DOI:
10.1039/C9QO01229J
(Research Article)
Org. Chem. Front., 2020,
7, 126-130
Rhodium-catalyzed homodimerization–cyclization reaction of two vinyl isocyanides: a general route to 2-(isoquinolin-1-yl)oxazoles†
Received
8th October 2019
, Accepted 21st November 2019
First published on 22nd November 2019
Abstract
A novel rhodium-catalyzed homodimerization–cyclization reaction of two vinyl isocyanides has been developed for the first time. In this reaction, the highly reactive 1,4-diazabutatriene intermediates generated by the homodimerization of two vinyl isocyanides could undergo a novel type of intramolecular tandem cyclization and provides a new and highly efficient method for the construction of 2-(isoquinolin-1-yl)oxazoles by formation of three new bonds and two rings from readily available acyclic starting materials in a single step.
Isocyanides are useful building blocks in organic synthesis because of their unique modes of reaction.1 Accordingly, significant advances have been made in isocyanide-based multicomponent reactions,2 [3 + 2]-cycloaddition of activated methylene isocyanides with polar multiple bonds,3 transition metal-catalyzed isocyanide insertion4 and radical insertions.5 In stark contrast, reports on coupling-cyclization of two isocyanides are rare.6–10 In this context, the coupling-cyclization of two isocyanides using 1,4-diazabutatriene species generated in situ from the dimerization of isocyanides as key intermediates has emerged as an efficient protocol for the synthesis of fused heterocyclic compounds.7–10 In 1999, Beckert provided evidence for the existence of 1,4-diazabutatriene by reduction of bis-imidoyl chlorides of oxalic acid.7 Subsequently, the groups of Cheng and Kobayashi independently reported the related coupling-cyclization reactions of 2-pyridylisonitriles or o-isocyanostyrenes.8 Recently, Xu's and Li's groups developed a new cross-cyclization from nonsymmetrical 1,4-diazabutatriene generated by the heterodimerization of two different isocyanides,9 respectively. However, all of the above reactions were limited to functionalized aryl isocyanides.7–9 To the best of our knowledge, so far only one example of a homodimerization–cyclization reaction based on 1,4-diazabutatriene generated by the dimerization of nonaromatic isocyanides was described. The pioneering work by Lange and Höfle reported a homodimerization–cyclization reaction of two imidoyl isocyanides and 2,2′-diphenyl-4,4′-biquinazoline in 38% yield (Scheme 1, eqn (1)).10 Thus, the development of new coupling-cyclization of nonaromatic isocyanides, especially vinyl isocyanides, remains a challenging task. As a continuation of our studies on isocyanide chemistry,11 herein, we report a rhodium-catalyzed coupling-cyclization based on the homodimerization of two vinyl isocyanides (Scheme 1, eqn (2)). In this reaction, the highly reactive 1,4-diazabutatriene intermediate generated by the dimerization of two vinyl isocyanides undergoes a novel type of intramolecular tandem cyclization to furnish 2-(isoquinolin-1-yl)oxazoles in good to high yields.
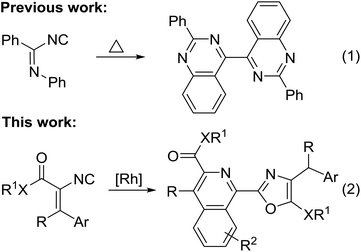 |
| Scheme 1 Homodimerization–cyclization reaction of two imidoyl isocyanides or vinyl isocyanides. | |
The isoquinoline skeleton is a privileged structure that is commonly found in numerous synthetic and natural products.12 Similar to the isoquinoline skeleton, the oxazole unit is a ubiquitous heterocyclic scaffold found in many bioactive natural products and pharmaceutical drugs.13 As a consequence, the combination of these two structural features within a single framework giving novel oxazole-isoquinolines has potential for biological and pharmacological activities. To date, only one method has been reported for the synthesis of 2-(isoquinolin-1-yl)oxazoles based on the cycloaddition of isoquinoline Reissert salts with acetylenic aldehydes.14 Our reaction provides a new and highly efficient approach for the construction of 2-(isoquinolin-1-yl)oxazoles via a rhodium-catalyzed cascade cyclization strategy by formation of three new bonds and two rings from readily available acyclic starting materials in a single step (Scheme 1, eqn (2)).
Initially, the tandem cyclization reaction of vinyl isocyanide 1a was investigated to optimize the reaction conditions. When 1a (0.2 mmol) was heated at 160 °C in toluene for 12 h, the 2-(isoquinolin-1-yl)oxazole 2a was obtained in 22% yield (Table 1, entry 1). The yield of 2a was increased to 74% in the presence of [(C6H5)3P]3RhCl (1 mol%) under otherwise identical conditions as mentioned above (entry 4). Decreasing the reaction temperature led to comparatively lower yields of 2a (entries 2 and 3). Further increasing the amount of [(C6H5)3P]3RhCl did not improve the yield of 2a (entry 5). Other catalysts, such as [Cp*RhCl2]2, [Rh(COD)Cl]2, [Rh(nbd)Cl]2 and ReCl3 were less effective (entries 6–9). Among the solvents tested, toluene seemed to be the best choice. Other solvents, such as o-xylene, MeCN and DMF gave lower product yields (entries 10, 11 and 13). No desired product 2a was observed when the reaction was performed in DMSO (entry 12).
Table 1 Optimization of the reaction conditionsa
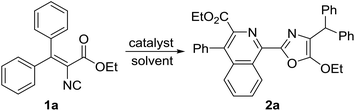
|
Entry |
[M] (mol%) |
Temp. |
Solvent |
t/h |
Yieldb (%) |
Reaction conditions: 1a (0.2 mmol), catalyst (0.002–0.01 mmol), at 100–160 °C in solvent for 12–18 h in a sealed tube. [Cp*RhCl2]2 = bis[(pentamethylcyclopentadienyl)dichloro-rhodium], [Rh(COD)Cl]2 = chloro(1,5-cyclooctadiene)rhodium(I) dimer, [Rh(nbd)Cl]2 = bicyclo[2.2.1]hepta-2,5-diene-rhodium(I) chloride dimer.
Estimated by 1H NMR spectroscopy using dimethyl phthalate as an internal standard.
Isolated yield.
|
1 |
— |
160 |
Toluene |
12 |
22 |
2 |
[(C6H5)3P]3RhCl (1) |
100 |
Toluene |
18 |
49 |
3 |
[(C6H5)3P]3RhCl (1) |
130 |
Toluene |
12 |
58 |
4 |
[(C6H5)3P]3RhCl (1) |
160 |
Toluene |
12 |
74c |
5 |
[(C6H5)3P]3RhCl (5) |
160 |
Toluene |
12 |
73 |
6 |
[Cp*RhCl2]2 (1) |
160 |
Toluene |
12 |
32 |
7 |
[Rh(COD)Cl]2 (1) |
160 |
Toluene |
12 |
48 |
8 |
[Rh(nbd)Cl]2 (1) |
160 |
Toluene |
12 |
48 |
9 |
ReCl3 (1) |
160 |
Toluene |
12 |
22 |
10 |
[(C6H5)3P]3RhCl (1) |
160 |
o-Xylene |
12 |
54 |
11 |
[(C6H5)3P]3RhCl (1) |
160 |
MeCN |
12 |
16 |
12 |
[(C6H5)3P]3RhCl (1) |
160 |
DMSO |
12 |
— |
13 |
[(C6H5)3P]3RhCl (1) |
160 |
DMF |
12 |
16 |
With the optimized reaction conditions in hand, the scope of the reaction was examined and the results are summarized in Table 2. The tandem reaction showed broad tolerance for vinyl isocyanides. Various diaryl ketone-derived vinyl isocyanides 1a–g undergo efficient dimerization–cyclization reactions to give the corresponding 2-(isoquinolin-1-yl)oxazoles 2a–g in good to high yields. In addition, the electronic properties of aromatic rings can affect this transformation. No reaction was observed when vinyl isocyanides containing strong electron-withdrawing substituents on the aromatic rings, such as ethyl 3,3-bis(4-cyanophenyl)-2-isocyanoacrylate, ethyl 3,3-bis(4-methoxycarbonylphenyl)-2-isocyanoacrylate and ethyl 2-isocyano-3,3-bis(4-nitrophenyl)acrylate, were employed as partners under optimal conditions. It is noteworthy that the dimerization–cyclization reaction of substrates 1f and 1g with differently substituted aromatic rings also proceeded smoothly, resulting in an expected mixture of 2-(isoquinolin-1-yl)oxazoles 2f/2f′ and 2g/2g′ with moderate regioselectivities, which could not be separated by silica gel column chromatography. Furthermore, the regioselectivity of this reaction mainly depends on the electronic properties of the substituents on both aromatic rings. Similarly, aliphatic aryl ketone-derived vinyl isocyanides 1h and 1i were suitable for this reaction, generating the desired 2-(isoquinolin-1-yl)oxazoles 2h and 2i in 75% and 64% yields, respectively. More importantly, in the case of aryl and heteroaryl aldehyde-derived vinyl isocyanides 1j–o, the dimerization–cyclization reaction also worked well, affording the desired products 2j–o in moderate to high yields.15 In addition, methyl ester- or amide-based vinyl isocyanides 1p and 1q also smoothly underwent this transformation, affording the corresponding products 2p and 2q in 72% and 69% yields, respectively (Table 2). In addition, we found that the dimerization–cyclization reaction is easy to scale-up. A scale-up reaction of 1d (4.0 mmol, 1.22 g) was carried out for 12 h under otherwise identical conditions as mentioned above, furnishing 1.49 g of the desired product 2d in 61% yield.
Table 2 Rhodium-catalyzed homodimerization–cyclization of vinyl isocyanides 1a,b
Reaction conditions: 1 (0.2 mmol), [(C6H5)3P]3RhCl (0.002 mmol), at 160 °C in toluene for 12–16 h in a sealed tube.
Isolated yield.
The ratio was determined by the 1H NMR spectroscopy of the crude reaction mixtures.
|
|
To test the synthetic utility of the present protocol, the intramolecular cyclization of 2 was further investigated. As shown in Scheme 2, the hydrolysis of 2a or 2d (0.5 mmol) in the presence of NaOH (1.0 mmol) in refluxing C2H5OH–H2O (10 mL, v/v = 1/1) provided the 1-(oxazol-2-yl)isoquinoline-3-carboxylic acids 3a and 3d in 99% and 98% yields (Scheme 2), respectively. In addition, we found that the intramolecular cyclization reactions of 3a and 3d proceeded smoothly to provide 7H-indeno[2,1-c]isoquinolin-7-ones 4a and 4d in 57% and 53% yields, respectively, via a sequential acyl chlorination and intramolecular Friedel–Crafts acylation reaction in the presence of SOCl2 (1.0 eq.) and AlCl3 (1.0 eq.) in dichloromethane (DCM) at room temperature for 12 h (Scheme 2), respectively. Undoubtedly, the above reaction provides a novel and efficient method for the synthesis of 7H-indeno[2,1-c]isoquinolin-7-ones under mild conditions.16
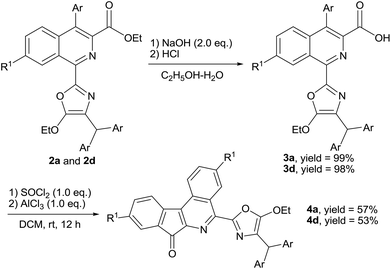 |
| Scheme 2 Potential synthetic applications of 2. | |
To further probe the mechanisms for the formation of 2, some control experiments were performed (Scheme 3). When the dimerization–cyclization reaction of 1a was carried out under an atmosphere of nitrogen under otherwise identical conditions, the desired product 2a was produced in 70% yield (Scheme 3, eqn (1)). Similarly, product 2a was obtained in 68% yield when 2,6-di-tert-butyl-4-methylphenol (BHT, 1.0 eq.) was used as a radical inhibitor under the optimized conditions (Scheme 3, eqn (2)). These results imply that a radical process is not involved.
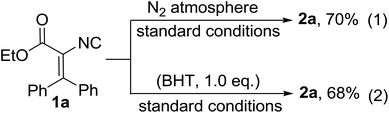 |
| Scheme 3 Control experiments for mechanistic studies. | |
On the basis of the above experimental results together with related reports,7–10,11c,17 a possible mechanism of this homodimerization–cyclization is proposed in Scheme 4. Initially, the coordination of [(C6H5)3P]3RhCl with vinyl isonitrile 1 generates intermediate A,11c which undergoes migratory insertion to produce 1,4-diazabutatriene intermediate B. Subsequently, intermediate B undergoes efficient 6π electrocyclization to afford intermediate C, followed by a 1,3-proton shift to give intermediate D. Likely activated by rhodium, the carbonyl oxygen atom of intermediate D attacks the carbon atom of the carbon–nitrogen double bond to form intermediate E.17 During this process, the rhodium catalyst dissociates to complete the catalytic cycle. Finally, intermediate E undergoes isomerization, followed by protonation to produce the corresponding 2-(isoquinolin-1-yl)oxazoles 2 (Scheme 4).
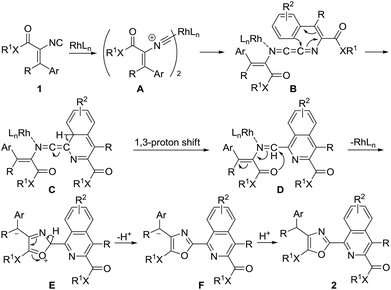 |
| Scheme 4 Proposed mechanisms for the formation of 2. | |
In addition, the fluorescence properties of compound 2a in the solid state under the excitation of ultraviolet light were investigated. We found that powder 2a exhibits blue fluorescence at λmax 440 nm (Fig. 1), which implies that this compound 2a may be a promising candidate in the field of optoelectronic materials.18
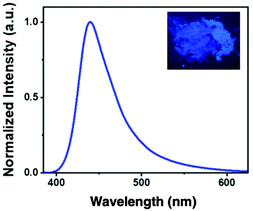 |
| Fig. 1 Normalized emission spectra of compound 2a. | |
Conclusions
In conclusion, we have developed a novel rhodium-catalyzed homodimerization–cyclization reaction of two vinyl isocyanides. Importantly, this reaction provides a simple and highly efficient method for the construction of 2-(isoquinolin-1-yl)oxazoles from readily available acyclic starting materials in a single step. This new strategy allows the formation of three new bonds and two rings via a sequential intermolecular coupling and intramolecular tandem cyclization. This reaction features readily available substrates, wide substrate scope, good functional group tolerance, and high efficiency. Further studies on other cascade cyclizations based on isocyanides are currently underway.
Experimental section
General procedure for the preparation of 2 (2a as an example)
To a solution of ethyl 2-isocyano-3,3-diphenylacrylate 1a (0.2 mmol, 55.4 mg) in toluene (2.0 mL) was added [(C6H5)3P]3RhCl (1.8 mg, 0.002 mmol). The reaction mixture was stirred for 12 h at 160 °C in a 10 mL sealed tube. After the reaction was complete, the reaction mixture was poured into saturated aqueous NaCl (5.0 mL) and extracted with CH2Cl2 (2.0 mL × 3). The combined organic extracts were dried over anhydrous Mg2SO4, filtered and concentrated under reduced pressure to yield the crude product, which was purified by chromatography (ethyl acetate/petroleum ether = 1/5, V/V) to give 2a (82.1 mg, 74%) as a yellow solid. Rf = 0.45 (ethyl acetate/petroleum ether: 3/10). Mp. 60–61 °C; 1H NMR (600 MHz, CDCl3) δ: 9.58 (d, J = 8.5 Hz, 1H), 7.72–7.69 (m, 1H), 7.67–7.64 (m, 2H), 7.49 (d, J = 7.2 Hz, 3H), 7.41 (d, J = 7.8 Hz, 4H), 7.36–7.35 (m, 2H), 7.32 (t, J = 7.7 Hz, 4H), 7.23 (t, J = 7.3 Hz, 2H), 5.47 (s, 1H), 4.27 (q, J = 7.1 Hz, 2H), 4.14 (q, J = 7.1 Hz, 2H), 1.30 (t, J = 7.1 Hz, 3H), 0.99 (t, J = 7.1 Hz, 3H); 13C NMR (151 MHz, CDCl3) δ: 166.95, 155.86, 150.09, 144.27, 142.72, 141.63, 136.64, 136.08, 134.13, 130.73, 129.87, 129.41, 129.00, 128.29, 128.22, 128.09, 127.94, 126.55, 126.46, 126.38, 120.34, 70.29, 61.37, 47.22, 15.02, 13.69; HRMS (ESI-TOF): [M + Na]+ calculated for C36H30N2NaO4+: 577.2098, found: 577.2104.
Conflicts of interest
The authors declare no competing financial interest.
Acknowledgements
Financial support of this research from the National Natural Sciences Foundation of China (21871044 and 21472017) and the Natural Sciences Foundation of Jilin Province (20190201073JC) is greatly acknowledged.
Notes and references
- For recent reviews, see:
(a)
Isocyanide Chemistry Applications in Synthesis and Materials Science, ed. V. Nenajdenko, Wiley-VCH, Weinheim, 2012 Search PubMed;
(b) A. V. Lygin and A. D. Meijere, Angew. Chem., Int. Ed., 2010, 49, 9094 CrossRef CAS PubMed;
(c) S. Chakrabarty, S. Choudhary, A. Doshi, F.-Q. Liu, R. Mohan, M. P. Ravindra, D. Shah, X. Yang and F. F. Fleming, Adv. Synth. Catal., 2014, 356, 2315 CrossRef.
-
(a)
J. Zhu and H. Bienaymé, Multicomponent Reactions, Wiley-VCH, Weinheim, 2005 CrossRef;
(b) A. Dömling, Chem. Rev., 2006, 106, 17 CrossRef PubMed;
(c)
L. Banfi and R. Riva, in Organic Reactions, ed. A. B. Charette, Wiley, New York, 2005, vol. 65, p. 1 Search PubMed;
(d) A. Dömling and I. Ugi, Angew. Chem., Int. Ed., 2000, 39, 3168 CrossRef.
-
(a) D. V. Leusen and A. M. V. Leusen, Org. React., 2001, 57, 417 Search PubMed;
(b) A. V. Gulevich, A. G. Zhdanko, R. V. A. Orru and V. G. Nenajdenko, Chem. Rev., 2010, 110, 5235 CrossRef CAS.
- For reviews, see:
(a) V. P. Boyarskiy, N. A. Bokach, K. V. Luzyanin and V. Y. Kukushkin, Chem. Rev., 2015, 115, 2698 CrossRef CAS;
(b) G. Qiu, Q. Ding and J. Wu, Chem. Soc. Rev., 2013, 42, 5257 RSC;
(c) S. Lang, Chem. Soc. Rev., 2013, 42, 4867 RSC;
(d) T. Vlaar, E. Ruijter, B. U. W. Maes and R. V. A. Orru, Angew. Chem., Int. Ed., 2013, 52, 7084 CrossRef CAS.
- For a review, see: B. Zhang and S. Armido, Chem. Soc. Rev., 2015, 44, 3505 RSC.
-
(a) Z. Hou, J. Dong, Y. Men, Z. Lin, J. Cai and X. Xu, Angew. Chem., Int. Ed., 2017, 56, 1805 CrossRef PubMed;
(b) C. Kanazawa, S. Kamijo and Y. Yamamoto, J. Am. Chem. Soc., 2006, 128, 10662 CrossRef CAS PubMed;
(c) B. Pooi, J. Lee, K. Choi, H. Hirao and S. H. Hong, J. Org. Chem., 2014, 79, 9231 CrossRef CAS;
(d) M.-A. Bonin, D. GiguHre and R. Roy, Tetrahedron, 2007, 63, 4912 CrossRef CAS;
(e) R. Grigg, M. I. Lansdell and M. Thornton-Pett, Tetrahedron, 1999, 55, 2025 CrossRef CAS.
- M. Wenzel, F. Lehmann, R. Beckert, W. Günther and H. Gürls, Monatsh. Chem., 1999, 130, 1373 CAS.
-
(a) N. Shao, G.-X. Pang, X.-R. Wang, R.-J. Wu and Y. Cheng, Tetrahedron, 2010, 66, 7302 CrossRef CAS;
(b) K. Kobayashi, J. Yonemori, A. Matsunaga, T. Kitamura, M. Tanmatsu, O. Morikawa and H. Konishi, Heterocycles, 2001, 55, 33 CrossRef CAS.
-
(a) Z. Hu, H. Yuan, Y. Men, Q. Liu, J. Zhang and X. Xu, Angew. Chem., Int. Ed., 2016, 55, 7077 CrossRef CAS;
(b) S. Su, J. Hu, Y. Cui, C. Tang, Y. Chen and J. Li, Chem. Commun., 2019, 55, 12243 RSC.
- G. Höfle and B. Lange, Angew. Chem., Int. Ed. Engl., 1977, 16, 727 CrossRef.
- For selected recent examples, see:
(a) L. Zhang, T. Liu, Y. M. Wang, J. Chen and Y.-L. Zhao, Org. Lett., 2019, 21, 2973 CrossRef CAS PubMed;
(b) Y. Yu, Y. Zhang, Z. Wang, Y.-X. Liang and Y.-L. Zhao, Org. Chem. Front., 2019, 6, 3657 RSC;
(c) X.-B. Bu, Z.-X. Zhang, Q.-Q. Peng, X. Xu and Y.-L. Zhao, J. Org. Chem., 2019, 84, 53 CrossRef CAS PubMed;
(d) X.-B. Bu, Z. Wang, X.-D. Wang, X.-H. Meng and Y.-L. Zhao, Adv. Synth. Catal., 2018, 360, 2945 CrossRef CAS;
(e) X.-B. Bu, Y. Yu, B. Li, L. Zhang, J.-J. Chen and Y.-L. Zhao, Adv. Synth. Catal., 2017, 359, 351 CrossRef CAS;
(f) X.-B. Bu, Z. Wang, Y.-H. Wang, T. Jiang, L. Zhang and Y.-L. Zhao, Eur. J. Org. Chem., 2017, 1132 CrossRef CAS.
- For selected reviews, see:
(a) F. Pan, C. Shu and L.-W. Ye, Org. Biomol. Chem., 2016, 14, 9456 RSC;
(b) M. Giustiniano, A. Basso, V. Mercalli, A. Massarotti, E. Novellino, G. C. Tron and J. Zhu, Chem. Soc. Rev., 2017, 46, 1295 RSC;
(c) B. Zhang and A. Studer, Chem. Soc. Rev., 2015, 44, 3505 RSC;
(d) L. Ackermann, Acc. Chem. Res., 2014, 47, 281 CrossRef CAS PubMed;
(e) A. Zhang, J. L. Neumeyer and R. J. Baldessarini, Chem. Rev., 2006, 107, 274 CrossRef PubMed;
(f) P. G. Baraldi, M. A. Tabrizi, S. Gessi and P. A. Borea, Chem. Rev., 2008, 108, 238 CrossRef CAS;
(g) K. W. Bentley, Nat. Prod. Rep., 2006, 23, 444 RSC;
(h) F. Dzierszinski, A. Coppin, M. Mortuaire, E. Dewailly, C. Slomianny, J.-C. Ameisen, F. DeBels and S. Tomavo, Antimicrob. Agents Chemother., 2002, 46, 3197 CrossRef CAS.
- For selected reviews, see:
(a) A. Ibrar, I. Khan, N. Abbas, U. Farooq and A. Khan, RSC Adv., 2016, 6, 93016 RSC;
(b) A. V. Gulevich, A. S. Dudnik, N. Chernyak and V. Gevorgyan, Chem. Rev., 2013, 113, 3084 CrossRef CAS PubMed;
(c)
D. C. Palmer and E. C. Taylor, The Chemistry of Heterocyclic Compounds Oxazoles: Synthesis, Reactions, and Spectroscopy, Wiley, 2004, vol. 60 Search PubMed;
(d) Y. Hu, X. Xin and B. Wan, Tetrahedron Lett., 2015, 56, 32 CrossRef CAS;
(e) A. S. K. Hashmi, Chem. Rev., 2007, 107, 3180 CrossRef CAS;
(f) P. Wipf, Chem. Rev., 1995, 95, 2115 CrossRef CAS;
(g) I. J. Turchi and M. J. S. Dewarb, Chem. Rev., 1975, 75, 389 CrossRef CAS.
- A. W. Bridge, M. B. Hursthouse, C. W. Lehmann, D. J. Lythgoe and C. G. Newton, J. Chem. Soc., Perkin Trans., 1993, 1, 1839 RSC.
- CCDC 1887535† (2k) contains the supplementary crystallographic data in the Cambridge Crystallographic Data Centre.
-
(a) A. E. Wendlandt and S. S. Stahl, J. Am. Chem. Soc., 2014, 136, 11910 CrossRef CAS;
(b) Z. Shi and F. Glorius, Chem. Sci., 2013, 4, 829 RSC.
-
(a) D. Bonne, M. Dekhane and J. Zhu, Angew. Chem., Int. Ed., 2007, 46, 2485 CrossRef CAS PubMed;
(b) J. Wang, S. Luo, J. Huang, T. Mao and Q. Zhu, Chem. – Eur. J., 2014, 20, 11220 CrossRef CAS PubMed.
- For selected reviews, see:
(a) O. Ostroverkhova, Chem. Rev., 2016, 116, 13279 CrossRef CAS PubMed;
(b) C. Wang, H. Dong, W. Hu, Y. Liu and D. Zhu, Chem. Rev., 2018, 118, 3447 CrossRef;
(c) W. Wu, R. Tang, Q. Li and Z. Li, Chem. Soc. Rev., 2015, 44, 3997 RSC;
(d) Z. Yang, Z. Mao, Z. Xie, Y. Zhang, S. Liu, J. Zhao, J. Xu, Z. Chi and M. P. Aldred, Chem. Soc. Rev., 2017, 46, 915 RSC.
Footnote |
† Electronic supplementary information (ESI) available: Experimental details and spectral data of 2. CCDC 1887535. For ESI and crystallographic data in CIF or other electronic format see DOI: 10.1039/c9qo01229j |
|
This journal is © the Partner Organisations 2020 |