DOI:
10.1039/C9QO01228A
(Research Article)
Org. Chem. Front., 2020,
7, 119-125
Practical fluorothiolation and difluorothiolation of alkenes using pyridine-HF and N-thiosuccinimides†
Received
8th October 2019
, Accepted 22nd November 2019
First published on 22nd November 2019
Abstract
We have developed highly efficient fluorothiolation and difluorothiolation of alkenes under simple and mild conditions. Our fluorothiolation reagents are the commercially available Olah's reagent (Py-HF) and readily available N-thiosuccinimides. This transition metal-free system offers good chemical yields for a wide range of alkene substrates with excellent regioselectivity and good functional group tolerance.
Sulfur-containing molecules1 widely exist in natural products,2–4 pharmaceuticals5,6 and agrochemicals.7–9 Examples include the nonsteroidal anti-inflammatory drug sulindac10 and the basal cell carcinoma treatment drug vismodegib.11 Therefore, efficient introduction of sulfur atoms into organic molecules has drawn much attention.12–16 Similarly, the prominent roles of organofluorine compounds in pharmaceuticals, agrochemicals, and materials science17–21 have encouraged the development of new methods for the generation of C–F bonds.22–25 Thus, it will be an important topic to incorporate both the fluorine atom and sulfenyl group. Indeed, incorporation of fluorine and sulfur atoms simultaneously into alkenes has been developed by many groups. Saluzzo and coworkers26,27 reported a direct fluoromethane sulfenylation of alkenes using dimethyl(methylthio)sulfonium fluoroborate (DMTSF) and triethylamine tris-hydrofluoride (Scheme 1a). However, this approach is limited to methane sulfenylation and it is relatively difficult to access DMTSF. Correa and co-workers28 reported that the addition of benzenesulfenyl chloride to alkenes in the presence of silver or mercury fluorides resulted in the formation of β-fluoro phenyl thioethers (Scheme 1b). Although this method is efficient, it still has limitations such as requirements of hazardous sulfenyl chlorides and toxic metal reagents.
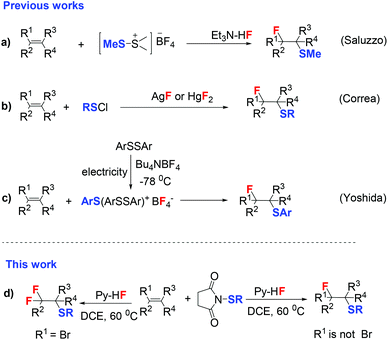 |
| Scheme 1 Literature background for fluorothiolation of alkenes. | |
In 2009, Yoshida and coworkers29 reported an innovative method for fluorothiolation of alkenes using electrochemically generated ArS(ArSSAr)+BF4− (ref. 30) as the fluorothiolated reagent (Scheme 1c). This work reveals that ArS(ArSSAr)+BF4− is firstly generated from electrooxidation of ArSSAr with Bu4NBF4 at −78 °C and then added into alkenes. This method avoids using unstable and hazardous reagents. But limitations still exist, such as the requiring low temperature and only being suitable for the introduction of aromatic sulfide (ArS). Therefore, it is highly desirable to develop new strategies for the synthesis of β-fluorinated thioethers which meet the following criteria: (1) avoid the use of expensive, unstable and hazardous reagents and (2) be suitable for a wide range of substrates.
The electrophilic addition of sulfenyl halides to alkenes to yield β-halo thioethers via a bridged episulfonium ion is a well-established reaction.31 Inspired by this protocol, we speculate that the direct addition of sulfenyl fluorides to alkenes may also occur. However, sulfenyl fluorides are extremely unstable. Thus, we assume that sulfenyl fluorides could form in situ when using electrophilic SR such as N-thiosuccinimides and hydrogen fluoride. In recent years, our group has developed many transition metal-free halogenations of alkynes and alkenes by using environmentally benign nucleophilic halide reagents enabled by hydrogen bond networks.25,32–46 Herein, we present widely applicable and highly efficient fluorothiolation and difluorothiolation of alkenes using N-thiosuccinimides and Py-HF under mild conditions with good functional group tolerance (Scheme 1d).
We started the investigation of a fluorothiolation protocol using N-thiosuccinimide 2a and 1-decene 1a as model substrates (Table 1). Firstly, screening of the fluoride source showed that pyridine-HF (Py-HF, 70% HF content) afforded the desired product 3a in 71% yield (Table 1, entry 6). No product was observed using other fluoride sources such as KF, CsF, TBAF, and even Et3N-HF or DMPU-HF (Table 1, entries 1–5). Further screening of solvents revealed that this transformation could hardly proceed in polar solvents such as DMF, THF, and MeCN (Table 1, entries 9–11). On the other hand, the reaction proceeded well in non-polar solvents (Table 1, entries 6–8). The yield of the desired product 3a could be significantly improved by increasing the reaction temperature to 60 °C (Table 1, entry 12). Finally, for the complete transformation of alkenes into the desired product, five equivalents of Py-HF were needed, and the desired product 3a was obtained in 99% yield (Table 1, entry 14).
Table 1 Optimization of the reaction conditions

|
Entrya |
[F] |
Solvent |
Temp. (°C) |
Yieldb (%) |
Reaction conditions: 1a (0.1 mmol), 2a (0.12 mmol), [F] (0.3 mmol), and solvent (1 mL) under air for 12 h at room temperature.
Determined by GC-MS.
0.5 mmol Py-HF was used.
|
1 |
KF |
HFIP |
rt |
0 |
2 |
TBAF |
DCM |
rt |
0 |
3 |
CsF |
DCM |
rt |
0 |
4 |
Et3N-HF |
DCM |
rt |
0 |
5 |
DMPU-HF |
DCM |
rt |
0 |
6 |
Py-HF |
DCM |
rt |
71 |
7 |
Py-HF |
DCE |
rt |
80 |
8 |
Py-HF |
Tol |
rt |
60 |
9 |
Py-HF |
THF |
rt |
13 |
10 |
Py-HF |
MeCN |
rt |
22 |
11 |
Py-HF |
DMF |
rt |
0 |
12 |
Py-HF |
DCE |
60 |
90 |
13 |
Py-HF |
DCE |
80 |
88 |
14
|
Py-HF
|
DCE
|
60
|
99
|
With the optimized conditions in hand, we next turned our attention to explore the substrate scope of alkenes (Table 2). Aliphatic terminal alkenes bearing halogen, benzyl, and naphthalene (Table 2, 3b, 3d, and 3e) gave good isolated yields (76%–83%). Internal alkenes such as cyclohexene, cyclooctene, and 4-octylene were also suitable substrates, affording the desired products in good yields from 84% to 90% (Table 2, 3f–3h). According to Yoshida's work29 and the 1H NMR analysis of products, this transformation proceeded through anti-addition of RS and F to alkenes. As a result, Z-alkenes gave trans-β-fluorinated thioethers, while E-alkenes gave cis-β-fluorinated thioethers.
Table 2 Scope for fluorothiolation of alkenesa
Reaction conditions: 1 (0.1 mmol), 2 (0.12 mmol), Py-HF (0.5 mmol), and DCE (1 mL) under air for 12 h at 60 °C.
Isolated yields.
|
|
Then, aliphatic terminal alkenes containing various functional groups were investigated. Aliphatic alkenes bearing esters (OBz) and sulfonyl esters (OTs and OMs) were all well tolerated (Table 2, 3m–3o). Besides, excellent yields could be obtained for aliphatic alkenes bearing heterocyclic moieties such as thiophene, furan, and the derivative of lipoic acid (Table 2, 3i–3k). Also, aliphatic alkenes containing cyano, azide, and amide afforded the corresponding products with moderate to good yields (Table 2, 3p–3r). Notably, ethers sensitive to acids also tolerated these conditions (Table 2, 3s–3v). Similarly, the reaction yields were not affected by functional groups such as nitro, aldehyde, methoxyl, and cyano on substrates. Furthermore, this excellent functional group tolerance enabled potential applications in the synthesis of biologically active natural products, pharmaceuticals, and agrochemicals. For example, this protocol could be successfully applied to the derivatives of adamantanic acid (Table 2, 3w), zaltoprofen (Table 2, 3z), estrone (Table 2, 3x), and glycoside (Table 2, 3y).
After the examination of the alkene scope, we started to explore the scope of N-thiosuccinimides. To our delight, the introduction of electron-donating groups into the aromatic rings of N-arylsulfenylsuccinimides had little influence on this reaction. Also, substitution patterns (ortho, meta, or para) have little effect on the reactivity (Table 2, 3aa–3d and 3af–3ah). However, the presence of electron-withdrawing groups such as the acetyl group led to a significant decrease in the isolated yield (Table 2, 3ae). Besides, this reaction could be extended to N-alkylsulfenylsuccinimides (Table 2, 3ai–3aj), affording the desired products with moderate yields. After exploring the scope of aliphatic alkenes, we started to investigate the scope of aromatic alkenes. Under the optimized conditions, low yields were obtained although the starting materials were completely consumed. This may be due to the fact that aromatic alkenes tend to polymerize under acidic conditions.
The introduction of the difluoromethyl moiety may improve the pharmacokinetic properties of drugs and a large number of difluoromethylated compounds show unique biological activities.47–49 Compared to the incorporation of the fluorine atom23,50–58 and trifluoromethyl group,59–68 difluoromethylations are more challenging.69–73 Direct74–80 and indirect81–86 difluoromethylations are the two main strategies.87–89 We found that β-bromo-β-fluoroalkyl phenyl thioethers could be obtained after the fluorothiolation of α-bromostyrenes. And a tandem nucleophilic displacement of the bromide leaving group by excess hydrofluoride afforded difluorothiolated products. Along with this line, we explored the difluorothiolation of α-bromostyrenes. Under the optimized conditions, α-bromostyrene was used to examine the scope of N-thiosuccinimides and moderate isolated yields could be obtained (Table 3, 4a–4h). However, no product was observed for the electron-withdrawing group on the phenyl ring of N-arylsulfenylsuccinimide. N-Alkylsulfenylsuccinimides afforded the desired products with a relatively low yield (Table 3, 4i).
Table 3 Difluorothiolation of α-bromostyrenesa
Reaction conditions: 1 (0.1 mmol), 2 (0.12 mmol), Py-HF (0.5 mmol), and DCE (1 mL) under air for 12 h at 60 °C.
Isolated yields.
|
|
Encouraged by the success of fluorothiolation of alkenes, we next focused on bromo- and chlorothiolation of alkenes. Instead of pyridine-HF, pyridine-HCl and pyridine-HBr were used as the halogen source. Similarly, good yields (Scheme 2) could also be obtained under the optimized conditions.
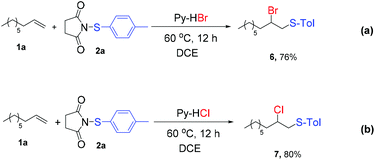 |
| Scheme 2 Bromo- and chlorothiolation of alkenes. | |
Based on the previous studies,90,91 a plausible reaction pathway for the intermolecular fluorothiolation of alkenes was proposed, as shown in Scheme 3. Firstly, an active intermediate A may be formed when Py-HF and N-thiosuccinimide were mixed under the optimized conditions. Then, sulfonium B may be produced from intermediate A with alkene 1, followed by a nucleophilic attack of the fluorine anion, giving the corresponding fluorothiolated products. When R1 was bromine, due to the high reactivity of benzyl bromide, difluoromethylated products could be obtained through the nucleophilic displacement of the bromo substituent by excess hydrogen fluoride.
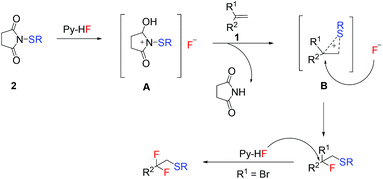 |
| Scheme 3 Plausible mechanism. | |
Conclusions
In summary, we have developed practical and efficient fluorothiolation and difluorothiolation of alkenes using Py-HF and N-thiosuccinimides under simple and mild conditions. This metal-free system offers good chemical yields and good functional group tolerance.
Conflicts of interest
There are no conflicts to declare.
Acknowledgements
We are grateful to the National Science Foundation of China for financial support (NSFC-21672035).
Notes and references
- M. Wang and X. Jiang, Sulfur–Sulfur Bond Construction, Top. Curr. Chem., 2018, 376, 14 CrossRef PubMed.
- M. Fontecave, S. Ollagnier-de-Choudens and E. Mulliez, Biological Radical Sulfur Insertion Reactions, Chem. Rev., 2003, 103, 2149–2166 CrossRef CAS PubMed.
- S. Oida, Y. Tajima, T. Konosu, Y. Nakamura, A. Somada, T. Tanaka, S. Habuki, T. Harasaki, Y. Kamai, T. Fukuoka, S. Ohya and H. Yasuda, Synthesis and Antifungal Activities of R-102557 and Related Dioxane-Triazole Derivatives, Chem. Pharm. Bull., 2000, 48, 694–707 CrossRef CAS PubMed.
- N. Wang, P. Saidhareddy and X. Jiang, Construction of sulfur-containing moieties in the total synthesis of natural products, Nat. Prod. Rep., 2019 10.1039/C8NP00093J.
- E. A. Ilardi, E. Vitaku and J. T. Njardarson, Data-Mining for Sulfur and Fluorine: An Evaluation of Pharmaceuticals To Reveal Opportunities for Drug Design and Discovery, J. Med. Chem., 2014, 57, 2832–2842 CrossRef CAS PubMed.
- F. Minghao, T. Bingqing, H. L. Steven and J. Xuefeng, Sulfur Containing Scaffolds in Drugs: Synthesis and Application in Medicinal Chemistry, Curr. Top. Med. Chem., 2016, 16, 1200–1216 CrossRef PubMed.
- C. Jacob, E. Battaglia, T. Burkholz, D. Peng, D. Bagrel and M. Montenarh, Control of Oxidative Posttranslational Cysteine Modifications: From Intricate Chemistry to Widespread Biological and Medical Applications, Chem. Res. Toxicol., 2012, 25, 588–604 Search PubMed.
- J. E. Casida, Unexpected Metabolic Reactions and Secondary Targets of Pesticide Action, J. Agric. Food Chem., 2016, 64, 4471–4477 CrossRef CAS PubMed.
- J. E. Casida and K. A. Durkin, Pesticide Chemical Research in Toxicology: Lessons from Nature, Chem. Res. Toxicol., 2017, 30, 94–104 Search PubMed.
- A. A. Constantinescu, K. Caliskan, O. C. Manintveld, R. van Domburg, L. Jewbali and A. H. M. M. Balk, Weaning from inotropic support and concomitant beta-blocker therapy in severely ill heart failure patients: take the time in order to improve prognosis, Eur. J. Heart Failure, 2014, 16, 435–443 CrossRef CAS PubMed.
- A. M. Giannetti, H. Wong, G. J. P. Dijkgraaf, E. C. Dueber, D. F. Ortwine, B. J. Bravo, S. E. Gould, E. G. Plise, B. L. Lum, V. Malhi and R. A. Graham, Identification, Characterization, and Implications of Species-Dependent Plasma Protein Binding for the Oral Hedgehog Pathway Inhibitor Vismodegib (GDC-0449), J. Med. Chem., 2011, 54, 2592–2601 CrossRef CAS PubMed.
- D. Huang, J. Chen, W. Dan, J. Ding, M. Liu and H. Wu, A Metal-Free Sulfenylation and Bromosulfenylation of Indoles: Controllable Synthesis of 3-Arylthioindoles and 2-Bromo-3-arylthioindoles, Adv. Synth. Catal., 2012, 354, 2123–2128 CrossRef CAS.
- C. J. Nalbandian, E. M. Miller, S. T. Toenjes and J. L. Gustafson, A conjugate Lewis base-Brønsted acid catalyst for the sulfenylation of nitrogen containing heterocycles under mild conditions, Chem. Commun., 2017, 53, 1494–1497 RSC.
- D. Zhu, Y. Gu, L. Lu and Q. Shen, N-Difluoromethylthiophthalimide: A Shelf-Stable, Electrophilic Reagent for Difluoromethylthiolation, J. Am. Chem. Soc., 2015, 137, 10547–10553 CrossRef CAS PubMed.
- S. Song, Y. Zhang, A. Yeerlan, B. Zhu, J. Liu and N. Jiao, Cs2CO3-Catalyzed Aerobic Oxidative Cross-Dehydrogenative Coupling of Thiols with Phosphonates and Arenes, Angew. Chem., Int. Ed., 2017, 56, 2487–2491 CrossRef CAS PubMed.
- S. Vásquez-Céspedes, A. Ferry, L. Candish and F. Glorius, Heterogeneously Catalyzed Direct C–H Thiolation of Heteroarenes, Angew. Chem., Int. Ed., 2015, 54, 5772–5776 CrossRef PubMed.
- Y. Zhou, J. Wang, Z. Gu, S. Wang, W. Zhu, J. L. Aceña, V. A. Soloshonok, K. Izawa and H. Liu, Next Generation of Fluorine-Containing Pharmaceuticals, Compounds Currently in Phase II–III Clinical Trials of Major Pharmaceutical Companies: New Structural Trends and Therapeutic Areas, Chem. Rev., 2016, 116, 422–518 CrossRef CAS PubMed.
- W. Zhu, J. Wang, S. Wang, Z. Gu, J. L. Aceña, K. Izawa, H. Liu and V. A. Soloshonok, Recent advances in the trifluoromethylation methodology and new CF3-containing drugs, J. Fluorine Chem., 2014, 167, 37–54 CrossRef CAS.
- Y. Zhu, J. Han, J. Wang, N. Shibata, M. Sodeoka, V. A. Soloshonok, J. A. S. Coelho and F. D. Toste, Modern Approaches for Asymmetric Construction of Carbon–Fluorine Quaternary Stereogenic Centers: Synthetic Challenges and Pharmaceutical Needs, Chem. Rev., 2018, 118, 3887–3964 CrossRef CAS PubMed.
- H. Mei, J. Han, S. Fustero, M. Medio-Simon, D. M. Sedgwick, C. Santi, R. Ruzziconi and V. A. Soloshonok, Fluorine-Containing Drugs Approved by the FDA in 2018, Chem. – Eur. J., 2019, 25, 11797–11819 CrossRef CAS PubMed.
- H. Mei, J. Han, K. D. Klika, K. Izawa, T. Sato, N. A. Meanwell and V. A. Soloshonok, Applications of fluorine-containing amino acids for drug design, Eur. J. Med. Chem., 2019, 111826, DOI:10.1016/j.ejmech.2019.111826.
- Z. Yuan, H.-Y. Wang, X. Mu, P. Chen, Y.-L. Guo and G. Liu, Highly Selective Pd-Catalyzed Intermolecular Fluorosulfonylation of Styrenes, J. Am. Chem. Soc., 2015, 137, 2468–2471 CrossRef CAS.
- H. Chen, Z. Liu, Y. Lv, X. Tan, H. Shen, H.-Z. Yu and C. Li, Selective Radical Fluorination of Tertiary Alkyl Halides at Room Temperature, Angew. Chem., Int. Ed., 2017, 56, 15411–15415 CrossRef CAS PubMed.
- X. Bertrand and J.-F. Paquin, Direct Hydrofluorination of Methallyl Alkenes Using a Methanesulfonic Acid/Triethylamine Trihydrofluoride Combination, Org. Lett., 2019 DOI:10.1021/acs.orglett.9b03950.
- Z. Lu, X. Zeng, G. B. Hammond and B. Xu, Widely Applicable Hydrofluorination of Alkenes via Bifunctional Activation of Hydrogen Fluoride, J. Am. Chem. Soc., 2017, 139, 18202–18205 CrossRef CAS PubMed.
- G. Haufe, G. Alvernhe, D. Anker, A. Laurent and C. Saluzzo, Formal addition of methanesulfenyl fluoride to unsaturated substrates, Tetrahedron Lett., 1988, 29, 2311–2314 CrossRef CAS.
- G. Haufe, G. Alvernhe, D. Anker, A. Laurent and C. Saluzzo, Synthesis of fluoroalkyl methyl thioethers by formal addition of methanesulfenyl fluoride to alkenes, J. Org. Chem., 1992, 57, 714–719 CrossRef CAS.
- S. T. Purrington and I. D. Correa, The preparation of .beta.-fluoro thioethers, J. Org. Chem., 1986, 51, 1080–1083 CrossRef CAS.
- S. Fujie, K. Matsumoto, S. Suga and J.-I. Yoshida, Thiofluorination of Carbon–Carbon Multiple Bonds Using Electrochemically Generated ArS(ArSSAr)+BF4−, Chem. Lett., 2009, 38, 1186–1187 CrossRef CAS.
- A. S. Gybin, W. A. Smit, V. S. Bogdanov, M. Z. Krimer and J. B. Kalyan, Aryl-bis(thioaryl)sulfonium salts and their use for the preparation of S-aryl-episulfonium salts, Tetrahedron Lett., 1980, 21, 383–386 CrossRef.
- V. A. Smit, N. S. Zefirov, I. V. Bodrikov and M. Z. Krimer, Episulfonium ions: myth and reality, Acc. Chem. Res., 1979, 12, 282–288 CrossRef CAS.
- X. Zeng, J. Li, C. K. Ng, G. B. Hammond and B. Xu, (Radio)fluoroclick Reaction Enabled by a Hydrogen-Bonding Cluster, Angew. Chem., Int. Ed., 2018, 57, 2924–2928 CrossRef CAS PubMed.
- M. R. Uehling, R. P. Rucker and G. Lalic, Catalytic Anti-Markovnikov Hydrobromination
of Alkynes, J. Am. Chem. Soc., 2014, 136, 8799–8803 CrossRef CAS PubMed.
- T. Xu, C. W. Cheung and X. Hu, Iron-Catalyzed 1,2-Addition of Perfluoroalkyl Iodides to Alkynes and Alkenes, Angew. Chem., Int. Ed., 2014, 53, 4910–4914 CrossRef CAS PubMed.
- B. E. Mann, P. M. Bailey and P. M. Maitlis, Definitive example of cis chloropalladation. Structure of chloro(2,5-dithiahexane){1-(1,4-di-tert-butyl-4-chloro)butadienyl}palladium, J. Am. Chem. Soc., 1975, 97, 1275–1276 CrossRef CAS.
- Z. Lu, W. Kong, Z. Yuan, X. Zhao and G. Zhu, Synthesis of Multisubstituted Enamides via Pd-Catalyzed Chloroallylation of Ynamides, J. Org. Chem., 2011, 76, 8524–8529 CrossRef CAS PubMed.
- K. Kokubo, K. Matsumasa, M. Miura and M. Nomura, Rhodium-Catalyzed Reaction of Aroyl Chlorides with Alkynes, J. Org. Chem., 1996, 61, 6941–6946 CrossRef CAS PubMed.
- X.-Y. Lu, G.-X. Zhu and S.-M. Ma, A novel regio- and stereo-specific hydrohalogenation reaction of 2-alkynoic acids and their derivatives, Chin. J. Chem., 1993, 11, 267–271 CrossRef CAS.
- W. Li, L. Gao, Z. Yue and J. Zhang, Phosphine-Catalyzed Regioselective and Stereoselective Hydrohalogenation Reaction of 2-(1-Alkynyl)-2-alken-1-ones: Synthesis of 2-Halo-1,3-dienes, Adv. Synth. Catal., 2015, 357, 2651–2655 CrossRef CAS.
- J. A. Mulder, K. C. M. Kurtz, R. P. Hsung, H. Coverdale, M. O. Frederick, L. Shen and C. A. Zificsak, Highly Stereoselective Synthesis of Novel α-Haloenamides via a Mild and Efficient Hydrohalogenation of Ynamides, Org. Lett., 2003, 5, 1547–1550 CrossRef CAS PubMed.
- K. Ohashi, S. Mihara, A. H. Sato, M. Ide and T. Iwasawa, Synthesis of 1-haloethenamides from ynamide through halotrimethylsilane-mediated hydrohalogenation, Tetrahedron Lett., 2014, 55, 632–635 CrossRef CAS.
- Z. Li, R. Ebule, J. Kostyo, G. B. Hammond and B. Xu, HBr–DMPU: The First Aprotic Organic Solution of Hydrogen Bromide, Chem. – Eur. J., 2017, 23, 12739–12743 CrossRef CAS.
- S. Liang, R. Ebule, G. B. Hammond and B. Xu, A Chlorinating Reagent Yields Vinyl Chlorides with High Regioselectivity under Heterogeneous Gold Catalysis, Org. Lett., 2017, 19, 4524–4527 CrossRef CAS PubMed.
- X. Zeng, S. Liu, G. B. Hammond and B. Xu, Hydrogen-Bonding-Assisted Brønsted Acid and Gold Catalysis: Access to Both (E)- and (Z)-1,2-Haloalkenes via Hydrochlorination of Haloalkynes, ACS Catal., 2018, 8, 904–909 CrossRef CAS PubMed.
- X. Zeng, Z. Lu, S. Liu, G. B. Hammond and B. Xu, Metal-free, Regio-, and Stereo-Controlled Hydrochlorination and Hydrobromination of Ynones and Ynamides, J. Org. Chem., 2017, 82, 13179–13187 CrossRef CAS PubMed.
- B. Chen, X. Xia, X. Zeng and B. Xu, Hydrogen bonding network assisted regio- and stereo- controlled hydrohalogenations of sulfonyl alkynes, Tetrahedron Lett., 2018, 59, 3950–3954 CrossRef CAS.
- A. M. Silva, R. E. Cachau, H. L. Sham and J. W. Erickson, Inhibition and catalytic mechanism of HIV-1 aspartic protease, J. Mol. Biol., 1996, 255, 321–340 CrossRef CAS PubMed.
- K. Müller, C. Faeh and F. Diederich, Fluorine in Pharmaceuticals: Looking Beyond Intuition, Science, 2007, 317, 1881–1886 CrossRef PubMed.
- J. Wang, M. Sánchez-Roselló, J. L. Aceña, C. del Pozo, A. E. Sorochinsky, S. Fustero, V. A. Soloshonok and H. Liu, Fluorine in Pharmaceutical Industry: Fluorine-Containing Drugs Introduced to the Market in the Last Decade (2001–2011), Chem. Rev., 2014, 114, 2432–2506 CrossRef CAS PubMed.
- J.-W. Lee, M. T. Oliveira, H. B. Jang, S. Lee, D. Y. Chi, D. W. Kim and C. E. Song, Hydrogen-bond promoted nucleophilic fluorination: concept, mechanism and applications in positron emission tomography, Chem. Soc. Rev., 2016, 45, 4638–4650 RSC.
- Z. Qiu, Y. Quan and Z. Xie, Palladium-Catalyzed Selective Fluorination of o-Carboranes, J. Am. Chem. Soc., 2013, 135, 12192–12195 CrossRef CAS PubMed.
- T. Furuya, A. E. Strom and T. Ritter, Silver-Mediated Fluorination of Functionalized Aryl Stannanes, J. Am. Chem. Soc., 2009, 131, 1662–1663 CrossRef CAS PubMed.
- M.-G. Braun and A. G. Doyle, Palladium-Catalyzed Allylic C–H Fluorination, J. Am. Chem. Soc., 2013, 135, 12990–12993 CrossRef CAS.
- T. D. Beeson and D. W. C. MacMillan, Enantioselective Organocatalytic α-Fluorination of Aldehydes, J. Am. Chem. Soc., 2005, 127, 8826–8828 CrossRef CAS.
- X. Ma, M. Diane, G. Ralph, C. Chen and M. R. Biscoe, Stereospecific Electrophilic Fluorination of Alkylcarbastannatrane Reagents, Angew. Chem., Int. Ed., 2017, 56, 12663–12667 CrossRef CAS PubMed.
- S. Arimitsu, T. Yonamine and M. Higashi, Cinchona-Based Primary Amine Catalyzed a Proximal Functionalization of Dienamines: Asymmetric α-Fluorination of α-Branched Enals, ACS Catal., 2017, 7, 4736–4740 CrossRef CAS.
- J. C. Mixdorf, A. M. Sorlin, Q. Zhang and H. M. Nguyen, Asymmetric Synthesis of Allylic Fluorides via Fluorination of Racemic Allylic Trichloroacetimidates Catalyzed by a Chiral Diene-Iridium Complex, ACS Catal., 2018, 8, 790–801 CrossRef CAS.
- G. Tarantino and C. Hammond, Catalytic Formation of C(sp3)–F Bonds via Heterogeneous Photocatalysis, ACS Catal., 2018, 8, 10321–10330 CrossRef CAS.
- H. Liu, Z. Gu and X. Jiang, Direct Trifluoromethylation of the C–H Bond, Adv. Synth. Catal., 2013, 355, 617–626 CrossRef CAS.
- E. Mejía and A. Togni, Rhenium-Catalyzed Trifluoromethylation of Arenes and Heteroarenes by Hypervalent Iodine Reagents, ACS Catal., 2012, 2, 521–527 CrossRef.
- X. Gao, Y.-L. Xiao, X. Wan and X. Zhang, Copper-Catalyzed Highly Stereoselective Trifluoromethylation and Difluoroalkylation of Secondary Propargyl Sulfonates, Angew. Chem., Int. Ed., 2018, 57, 3187–3191 CrossRef CAS PubMed.
- P. Gao, X.-R. Song, X.-Y. Liu and Y.-M. Liang, Recent Developments in the Trifluoromethylation of Alkynes, Chem. – Eur. J., 2015, 21, 7648–7661 CrossRef CAS PubMed.
- Y. Ouyang, X.-H. Xu and F.-L. Qing, Trifluoromethanesulfonic Anhydride as a Low-Cost and Versatile Trifluoromethylation Reagent, Angew. Chem., Int. Ed., 2018, 57, 6926–6929 CrossRef CAS PubMed.
- S. Guo, D. I. AbuSalim and S. P. Cook, 1,2-(Bis)trifluoromethylation of Alkynes: A One-Step Reaction to Install an Underutilized Functional Group, Angew. Chem., Int. Ed., 2019, 58, 11704–11708 CrossRef CAS.
- J.-A. Ma and D. Cahard, Asymmetric Fluorination, Trifluoromethylation, and Perfluoroalkylation Reactions, Chem. Rev., 2004, 104, 6119–6146 CrossRef CAS PubMed.
- J. Charpentier, N. Früh and A. Togni, Electrophilic Trifluoromethylation by Use of Hypervalent Iodine Reagents, Chem. Rev., 2015, 115, 650–682 CrossRef CAS PubMed.
- X. Yang, T. Wu, R. J. Phipps and F. D. Toste, Advances in Catalytic Enantioselective Fluorination, Mono-, Di-, and Trifluoromethylation, and Trifluoromethylthiolation Reactions, Chem. Rev., 2015, 115, 826–870 CrossRef CAS PubMed.
- X.-H. Xu, K. Matsuzaki and N. Shibata, Synthetic Methods for Compounds Having CF3–S Units on Carbon by Trifluoromethylation, Trifluoromethylthiolation, Triflylation, and Related Reactions, Chem. Rev., 2015, 115, 731–764 CrossRef CAS PubMed.
- V. Bacauanu, S. Cardinal, M. Yamauchi, M. Kondo, D. F. Fernández, R. Remy and D. W. C. MacMillan, Metallaphotoredox Difluoromethylation of Aryl Bromides, Angew. Chem., Int. Ed., 2018, 57, 12543–12548 CrossRef CAS PubMed.
- D. E. Yerien, S. Barata-Vallejo and A. Postigo, Difluoromethylation Reactions of Organic Compounds, Chem. – Eur. J., 2017, 23, 14676–14701 CrossRef CAS PubMed.
- H.-H. Xu, J. Song and H.-C. Xu, Electrochemical Difluoromethylation of Electron-Deficient Alkenes, ChemSusChem, 2019, 12, 3060–3063 CrossRef CAS PubMed.
- T. T. Tung, S. B. Christensen and J. Nielsen, Difluoroacetic Acid as a New Reagent for Direct C–H Difluoromethylation of Heteroaromatic Compounds, Chem. – Eur. J., 2017, 23, 18125–18128 CrossRef CAS PubMed.
- Q. Xie, Z. Zhu, L. Li, C. Ni and J. Hu, A General Protocol for C–H Difluoromethylation of Carbon Acids with TMSCF2Br, Angew. Chem., Int. Ed., 2019, 58, 6405–6410 CrossRef CAS PubMed.
- L. Xu and D. A. Vicic, Direct Difluoromethylation of Aryl Halides via Base Metal Catalysis at Room Temperature, J. Am. Chem. Soc., 2016, 138, 2536–2539 CrossRef CAS PubMed.
- P. S. Fier and J. F. Hartwig, Copper-Mediated Difluoromethylation of Aryl and Vinyl Iodides, J. Am. Chem. Soc., 2012, 134, 5524–5527 CrossRef CAS PubMed.
- G. K. S. Prakash, S. K. Ganesh, J.-P. Jones, A. Kulkarni, K. Masood, J. K. Swabeck and G. A. Olah, Copper-Mediated Difluoromethylation of (Hetero)aryl Iodides and β-Styryl Halides with Tributyl(difluoromethyl)stannane, Angew. Chem., Int. Ed., 2012, 51, 12090–12094 CrossRef CAS PubMed.
- J. Zhu, Y. Liu and Q. Shen, Direct Difluoromethylation of Alcohols with an Electrophilic Difluoromethylated Sulfonium Ylide, Angew. Chem., Int. Ed., 2016, 55, 9050–9054 CrossRef CAS PubMed.
- Q.-Y. Lin, X.-H. Xu, K. Zhang and F.-L. Qing, Visible-Light-Induced Hydrodifluoromethylation of Alkenes with a Bromodifluoromethylphosphonium Bromide, Angew. Chem., Int. Ed., 2016, 55, 1479–1483 CrossRef CAS PubMed.
- D. Chen, C. Ni, Y. Zhao, X. Cai, X. Li, P. Xiao and J. Hu, Bis(difluoromethyl)trimethylsilicate Anion: A Key Intermediate in Nucleophilic Difluoromethylation of Enolizable Ketones with Me3SiCF2H, Angew. Chem., Int. Ed., 2016, 55, 12632–12636 CrossRef CAS PubMed.
- T. Iida, R. Hashimoto, K. Aikawa, S. Ito and K. Mikami, Umpolung of Fluoroform by C–F Bond Activation: Direct Difluoromethylation of Lithium Enolates, Angew. Chem., Int. Ed., 2012, 51, 9535–9538 CrossRef CAS PubMed.
- Y.-M. Su, Y. Hou, F. Yin, Y.-M. Xu, Y. Li, X. Zheng and X.-S. Wang, Visible Light-Mediated C–H Difluoromethylation of Electron-Rich Heteroarenes, Org. Lett., 2014, 16, 2958–2961 CrossRef CAS PubMed.
- V. V. Levin, A. L. Trifonov, A. A. Zemtsov, M. I. Struchkova, D. E. Arkhipov and A. D. Dilman, Difluoromethylene Phosphabetaine as an Equivalent of Difluoromethyl Carbanion, Org. Lett., 2014, 16, 6256–6259 CrossRef CAS PubMed.
- M.-C. Belhomme, T. Poisson and X. Pannecoucke, Copper-Catalyzed Direct C-2 Difluoromethylation of Furans and Benzofurans: Access to C-2 CF2H Derivatives, J. Org. Chem., 2014, 79, 7205–7211 CrossRef CAS PubMed.
- Z. He, M. Hu, T. Luo, L. Li and J. Hu, Copper-Catalyzed Difluoromethylation of β,γ-Unsaturated Carboxylic Acids: An Efficient Allylic Difluoromethylation, Angew. Chem., Int. Ed., 2012, 51, 11545–11547 CrossRef CAS PubMed.
- X. Shen, W. Zhang, C. Ni, Y. Gu and J. Hu, Tuning the Reactivity of Difluoromethyl Sulfoximines from Electrophilic to Nucleophilic: Stereoselective Nucleophilic Difluoromethylation of Aryl Ketones, J. Am. Chem. Soc., 2012, 134, 16999–17002 CrossRef CAS PubMed.
- K. Fujikawa, Y. Fujioka, A. Kobayashi and H. Amii, A New Method for Aromatic Difluoromethylation: Copper-Catalyzed Cross-Coupling and Decarboxylation Sequence from Aryl Iodides, Org. Lett., 2011, 13, 5560–5563 CrossRef CAS PubMed.
- F. Leroux, L. Garamszegi and M. Schlosser, A simple method for the displacement of bromine by fluorine at tertiary, benzylic or non-classical secondary sites, J. Fluorine Chem., 2002, 117, 177–180 CrossRef CAS.
- P. S. Bhadury, S. K. Raza and D. K. Jaiswal, A semi-molten mixture of hexadecyltributylphosphonium bromide and potassium fluoride in the synthesis of organofluorine compounds, J. Fluorine Chem., 1999, 99, 115–117 CrossRef CAS.
- D. Guijarro and M. Yus, Generation of allylic and benzylic organolithium compounds by fluorine–lithium exchange: reaction with electrophiles, J. Organomet. Chem., 2001, 624, 53–57 CrossRef CAS.
- X. Li, Y. Guo and Z. Shen, Hydrochloric Acid-Promoted Intermolecular 1,2-Thiofunctionalization of Aromatic Alkenes, J. Org. Chem., 2018, 83, 2818–2829 CrossRef CAS PubMed.
- S. E. Denmark, D. J. P. Kornfilt and T. Vogler, Catalytic Asymmetric Thiofunctionalization of Unactivated Alkenes, J. Am. Chem. Soc., 2011, 133, 15308–15311 CrossRef CAS PubMed.
Footnote |
† Electronic supplementary information (ESI) available. See DOI: 10.1039/c9qo01228a |
|
This journal is © the Partner Organisations 2020 |