 Visible-light induced Friedel–Craft alkylation of indoles with nitroalkenes in the presence of rose bengala
Indole 1a–1m (1 equiv. 0.3 mmol), trans-β-nitrostyrenes 2a–2j (1.5 equiv. 0.45 mmol), water (3 mL) and 10 mol% of the rose bengal under a 21 W white LED lamps at 60 °C for 12 h. Isolated yield by column chromatography. |
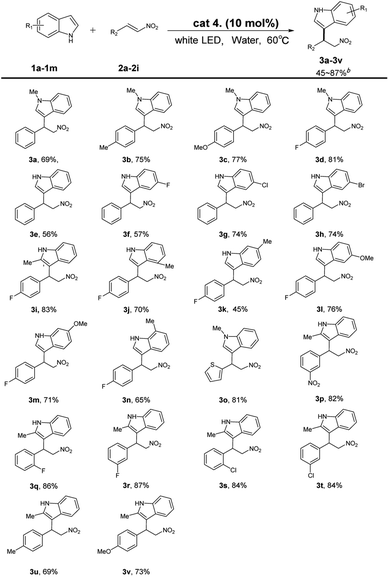 |
The reaction was scaled up to gram scale. Under the optimized reaction conditions, 2-methylindole 1i (1.318 g, 10 mmol) was treated with 4-fluoro-β-(E)-2-nitroethenylbenzene 2e (2.51 g, 15 mmol). Corresponding alkylated product 3i was obtained in 82% yield (Scheme 2).
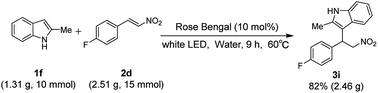 |
| Scheme 2 Gram scale Friedel–Crafts alkylation reaction. | |
In order to expound the mechanism of the process, a series of control experiments were carried out (Scheme 3). A trace amount of expected product 3a was observed in the presence of 2 equiv. of radical scavenger (TEMPO). And the raw material 1a was nearly quantitatively recovered (Scheme 3a). To further investigate whether oxygen is involved in this process, control experiments under oxygen and nitrogen atmosphere were performed (Scheme 3b). The results turned that oxygen is unnecessary in the reaction.
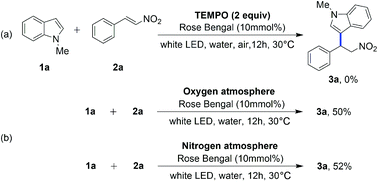 |
| Scheme 3 Control experiment. | |
Cyclic voltammetry experiments were performed in a CH Instruments Electrochemical Analyzer. From the result, E1/2ox (4) = −1.211 V vs. SCE (Fig. 1) is higher than E1/2red (1a) = −1.977 V vs. SCE (Fig. 2) so the photoredox reaction between rose bengal 4 and N-methyl indole (1a) could occur spontaneously. (Fig. S1 and S2†).
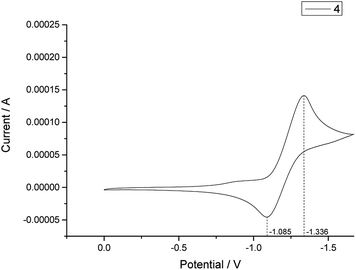 |
| Fig. 1 Cyclic voltammetry experiment of 4. | |
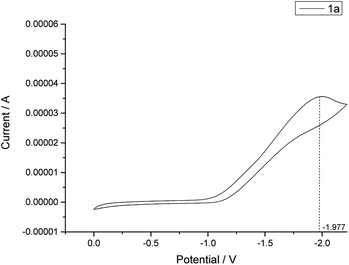 |
| Fig. 2 Cyclic voltammetry experiment of 1a. | |
Based on the experiment results and relevant references, plausible mechanism of this transformation was established. Due to the high oxidizing power of excited state rose bengal,14,15 olefins could be conveniently oxidized to radical cations by 4*. We proposed that the radical cation I tends to deprotonate to give the radical II.3 Addition of II to a Michael acceptor such as (E)-2-nitroethenylbenzene 2a will give alkyl III. Electron-concentrating group III was reduced by the rose bengal catalyst 4˙, and deliver the alkylated product 3a after protonation while regenerating the photocatalyst 4 (Scheme 4).16
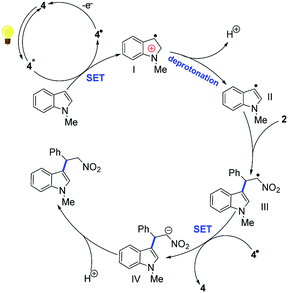 |
| Scheme 4 Plausible mechanism. | |
Conclusions
In summary, we have developed a visible-light-mediated alkylation of indoles with nitroalkenes employing rose bengal as the photocatalyst. Various types of nitroalkylated indoles were obtained in 45–87% yields. Compared with the previous methods, this process is environmental friendly, using water as the solvent without any bases and metals being involved. As a result, the method is simple in operation, mild in condition and high in atomic economy.
Experimental
General
Unless otherwise stated, all commercial reagents and solvents were used without further additional purification. Purification of reaction products was carried out with chromatography on silica gel 60 (200–400 mesh). 1H NMR (400 MHz) or (500 MHz) spectra was obtained at 25 °C; 13C NMR (101 MHz) were recorded on a Varian INOVA-400M and AVANCE II 400 spectrometer at 25 °C. Chemical shifts are reported as δ (ppm) values relative to TMS as internal standard and coupling constants (J) in Hz. HPLC analysis were performed on a waters liquid chromatograph equipped with a Yilite SinoChrom ODS-BP 5 μm column (4.6 mm × 250 mm), using mixtures of methanol/H2O as mobile phase, at 25 °C. HRMS analysis were performed on LTQ Orbitrap XL of Thermo Scientific. Cyclic voltammetry (CV) experiments were performed on CHI600E of Chenhua.
General procedure for the catalytic Friedel–Crafts reaction
In a dry vial bottle equipped with magnetic stirrer was added rose bengal (33.9 mg, 0.03 mmol), N-methylindole (41.0 mg, 0.3 mmol) and nitroalkenes (69.2 mg, 0.45 mmol) under an air atmosphere followed by the addition of water (3 mL). The solution was stirred under the irradiation of 21 W white LED lamps for 5 hours at 60 °C. The resulting suspension was cooled to room temperature. The aqueous layer was extracted with ethyl acetate (5 × 3 mL). The combined organic layer was dried over anhydrous Na2SO4 and filtered. The solvent was evaporated under reduced pressure. The residue was purified through silica gel column chromatography using hexane/ethyl acetate (5
:
1) as the eluent to afford 1-methyl-3-(2-nitro-1-phenylethyl)-1H-indole. There is about 5.0 cm distance between the lamps and reactors. The light intensity of the reaction system is 50
000 lux.
1-Methyl-3-(2-nitro-1-phenylethyl)-1H-indole (3a). Compound 3a was purified through silica gel column chromatography using hexane/ethyl acetate (5
:
1) as the eluent and was obtained as a yellow liquid, yield: 0.061 g, 71%; 1H NMR (500 MHz, CDCl3): δ 7.44 (d, J = 8.0 Hz, 1H), 7.37–7.17 (m, 7H), 7.07 (t, J = 7.5 Hz, 1H), 6.85 (s, 1H), 5.17 (t, J = 8.0 Hz, 1H), 5.04 (dd, J = 12.5, 7.5 Hz, 1H), 4.92 (dd, J = 12.6, 8.5 Hz, 1H), 3.73 (s, 3H).
1-Methyl-3-(2-nitro-1-(p-tolyl)ethyl)-1H-indole (3b). Compound 3b was purified through silica gel column chromatography using hexane/ethyl acetate (5
:
1) as the eluent and was obtained as a yellow liquid, yield: 0.066 g, 75%; 1H NMR (500 MHz, CDCl3): δ 7.43 (d, J = 8.0 Hz, 1H), 7.25 (d, J = 8.2 Hz, 1H), 7.20 (dd, J = 7.6, 4.6 Hz, 3H), 7.09 (d, J = 7.8 Hz, 2H), 7.04 (t, J = 7.5 Hz, 1H), 6.80 (s, 1H), 5.11 (t, J = 8.1 Hz, 1H), 4.98 (dd, J = 12.5, 7.5 Hz, 1H), 4.86 (dd, J = 12.5, 8.6 Hz, 1H), 3.66 (s, 3H), 2.28 (s, 3H).
3-(1-(4-Methoxyphenyl)-2-nitroethyl)-1-methyl-1H-indole (3c). Compound 3c was purified through silica gel column chromatography using hexane/ethyl acetate (5
:
1) as the eluent and was obtained as a yellow liquid, yield: 0.071 g, 77%; 1H NMR (500 MHz, CDCl3): δ 7.43 (dd, J = 8.2, 1.2 Hz, 1H), 7.36–7.14 (m, 4H), 7.05 (ddd, J = 8.0, 7.0, 1.1 Hz, 1H), 6.83 (d, J = 8.6 Hz, 3H), 5.11 (t, J = 8.0 Hz, 1H), 5.00 (dd, J = 12.4, 7.4 Hz, 1H), 4.86 (dd, J = 12.4, 8.6 Hz, 1H), 3.74 (s, 3H), 3.70 (s, 3H).
3-(1-(4-Fluorophenyl)-2-nitroethyl)-1-methyl-1H-indole (3d). Compound 3d was purified through silica gel column chromatography using hexane/ethyl acetate (5
:
1) as the eluent and was obtained as a yellow liquid, yield: 0.073 g, 81%; 1H NMR (500 MHz, CDCl3): δ 7.39 (d, J = 8.0 Hz, 1H), 7.28 (dt, J = 8.9, 2.5 Hz, 3H), 7.21 (dd, J = 9.5, 5.8 Hz, 1H), 7.06 (t, J = 7.5 Hz, 1H), 6.98 (t, J = 8.5 Hz, 2H), 6.82 (s, 1H), 5.14 (t, J = 8.0 Hz, 1H), 5.00 (dd, J = 12.5, 7.4 Hz, 1H), 4.86 (dd, J = 12.6, 8.7 Hz, 1H), 3.71 (s, 3H).
3-(2-Nitro-1-phenylethyl)-1H-indole (3e). Compound 3e was purified through silica gel column chromatography using hexane/ethyl acetate (5
:
1) as the eluent and was obtained as a yellow liquid, yield: 0.045 g, 56%; 1H NMR (500 MHz, CDCl3): δ 8.02 (s, 1H), 7.42 (d, J = 8.0 Hz, 1H), 7.29 (d, J = 6.9 Hz, 5H), 7.25–7.20 (m, 1H), 7.17 (t, J = 7.6 Hz, 1H), 7.05 (t, J = 7.5 Hz, 1H), 6.93 (d, J = 2.5 Hz, 1H), 5.15 (t, J = 8.0 Hz, 1H), 5.01 (dd, J = 12.5, 7.7 Hz, 1H), 4.90 (dd, J = 12.6, 8.4 Hz, 1H).
5-Fluoro-3-(2-nitro-1-phenylethyl)-1H-indole (3f). Compound 3f was purified through silica gel column chromatography using hexane/ethyl acetate (5
:
1) as the eluent and was obtained as a yellow liquid, yield: 0.051 g, 57%; 1H NMR (500 MHz, CDCl3): δ 8.11 (s, 1H), 7.33–7.16 (m, 6H), 7.05–7.00 (m, 2H), 6.90 (td, J = 9.0, 2.5 Hz, 1H), 5.08 (t, J = 7.9 Hz, 1H), 5.00 (dd, J = 12.4, 8.0 Hz, 1H), 4.89 (dd, J = 12.3, 7.9 Hz, 1H).
5-Chloro-3-(2-nitro-1-phenylethyl)-1H-indole (3g). Compound 3g was purified through silica gel column chromatography using hexane/ethyl acetate (5
:
1) as the eluent and was obtained as a yellow liquid, yield: 0.067 g, 74%; 1H NMR (500 MHz, CDCl3): δ 8.15 (s, 1H), 7.39–7.21 (m, 7H), 7.12 (dd, J = 8.6, 2.0 Hz, 1H), 7.04 (dd, J = 10.8, 2.5 Hz, 1H), 5.11 (t, J = 8.0 Hz, 1H), 5.06–4.94 (m, 1H), 4.90 (dd, J = 12.5, 7.9 Hz, 1H).
5-Bromo-3-(2-nitro-1-phenylethyl)-1H-indole (3h). Compound 3h was purified through silica gel column chromatography using hexane/ethyl acetate (5
:
1) as the eluent and was obtained as a yellow liquid, yield: 0.076 g, 74%; 1H NMR (500 MHz, CDCl3): δ 8.14 (s, 1H), 7.54 (d, J = 1.7 Hz, 1H), 7.32 (t, J = 1.3 Hz, 1H), 7.31–7.28 (m, 2H), 7.29–7.25 (m, 2H), 7.24 (d, J = 1.7 Hz, 1H), 7.18 (d, J = 8.6 Hz, 1H), 7.03 (d, J = 2.6 Hz, 1H), 5.11 (t, J = 8.0 Hz, 1H), 5.03–4.97 (m, 1H), 4.90 (dd, J = 12.6, 8.0 Hz, 1H).
3-(1-(4-Fluorophenyl)-2-nitroethyl)-2-methyl-1H-indole (3i). Compound 3i was purified through silica gel column chromatography using hexane/ethyl acetate (5
:
1) as the eluent and was obtained as a yellow liquid, yield: 0.105 g, 83%; 1H NMR (500 MHz, CDCl3): δ 8.09 (s, 1H), 7.45 (d, J = 1.6 Hz, 1H), 7.27–7.21 (m, 1H), 7.20–7.07 (m, 5H), 6.98 (d, J = 2.5 Hz, 1H), 5.08 (t, J = 8.0 Hz, 1H), 4.99 (dd, J = 12.4, 8.0 Hz, 1H), 4.88 (dd, J = 12.4, 7.9 Hz, 1H), 2.29 (s, 3H).
3-(1-(4-Fluorophenyl)-2-nitroethyl)-4-methyl-1H-indole (3j). Compound 3j was purified through silica gel column chromatography using hexane/ethyl acetate (5
:
1) as the eluent and was obtained as a yellow liquid, yield: 0.062 g, 70%; 1H NMR (500 MHz, CDCl3): 1H NMR (500 MHz, chloroform-d) δ 8.15–7.95 (m, 1H), 7.32–7.27 (m, 2H), 7.26 (d, J = 4.7 Hz, 1H), 7.09–6.81 (m, 5H), 5.16 (t, J = 8.0 Hz, 1H), 5.05 (dd, J = 12.5, 7.4 Hz, 1H), 4.90 (dd, J = 12.5, 8.6 Hz, 1H), 2.47 (s, 3H). 13C NMR (101 MHz, CDCl3) δ 163.29, 160.85, 136.13, 129.40, 129.32, 125.51, 123.34, 121.15, 120.69, 120.30, 116.57, 115.91, 115.70, 114.77, 79.54, 40.99, 16.51. HRMS (m/z): (ESI) calcd for C17H15FN2O2 [M + H]+: 219.1190, found: 219.1196.
3-(1-(4-Fluorophenyl)-2-nitroethyl)-6-methyl-1H-indole (3k). Compound 3k was purified through silica gel column chromatography using hexane/ethyl acetate (5
:
1) as the eluent and was obtained as a yellow liquid, yield: 0.041 g, 45%; 1H NMR (500 MHz, CDCl3): δ 7.98 (s, 1H), 7.35–7.21 (m, 3H), 7.15 (s, 1H), 7.06–6.96 (m, 2H), 6.96–6.83 (m, 2H), 5.14 (t, J = 8.0 Hz, 1H), 5.04 (dd, J = 12.5, 7.4 Hz, 1H), 4.88 (dd, J = 12.4, 8.6 Hz, 1H), 2.43 (s, 3H). 13C NMR (101 MHz, CDCl3) δ 163.31, 160.87, 137.03, 135.09, 132.80, 129.43, 123.83, 121.85, 120.83, 118.52, 115.92, 115.71, 114.13, 111.40, 79.60, 40.97, 21.66. HRMS (m/z): (ESI) calcd for C17H15FN2O2 [M + H]+: 219.1190, found: 219.1197.
3-(1-(4-Fluorophenyl)-2-nitroethyl)-5-methoxy-1H-indole (3l). Compound 3l was purified through silica gel column chromatography using hexane/ethyl acetate (5
:
1) as the eluent and was obtained as a yellow liquid, yield: 0.072 g, 76%; 1H NMR (500 MHz, CDCl3): δ 8.04 (s, 1H), 7.25 (dd, J = 8.3, 5.3 Hz, 2H), 7.18 (d, J = 8.8 Hz, 1H), 6.96 (t, J = 8.5 Hz, 2H), 6.93–6.89 (m, 1H), 6.84 (dd, J = 8.8, 2.3 Hz, 1H), 6.79 (d, J = 2.5 Hz, 1H), 5.08 (t, J = 8.0 Hz, 1H), 4.98 (dd, J = 12.4, 7.5 Hz, 1H), 4.84 (dd, J = 12.5, 8.5 Hz, 1H), 3.75 (s, 3H).
3-(1-(4-Fluorophenyl)-2-nitroethyl)-6-methoxy-1H-indole (3m). Compound 3m was purified through silica gel column chromatography using hexane/ethyl acetate (5
:
1) as the eluent and was obtained as a yellow liquid, yield: 0.067 g, 71%; 1H NMR (500 MHz, CDCl3): δ 8.00 (s, 1H), 7.35–7.17 (m, 3H), 7.04–6.93 (m, 2H), 6.88 (dd, J = 2.5, 0.9 Hz, 1H), 6.82 (d, J = 2.2 Hz, 1H), 6.73 (dd, J = 8.7, 2.3 Hz, 1H), 5.10 (t, J = 7.9 Hz, 1H), 5.01 (dd, J = 12.4, 7.4 Hz, 1H), 4.86 (dd, J = 12.4, 8.5 Hz, 1H), 3.80 (s, 3H). HRMS (m/z): (ESI) calcd for C17H15FN2O3 [M + H]+: 315.1140, found: 315.1140.
3-(1-(4-Fluorophenyl)-2-nitroethyl)-7-methyl-1H-indole (3n). Compound 3n was purified through silica gel column chromatography using hexane/ethyl acetate (5
:
1) as the eluent and was obtained as a yellow liquid, yield: 0.058 g, 65%; 1H NMR (500 MHz, CDCl3): δ 8.15 (s, 1H), 7.28–7.14 (m, 3H), 7.14–7.04 (m, 2H), 7.03–6.94 (m, 2H), 6.81 (d, J = 7.2 Hz, 1H), 5.52 (t, J = 8.1 Hz, 1H), 4.95 (dd, J = 12.6, 8.3 Hz, 1H), 4.81 (dd, J = 12.6, 7.9 Hz, 1H), 2.53 (s, 3H). HRMS (m/z): (ESI) calcd for C17H15FN2O2 [M + H]+: 219.1190, found: 219.1194.
1-Methyl-3-(2-nitro-1-(thiophen-2-yl)ethyl)-1H-indole (3o). Compound 3o was purified through silica gel column chromatography using hexane/ethyl acetate (5
:
1) as the eluent and was obtained as a yellow liquid, yield: 0.070 g, 81%; 1H NMR (500 MHz, CDCl3): δ 7.49 (d, J = 8.0 Hz, 1H), 7.28 (d, J = 8.2 Hz, 1H), 7.22 (t, J = 7.6 Hz, 1H), 7.16 (d, J = 5.1 Hz, 1H), 7.08 (t, J = 7.5 Hz, 1H), 7.00–6.87 (m, 3H), 5.42 (t, J = 7.9 Hz, 1H), 4.96 (qd, J = 12.6, 8.0 Hz, 2H), 3.69 (s, 3H).
2-Methyl-3-(2-nitro-1-(3-nitrophenyl)ethyl)-1H-indole (3p). Compound 3p was purified through silica gel column chromatography using hexane/ethyl acetate (5
:
1) as the eluent and was obtained as a yellow liquid, yield: 0.079 g, 82%; 1H NMR (500 MHz, CDCl3): δ 7.81 (s, 1H), 7.46 (d, J = 7.8 Hz, 1H), 7.36 (td, J = 7.8, 1.8 Hz, 1H), 7.21–7.13 (m, 2H), 7.11–6.95 (m, 4H), 5.38 (dd, J = 9.3, 6.8 Hz, 1H), 5.18 (dd, J = 12.7, 6.8 Hz, 1H), 5.11 (dd, J = 12.7, 9.3 Hz, 1H), 2.32 (s, 3H).
3-(1-(2-Fluorophenyl)-2-nitroethyl)-2-methyl-1H-indole (3q). Compound 3q was purified through silica gel column chromatography using hexane/ethyl acetate (5
:
1) as the eluent and was obtained as a yellow liquid, yield: 0.077 g, 86%; 1H NMR (500 MHz, CDCl3): δ 8.18 (t, J = 2.0 Hz, 1H), 8.08 (ddd, J = 8.2, 2.3, 1.0 Hz, 1H), 8.01 (s, 1H), 7.69–7.61 (m, 1H), 7.46 (t, J = 8.0 Hz, 1H), 7.33–7.20 (m, 2H), 7.17–7.09 (m, 1H), 7.03 (td, J = 7.5, 7.1, 1.0 Hz, 1H), 5.33–5.23 (m, 2H), 5.16–5.06 (m, 1H), 2.43 (s, 3H). 13C NMR (101 MHz, CDCl3) δ 148.53, 141.88, 135.50, 133.49, 133.19, 129.84, 126.33, 122.29, 121.77, 120.16, 118.02, 111.03, 107.68, 77.90, 40.14, 29.73, 12.07. HRMS (m/z): (ESI) calcd for C17H15FN2O2 [M + H]+: 219.1190, found: 219.1197.
3-(1-(3-Fluorophenyl)-2-nitroethyl)-2-methyl-1H-indole (3r). Compound 3r was purified through silica gel column chromatography using hexane/ethyl acetate (5
:
1) as the eluent and was obtained as a yellow liquid, yield: 0.078 g, 87%; 1H NMR (500 MHz, CDCl3): δ 7.86 (s, 1H), 7.31 (d, J = 7.9 Hz, 1H), 7.26–7.17 (m, 2H), 7.13–7.05 (m, 2H), 7.04–6.94 (m, 2H), 6.89 (td, J = 8.4, 2.6 Hz, 1H), 5.20–5.11 (m, 2H), 5.09–4.99 (m, 1H), 2.31 (s, 3H). HRMS (m/z): (ESI) calcd for C17H15FN2O2 [M + H]+: 219.1190, found: 219.1195.
3-(1-(2-Chlorophenyl)-2-nitroethyl)-2-methyl-1H-indole (3s). Compound 3s was purified through silica gel column chromatography using hexane/ethyl acetate (5
:
1) as the eluent and was obtained as a yellow liquid, yield: 0.079 g, 84%; 1H NMR (500 MHz, CDCl3): δ 7.81 (s, 1H), 7.54–7.44 (m, 2H), 7.34 (dd, J = 7.6, 1.8 Hz, 1H), 7.22–7.12 (m, 3H), 7.11–6.98 (m, 2H), 5.47 (dd, J = 8.8, 7.3 Hz, 1H), 5.16–5.06 (m, 2H), 2.31 (s, 3H).
3-(1-(3-Chlorophenyl)-2-nitroethyl)-2-methyl-1H-indole (3t). Compound 3t was purified through silica gel column chromatography using hexane/ethyl acetate (5
:
1) as the eluent and was obtained as a yellow liquid, yield: 0.079 g, 84%; 1H NMR (500 MHz, CDCl3): δ 7.91 (s, 1H), 7.36–7.30 (m, 1H), 7.29–7.22 (m, 2H), 7.22–7.15 (m, 3H), 7.11 (ddd, J = 8.0, 7.1, 1.1 Hz, 1H), 7.03 (td, J = 7.5, 7.0, 1.1 Hz, 1H), 5.22–5.11 (m, 2H), 5.06 (dd, J = 11.2, 7.7 Hz, 1H), 2.37 (s, 3H). HRMS (m/z): (ESI) calcd for C17H15ClN2O2 [M + H]+: 315.0895, found: 315.0881.
2-Methyl-3-(2-nitro-1-(p-tolyl)ethyl)-1H-indole (3u). Compound 3u was purified through silica gel column chromatography using hexane/ethyl acetate (5
:
1) as the eluent and was obtained as a yellow liquid, yield: 0.061 g, 69%; 1H NMR (500 MHz, CDCl3): δ 7.83 (s, 1H), 7.36 (d, J = 8.0 Hz, 1H), 7.27–7.22 (m, 1H), 7.19 (d, J = 8.0 Hz, 2H), 7.13–7.06 (m, 3H), 7.02 (td, J = 7.6, 7.1, 1.1 Hz, 1H), 5.20 (dd, J = 11.1, 6.5 Hz, 1H), 5.17–5.12 (m, 1H), 5.08 (dd, J = 11.1, 8.2 Hz, 1H), 2.37 (s, 3H), 2.29 (s, 3H).
3-(1-(4-Methoxyphenyl)-2-nitroethyl)-2-methyl-1H-indole (3v). Compound 3v was purified through silica gel column chromatography using hexane/ethyl acetate (5
:
1) as the eluent and was obtained as a yellow liquid, yield: 0.068 g, 73%; 1H NMR (500 MHz, CDCl3): δ 7.84 (s, 1H), 7.35 (d, J = 7.9 Hz, 1H), 7.23–7.15 (m, 3H), 7.10–7.04 (m, 1H), 7.00 (ddd, J = 8.2, 7.1, 1.1 Hz, 1H), 6.82–6.76 (m, 2H), 5.18–5.07 (m, 2H), 5.03 (dd, J = 10.6, 7.8 Hz, 1H), 3.71 (s, 3H), 2.28 (s, 3H).
Conflicts of interest
There are no conflicts to declare.
Acknowledgements
We would like to thank the National Natural Science Foundation of China (No. 21476041, U1608224, 61633006) and the State Key Laboratory of Fine Chemicals for their support.
Notes and references
-
(a) G. A. Olah, R. Khrisnamurthi and G. K. S. Prakash, in Comprehensive Organic Synthesis, Pergamon Press, New York, 1st edn, 1991, pp. 293–339 Search PubMed;
(b) R. M. Roberts and A. A. Khalaf, Friedel–Crafts Alkylation Chemistry A Century of Discovery, Marcel Dekker, New York, 1984 Search PubMed.
-
(a) R. P. Herrera, V. Sgarzani, L. Bernardi and A. Ricci, Angew. Chem., Int. Ed., 2005, 44(40), 6576–6579 CrossRef CAS PubMed;
(b) N. Takenaka, R. S. Sarangthem and S. K. Seerla, Org. Lett., 2007, 9(15), 2819–2822 CrossRef CAS PubMed;
(c) E. M. Fleming, T. Mccabe and S. J. Connon, Tetrahedron Lett., 2006, 47(39), 7037–7042 CrossRef CAS;
(d) F. Yang, P. Curtis and K. S. Robert, J. Org. Chem., 2018, 83, 10855–10863 CrossRef;
(e) W. Zhuang, R. G. Hazell and K. A. Joergensen, Org. Biomol. Chem., 2005, 3(14), 2566–2571 RSC;
(f) G. Yanlong, B. Joel and J. Francois, Adv. Synth. Catal., 2008, 350, 2007–2012 CrossRef;
(g) H. Liu and D. M. Du, Adv. Synth. Catal., 2010, 352, 1113–1118 CrossRef CAS.
-
(a) D. A. Evans, K. A. Scheidt, K. R. Fandrick, H. W. Lam and J. Wu, J. Am. Chem. Soc., 2003, 125, 10780–10781 CrossRef CAS PubMed;
(b) K. A. Joergensen, Synthesis, 2003, 2003(07), 594–605 Search PubMed;
(c) S. F. Lu, D. A. Daming and J. X. Xu, Org. Lett., 2006, 8(10), 2115–2118 CrossRef CAS PubMed;
(d) S. Z. Lin and T. P. You, Tetrahedron, 2010, 40(23), 1010–1016 Search PubMed;
(e) M. Bandini, M. Fagioli, P. Melchiorre, A. Melloni and A. U. Ronchi, Tetrahedron Lett., 2003, 44(31), 5843–5846 CrossRef CAS.
-
(a) G. Bartoli, M. Bosco, S. Giuli, A. Giuliani, L. Lucarelli and E. Torregiani, J. Org. Chem., 2005, 70(5), 1941–1944 CrossRef CAS PubMed;
(b) M. M. Alam, R. Varala and S. R. Adapa, Tetrahedron, 2003, 44(27), 5115–5119 CrossRef CAS;
(c) M. Bandini, P. G. Cozzi and M. J. Giacomini, J. Org. Chem., 2002, 33(44), 3700–3704 CrossRef PubMed;
(d) M. Agnusdei, M. Bandini and A. J. Melloni, J. Org. Chem., 2003, 68(18), 7126–7129 CrossRef CAS;
(e) H. Firouzabadi, N. Iranpoor and F. Nowrouzi, Chem. Commun., 2005, 789–791 RSC;
(f) J. Xie, X. Zhu, M. Huang, M. Wang and Y. Wan, Synth. Commun., 2010, 40(21), 3259–3267 CrossRef CAS;
(g) R. S. Kumar, J. Heterocycl. Chem., 2006, 43(5), 1383–1385 CrossRef CAS;
(h) C. Lin, J. Hsu and M. N. V. Sastry, Tetrahedron, 2006, 37(12), 11751–11757 Search PubMed;
(i) K. Venkatanna, S. Y. Kumar, M. Karthick, R. Padmanaban and C. R. Ramanathan, Org. Biomol. Chem., 2019, 17(16), 4077–4086 RSC;
(j) N. Azizi, F. Arynasab and M. R. Saidi, Org. Biomol. Chem., 2006, 4(23), 4275 RSC.
-
(a) Z. C. Fu, N. Chen and Z. H. Yang, Green
Chem., 2018, 20, 4484 RSC;
(b) Z. H. Yang, Z. P. Zhu and R. Luo, Green Chem., 2017, 19, 3296 RSC.
-
(a) Z. H. Yang, R. S. Luo and Z. P. Zhu, Organometallics, 2017, 36, 4095–4098 CrossRef CAS;
(b) Z. H. Yang, Z. P. Zhu and R. S. Luo, Green Chem., 2017, 19, 3296–3301 RSC;
(c) D. S. Merel and J. L. Renaud, ChemCatChem, 2013, 5(10), 2939–2945 CrossRef CAS.
- Y. L. Gu, J. l. Barrault and F. Jérôme, Adv. Synth. Catal., 2008, 350, 2007–2012 CrossRef CAS.
-
(a) G. Bartoli, M. Bosco, S. Giuli, A. Giuliani, L. Lucarelli, E. Marcantoni, L. Sambri and E. Torregiani, J. Org. Chem., 2005, 70, 1941–1944 CrossRef CAS PubMed;
(b) M. Agnusdei, M. Bandini, A. Melloni and A. U. Ronchi, J. Org. Chem., 2003, 68, 7126–7129 CrossRef CAS PubMed;
(c) J. W. Xie, X. H. Zhu, M. Huang, F. Meng, M. Wang and Y. Wan, Synth. Commun., 2010, 40(21), 3259–3267 CrossRef CAS;
(d) R. S. Kumar and P. T. Perumal, J. Heterocycl. Chem., 2006, 43, 1383 CrossRef CAS;
(e) M. Damodiran, R. S. Kumar, P. M. Sivakumar, M. Doble and P. T. Perumal, J. Chem. Sci., 2009, 121(1), 65–73 CrossRef CAS;
(f) H. Firouzabadi, N. Iranpoor and F. Nowrouzi, Chem. Commun., 2005, 789–791 RSC.
-
(a) S. Yadav, M. Srivastava and J. Singh, New J. Chem., 2016, 40, 9694 RSC;
(b) R. Zhou, H. Liu, H. Tao and J. Wu, Chem. Sci., 2017, 8, 4654–4659 RSC;
(c) Y. Pan, C. W. Kee, L. Chen and C. H. Tan, Green Chem., 2011, 13(10), 2682 RSC;
(d) L. Y. Xie, T. G. Fang, J. X. Tan and W. M. He, Green Chem., 2019, 5(21), 3858 RSC;
(e) Y. Liang, L. Teng, Y. Wang and H. Cao, Green Chem., 2019, 21(15), 4025–4029 RSC;
(f) H. P. Deng, Q. Zhou and J. Wu, Angew. Chem., Int. Ed., 2018, 57, 12661–12665 CrossRef CAS;
(g) X. Z. Fan, J. W. Rong, H. L. Wu and J. Wu, Angew. Chem., Int. Ed., 2018, 57, 8514–8518 CrossRef CAS PubMed;
(h) J. H. Xu, W. B. Wu and J. Wu, Org. Lett., 2019, 21, 5321–5325 CrossRef CAS PubMed;
(i) D. Chandrasekhar, S. Adhikary, J. B. Nanubolu and R. A. Maurya, Org. Lett., 2016, 18, 2974–2977 CrossRef CAS PubMed;
(j) A. S. Jadhav and R. V. Anand, Org. Biomol. Chem., 2017, 15, 56–60 RSC.
-
(a) Y. Pan, C. W. Kee, L. Chen and C. H. Tan, Green Chem., 2011, 13(10), 2682 RSC;
(b) Q. Liu, Y. N. Li, H. H. Zhang, B. Chen, C. H. Tung and L. Z. Wu, Chem.–Eur. J., 2012, 18, 620–627 CrossRef CAS PubMed;
(c) D. P. Hari and B. Konig, Org. Lett., 2011, 13(15), 3852–3855 CrossRef CAS PubMed;
(d) K. Wang, L. G. Meng, Q. Zhang and L. Wang, Green Chem., 2016, 18, 2864–2870 RSC.
-
(a) F. Lima, L. Grunenberg, H. B. A. Rahman and R. Labes, Chem. Commun., 2018, 54, 5606–5609 RSC;
(b) I. Ghosh and B. Konig, Angew. Chem., Int. Ed., 2016, 55, 7676–7679 CrossRef CAS PubMed;
(c) G. Kibriya, A. K. Bagdi and A. Hajra, J. Org. Chem., 2018, 83, 10619–10626 CrossRef CAS PubMed;
(d) G. Y. Zhao, S. Kaur and T. Wan, Org. Lett., 2017, 19, 3291–3294 CrossRef CAS PubMed;
(e) J. D. Tibbetts, D. R. Carbery and E. A. C. Emanuelsson, ACS Sustainable Chem. Eng., 2017, 5, 9826–9835 CrossRef CAS;
(f) I. I. Roslan, K. Ng, M. A. Gondal, C. Basheer, M. A. Dastageerb and S. J. G. K. Chuah, Adv. Synth. Catal., 2018, 360(8), 1584–1589 CrossRef CAS.
-
(a) N. A. Romero and D. A. Nicewicz, Chem. Rev., 2016, 116(17), 10075–10166 CrossRef CAS;
(b) L. Buzzetti, G. E. M. Crisenza and P. Melchiorre, Angew. Chem., Int. Ed., 2019, 58(12), 3730–3747 CrossRef CAS;
(c) Y. Y. Chen, L. Q. Lu, D. G. Yu, C. J. Zhu and W. J. Xiao, Sci. China: Chem., 2019, 62, 24–57 CrossRef CAS;
(d) C. R. Lambert and I. E. Kocheva, Photochem. Photobiol., 1997, 66(1), 15–25 CrossRef CAS PubMed;
(e) P. C. C. Lee and M. A. J. Rodgers, Photochem. Photobiol., 1987, 45(1), 79–86 CrossRef CAS PubMed;
(f) P. M. Suardi, E. Gassmann, M. Braun and E. Oliveros, Helv. Chim. Acta, 1987, 70, 1760–1773 CrossRef.
-
(a) I. Willner and E. Joselevich, J. Phys. Chem. B, 1999, 103(43), 9262–9268 CrossRef CAS;
(b) L. Ludvíkova, P. Stacko, J. Sperry and P. Klan, J. Org. Chem., 2018, 83, 10835–10844 CrossRef PubMed;
(c) H. G. Roth and D. A. Nicewicz, Synlett, 2016, 27, 714–723 CAS.
-
(a) N. A. Romero and D. A. Nicewicz, Chem. Rev., 2016, 116(17), 10075–10166 CrossRef CAS PubMed;
(b) D. S. Merel and J. L. Renaud, ChemCatChem, 2013, 5(10), 2939–2945 CrossRef CAS.
-
(a) Y. Pan, S. Wang, C. W. Kee, E. Dubuisson and C. H. Tan, Green Chem., 2011, 13, 3341–3347 RSC;
(b) Y. Pan, C. W. Kee, L. Chen and C. H. Tan, Green Chem., 2011, 13, 2682–2689 RSC;
(c) C. R. Lambert and I. E. Kocheva, Photochem. Photobiol., 1997, 66(1), 15–25 CrossRef CAS PubMed.
- R. Zhou, H. Liu, H. Tao and J. Wu, Chem. Sci., 2017, 8, 4654–4659 RSC.
Footnote |
† Electronic supplementary information (ESI) available. See DOI: 10.1039/c9ra09227g |
|
This journal is © The Royal Society of Chemistry 2020 |