Synthesis of substituted 2-alkylquinolines by visible-light photoredox catalysis†
Received
18th October 2019
, Accepted 23rd November 2019
First published on 26th November 2019
Abstract
The condensation of anilines and alkenyl ethers has been demonstrated by employing visible-light photoredox catalysis. The resulting method enables the synthesis of substituted 2-alkylquinolines under mild and simple conditions with good substrate scope and high yields.
Introduction
The synthesis of quinoline derivatives has been extensively developed for more than 100 years, because of their “privileged” structures that are widely featured in many natural products,1 important intermediates for asymmetric synthesis2 and pharmaceuticals.3 2-Alkylquinolines, for example, are associated with a wide range of biomedical properties, such as antibacterial, antitumor, and antimalarial, and are used as anti-HIV agents.4 As a consequence of their proven bioactivity, the synthesis of substituted quinolines has been a subject of great focus in medical and organic chemistry over the years. Traditionally, there are two distinctive methods to prepare quinoline derivatives, one is the classic transformation under metal-free conditions, such as the Skraup reaction, Doebner–Von Miller reaction, Friedländer reaction, Conrad–Limpach reaction and so on.5 The other is the transition-metal-catalyzed reaction, with metal catalysts including rhodium,6 ruthenium,7 palladium,8 cobalt,9 nickel10 and other metal complexes.11 Cheng and co-workers reported the nickel-catalyzed cyclization of 2-iodoanilines with alkynyl aryl ketones to give 2,4-disubstituted quinolones, which mainly can be employed for the synthesis of 2-aryl-4-substituted quinolines.10 In 2016, Jiang and co-workers reported a palladium-catalyzed aerobic oxidative approach to afford 2-arylquinolines from allylbenzenes with aniline.8a These reactions can be employed for the synthesis of various quinoline skeletons, but only a few of them are for the construction of 2-alkylquinolines. Generically, 2-alkylquinolines are synthesized from functionalized anilines and unsaturated carbonyl compounds or olefins. Alkenyl ethers in the synthesis of 2-alkylquinolines have limited applications and, to the best of our knowledge, there are only four related literature studies.12 New synthesis routes to 2-alkylquinolines in one step under mild conditions are still desired and would have major impacts on the availability of 2-alkylquinolines.
Visible-light-initiated organic reactions have demonstrated their synthetic utility and attracted growing interest over the past decade. By activation with visible light, a photocatalyst can convert the photoenergy into chemical energy to activate a range of organic substrates via single-electron transfer (SET).13 A variety of organic transformations through a single-electron-transfer process have been elegantly carried out under irradiation of visible light with the assistance of photocatalysts. Molecular oxygen, an ideal, clean and inexpensive source, is often used in many visible light mediated reactions, one of the reasons is that it can act as oxidases14 or oxygenases15 and afford active superoxide radical anions. As part of our ongoing project on the study of molecular oxygen as oxidases under visible light conditions,16 we describe herein a new route to construct 2-alkylquinoline frameworks from anilines and alkenyl ethers using 2,4,6-triphenylpyranium tetrafluoride (TPT) as the photocatalyst and oxygen in air as oxidases under blue LED irradiation at room temperature (Scheme 1).
 |
| Scheme 1 Synthetic strategies for the synthesis of 2-alkylquinolines from anilines and alkenyl ethers. | |
Results and discussion
Initially, we examined the model reaction of methyl 4-amino-3-methylbenzoate (1a) with ethoxyethene (2a) in DMF by using 2,4,6-triphenylpyryliumfluoroborate (TPT, 4 mol%) as the photoredox catalyst at room temperature under irradiation of 3 W blue LED (450–455 nm) in air for 16 h. It was pleasing to find that the reaction does indeed proceed and afford the desired product 3a in 61% yield (Table 1, entry 1). While in the absence of TPT, no reaction was observed (Table 1, entry 2). The structure of 3a was characterized by using HRMS and NMR spectra. Inspired by this result, various solvents were screened. No desired product was observed while the reactions were carried out in EtOH and THF (Table 1, entries 3 and 4). Gratifyingly, an improved yield (88%) of 3a was obtained when the reaction was conducted in CH3CN (Table 1, entry 5). Other solvents including CHCl3, 1,2-dichloroethane (DCE), 1,4-dioxane and PhCl gave inferior results and led to the formation of 3a in 14–45% yields (Table 1, entries 6–9). However, Ru(bpy)3Cl2, fac-Ir(ppy)3 and organic dyes (including methylene blue, Mes-Acr+ClO4−, rose bengal, and eosin Y) as photocatalysts exhibited no efficiency to the model reaction (Table 1, entries 10–15). The ratio of 1a to 2a, as well as the reaction time was optimized, and the results are presented in Table 1 (entries 16 and 17). When air was replaced by O2 and N2, 87% and 0% of the desired product were formed respectively (Table 1, entry 18), suggesting that O2 in air is essential for the reaction.
Table 1 Optimization of the reaction conditionsa

|
Entry |
Photocatalyst |
Solvent |
Yieldb (%) |
Reaction conditions: Methyl 4-amino-3-methylbenzoate (1a, 0.20 mmol), ethoxyethene (2a, 0.60 mmol), and the photocatalyst (0.008 mmol, 4 mol%) in the solvent (2.0 mL) at room temperature in air under 3 W blue LED (450–455 nm) for 16 h.
Isolated yield.
2a (1.0 equiv.).
2a (2.0 equiv.).
2a (4.0 equiv.).
6 h.
24 h.
Oxygen balloon instead of air.
Under N2.
|
1 |
TPT |
DMF |
61 |
2 |
— |
DMF |
0 |
3 |
TPT |
EtOH |
0 |
4 |
TPT |
THF |
0 |
5
|
TPT
|
CH
3
CN
|
88
|
6 |
TPT |
CHCl3 |
40 |
7 |
TPT |
DCE |
45 |
8 |
TPT |
1,4-Dioxane |
14 |
9 |
TPT |
PhCl |
35 |
10 |
Ru(bpy)3Cl2 |
CH3CN |
0 |
11 |
fac-Ir(ppy)3 |
CH3CN |
0 |
12 |
Methylene blue |
CH3CN |
0 |
13 |
Mes-Acr+ClO4− |
CH3CN |
0 |
14 |
Rose bengal |
CH3CN |
0 |
15 |
Eosin Y |
CH3CN |
0 |
16 |
TPT |
CH3CN |
42c, 61d, 89e |
17 |
TPT |
CH3CN |
56f, 85g |
18 |
TPT |
CH3CN |
87h,0i |
With the optimized conditions in hand, we next tested the versatility of this system for the construction of functionalized alkyl quinolines. Various o-toluidines and several other alkenyl ethers were investigated and the results are summarized in Scheme 2. This reaction was amenable to a wide range of o-toluidines. Their derivatives containing electron-withdrawing groups, such as Cl, Br, I, COOEt, CN and NO2 at the para-position of o-toluidine rings, generated the corresponding 6-substituted-2,8-dimethylquinolines in yields ranging from 57% to 81% (Scheme 2, 3b–3g). The electron-donating groups (p-chlorophenyl, p-trifluoromethylphenyl, m-methoxyphenyl and Me at the para-position of o-toluidine rings) could also be tolerated, giving the desired products (Scheme 2, 3h–3k) in 52–71% yields. Notably, o-toluidines with electron-withdrawing groups on the benzene rings generated higher yields of the products than those having benzene rings with electron-donating groups due to their electronic effect. After investigating the para-position of o-toluidine rings, the meta-position of the o-toluidine rings was further tested under the optimal conditions. 3-Substituted and 5-substituted o-toluidines did not affect the efficiency of the reaction, affording the desired products (Scheme 2, 3l–3x) in moderate yields. The position of the substituents on the o-toluidine rings has no significant influence on their reactivity. We next evaluated the feasibility of various alkenyl ethers in this transformation. It was found that terminal olefins, such as, 2-methyl-1-(vinyloxy)propane, 2-methyl-2-(vinyloxy)propane, 1-(vinyloxy)butane and 1-(vinyloxy)propane all gave the same product 3a with moderate yields. It was gratifying to find that non-terminal olefins including (E)-1-ethoxyprop-1-ene and (E)-1-ethoxybut-1-ene could also react with diverse 4-substituted o-toluidines to give the corresponding 6-substituted-2,8-dimethylquinolines (Scheme 2, 3ab–3ec) in 61–76% yields. However, 4-ethoxy-2-methyl-1,2,3,4-tetrahydroquinolines (Scheme 3, 4a and 4b) were obtained in 24% and 39% yields when the reaction of aniline and p-chloroaniline with ethoxyethene (2a) was carried out under standard conditions. It has been shown that the ortho-methyl substitution of aniline is necessary. Surprisingly, when the ortho-methyl was replaced with ortho-trifluoromethyl, ortho-ethyl and ortho-methoxyl, the corresponding 4-ethoxy-2-methyl-1,2,3,4-tetrahydroquinolines (Scheme 3, 4c–4d) were also obtained in 32–50% yields. Until now, the role of the ortho-methyl group is unclear, and more studies to identify the role of the o-methyl are currently underway in our laboratory.
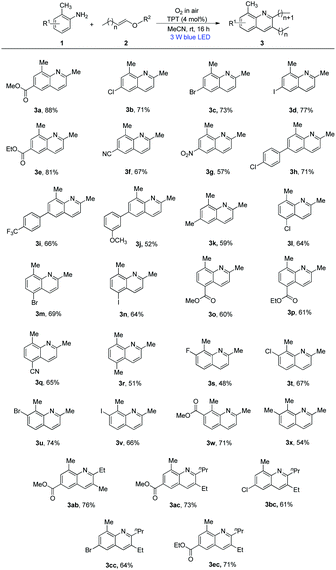 |
| Scheme 2 The scope of substrates [reaction conditions: 1 (0.20 mmol), 2 (0.60 mmol), TPT (0.008 mmol, 4 mol%) in CH3CN (2.0 mL) at room temperature in air under 3 W blue LED (450–455 nm) for 16 h; isolated yield of the product after column chromatography]. | |
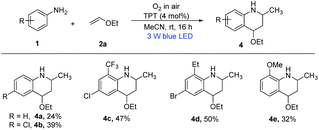 |
| Scheme 3 The scope of substrates [reaction conditions: 1 (0.20 mmol), 2a (0.60 mmol), TPT (0.008 mmol, 4 mol%) in CH3CN (2.0 mL) at room temperature in air under 3 W blue LED (450–455 nm) for 16 h; isolated yield of the product after column chromatography]. | |
To further investigate the reaction mechanism of this transformation, some control experiments were performed, shown in Scheme 4. When a known radical scavenger, such as 2,2,6,6-tetramethyl-1-piperidinyloxy (TEMPO, 1.5 equiv.), was added to the reaction of methyl 4-amino-3-methylbenzoate (1a) with ethoxyethene (2a) under standard conditions, the reaction was inhibited completely. When a radical scavenger, 1,1-diphenylethylene was added to the reaction of 1a and 2a, intermediate IV was surprisingly stabilized by 1,1-diphenylethylene and compound 5 was observed by HRMS analysis (see the ESI† for details). These results suggested that a radical process was possibly involved in the reaction. Additionally, in order to understand the role of oxygen and the species of oxygen involved in the present reaction, 5,5-dimethyl-pyrroline-N-oxide (DMPO) was employed to capture O2˙− and the resulting ESR spectra are presented in Fig. S2 of the ESI.† There was no signal when DMPO was added into a solution of 2a and TPT in air-saturated acetonitrile in the absence of light irradiation (Fig. S2a†). But irradiation of the air-saturated acetonitrile solution of 2a and TPT in air with blue LED (450–455 nm, 3 W) resulted in the formation of a strong characteristic signal of the O2˙− adduct with DMPO (Fig. S2b†). When the reaction time was prolonged, a stronger characteristic signal of O2˙− was observed (Fig. S2c and S2d†), indicating the formation of O2˙− in the reaction. Finally, fluorescence quenching experiments were also carried out to check for an energy transfer process between TPT and ethoxyethene under visible-light irradiation. As shown in ESI,† the emission intensity of excited TPT gradually decreased with the increasing of the concentration of ethoxyethene (2a). The result indicated that the single-electron transfer process actually occurred between TPT and ethoxyethene (2a) under visible-light irradiation.
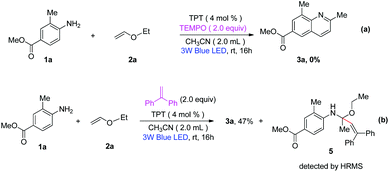 |
| Scheme 4 The control experiment. | |
Although the exact mechanism of the reaction is not clear, the possible mechanistic pathway based on current mechanistic investigations is shown in Scheme 5. Firstly, the photocatalyst (PC, TPT) was excited by the irradiation of blue LED to its excited state PC*, which then undergoes the single electron transfer (SET) process with ethoxyethane to generate the highly electrophilic radical cation I and PC˙−. The generated radical cation I reacts with o-toluidine 1a to generate radical cation II, which undergoes rearrangement to afford radical cation III. Simultaneously, the oxidation of PC˙− by O2 in air regenerated PC for the next run and produced a superoxide radical anion (O2˙−), which was confirmed by electron spin resonance (ESR). The radical cation III was subjected to deprotonation to convert radical IV along with the formation of ˙OOH. Cation V was formed by the oxidation of radical IV by ˙OOH along with the formation of −OOH. Subsequently, cation V attacks another 2a molecule, leading to the formation of intermediate VI, then VII was formed by the electrophilic substitution reaction. Finally, an aromatization process was used to generate the final product 3a by the elimination of ethanol with the assistance of the hydrogen proton.
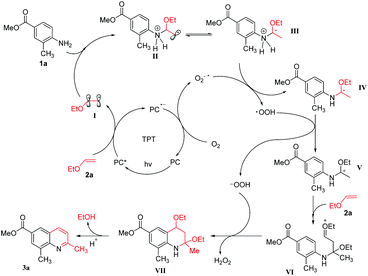 |
| Scheme 5 Plausible mechanism for the reaction. | |
Experimental section
General remarks
All 1H NMR and 13C NMR spectra were recorded on a Bruker FT-NMR spectrometer (400 MHz or 600 MHz and 100 MHz or 150 MHz, respectively). All chemical shifts are given as δ value (ppm) with reference to tetramethylsilane (TMS) as an internal standard. The peak patterns are indicated as follows: s, singlet; d, doublet; t, triplet; m, multiplet; q, quartet. The coupling constants, J, are reported in hertz (Hz). High resolution mass spectroscopy data of the products were collected on Agilent Technologies 6540 UHD Accurate-Mass Q-TOF LC/MS (ESI).
All reactions were setup in air. All chemicals were purchased commercially and used without further purification. Products were purified by flash chromatography on 200–300 mesh silica gels, SiO2.
Typical procedure for the synthesis of substituted 2-alkylquinolines by visible-light photoredox catalysis
A 5 mL oven-dried reaction vessel equipped with a magnetic stirrer was charged with methyl 4-amino-3-methylbenzoate (1a, 0.20 mmol), ethoxyethene (2a, 0.60 mmol), TPT (4 mol%) and acetonitrile (2.0 mL). The reaction vessel was exposed to blue LED (450–455 nm, 3 W) irradiation at room temperature in air with stirring for 16 h. After completion of the reaction, the mixture was concentrated to yield the crude product, which was further purified by flash chromatography (silica gel, petroleum ether/ethyl acetate = 100
:
1) to give the desired product 3a.
Characterization data for the products
Methyl 2,8-dimethylquinoline-6-carboxylate (3a).
White solid. 1H NMR (400 MHz, CDCl3) δ: 8.30 (s, 1H), 8.07 (s, 1H), 8.01 (d, J = 8.4 Hz, 1H), 7.26 (d, J = 8.4 Hz, 1H), 3.95 (s, 3H), 2.79 (s, 3H), 2.74 (s, 3H); 13C NMR (100 MHz, CDCl3) δ: 166.9, 160.3, 148.7, 137.2, 136.9, 128.6, 128.5, 126.4, 125.3, 122.2, 52.1, 25.7, 17.8. HRMS (ESI) [M + H]+ calcd for C13H14NO2: 216.1019, found: 216.1016.
6-Chloro-2,8-dimethylquinoline (3b).
White solid. 1H NMR (400 MHz, CDCl3) δ: 7.88 (d, J = 8.4 Hz, 1H), 7.57–7.56 (m, 1H), 7.46 (s, 1H), 7.26 (d, J = 8.4 Hz, 1H), 2.76 (s, 3H), 2.73 (s, 3H); 13C NMR (100 MHz, CDCl3) δ: 158.1, 145.4, 138.9, 135.3, 130.6, 130.0, 127.0, 124.0, 122.5, 25.5, 17.7. HRMS (ESI) [M + H]+ calcd for C11H11ClN: 192.0575, found: 192.0577.
6-Bromo-2,8-dimethylquinoline (3c).
White solid. 1H NMR (400 MHz, CDCl3) δ: 7.89 (d, J = 8.4 Hz, 1H), 7.75 (s, 1H), 7.60 (s, 1H), 7.28–7.26 (m, 1H), 2.76 (s, 3H), 2.73 (s, 3H); 13C NMR (100 MHz, CDCl3) δ: 158.3, 145.7, 139.1, 135.2, 132.5, 127.5, 127.4, 122.5, 118.9, 25.6, 17.6. HRMS (ESI) [M + H]+ calcd for C11H11BrN: 236.0069, found: 236.0071.
6-Iodo-2,8-dimethylquinoline (3d).
White solid. 1H NMR (400 MHz, CDCl3) δ: 7.97–7.96 (m, 1H), 7.84 (d, J = 8.4 Hz, 1H), 7.76 (s, 1H), 7.24 (d, J = 8.4 Hz, 1H), 2.73 (s, 3H), 2.72 (s, 3H); 13C NMR (100 MHz, CDCl3) δ: 158.5, 146.0, 138.9, 137.7, 134.9, 134.2, 128.0, 122.3, 90.7, 25.6, 17.4. HRMS (ESI) [M + H]+ calcd for C11H11IN: 283.9931, found: 283.9933.
Ethyl 2,8-dimethylquinoline-6-carboxylate (3e).
White solid. 1H NMR (400 MHz, CDCl3) δ: 8.34 (s, 1H), 8.10 (s, 1H), 8.05 (d, J = 8.4 Hz, 1H), 7.29 (d, J = 8.4 Hz, 1H), 4.43 (q, J = 7.2 Hz, 2H), 2.81 (s, 3H), 2.76 (s, 3H), 1.44 (t, J = 7.2 Hz, 3H); 13C NMR (100 MHz, CDCl3) δ: 166.5, 160.2, 148.8, 137.3, 136.9, 128.7, 128.5, 126.9, 125.4, 122.2, 61.0, 25.7, 17.8, 14.3. HRMS (ESI) [M + H]+ calcd for C14H16NO2: 230.1176, found: 230.1178.
2,8-Dimethylquinoline-6-carbonitrile (3f).
White solid. 1H NMR (400 MHz, CDCl3) δ: 8.03 (d, J = 8.4 Hz, 1H), 8.00 (s, 1H), 7.64 (s, 1H), 7.38 (d, J = 8.4 Hz, 1H), 2.79 (s, 3H), 2.78 (s, 3H); 13C NMR (100 MHz, CDCl3) δ: 161.3 148.1, 138.8, 136.4, 131.7, 129.7, 125.5, 123.2, 119.0, 108.8, 25.8, 17.7. HRMS (ESI) [M + H]+ calcd for C12H11N2: 183.0917, found: 183.0915.
2,8-Dimethyl-6-nitroquinoline (3g).
Yellow solid. 1H NMR (400 MHz, CDCl3) δ: 8.57–8.56 (m, 1H), 8.29 (s, 1H), 8.17 (d, J = 8.4 Hz, 1H), 7.42 (d, J = 8.4 Hz, 1H), 2.86 (s, 3H), 2.81 (s, 3H); 13C NMR (100 MHz, CDCl3) δ: 162.0, 149.2, 144.4, 139.4, 137.9, 125.0, 123.5, 122.4, 122.1, 25.9, 18.1. HRMS (ESI) [M + H]+ calcd for C11H11N2O2: 203.0815, found: 203.0815.
6-(4-Chlorophenyl)-2,8-dimethylquinoline (3h).
White solid. 1H NMR (400 MHz, CDCl3) δ: 8.03 (d, J = 8.4 Hz, 1H), 7.75–7.74 (m, 2H), 7.63–7.61 (m, 2H), 7.45–7.42 (m, 2H), 7.29 (d, J = 8.4 Hz, 1H), 2.85 (s, 3H), 2.77 (s, 3H); 13C NMR (100 MHz, CDCl3) δ: 158.2, 146.5, 139.3, 137.2, 136.6, 136.4, 133.5, 129.0, 128.8, 128.5, 126.5, 123.2, 122.2, 25.7, 18.1. HRMS (ESI) [M + H]+ calcd for C17H15ClN: 268.0888, found: 268.0892.
2,8-Dimethyl-6-(4-(trifluoromethyl)phenyl)quinoline (3i).
White solid. 1H NMR (400 MHz, CDCl3) δ: 8.00 (d, J = 8.4 Hz, 1H), 7.75 (s, 2H), 7.73 (s, 2H), 7.70 (s, 1H), 7.67 (s, 1H), 7.27 (d, J = 8.4 Hz, 1H), 2.85 (s, 3H), 2.75 (s, 3H); 13C NMR (100 MHz, CDCl3) δ: 158.5, 146.7, 144.3, 137.4, 136.4, 136.3, 129.4 (q, J = 32.3 Hz), 128.7, 127.5, 126.4, 125.7 (q, J = 3.7 Hz), 125.0 (q, J = 270.0 Hz), 123.8, 122.2, 25.6, 18.1. HRMS (ESI) [M + H]+ calcd for C18H15F3N: 302.1151, found: 302.1154.
6-(3-Methoxyphenyl)-2,8-dimethylquinoline (3j).
White solid. 1H NMR (400 MHz, CDCl3) δ: 8.02 (d, J = 8.4 Hz, 1H), 7.77 (s, 2H), 7.38 (t, J = 7.6 Hz, 1H), 7.29–7.26 (m, 2H), 7.24–7.22 (m, 1H), 6.93–6.90 (m, 1H) 3.88 (s, 3H), 2.85 (s, 3H) 2.76 (s, 3H); 13C NMR (100 MHz, CDCl3) δ: 160.0, 157.9, 146.5, 142.3, 137.7, 136.9, 136.4, 129.8, 129.1, 126.5, 123.3, 122.0, 119.8, 113.1, 112.8, 55.3, 25.6, 18.1. HRMS (ESI) [M + H]+ calcd for C18H18NO: 264.1383, found: 264.1383.
2,6,8-Trimethylquinoline (3k).
White solid. 1H NMR (400 MHz, CDCl3) δ: 7.90 (d, J = 8.4 Hz, 1H), 7.36 (s, 2H), 7.22 (d, J = 8.4 Hz, 1H), 2.76 (s, 3H), 2.73 (s, 3H), 2.46 (s, 3H). 13C NMR (100 MHz, CDCl3) δ: 156.9, 145.6, 136.1, 135.6, 134.8, 131.8, 126.4, 124.3, 121.6, 25.5, 21.4, 17.8. HRMS (ESI) [M + H]+ calcd for C12H14N: 172.1121, found: 172.1123.
5-Chloro-2,8-dimethylquinoline (3l).
White solid. 1H NMR (400 MHz, CDCl3) δ: 8.33 (d, J = 8.8 Hz, 1H), 7.38–7.33 (m, 2H), 7.27 (d, J = 8.8 Hz, 1H), 2.720–2.718 (m, 6H); 13C NMR (100 MHz, CDCl3) δ: 158.4, 147.4, 135.9, 132.8, 128.9, 128.5, 125.1, 124.3, 122.2, 25.3, 17.7. HRMS (ESI) [M + H]+ calcd for C11H11ClN: 192.0575, found: 192.0578.
5-Bromo-2,8-dimethylquinoline (3m).
White solid. 1H NMR (400 MHz, CDCl3) δ: 8.35 (d, J = 8.4 Hz, 1H), 7.60 (d, J = 7.6 Hz, 1H), 7.36–7.32 (m, 2H), 2.76 (s, 3H), 2.73 (s, 3H). 13C NMR (100 MHz, CDCl3) δ: 158.6, 147.6, 136.7, 135.5, 129.6, 128.9, 125.6, 122.7, 119.0, 25.3, 17.8. HRMS (ESI) [M + H]+ calcd for C11H11BrN: 236.0069, found: 236.0068.
5-Iodo-2,8-dimethylquinoline (3n).
White solid. 1H NMR (400 MHz, CDCl3) δ: 8.19 (d, J = 8.4 Hz, 1H), 7.89 (d, J = 7.2 Hz, 1H), 7.29 (d, J = 8.4 Hz, 1H), 7.22 (d, J = 7.6 Hz, 1H), 2.76 (s, 3H), 2.74 (s, 3H); 13C NMR (100 MHz, CDCl3) δ: 158.7, 147.4, 140.3, 137.8, 136.3, 130.5, 128.1, 123.2, 95.1, 25.2, 17.8. HRMS (ESI) [M + H]+ calcd for C11H11IN: 283.9931, found: 283.9930.
Methyl 2,8-dimethylquinoline-5-carboxylate (3o).
White solid. 1H NMR (400 MHz, CDCl3) δ: 9.22 (d, J = 8.8 Hz, 1H), 8.09 (d, J = 7.2 Hz, 1H), 7.52 (d, J = 7.6 Hz, 1H), 7.36 (d, J = 9.2 Hz, 1H), 3.96 (s, 3H), 2.83 (s, 3H), 2.74 (s, 3H); 13C NMR (100 MHz, CDCl3) δ: 167.3, 157.9, 146.8, 143.0, 134.3, 129.6, 128.0, 125.1, 124.2, 123.0, 52.0, 25.4, 18.7. HRMS (ESI) [M + H]+ calcd for C13H14NO2: 216.1019, found: 216.1020.
Ethyl 2,8-dimethylquinoline-5-carboxylate (3p).
White solid. 1H NMR (400 MHz, CDCl3) δ: 9.20 (d, J = 8.8 Hz, 1H), 8.06 (d, J = 7.6 Hz, 1H), 7.46 (d, J = 7.6 Hz, 1H), 7.31 (d, J = 9.2 Hz, 1H), 4.42 (q, J = 7.2 Hz, 2H), 2.80 (s, 3H), 2.71 (s, 3H), 1.42 (t, J = 7.2 Hz, 3H); 13C NMR (100 MHz, CDCl3) δ: 166.7, 157.7, 146.7, 142.7, 134.1, 129.4, 127.9, 125.0, 124.5, 122.7, 60.7, 25.2, 18.6, 14.2. HRMS (ESI) [M + H]+ calcd for C14H16NO2: 230.1176, found: 230.1177.
2,8-Dimethylquinoline-5-carbonitrile (3q).
White solid. 1H NMR (400 MHz, CDCl3) δ: 8.36 (d, J = 8.4 Hz, 1H), 7.78 (d, J = 7.2 Hz, 1H), 7.55 (d, J = 7.6 Hz, 1H), 7.45 (d, J = 8.8 Hz, 1H), 2.84 (s, 3H), 2.79 (s, 3H); 13C NMR (100 MHz, CDCl3) δ: 159.7, 146.2, 143.8, 133.3, 131.6, 128.6, 125.9, 123.8, 117.3, 107.6, 25.5, 18.4. HRMS (ESI) [M + H]+ calcd for C12H11N2: 183.0917, found: 183.0918.
2,6,8-Trimethylquinoline (3r).
White solid. 1H NMR (400 MHz, CDCl3) δ: 8.14 (d, J = 8.8 Hz, 1H), 7.38 (d, J = 7.2 Hz, 1H), 7.25 (d, J = 8.8 Hz, 1H), 7.16 (d, J = 7.2 Hz, 1H), 2.76 (s, 3H), 2.74 (s, 3H), 2.59 (s, 3H); 13C NMR (100 MHz, CDCl3) δ: 157.2, 147.1, 134.4, 132.6, 131.9, 129.0, 125.7, 125.6, 121.0, 25.5, 18.4, 17.9. HRMS (ESI) [M + H]+ calcd for C12H14N: 172.1121, found: 172.1119.
7-Fluoro-2,8-dimethylquinoline (3s).
White solid. 1H NMR (400 MHz, CDCl3) δ: 7.94 (d, J = 8.4 Hz, 1H), 7.56–7.52 (m, 1H), 7.23–7.18 (m, 2H), 2.73 (s, 3H), 2.68 (d, J = 2.4 Hz, 3H); 13C NMR (100 MHz, CDCl3) δ: 160.8 (d, J = 243.4 Hz), 158.8, 147.8 (d, J = 9.8 Hz), 136.1, 126.1 (d, J = 10.4 Hz), 123.3, 120.6 (d, J = 10.0 Hz), 120.5 (d, J = 15.5 Hz), 115.5 (d, J = 26.7 Hz), 25.6, 8.8 (d, J = 4.3 Hz). HRMS (ESI) [M + H]+ calcd for C11H11FN: 176.0870, found: 176.0874.
7-Chloro-2,8-dimethylquinoline (3t).
White solid. 1H NMR (400 MHz, CDCl3) δ: 7.89 (d, J = 8.4 Hz, 1H), 7.42–7.37 (m, 2H), 7.19 (d, J = 8.4 Hz, 1H), 2.84 (s, 3H), 2.71 (s, 3H); 13C NMR (100 MHz, CDCl3) δ: 158.7, 147.3, 136.0, 134.6, 134.2, 126.7, 125.7, 124.8, 121.4, 25.5, 14.2. HRMS (ESI) [M + H]+ calcd for C11H11ClN: 192.0575, found: 192.0576.
7-Bromo-2,8-dimethylquinoline (3u).
White solid. 1H NMR (400 MHz, CDCl3) δ: 7.91 (d, J = 8.4 Hz, 1H), 7.56 (d, J = 8.8 Hz, 1H), 7.40 (d, J = 8.8 Hz, 1H), 7.23 (d, J = 8.4 Hz, 1H), 2.89 (s, 3H), 2.72 (s, 3H); 13C NMR (100 MHz, CDCl3) δ: 158.7, 147.3, 136.4, 136.1, 129.6, 125.9, 125.6, 125.2, 121.6, 25.6, 17.4. HRMS (ESI) [M + H]+ calcd for C11H11BrN: 236.0069, found: 236.0072.
7-Iodo-2,8-dimethylquinoline (3v).
White solid. 1H NMR (400 MHz, CDCl3) δ: 7.92 (d, J = 8.4 Hz, 1H), 7.82 (d, J = 8.8 Hz, 1H), 7.29–7.24 (m, 2H), 2.97 (s, 3H), 2.73 (s, 3H); 13C NMR (100 MHz, CDCl3) δ: 158.5, 146.6, 140.5, 136.1, 135.5, 126.3, 125.8, 121.9, 102.6, 25.6, 23.1. HRMS (ESI) [M + H]+ calcd for C11H11IN: 283.9931, found: 283.9928.
Methyl 2,8-dimethylquinoline-7-carboxylate (3w).
White solid. 1H NMR (400 MHz, CDCl3) δ: 7.96 (d, J = 8.4 Hz, 1H), 7.82 (d, J = 8.4 Hz, 1H), 7.58 (d, J = 8.8 Hz, 1H), 7.29 (d, J = 8.4 Hz, 1H), 3.95 (s, 3H), 3.07 (s, 3H), 2.75 (s, 3H); 13C NMR (100 MHz, CDCl3) δ: 168.7, 158.5, 146.8, 139.7, 135.9, 130.2, 127.7, 125.6, 124.9, 122.9, 52.0, 25.6, 14.5. HRMS (ESI) [M + H]+ calcd for C13H14NO2: 216.1019, found: 216.1018.
2,7,8-Trimethylquinoline (3x).
White solid. 1H NMR (400 MHz, CDCl3) δ: 7.96 (d, J = 8.4 Hz, 1H), 7.51 (d, J = 8.0 Hz, 1H), 7.29 (d, J = 8.0 Hz, 1H), 7.20 (d, J = 8.0 Hz, 1H), 2.76 (s, 3H), 2.75 (s, 3H), 2.50 (s, 3H); 13C NMR (100 MHz, CDCl3) δ: 157.7, 146.9, 137.0, 136.1, 133.8, 128.3, 124.7, 124.4, 120.6, 25.7, 20.7, 13.1. HRMS (ESI) [M + H]+ calcd for C12H14N: 172.1121, found: 172.1119.
Methyl 2-ethyl-3,8-dimethylquinoline-6-carboxylate (3ab).
White solid. 1H NMR (400 MHz, CDCl3) δ: 8.251–8.247 (m, 1H), 8.012–8.010 (m, 1H), 7.77 (s, 1H), 3.95 (s, 3H), 2.95 (q, J = 7.6 Hz, 2H), 2.79 (s, 3H), 2.42 (s, 3H), 1.41 (t, J = 7.2 Hz, 3H); 13C NMR (100 MHz, CDCl3) δ: 167.2, 163.9, 147.4, 137.1, 136.4, 129.9, 127.8, 127.5, 126.4, 126.1, 52.0, 29.3, 18.8, 17.7, 11.7. HRMS (ESI) [M + H]+ calcd for C15H18NO2: 244.1332, found: 244.1333.
Methyl 3-ethyl-8-methyl-2-propylquinoline-6-carboxylate (3ac).
White solid. 1H NMR (400 MHz, CDCl3) δ: 8.33–8.32 (m, 1H), 8.04–8.03 (m, 1H), 7.86 (s, 1H), 3.96 (s, 3H), 2.96 (t, J = 7.2 Hz, 2H), 2.84–2.79 (m, 5H), 1.99–1.90 (m, 2H), 1.33 (t, J = 7.2 Hz, 3H), 1.08 (t, J = 7.2 Hz, 3H); 13C NMR (100 MHz, CDCl3) δ: 167.3, 162.8, 147.3, 137.1, 135.9, 134.7, 128.1, 127.5, 126.5, 126.2, 52.1, 37.5, 25.0, 21.5, 17.7, 14.2, 14.1. HRMS (ESI) [M + H]+ calcd for C17H22NO2: 272.1645, found: 272.1651.
6-Chloro-3-ethyl-8-methyl-2-propylquinoline (3bc).
White solid. 1H NMR (600 MHz, CDCl3) δ: 7.64 (s, 1H), 7.492–7.488 (m, 1H), 7.37–7.36 (m, 1H), 2.90 (t, J = 7.2 Hz, 2H), 2.78–2.75 (m, 2H), 2.73 (s, 3H), 1.94–1.88 (m, 2H), 1.29 (t, J = 7.2 Hz, 3H), 1.06 (t, J = 7.2 Hz, 3H); 13C NMR (150 MHz, CDCl3) δ: 160.4, 143.7, 138.9, 136.0, 132.6, 130.4, 128.7, 127.7, 123.3, 37.3, 25.0, 21.6, 17.6, 14.2, 14.1. HRMS (ESI) [M + H]+ calcd for C15H19ClN: 248.1201, found: 248.1203.
6-Bromo-3-ethyl-8-methyl-2-propylquinoline (3cc).
White solid. 1H NMR (600 MHz, CDCl3) δ: 7.65 (s, 1H), 7.60 (s, 1H), 7.483–7.481 (m, 1H), 2.88 (t, J = 7.8 Hz, 2H), 2.76–2.72 (m, 5H), 1.92–1.88 (m, 2H), 1.27 (t, J = 7.2 Hz, 3H), 1.07–1.04 (m, 3H); 13C NMR (150 MHz, CDCl3) δ: 160.5, 143.9, 139.0, 136.0, 132.4, 131.2, 128.2, 126.7, 118.7, 37.3, 25.0, 21.5, 17.5, 14.2, 14.1. HRMS (ESI) [M + H]+ calcd for C15H19BrN: 292.0695, found: 292.0697.
Ethyl 3-ethyl-8-methyl-2-propylquinoline-6-carboxylate (3ec).
White solid. 1H NMR (400 MHz, CDCl3) δ: 8.34 (s, 1H), 8.05 (s, 1H), 7.88 (s, 1H), 4.43 (q, J = 7.2 Hz, 2H), 2.96 (t, J = 7.2 Hz, 2H), 2.85–2.79 (m, 5H), 1.99–1.90 (m, 2H), 1.44 (t, J = 7.2 Hz, 3H), 1.33 (t, J = 7.6 Hz, 3H), 1.08 (t, J = 7.6 Hz, 3H); 13C NMR (100 MHz, CDCl3) δ: 166.8, 162.7, 147.2, 137.0, 135.8, 134.7, 128.0, 127.5, 126.8, 126.2, 61.0, 37.5, 25.0, 21.5, 17.8, 14.4, 14.2, 14.1. HRMS (ESI) [M + H]+ calcd for C18H24NO2: 286.1802, found: 286.1801.
4-Ethoxy-2-methyl-1,2,3,4-tetrahydroquinoline (4a).
Yellow liquid. 1H NMR (600 MHz, CDCl3) δ: 7.34 (d, J = 7.8 Hz, 1H), 7.01–6.99 (m, 1H), 6.69–6.66 (m, 1H), 6.46–6.45 (m, 1H), 4.67–4.64 (m, 1H), 3.73–3.68 (m, 2H), 3.59–3.55 (m, 1H), 3.54–3.50 (m, 1H), 2.24–2.21 (m, 1H), 1.66 (q, J = 10.8 Hz, 1H), 1.28 (t, J = 7.2 Hz, 3H), 1.24 (d, J = 6.6 Hz, 3H); 13C NMR (150 MHz, CDCl3) δ: 144.5, 128.1, 127.3, 122.5, 117.4, 113.8, 73.7, 63.3, 46.3, 36.0, 22.5, 15.7. HRMS (ESI) [M + H]+ calcd for C12H18NO: 192.1383, found: 192.1381.
6-Chloro-4-ethoxy-2-methyl-1,2,3,4-tetrahydroquinoline (4b).
Yellow liquid. 1H NMR (600 MHz, CDCl3) δ: 7.43–7.42 (m, 1H), 7.07–7.05 (m, 1H), 6.31 (d, J = 8.4 Hz, 1H), 4.57–4.55 (m, 1H), 3.75–3.69 (m, 1H), 3.67 (s, 1H), 3.58–3.53 (m, 1H), 3.50–3.47 (m, 1H), 2.24–2.20 (m, 1H), 1.58 (q, J = 10.8 Hz, 1H), 1.28 (t, J = 7.2 Hz, 3H), 1.23 (d, J = 6.0 Hz, 3H); 13C NMR (150 MHz, CDCl3) δ: 143.4, 130.7, 129.8, 124.5, 115.3, 108.9, 73.3, 63.8, 46.1, 35.5, 22.3, 15.6. HRMS (ESI) [M + H]+ calcd for C12H17ClNO: 226.0993, found: 226.0995.
6-Chloro-4-ethoxy-2-methyl-8-(trifluoromethyl)-1,2,3,4-tetrahydroquinoline (4c).
Yellow liquid. 1H NMR (600 MHz, CDCl3) δ: 7.38–7.37 (m, 1H), 7.29–7.27 (m, 1H), 4.63 (t, J = 5.6 Hz, 1H), 4.51 (d, J = 7.2 Hz, 1H), 3.73–3.70 (m, 1H), 3.69–3.62 (m, 2H), 1.88–1.86 (m, 2H), 1.24–1.22 (m, 3H), 1.21–1.19 (m, 3H); 13C NMR (150 MHz, CDCl3) δ: 143.6, 132.8, 126.6 (q, J = 5.9 Hz), 124.3 (q, J = 270.8 Hz), 114.4 (q, J = 29.7 Hz), 113.7, 100.7, 61.8, 61.5, 45.6, 40.8, 20.5, 15.3. HRMS (ESI) [M + H]+ calcd for C13H16ClF3NO: 294.0867, found: 294.0868.
6-Bromo-4-ethoxy-8-ethyl-2-methyl-1,2,3,4-tetrahydroquinoline (4d).
Yellow liquid. 1H NMR (600 MHz, CDCl3) δ: 7.35–7.34 (m, 1H), 7.02–7.01 (m, 1H), 4.60–4.58 (m, 1H), 3.72–3.69 (m, 1H), 3.58–3.54 (m, 1H), 3.53–3.49 (m, 2H), 2.36 (q, J = 7.8 Hz, 2H), 2.24–2.21 (m, 1H), 1.63–1.57 (m, 1H), 1.29–1.27 (m, 6H), 1.20 (t, J = 7.2 Hz, 3H); 13C NMR (150 MHz, CDCl3) δ: 140.9, 129.3, 128.4, 127.4, 124.1, 109.0, 73.6, 63.7, 46.1, 35.4, 23.3, 22.6, 15.6, 12.4. HRMS (ESI) [M + H]+ calcd for C14H21BrNO: 298.0801, found: 298.0803.
4-Ethoxy-8-methoxy-2-methyl-1,2,3,4-tetrahydroquinoline (4e).
Yellow liquid. 1H NMR (600 MHz, CDCl3) δ: 7.01–6.99 (m, 1H), 6.65–6.64 (m, 2H), 4.72–4.69 (m, 1H), 4.11 (s, 1H), 3.81 (s, 3H), 3.72–3.67 (m, 1H), 3.58–3.54 (m, 1H), 3.53–3.48 (m, 1H), 2.24–2.20 (m, 1H), 1.70 (q, J = 10.8 Hz, 1H), 1.28–1.26 (m, 6H); 13C NMR (150 MHz, CDCl3) δ: 145.7, 134.5, 122.5, 119.3, 116.2, 108.3, 73.7, 63.1, 55.3, 46.0, 35.9, 22.5, 15.6. HRMS (ESI) [M + H]+ calcd for C13H20NO: 222.1489, found: 222.1491.
Conclusions
In summary, we have developed a convenient method for the direct synthesis of 8-methyl-2-alkylquinolines from o-toluidines and alkenyl ethers through using visible-light photoredox catalysis under mild conditions. This method provides a new strategy for the construction of quinoline derivatives with excellent functional group compatibility, providing rapid access to biological quinoline motifs. A plausible reaction mechanism through the electronic transfer pathway has been proposed and further studies are currently underway in our laboratory.
Conflicts of interest
There are no conflicts to declare.
Acknowledgements
We gratefully acknowledge the National Natural Science Foundation of China (21901081 and 21772062) and the Young Talent Key Project of Anhui Province (170808J02) for financial support.
Notes and references
-
(a) D. C. Behenna, J. L. Stockdill and B. M. Stoltz, Angew. Chem., Int. Ed., 2008, 47, 2365 CrossRef CAS
;
(b) L. K. Kong, Y. Y. Zhou, H. Huang, Y. Yang, Y. Liu and Y. Z. Li, J. Org. Chem., 2015, 80, 1275 CrossRef CAS PubMed
.
-
(a) X.-F. Cai, W.-X. Huang, Z.-P. Chen and Y.-G. Zhou, Chem. Commun., 2014, 50, 9588 RSC
;
(b) Y.-Y. Huang, C. Cai, X. Yang, Z.-C. Lv and U. Schneider, ACS Catal., 2016, 6, 5747 CrossRef CAS
.
-
(a) M. Krishnamurthy, K. Simon, A. M. Orendt and P. A. Beal, Angew. Chem., Int. Ed., 2007, 46, 7044 CrossRef CAS
;
(b) V. R. Solomon and H. Lee, Curr. Med. Chem., 2011, 18, 1488 CrossRef CAS
;
(c) M. Rouffet, C. A. F. Oliveira, Y. Udi, A. Agrawal, I. Sagi, J. A. McCammon and S. M. Cohen, J. Am. Chem. Soc., 2010, 132, 8232 CrossRef CAS
.
-
(a) F.-S. Chang, W. Chen, C. Wang, C.-C. Tzeng and Y.-L. Chen, Bioorg. Med. Chem., 2010, 18, 124 CrossRef CAS PubMed
;
(b) G. Verniest, X. Wang, N. De Kimpe and A. J. Padwa, Org. Chem., 2010, 75, 424 CrossRef CAS
;
(c) J.-F. Mouscadet and D. Desmaele, Molecules, 2010, 15, 3048 CrossRef CAS
;
(d) Z.-G. Jiao, H.-Q. He, C.-C. Zeng, J.-J. Tan, L.-M. Hu and C.-X. Wang, Molecules, 2010, 15, 1903 CrossRef CAS
.
-
(a) H. R. Henze and D. W. Carroll, J. Am. Chem. Soc., 1954, 76, 4580 CrossRef CAS
;
(b) S. E. Denmark and S. Venkatraman, J. Org. Chem., 2006, 71, 1668 CrossRef CAS PubMed
;
(c) F.-F. Zhuo, W.-W. Xie, Y.-X. Yang, L. Zhang, P. Wang, R. Yuan and C.-S. Da, J. Org. Chem., 2013, 78, 3243 CrossRef CAS PubMed
;
(d) E. A. Steck, L. L. Hallock, A. J. Holland and L. T. Fletcher, J. Am. Chem. Soc., 1948, 70, 1012 CrossRef CAS
;
(e) N. P. Buu-Hoie, R. Royer, N. D. Xuong and P. Jacquignon, J. Am. Chem. Soc., 1953, 75, 1209 CrossRef
;
(f) J. Collin, N. Jaber and M. I. Lannou, Tetrahedron Lett., 2001, 42, 7405 CrossRef CAS
;
(g) J. Marco-Contelles, E. Perez-Mayoral, A. Samadi, M. C. Carreiras and E. Soriano, Chem. Rev., 2009, 109, 2652 CrossRef CAS
.
-
(a) S. E. Diamond, A. Szalkiewicz and F. Mares, J. Am. Chem. Soc., 1979, 101, 490 CrossRef CAS
;
(b) M. Beller, O. R. Thiel, H. Trauthwein and C. G. Hartung, Chem. – Eur. J., 2000, 6, 2513 CrossRef CAS
.
- Y. Watanabe, Y. Tsuji and Y. Ohsugi, Tetrahedron Lett., 1981, 22, 2667 CrossRef CAS
.
-
(a) C. Li, J. Li, Y. An, J. Peng, W. Wu and H. Jiang, J. Org. Chem., 2016, 81, 12189 CrossRef CAS
;
(b) B. Gabriele, R. Mancuso, G. Salerno, G. Ruffolo and P. Plastina, J. Org. Chem., 2007, 72, 6873 CrossRef CAS
;
(c) Z. Zhang, J. Tan and Z. Wang, Org. Lett., 2008, 10, 173 CrossRef CAS
.
- L. Li and W. D. Jones, J. Am. Chem. Soc., 2007, 129, 10707 CrossRef CAS
.
- R. P. Korivi and C.-H. Cheng, J. Org. Chem., 2006, 71, 7079 CrossRef CAS
.
-
(a) L.-Y. Xi, R.-Y. Zhang, L. Zhang, S.-Y. Chen and X.-Q. Yu, Org. Biomol. Chem., 2015, 13, 3924 RSC
;
(b) B. R. McNaughton and B. L. Miller, Org. Lett., 2003, 5, 4257 CrossRef CAS
;
(c) F. Basuli, H. Aneetha, J. C. Huffman and D. J. Mindiola, J. Am. Chem. Soc., 2005, 127, 17992 CrossRef CAS
.
-
(a) Y. Matsubara, S. Hirakawa, Y. Yamaguchi and Z. Yoshida, Angew. Chem., Int. Ed., 2011, 50, 7670 CrossRef CAS
;
(b) S. Verma, D. Verma and S. L. Jain, Tetrahedron Lett., 2014, 55, 2406 CrossRef CAS
;
(c) S. T. Le, C. Yasuoka, H. Asahara and N. Nishiwaki, Molecules, 2016, 21, 827 CrossRef
;
(d) K. K. H. Chandrashekarappa, K. M. Mahadevan and K. B. Manjappa, Tetrahedron Lett., 2013, 54, 1368 CrossRef CAS
.
- For selected reviews, see:
(a) C. K. Prier, D. A. Rankic and D. W. C. MacMillan, Chem. Rev., 2013, 113, 5322 CrossRef CAS PubMed
;
(b) K. A. Margrey and D. A. Nicewicz, Acc. Chem. Res., 2016, 49, 1997 CrossRef CAS
;
(c) K. L. Skubi, T. R. Blum and T. P. Yoon, Chem. Rev., 2016, 116, 10035 CrossRef CAS
;
(d) J. Xuan and W.-J. Xiao, Angew. Chem., Int. Ed., 2012, 51, 6828 CrossRef CAS
;
(e) D. Ravelli, S. Protti and M. Fagnoni, Chem. Rev., 2016, 116, 9850 CrossRef CAS
;
(f) T. Chatterjee, N. Iqbal, Y. You and E. J. Cho, Acc. Chem. Res., 2016, 49, 2284 CrossRef CAS
.
-
(a) J. Liu, Q. Liu, H. Yi, C. Qin, R. Bai, X. Qi, Y. Lan and A. Lei, Angew. Chem., Int. Ed., 2014, 53, 502 CrossRef CAS
;
(b) S. Maity and N. Zheng, Angew. Chem., Int. Ed., 2012, 51, 9562 CrossRef CAS
;
(c) J. Xuan, Z.-J. Feng, S.-W. Duan and W.-J. Xiao, RSC Adv., 2012, 2, 4065 RSC
;
(d) Y.-Q. Zou, L.-Q. Lu, L. Fu, N.-J. Chang, J. Rong, J.-R. Chen and W.-J. Xiao, Angew. Chem., Int. Ed., 2011, 50, 7171 CrossRef CAS
.
-
(a) Y. Q. Zou, J. R. Chen, X. P. Liu, L. Q. Lu, R. L. Davis, K. A. Jorgensen and W.-J. Xiao, Angew. Chem., Int. Ed., 2012, 51, 784 CrossRef CAS
;
(b) H. Yi, C. Bian, X. Hu, L. Niu and A. Lei, Chem. Commun., 2015, 51, 14046 RSC
;
(c) K. Wang, L.-G. Meng, Q. Zhang and L. Wang, Green Chem., 2016, 18, 2864 RSC
;
(d) S. Yang, P. Li, Z. Wang and L. Wang, Org. Lett., 2017, 19, 3386 CrossRef CAS
.
-
(a) C. Zhou, P. Li, X. Zhu and L. Wang, Org. Lett., 2015, 17, 6198 CrossRef CAS
;
(b) L. Zou, P. Li, B. Wang and L. Wang, Chem. Commun., 2019, 55, 3737 RSC
;
(c) B. Wang, P. Li, T. Miao, L. Zou and L. Wang, Org. Biomol. Chem., 2019, 17, 115 RSC
.
Footnote |
† Electronic supplementary information (ESI) available. See DOI: 10.1039/c9ob02259g |
|
This journal is © The Royal Society of Chemistry 2020 |
Click here to see how this site uses Cookies. View our privacy policy here.