Lewis or Brønsted acid-catalysed reaction of propargylic alcohol-tethered alkylidenecyclopropanes with indoles and pyrroles for the preparation of polycyclic compounds tethered with indole or pyrrole motif†
Received
12th October 2019
, Accepted 21st November 2019
First published on 22nd November 2019
Abstract
We developed a facile synthetic method to access cyclopenta[b]naphthalene derivatives via the Lewis or Brønsted acid catalysed cascade nucleophilic addition, electronic cyclization, ring-opening rearrangement of propargylic alcohol-tethered alkylidenecyclopropanes with indole and pyrrole derivatives. The reaction exhibited a broad substrate scope and good functional group tolerance under metal-free conditions, affording the desired products in moderate to good yields.
Introduction
Cyclopenta[b]naphthalene structural motifs exist in numerous natural products1 and functional materials.2 Besides, naphthalene-based dyes consisting of cyclopenta[b]naphthalene and other groups possess desirable photophysical properties such as wavelength-dependent emission with changes in microenvironment polarity, high quantum yield, and decent photostability (Scheme 1).3
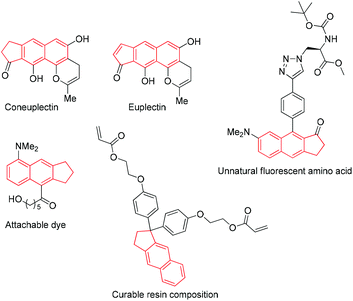 |
| Scheme 1 Representative examples containing cyclopenta[b]naphthalene skeleton. | |
Indole and its derivatives are extensively distributed in natural products and are general structural motifs in pharmaceuticals.4 Their different and productive biological activities have resulted in their classification as “privileged” heterocycles in medicinal chemistry.4a,g,5 Methylenecyclopropanes (MCPs) are a type of highly strained molecule and have been widely applied in organic synthesis,6 pharmaceutical chemistry,7 agricultural chemistry,8 and even materials science.9 MCPs can undergo an assortment of reactions, which are facilitated owing to the release of the intramolecular strain of its small ring and exocyclic C–C bond. Propargylic alcohols can undergo various cascade processes to furnish structurally attractive carbocycles or heterocycles by forming in situ generated allenes. Lewis/Brønsted acid-catalysed nucleophilic substitution of propargylic alcohols is essential in organic synthetic chemistry, which can be converted into diverse acyclic, carbocyclic, and heterocyclic synthetic building blocks.10 To design a novel cascade reaction under mild conditions, we considered applying indole or pyrrole as a nucleophile to trigger an intermolecular cascade reaction of propargylic alcohol-tethered methylene cyclopropanes.
In 2016, our group reported a thermally induced ring-opening and cyclization reaction from ortho-aminoaryl-tethered alkylidenecyclopropanes with in situ generated isocyanates or isothiocyanates to afford furoquinoline and thienoquinoline derivatives (Scheme 2, eqn (1) and (2)).6g In 2010, Hu and co-workers reported a method to construct 4,9-diphenyl-2,3-dihydro-1H-cyclopenta[b]naphthalene derivatives via the palladium(0)-catalysed reaction of diynes with aryl halides through C–C coupling and C–H bond activation of the incorporated aryl group (Scheme 2, eqn (3)).11 Based on these previous findings, herein, we report the Lewis or Brønsted acid catalysed and pronucleophile-involved [3 + 2] cyclization reaction of propargyl alcohol-tethered methylene cyclopropanes with the in situ generated allene moiety for the facile synthesis of cyclopenta[b]naphthalene derivatives under metal-free conditions.
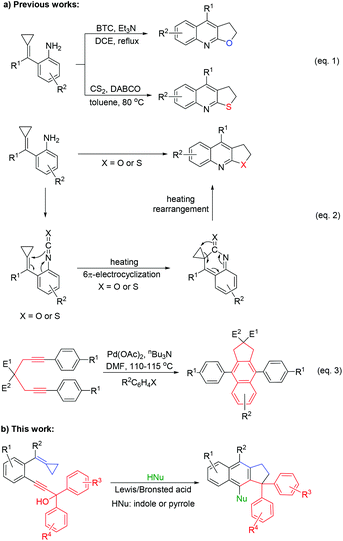 |
| Scheme 2 Previous works and this work. | |
Results and discussion
We initially investigated the reaction outcome using 1a as a model substrate in the presence of indole (1.0 equiv.) and found that the desired cyclized product 2a was obtained in 50% yield accompanied with by-product 3a at room temperature in acetonitrile using BF3·OEt2 (20 mol%) as the catalyst (Table 1, entry 1). Then, we evaluated different temperatures ranging from 40 °C to 80 °C and identified that room temperature is the most appropriate temperature in acetonitrile for this reaction in the presence of BF3·OEt2 (Table 1, entries 2 and 3). The examination of solvent effects revealed that acetonitrile is the most suitable solvent for this reaction with BF3·OEt2 as the catalyst at room temperature (Table 1, entries 4–6). Several other Lewis acids such as Sc(OTf)3 and AgSbF6 were tested in acetonitrile, but no better result was obtained (Table 1, entries 7 and 8). Next, Brønsted acids such as trifluoromethylsulfonic acid (TfOH), acetic acid (CH3CO2H) and trifluoroacetic acid (CF3COOH) were selected as the catalyst for this reaction and we identified that the use of CF3COOH as the catalyst gave 2a in 55% yield along with 3a as the by-product (Table 1, entries 9–11). It should be noted that the acids used as catalysts should have enough acidity to remove the hydroxyl group to generate the carbocationic intermediate, but too strong Brønsted acid such as TfOH can decompose the MCP group, which will cause the production of 2a in lower yield. After screening solvents in the presence of CF3COOH at room temperature, we found that chlorobenzene was the solvent of choice, giving 2a in 63% yield (Table 1, entries 12–16). Using 2.0 equiv. of 1a to react with 1.0 equiv. of indole or carrying out the reaction in a diluted solution in 2.0 mL of MeCN did not further improve the yield of 2a (Table 1, entries 17 and 18) (for more details on the screening of reaction conditions including other Brønsted acids, see Tables S1 and S2 in the ESI†). It should be mentioned here that the production of 3a could not be avoided due to the two competitive addition reactions, which produced 2a and 3a (see Scheme 6 for the reaction mechanism).12 The structure of 2a was unambiguously established by X-ray diffraction. The ORTEP drawing of 2a is shown Fig. 1, and the corresponding CIF data is presented in the ESI.†
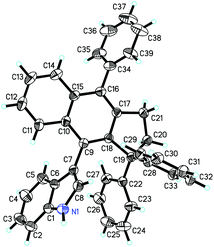 |
| Fig. 1 X-ray structures of 2a. | |
Table 1 Screening of reaction conditions
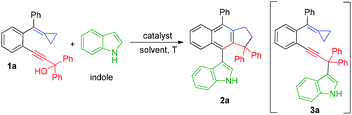
|
Entrya |
Catalyst |
Solvent |
T [°C] |
Yieldb/2a [%] |
2a : 3a e |
Reaction conditions: 1a (0.10 mmol, 1.0 equiv.), indole (0.10 mmol, 1.0 equiv.), catalyst (20 mol%), solvent (1.0 mL), T, for 6 h.
1H NMR yield using 1,3,5-trimethoxybenzene as an internal standard.
Reaction conditions: 1a (0.40 mmol, 0.2 equiv.), indole (0.20 mmol, 1.0 equiv.), catalyst (20 mol%), solvent (2.0 mL).
Reaction conditions: 1a (0.20 mmol, 1.0 equiv.), indole (0.20 mmol, 1.0 equiv.), catalyst (20 mol%), solvent (2.0 mL), isolated yield.
The ratio of 2a and 3a was determined by 1H NMR spectroscopic data.
|
1 |
BF3·OEt2 |
MeCN |
rt |
50 |
1.6 : 1 |
2 |
BF3·OEt2 |
MeCN |
40 |
41 |
1.6 : 1 |
3 |
BF3·OEt2 |
MeCN |
80 |
32 |
1.8 : 1 |
4 |
BF3·OEt2 |
EA |
rt |
43 |
1.0 : 1 |
5 |
BF3·OEt2 |
PhCl |
rt |
— |
— |
6 |
BF3·OEt2 |
PhMe |
rt |
22 |
1.1 : 1 |
7 |
Sc(OTf)3 |
MeCN |
rt |
37 |
1.5 : 1 |
8 |
AgSbF6 |
MeCN |
rt |
— |
— |
9 |
CF3SO3H |
MeCN |
rt |
40 |
1.3 : 1 |
10 |
CH3COOH |
MeCN |
rt |
— |
— |
11 |
CF3COOH |
MeCN |
rt |
55 |
2.0 : 1 |
12 |
CF3COOH |
DMF |
rt |
— |
— |
13 |
CF3COOH |
DMSO |
rt |
— |
— |
14 |
CF3COOH |
PhMe |
rt |
51 |
1.2 : 1 |
15 |
CF3COOH |
PhCl |
rt |
63 |
1.9 : 1 |
16 |
CF3COOH |
PhF |
rt |
50 |
1.2 : 1 |
17c |
CF3COOH |
PhCl |
rt |
60 |
1.9 : 1 |
18d |
CF3COOH |
PhCl |
rt |
57 |
1.4 : 1 |
With the optimized reaction condition in hand, we next surveyed a range of substrates for this reaction. We first turned our attention toward the scope of the catalytic cascade cyclization of propargylic alcohol-tethered alkylidenecyclopropanes (1b–1n) with indole as the nucleophile, and the results are summarized in Scheme 3. The R1 substituent could be electron-donating and electron-withdrawing at different positions, affording the desired products 2b–2e in moderate yields ranging from 40% to 46%. When R2 was a methoxyphenyl group or a heteroaromatic ring, the reaction proceeded smoothly, producing the desired products 2f and 2g in 30% and 49% yields, respectively. The electron-donating and electron-withdrawing substituent could be also introduced at the benzene ring in the propargylic alcohol moiety, giving the desired products 2h–2k in moderate to good yields. It should be noted that 2k was presented as two atropisomers owing to the rotation obstruction of the C–C bond connecting the indole moiety to the cyclopenta[b]naphthalene motif. The reaction was sensitive to the R2 group in the presence of indole (Scheme 3). When substrates 1l (R2 = Me) and 1n (R2 = 4-ClC6H4) were applied for the reaction, traces of the corresponding cyclized products were obtained because these groups with a lower electron density compared to the phenyl group would reduce the reactivity of the MCP group. In addition, when substrate 1m (R2 = 4-BnOC6H4) was used in the reaction, the BnO group could act as a leaving group in the presence of CF3COOH, rendering that the target product was not obtained. Furthermore, when a substrate bearing two methyl groups at the propargylic moiety was applied for this reaction with indole as the nucleophile, no reaction occurred. For the substrate having one phenyl group and one methyl group at the propargylic moiety, the corresponding by-product was mainly obtained. We attribute the results to the property of the carbocationic intermediates. The presence of aryl groups can stabilize the carbocationic intermediate formed in the propargylic moiety, and thus the process from 1 to intermediates A/B (see mechanistic explanation shown in Scheme 6) can proceed, subsequently improving the regioselectivity.
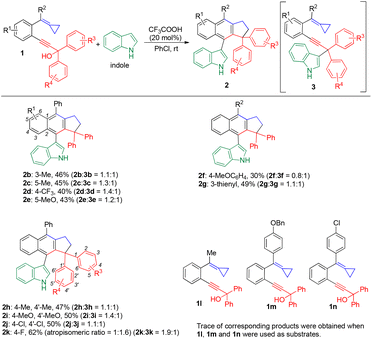 |
| Scheme 3 Scope of the catalytic cyclization of propargylic alcohol-tethered alkylidenecyclopropanes with indole as a nucleophile. Reaction conditions: 1 (0.20 mmol, 1.0 equiv.), indole (0.20 mmol, 1.0 equiv.), CF3COOH (0.2 equiv.), PhCl (2.0 mL) at r.t. for 6 h. | |
Subsequently, we also examined the substituted indoles in this reaction using 1a as a substrate and found that similar results were obtained, affording the desired products 2o–2w in moderate to good yields ranging from 40%–69%, suggesting that the electronic property of the substituent at the indole nucleophile did not have a significant impact on the reaction outcome (Scheme 4).
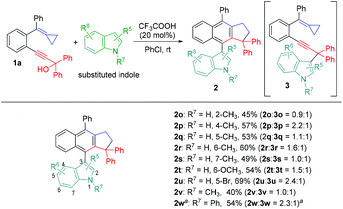 |
| Scheme 4 Scope of the catalytic cyclization of propargylic alcohol-tethered alkylidenecyclopropanes with substituted indoles as nucleophiles. Reaction conditions: 1a (0.20 mmol, 1.0 equiv.), substituted indole (0.20 mmol, 1.0 equiv.), CF3COOH (20 mol%), PhCl (2.0 mL), at r.t. for 6 h. a Reaction conditions: 1 (0.20 mmol, 1.0 equiv.), substituted indole (0.20 mmol, 1.0 equiv.), BF3·Et2O (20 mol%), PhCl (2.0 mL), at r.t. for 8 h. | |
Using pyrrole and its derivatives as nucleophiles for this cascade nucleophilic cyclization reaction, to our delight, the corresponding products 4a–4f were exclusively obtained in over 90% yield probably because the nucleophilicity of these pyrroles is weaker than that of indoles, leading to the nucleophilic substitution of the hydroxyl group in 1 being impossible (Scheme 5). Some other nucleophiles including furan, thiophene, imidazole, benzimidazole, and benzothiazole were used for this reaction under the standard conditions, but no corresponding product was obtained due to their weak nucleophilicity.
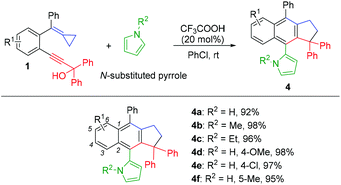 |
| Scheme 5 Scope of the catalytic cyclization of propargylic alcohol-tethered alkylidenecyclopropanes with pyrrole and its derivatives as nucleophiles. Reaction conditions: 1 (0.20 mmol, 1.0 equiv.), substituted pyrrole (0.20 mmol, 1.0 equiv.), CF3COOH (20 mol%), PhCl (2.0 mL), at r.t. for 6 h. | |
Based on these results and the previous reports, a plausible mechanism for this cascade nucleophilic cyclization of propargylic alcohol-tethered alkylidenecyclopropanes with pronucleophiles is proposed in Scheme 6.6g,13 Firstly, a carbocationic intermediate was formed upon treating 1 with a Lewis/Brønsted acid. The corresponding intermediates A and B are two types of resonances of the carbocationic intermediate. The nucleophilic attack of indole or pyrrole to the 2-position of the carbocationic intermediate afforded intermediate C, which underwent 6π-electrocyclization to give intermediate D. Then, a cyclopropane ring-opening rearrangement took place from intermediate D to afford the corresponding product 2. Meanwhile, the direct nucleophilic substitution of HNu at the 1-position of the carbocationic intermediate gave by-product 3.
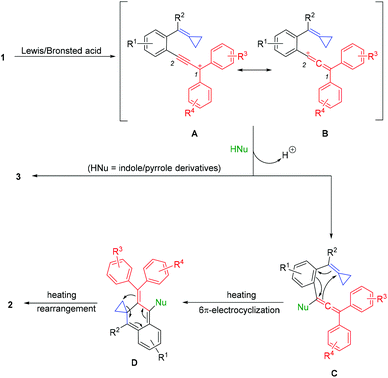 |
| Scheme 6 Plausible reaction mechanism. | |
Conclusions
In conclusion, we developed a novel Lewis or Brønsted acid-catalysed reaction of propargylic alcohol-tethered alkylidenecyclopropanes with indole and pyrrole derivatives for the preparation of cyclopenta[b]naphthalene skeletons tethered with indole and pyrrole motifs in moderate to excellent yields under mild conditions. This simple synthetic methodology avoided the use of transition metal catalysts, providing a useful tool for the synthesis of polycyclic compounds. Further exploration on the synthesis of useful polycyclic compounds with this new synthetic protocol is underway.
Experimental
General procedures for the preparation of substrates
To a stirred solution of iodine-substituted methylenecyclopropanes (1.1 equiv.) and propargylic alcohol (1.0 equiv.) in iPr2NH (30 mL), PdCl2(PPh3)2 (2 mol%) and CuI (2 mol%) were added under an argon atmosphere. The resulting mixture was stirred at 80 °C for 8 h. After the separation of ammonium salt by filtration and the removal of solvent under reduced pressure, the residue was purified by column chromatography on silica gel to afford the corresponding substrates in good yields ranging from 85% to 98% (for more details, see chapter 3 in the ESI†).
General procedures for the synthesis of the cyclized products
To a stirred solution of propargylic alcohol-tethered alkylidenecyclopropanes (0.2 mmol) and substituted indole (0.2 mmol) in chlorobenzene (2 mL), catalyst (20 mol%) was added, and the resulting mixture was stirred at room temperature for 6 h. After removing the solvent under reduced pressure, the residue was purified by column chromatography on silica gel to afford the corresponding cyclized products.
Conflicts of interest
There are no conflicts to declare.
Acknowledgements
We are grateful for the financial support from the National Basic Research Program of China [(973)-2015CB856603], the Strategic Priority Research Program of the Chinese Academy of Sciences (Grant No. XDB20000000) and sioczz201808, the National Natural Science Foundation of China (220472096, 21372241, 21572052, 20672127, 21421091, 21372250, 21121062, 21302203, 20732008, 21772037, 21772226, 21861132014 and 91956115), and the Fundamental Research Funds for the Central Universities 222201717003.
Notes and references
- M. A. Ernst-Russell, C. L. Chai, J. H. Wardlaw and J. A. Elix, J. Nat. Prod., 2000, 63, 129–131 CrossRef CAS PubMed.
-
I. Ayumi and M. Naoyuki, JP 1560594, 2014.
- E. Benedetti, A. B. Veliz, M. Charpenay, L. S. Kocsis and K. M. Brummond, Org. Lett., 2013, 15, 2578–2581 CrossRef CAS PubMed.
-
(a) L. Almagro, F. Fernandez-Perez and M. A. Pedreno, Molecules, 2015, 20, 2973–3000 CrossRef PubMed;
(b) N. Netz and T. Opatz, Mar. Drugs, 2015, 13, 4814–4914 CrossRef CAS PubMed;
(c) W. Zi, Z. Zuo and D. Ma, Acc. Chem. Res., 2015, 48, 702–711 CrossRef CAS PubMed;
(d) T. V. Sravanthi and S. L. Manju, Eur. J. Pharm. Sci., 2016, 91, 1–10 CrossRef CAS PubMed;
(e) E. Stempel and T. Gaich, Acc. Chem. Res., 2016, 49, 2390–2402 CrossRef CAS PubMed;
(f) S. Sugimoto, M. Naganuma and T. Kanai, J. Gastroenterol., 2016, 51, 853–861 CrossRef CAS PubMed;
(g) N. Chadha and O. Silakari, Eur. J. Med. Chem., 2017, 134, 159–184 CrossRef CAS;
(h) J. Bariwal, L. G. Voskressensky and E. V. Van der Eycken, Chem. Soc. Rev., 2018, 47, 3831–3848 RSC;
(i) X. Y. Liu and Y. Qin, Acc. Chem. Res., 2019, 52, 1877–1891 CrossRef CAS PubMed.
-
(a) R. Patil, S. A. Patil, K. D. Beaman and S. A. Patil, Future Med. Chem., 2016, 8, 1291–1316 CrossRef CAS PubMed;
(b) J. A. Homer and J. Sperry, J. Nat. Prod., 2017, 80, 2178–2187 CrossRef CAS PubMed;
(c) J. Liu, J. Cai, R. Wang and S. Yang, Int. J. Mol. Sci., 2016, 18, 20 CrossRef PubMed;
(d) Y. L. Sang, W. M. Zhang, P. C. Lv and H. L. Zhu, Curr. Top. Med. Chem., 2017, 17, 120–137 CrossRef CAS PubMed;
(e) Y. Wang, F. Xie, B. Lin, M. Cheng and Y. Liu, Chemistry, 2018, 24, 14302–14315 CrossRef CAS PubMed;
(f) A. Blanc and D. M. Perrin, Pept. Sci., 2019, 111, 14 Search PubMed;
(g) J. Kaur, D. Utreja, Ekta, N. Jain and S. Sharma, Curr. Org. Synth., 2019, 16, 17–37 CrossRef CAS;
(h) J. Lunagariya, P. Bhadja, S. Zhong, R. Vekariya and S. Xu, Mini-Rev. Med. Chem., 2019, 19, 751–761 CrossRef CAS PubMed.
-
(a) L. Yu, M. Liu, F. Chen and Q. Xu, Org. Biomol. Chem., 2015, 13, 8379–8392 RSC;
(b) L. Yu, J. Luan, L. Xu, Y. H. Ding and Q. Xu, Tetrahedron Lett., 2015, 56, 6116–6119 CrossRef CAS;
(c) Z. Z. Zhu, K. Chen, L. Z. Yu, X. Y. Tang and M. Shi, Org. Lett., 2015, 17, 5994–5997 CrossRef CAS PubMed;
(d) K. Chen, J. X. Liu, X. Y. Tang and M. Shi, Chemistry, 2016, 22, 11549–11553 CrossRef CAS PubMed;
(e) K. Chen, X. Y. Tang and M. Shi, Chem. Commun., 2016, 52, 1967–1970 RSC;
(f) L. Yu, F. L. Chen and Y. H. Ding, ChemCatChem, 2016, 8, 1033–1037 CrossRef CAS;
(g) L. Z. Yu, X. B. Hu, Q. Xu and M. Shi, Chem. Commun., 2016, 52, 2701–2704 RSC;
(h) L.-Z. Yu, Q. Xu, X.-Y. Tang and M. Shi, ACS Catal., 2015, 6, 526–531 CrossRef;
(i) Y. Liu, Q. L. Wang, C. S. Zhou, B. Q. Xiong, P. L. Zhang, C. A. Yang and K. W. Tang, J. Org. Chem., 2017, 82, 7394–7401 CrossRef CAS PubMed;
(j) Y. Liu, Q. L. Wang, C. S. Zhou, B. Q. Xiong, P. L. Zhang, C. A. Yang and K. W. Tang, J. Org. Chem., 2018, 83, 4657–4664 CrossRef CAS PubMed;
(k) Y. Liu, Q. L. Wang, Z. Chen, Q. Zhou, H. Li, C. S. Zhou, B. Q. Xiong, P. L. Zhang and K. W. Tang, J. Org. Chem., 2019, 84, 2829–2839 CrossRef CAS PubMed;
(l) H. Cao, F. Chen, C. Su and L. Yu, Adv. Synth. Catal., 2019 DOI:10.1002/adsc.201900615.
-
(a) J. Ward, A. Reece, K. Li and D. Choo, Antiviral Res., 2010, 86, A69–A69 CrossRef;
(b) G. Obame, P. Bremond, C. Pannecouque and G. Audran, Synthesis, 2013, 45, 2612–2618 CrossRef CAS;
(c) B. J. Hunt, Surv. Anesthesiol., 2014, 58, 274–275 CrossRef.
-
(a) B. Perrin, Revue française d'administration publique, 2009, 132, 711 CrossRef;
(b) D. Schuster and G. Wolber, Planta Med., 2010, 76, 1179–1179 Search PubMed.
-
(a) X. M. Yang, A. M. Seyam, P. F. Fu and T. J. Marks, Macromolecules, 1994, 27, 4625–4626 CrossRef CAS.
-
(a) K. W. Feng, Y. L. Ban, P. F. Yuan, W. L. Lei, Q. Liu and R. Fang, Org. Lett., 2019, 21, 3131–3135 CrossRef CAS PubMed;
(b) Y.-P. Han, X.-S. Li, M. Li, X.-Y. Zhu and Y.-M. Liang, Adv. Synth. Catal., 2018, 360, 2796–2800 CrossRef CAS;
(c) Y. P. Han, X. R. Song, Y. F. Qiu, H. R. Zhang, L. H. Li, D. P. Jin, X. Q. Sun, X. Y. Liu and Y. M. Liang, Org. Lett., 2016, 18, 940–943 CrossRef CAS PubMed;
(d) J. Y. Hu, J. Ma, Q. G. Zhu, Q. L. Qian, H. L. Han, Q. Q. Mei and B. X. Han, Green Chem., 2016, 18, 382–385 RSC;
(e) B. Kalvacherla, S. Batthula, S. Balasubramanian and R. K. Palakodety, Org. Lett., 2018, 20, 3824–3828 CrossRef CAS PubMed;
(f) X. S. Li, Y. P. Han, X. Y. Zhu, Y. Xia, W. X. Wei, M. Li and Y. M. Liang, Adv. Synth. Catal., 2018, 360, 4441–4445 CrossRef CAS;
(g) Q. W. Song, Z. H. Zhou, M. Y. Wang, K. Zhang, P. Liu, J. Y. Xun and L. N. He, ChemSusChem, 2016, 9, 2054–2058 CrossRef CAS PubMed;
(h) B. A. Trofimov, O. A. Shemyakina, A. G. Malkina, A. V. Stepanov, O. G. Volostnykh, I. A. Ushakov and A. V. Vashchenko, Eur. J. Org. Chem., 2016, 5465–5469 CrossRef CAS.
- Y. Hu, H. Yao, Y. Sun, J. Wan, X. Lin and T. Zhu, Chemistry, 2010, 16, 7635–7641 CrossRef CAS PubMed.
-
(a) R. Sanz, D. Miguel, A. Martinez, M. Gohain, P. Garcia-Garcia, M. A. Fernandez-Rodriguez, E. Alvarez and F. Rodriguez, Eur. J. Org. Chem., 2010, 7027–7039 CrossRef CAS;
(b) C. C. Silveira, S. R. Mendes, L. Wolf and G. M. Martins, Tetrahedron Lett., 2010, 51, 4560–4562 CrossRef CAS.
-
(a) W. Huang, Q. Shen, J. Wang and X. Zhou, J. Org. Chem., 2008, 73, 1586–1589 CrossRef CAS PubMed;
(b) W. Huang, P. Zheng, Z. Zhang, R. Liu, Z. Chen and X. Zhou, J. Org. Chem., 2008, 73, 6845–6848 CrossRef CAS PubMed;
(c) Y. Liu, B.-D. Barry, H. Yu, J. Liu, P. Liao and X. Bi, Org. Lett., 2013, 15, 2608–2611 CrossRef CAS PubMed.
Footnote |
† Electronic supplementary information (ESI) available: Detailed experimental procedures and analytical data. CCDC 1584674. For ESI and crystallographic data in CIF or other electronic format see DOI: 10.1039/c9ob02211b |
|
This journal is © The Royal Society of Chemistry 2020 |