DOI:
10.1039/C9NJ04932K
(Paper)
New J. Chem., 2020,
44, 231-238
La2MnTiO6 double perovskite nanostructures as highly efficient visible light photocatalysts†
Received
2nd October 2019
, Accepted 19th November 2019
First published on 5th December 2019
Abstract
In this paper, we reported, for the first time, the preparation of La2MnTiO6 double perovskite nanostructures using a simple sol–gel method in the presence of citric acid as the size controlling agent. The crystalline structure, morphology, and elemental composition of the prepared samples were characterized by XRD, TEM, FE-SEM, and EDX mapping analysis. Owing to superior electronic properties of the double perovskite, we used the prepared samples in the photocatalytic degradation of acid blue 113 (AB 113) under visible light irradiation. The photocatalytic degradation of AB 113 was nearly 72% after 120 min irradiation. Also, the effects of the photocatalyst amount and pH value of the dye solution were studied. Investigation on the photocatalytic degradation mechanism using various radical scavenging agents revealed that the photo-generated holes are the dominant oxidants for the degradation of AB 113. The reusability experiment showed that the prepared sample has good recyclability even for 6 reaction cycles.
1. Introduction
In recent years, the increasing development of industries and agriculture has resulted in the accumulation of toxic organic pollutants in water resources.1 Due to the non-biodegradability and destructive nature of these compounds, the treatment of these pollutants is urgent for protecting the environment, ecosystem, and human life.2
Unlike conventional treatment methods such as biological and chemical methods, the photocatalytic treatment of organic pollutants is an effective and green method that employs radiation energy to perform photocatalytic degradation without leaving more poisonous by-products.3
The photocatalytic treatment includes the photo-excitation of semiconductors by irradiation with energy equal or greater than the band gap of the semiconductors. Under this condition, highly reactive oxygen species such as OH˙ and O2−˙ could be produced from photo-generated electrons and holes.4 More common semiconductors utilized in the photocatalytic process include TiO2, ZnO, and ZnS.5
The most common semiconductor photocatalysts suffer from low quantum efficiency caused by the fast recombination of photo-generated electrons and holes6 and wide energy band gap, which implies that they are activated only by the absorption of visible irradiation.7 Therefore, these limitations are a great challenge for the practical applications of common semiconductor photocatalysts.
Mixed metal oxide perovskite structures are alternative photocatalyst materials due to their high temperature stability, non-toxicity, and lower dissolution in aqueous media with a wide range of pH.8
Double perovskite compounds with a stoichiometric structure of A2BB′O6 (A = rare earth metal ions (La, Nd, Sm, and Dy), B = divalent transition metal ions (Cu, Mn), B′ = tetravalent transition metal ions (Ti)) are interesting for use in many advanced technological fields such as catalysts, material science, physics, and chemistry.9–11 The superior properties that make double perovskite materials worthwhile are high electrical conductivity, enabling oxygen storage, significant tunneling magneto-resistance and magneto-dielectric effects.12–14
Because of the known catalytic properties of perovskite structures, recently, perovskite-based photocatalysts have been employed for the photocatalytic degradation of organic dye solutions. In this regard, Peng and coworkers prepared perovskite LaFeO3/montmorillonite nanocomposites and studied their photocatalytic activities towards the degradation of rhodamine B (99.34%) under visible light irradiation.11 Ghiasi et al. reported the photocatalytic degradation of methyl orange using La0.7Sr0.3MnO3 nano-perovskites under solar light.15 Sabeeh et al. prepared highly efficient photocatalysts based on LaFeO3 perovskites and their reduced graphene oxide composites for the degradation of methylene blue under visible light irradiation.12
The multi-metallic oxide character of double perovskite structures meets the demand for potential photocatalysts. The catalytic properties of double perovskite structures are strongly related to the nature of the metal incorporated into the double perovskite structure. Therefore, the properties of the double perovskite structure can be improved by the introduction of different metals in the lattice structure.16,17 Valian et al. reported the preparation of urchin-like Dy2CoMnO6 double perovskite nanostructures and their photocatalytic potentials for the degradation of methylene blue (88%), rhodamine B (38%), and erythrosine (64%) dye under UV irradiation.18 Feraru et al. succeeded in synthesizing visible light photocatalyst, Ca2Fe1−xSmxBiO6, for the degradation of rhodamine 6G (96.9%).16
Double perovskite compounds have been synthesized by employing different synthetic routes including ceramic synthesis19 and sol–gel method.19,20 The crystalline structure, morphology, and particle size are influencing factors on the properties of materials, which in turn affect the synthetic route.12,18 Among them, sol–gel process is considered to be a simple synthetic method, which is able to control the uniformity and size distribution of the particles.
Herein, we report, for the first time, the sol–gel synthesis of La2MnTiO6 double perovskite nanostructures by using citric acid as the size controlling agent. The photocatalytic activity of the as-prepared samples were tested towards the degradation of acid blue 113 (AB 113) under visible light irradiation. The structure, morphology, elemental composition, and optical properties of the prepared La2MnTiO6 were characterized by XRD, SEM, EDX mapping, and DRS. The mechanism of photocatalytic degradation of AB 113 was studied using various radical scavenging agents.
2. Experimental
2.1. Materials
All chemicals were purchased from Merck and used without further purification. La(NO3)3·6H2O, Mn(NO3)2·4H2O, tetrabutyl orthotitanate (TBOT) Ti(C4H9O)4, ethanol, citric acid.
2.2. Preparation of La2MnTiO6
La2MnTiO6 double perovskite nanostructure was prepared using a simple sol–gel route. First, 2 mmol La(NO3)3·6H2O and 1 mmol of Mn(NO3)2·4H2O were dissolved in 20 mL of ethanol. The mixture was stirred for 10 min to form a clear solution (solution I). 1 mmol of TBOT was dissolved in 5 mL ethanol (solution II). Then, solution II was added drop-wise into solution I with stirring. This solution was stirred for 15 min at room temperature. After that, a certain amount of citric acid was added as the particle size controlling agent. The mixture was stirred for 30 min to ensure sufficient interaction between all the reactant precursors. Then, 2 mL D.I. water was added and the solution was stirred for 120 min to complete the hydrolysis reaction. The final solution was kept in an oven at 80 °C for 12 h in order to form the gel product. After that, the gel product was heated at 700 °C for 5 hours to form the pure solid product without any impurity. The same synthetic procedure was followed with the addition of various amounts of citric acid (0.0 mmol, 0.5 mmol, and 1.0 mmol).
2.3. Characterization
The crystallinity and phase structure of the prepared samples were determined by X-ray diffraction (XRD) patterns (Philips X’pert Pro MPD, Cu Kα radiation λ = 1.54 Å). The elemental analysis, morphology, and other surface properties of the prepared La2MnTiO6 double perovskite were characterized using energy dispersive X-ray (EDX) and field emission scanning electron microscopy (FE-SEM) by TESCAN Mira3. Transmission electron microscopic (TEM) image of the prepared sample was obtained by Philips EM208S. The FTIR spectra of the samples were recorded on a Shimadzu Varian 4300 spectrometer to determine the chemical groups of the prepared samples. UV-vis diffuse reflectance spectra (DRS) of La2MnTiO6 prepared with various amounts of citric acid were recorded using a DRS spectrophotometer (A V-670 UV-vis-NIR).
2.4. Photocatalytic experiment of La2MnTiO6
The photocatalytic activity of the prepared La2MnTiO6 double perovskite nanostructure samples were studied by the degradation of AB 113 under visible light irradiation. In this respect, we conducted the photocatalytic experiment by exposing the aqueous solution of AB 113 (50 mL, 50 mg L−1) to visible light irradiation in the presence of 30 mg La2MnTiO6 photocatalyst. The Osram Lamp (HQIBT 400W/D/DE40FLH1 – λ > 400 nm) was used as the visible light source, and the distance between the light source and reaction solution was 30 cm.
First, to achieve the adsorption/desorption equilibrium between the photocatalyst particles and dye molecules, the solution was kept in darkness under magnetic stirring. Then, the photocatalytic reaction was initiated by visible light illumination of the dye solution for 120 min. At a time interval of 20 min, about 3 mL of the dye solution was collected and centrifuged for 5 min at 6000 rpm to separate the photocatalyst particles from the dye solution. The concentration of the dye solution was determined using UV-vis spectrophotometer at λmax = 566 nm.
3. Results and discussion
3.1. Characterization of La2MnTiO6 double perovskite
The crystalline structure of the prepared La2MnTiO6 double perovskite nanostructure was studied by X-ray diffraction (XRD) pattern in the range of 2θ = 10°–80°, which is shown in Fig. 1. The XRD pattern of the as-prepared sample represents the diffraction peaks at 21.89°, 24.91°, 31.55°, 39.08°, 45.62°, 51.52°, 56.82°, 66.99°, 71.70°, and 76.37°, which are clearly attributed to the (101), (111), (121), (022), (202), (311), (042), (004), (313), and (430) diffraction planes of the orthorhombic structure of La2MnTiO6 double perovskite (JCPDS file no. 01-089-6266) without any impurities.
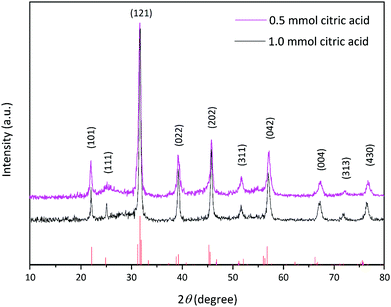 |
| Fig. 1 XRD patterns of the prepared La2MnTiO6 double perovskite nanostructure in the presence of different amounts of citric acid. | |
The average crystallite size of the prepared samples in the presence of various amounts of citric acid were calculated by the Scherrer equation21 employing (121) diffraction peak as follows:
|  | (1) |
where
D is the crystallite size,
k is a dimensionless shape factor with the value of 0.89,
λ is the X-ray wavelength (Cu Kα),
β is the full width at half maximum (FWHM), and
θ is the diffraction angle at maximum peak. The calculated average crystallite size of the prepared La
2MnTiO
6 samples using 0.5 mmol and 1.0 mmol of citric acid were 21.7 nm and 27.4 nm, respectively.
As seen in Fig. 1, the width of the diffraction patterns shows a slight decrease as the amount of citric acid increased, representing that the crystallite size increases with increasing amount of citric acid. According to the Scherrer equation (eqn (1)), the larger crystallite size (D) is related to the decrease in the width of the diffraction peak (β).22
The FE-SEM images represent the morphological properties of the prepared La2MnTiO6, which are provided in Fig. 2(a–f). The FE-SEM images revealed a nanospherical morphology for the prepared La2MnTiO6 double perovskite using citric acid as the size controlling agent (Fig. 2a–d). However, further increase in the amount of citric acid (1.0 mmol) caused larger nanoparticles, as shown in Fig. 2a and b. This may suggest that there is an optimum amount of citric acid and excess amount of citric acid leads to aggregated particles. In addition, small agglomeration can be seen for the sample prepared with 0.5 mmol citric acid (Fig. 2c and d), which is due to heat treatment of the sample at 700 °C. To confirm the effect of citric acid on the size of the prepared sample, the FE-SEM images of La2MnTiO6 without the addition of citric acid are provided in Fig. 2e and f. These latter FE-SEM images reveal that in the absence of citric acid, particle growth continues until the formation of microstructure particles.
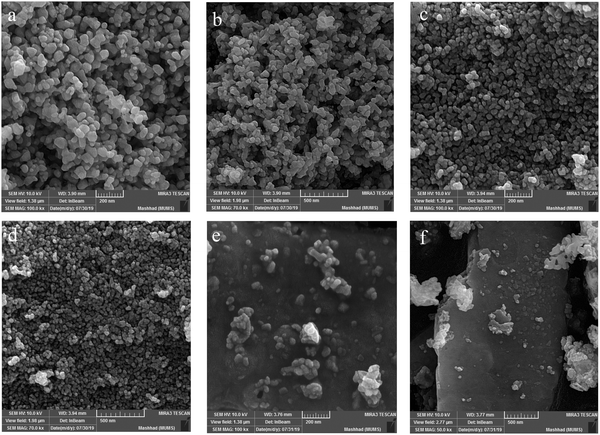 |
| Fig. 2 FE-SEM images with different magnification for La2MnTiO6 nanospheres prepared using 1.0 mmol citric acid (a and b), 0.5 mmol citric acid (c and d), and without the addition of citric acid (e and f). | |
The calculated particle size (Fig. 3a and b) shows particle size distribution for the prepared samples with two different amounts of citric acid. As can be seen, when the amount of citric acid is increased, the particle size distribution becomes wider and the number of particles with a larger average size increases.
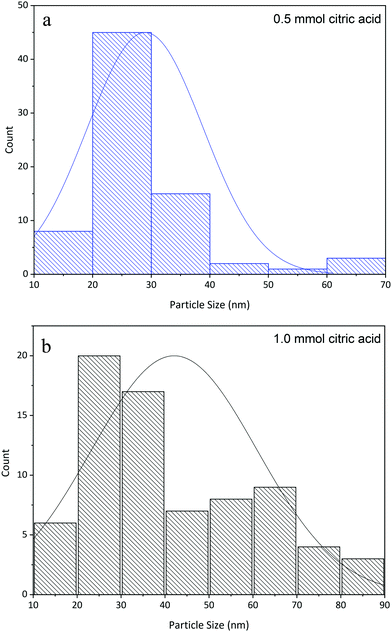 |
| Fig. 3 Particle size distribution for La2MnTiO6 nanospheres prepared using 0.5 mmol citric acid (a) and 1.0 mmol citric acid (b). | |
The TEM image of the prepared La2MnTiO6 double perovskite nanostructure in the presence of 0.5 mmol citric acid is shown in Fig. 4. It can be seen that the prepared sample possesses spherical morphology with size below 40 nm.
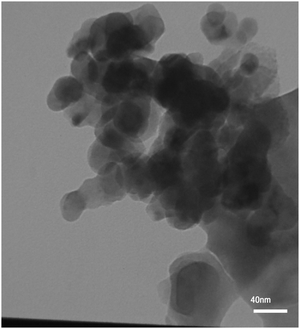 |
| Fig. 4 TEM image of the La2MnTiO6 nanostructure prepared using 0.5 mmol citric acid. | |
Elemental analysis of the prepared La2MnTiO6 was performed using EDX spectroscopy. Fig. 5 shows the EDX spectrum of the prepared sample. The elemental microanalysis discloses the presence of lanthanum, manganese, titanium, and oxygen in the prepared sample with relative amounts (wt%) of 62.38, 5.70, 4.12, and 27.80, respectively. There is no carbon and other elements as impurity, which reveals that the calcination temperature was sufficient for the elimination of organic solvent and citric acid. The element mapping of lanthanum, manganese, titanium, and oxygen are provided in Fig. S1–S4 (ESI†), respectively. All the elemental mapping images represent the uniform distribution of the sample components (La, Mn, Ti, and O) and confirm the objective of synthetic route of La2MnTiO6 double perovskite.
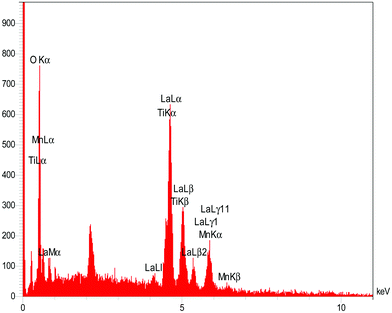 |
| Fig. 5 EDX spectrum of the La2MnTiO6 sample. | |
In order to identify the chemical groups on the surface of the prepared La2MnTiO6, FT-IR analysis was performed, as shown in Fig. 6. The FT-IR spectra represent O–H vibration at 3433 cm−1 and 1634 cm−1.23 Due to exposure of the prepared samples to ambient atmosphere, La-carbonate species are formed on the surface of the samples. The two bands at 1489 cm−1 and 1385 cm−1 are assigned to asymmetric stretching of carbonate groups.11,24 The characteristic peaks at nearly 620 cm−1 and 456 cm−1 could be attributed to Mn–O and Ti–O bonds, respectively.25,26
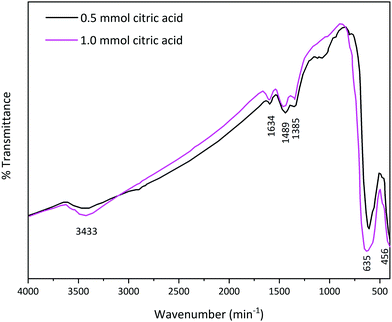 |
| Fig. 6 FT-IR spectra of La2MnTiO6 samples prepared using different amounts of citric acid. | |
The ability of a photocatalyst to absorb light is essential to obtain the highest photoactivity. Therefore, to investigate light absorption properties of the prepared La2MnTiO6 double perovskite nanostructure, UV-vis absorption spectra of the samples were studied. As shown in Fig. 7, La2MnTiO6 possesses broad absorption peak in the visible region from 700 nm to 400 nm. Meanwhile, the samples indicate considerable absorption in the UV region (400 nm to 300 nm). The optical band gap of the prepared samples were calculated by the following equation:
where
α is the coefficient of optical absorption,
h is the Planck's constant,
ν is the frequency of radiation, and
B is a constant dependent on the transition probability. As shown in the inset of
Fig. 7, by plotting (
αhν)
2versus hν, the optical band gap (
Eg) as calculated by extrapolation of the liner part of the spectra to the horizontal axis.
27,28 According to the result, the calculated optical band gap for the prepared La
2MnTiO
6 double perovskite nanostructure in the presence of two amounts of citric acid (0.5 mmol and 1.0 mmol) were 3.07 eV and 2.87 eV, respectively. The lower optical band gap value for the sample prepared with 1.0 mmol citric acid is attributed to the larger particle size for this sample (
Fig. 2 and 3).
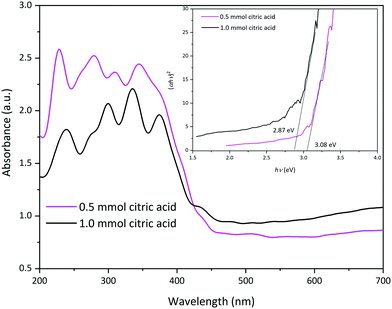 |
| Fig. 7 UV-vis diffuse reflectance spectra (DRS) of the La2MnTiO6 samples prepared using different amounts of citric acid. | |
3.2. Photocatalytic experiment of La2MnTiO6
According to the above results, it can be expected that the prepared La2MnTiO6 has excellent photocatalytic activity for the degradation of organic dyes. Therefore, the photocatalytic activity of the prepared sample was tested towards the degradation of acid blue 113 under visible light irradiation.
The photocatalytic activity of the prepared La2MnTiO6 samples are provided in Fig. 8. From Fig. 8, after 120 min illumination under visible light irradiation, the photocatalytic degradation of AB 113 is nearly 72.31% using the prepared La2MnTiO6 with 0.5 mmol citric acid. At the same time, the La2MnTiO6 photocatalyst prepared in the presence of 1.0 mmol citric acid was able to degrade AB 113 solution by 58.63%. This result could be clearly ascribed to more uniformity and smaller particle size of the prepared sample using 0.5 mmol citric acid. After the same time, the self-degradation of AB 113 without any photocatalyst is negligible.
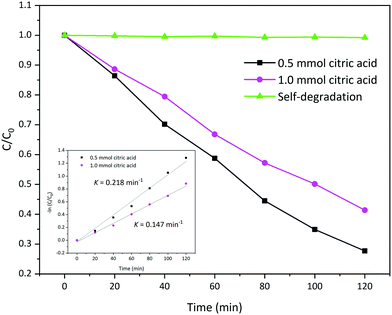 |
| Fig. 8 Photocatalytic degradation of AB 113 over La2MnTiO6 double perovskite nanostructures prepared using different amounts of citric acid. The inset shows the degradation kinetic curves using the prepared samples. | |
Moreover, the study of photocatalytic reaction kinetics, by plotting of −ln(C/C0) versus irradiation time, shows that the photocatalytic activity of the prepared La2MnTiO6 obeys first order reaction kinetics as follows:
|  | (3) |
where
C and
C0 are the concentrations of AB 113 after and before the photocatalytic reaction, respectively,
t is the irradiation time, and
k is the rate constant. The inset of
Fig. 8 shows the kinetics of photocatalytic degradation of AB 113 over the prepared La
2MnTiO
6 photocatalysts. The evaluation of the result indicates that the rate constants of photocatalytic degradation of AB 113 are 0.218 min
−1 and 0.147 min
−1 for the sample prepared with different amounts of citric acid (0.5 mmol and 1.0 mmol), respectively.
3.3. Effect of photocatalyst amount
In order to determine the optimum amount of the loaded photocatalyst, different amounts of La2MnTiO6 (prepared with 0.5 mmol citric acid) were dispersed into the AB 113 solution (50 mL, 50 ppm). Fig. 9 shows the photocatalytic activity of different amounts of La2MnTiO6 photocatalyst (0.01, 0.03, 0.05, and 0.07 g) loaded to fixed AB 113 solution. The photocatalytic degradation results indicate that the optimum amount of photocatalyst is 0.03 g and further increase in the amount of loaded photocatalyst has no considerable effect on the photocatalytic degradation of AB 113. The drastic reduction in the photocatalytic degradation of AB 113 could be clearly attributed to the decrease in light penetration into the dye solution in excess amount of the La2MnTiO6 photocatalyst.29
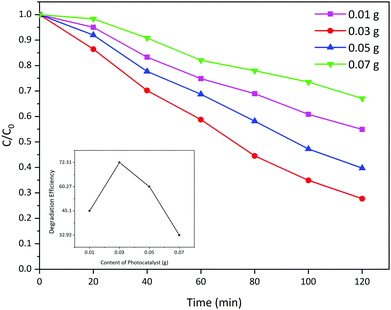 |
| Fig. 9 Photocatalytic degradation of AB 113 using different amounts of the La2MnTiO6 sample. The inset shows the efficiency of photocatalytic degradation of AB 113 using different amounts of the prepared sample. | |
3.4. Effect of pH on photocatalytic activity
The photocatalytic reaction is mainly a heterogeneous reaction, which proceeds on the surface of the photocatalyst. Therefore, the surface charges of the photocatalyst particles have a key role in the determination of photocatalytic activity of the photocatalyst, which in turn is affected by the pH of the dye solution.30Fig. 10 displays the photocatalytic activity of the La2MnTiO6 photocatalyst (prepared with 0.5 mmol citric acid) (0.03 g) towards the degradation of AB 113 solution (50 mL, 50 ppm) at various pH (3, 5, 7, 9, and 11).
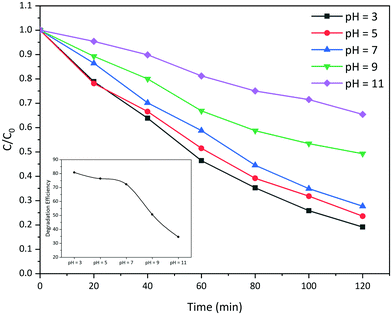 |
| Fig. 10 Photocatalytic degradation of AB 113 using La2MnTiO6 at different pH of the dye solution. The inset shows the efficiency of photocatalytic degradation of AB 113 at different pH. | |
As can be seen from Fig. 10, the photocatalytic degradation of AB 113 solution in acidic conditions is higher than that in alkaline conditions. This observation could be well demonstrated by the fact that AB 113 is an anionic double-azo dye;31 thus, the absorption of AB 113 molecules on the surface of positively charged photocatalyst particles is more achievable than on the negatively charged particles in alkaline solutions. In addition, the inset of Fig. 10 shows that the highest photoactivity of the prepared La2MnTiO6 is in the most acidic AB 113 solution (pH = 3).
3.5. Reusability experiment
One of the most important properties of a practical photocatalyst is its stability and reusability in several reaction cycles. In this regard, we conducted a reusability experiment for the prepared La2MnTiO6 (0.5 mmol citric acid) photocatalyst in 6 reaction cycles under the same conditions. After each photodegradation process, the photocatalyst particles were collected by centrifuging at 6000 rpm for 5 min and washed with D.I. water and ethanol, then dried at 70 °C for 4 hours.
Fig. 11 shows the reusability experiment and rate constant of the prepared photocatalyst for 6 reaction cycles. As shown in Fig. 11, the stability and photoactivity of the La2MnTiO6 nanostructure are high after 4 reaction cycles. However, the dissipation of photoactivity is obvious for 5th and 6th reaction cycles. In addition, the rate constant of photocatalytic degradation continuously decreased throughout the reusability experiments.
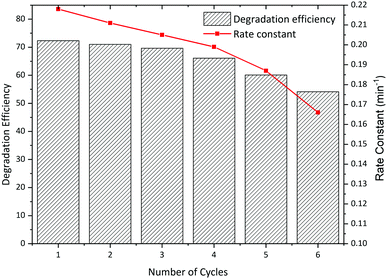 |
| Fig. 11 Reusability experiments of La2MnTiO6 nanostructure for photocatalytic degradation of AB 113. | |
3.6. Mechanism of photocatalytic degradation using La2MnTiO6 photocatalyst
The photocatalytic degradation of organic dyes is basically performed by the formation of highly reactive radicals (OH˙ and O2−˙) or by reactive photo-generated holes. In order to study the mechanism of photocatalytic activity of the prepared La2MnTiO6 and to find the dominant oxidant species in the photocatalytic reaction, we carried out photocatalytic experiments in the presence of some radical scavenging agents.
Fig. 12 shows the photocatalytic degradation of AB 113 (50 mL, 50 ppm) in the presence of tert-butanol,32 Na2-EDTA,32 and sodium azide33 as scavenging agents for hydroxyl radicals, photo-generated holes, and superoxide anion radicals, respectively. From the results, the photocatalytic activity of La2MnTiO6 using Na2-EDTA radical scavenging is lower than the photocatalytic reaction without the addition of any radical scavenging agents, which implies that the superior photocatalytic efficiency of the prepared La2MnTiO6 could clearly be attributed to the photo-generated holes. However, the photocatalytic degradation of AB 113 improved when we used tert-butanol and sodium azide. This observation is assigned to the enhanced efficiency of photo-generated charge carriers due to promotion of separation in them in the presence of tert-butanol and sodium azide.34
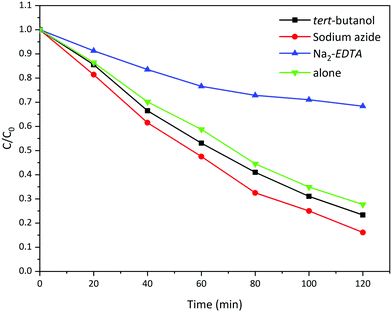 |
| Fig. 12 Photocatalytic activity of La2MnTiO6 in the presence of different radical scavenging agents. | |
4. Conclusions
We have presented the La2MnTiO6 double perovskite nanostructure as a highly effective photocatalyst towards the degradation of AB 131 under visible light irradiation. In this study, we used simple sol–gel method for the preparation of sample in the presence of citric acid as a size controlling agent. The prepared La2MnTiO6 nanostructure was characterized using TEM, FE-SEM-EDX mapping, XRD, FT-IR, and DRS analyses. The investigation of La2MnTiO6 nanostructure disclosed that the prepared sample possesses orthorhombic structure with a nanospherical morphology.
The visible light photocatalytic degradation of AB 113 was carried out using La2MnTiO6 double perovskite nanostructures. After studying the mechanism of photocatalytic degradation over La2MnTiO6, it was found that photocatalytic reaction is predominant with photo-generated holes. Also, in this study we investigate the effect of photocatalyst amount and pH of dye solution to find the optimum conditions for photocatalytic reaction. The reusability experiments using La2MnTiO6 double perovskite nanostructures revealed that the photoactivity of the prepared sample is high for 4 reaction cycles.
Conflicts of interest
We have no conflicts of interest to disclose.
Acknowledgements
The authors would like to acknowledge the financial support of this work by the University of Kashan, Iran.
References
- Z. Xing, J. Zhang, J. Cui, J. Yin, T. Zhao, J. Kuang, Z. Xiu, N. Wan and W. Zhou, Recent advances in floating TiO2-based photocatalysts for environmental application, Appl. Catal., B, 2018, 225, 452–467 CrossRef CAS.
- A. K. Verma, R. R. Dash and P. Bhunia, A review on chemical coagulation/flocculation technologies for removal of colour from textile wastewaters, J. Environ. Manage., 2012, 93, 154–168 CrossRef CAS.
- M. Behpour, P. Shirazi and M. Rahbar, Immobilization of the Fe2O3/TiO2 photocatalyst on carbon fiber cloth for the degradation of a textile dye under visible light irradiation, React. Kinet., Mech. Catal., 2019, 127, 1073–1085 CrossRef CAS.
- M. Pelaez, N. T. Nolan, S. C. Pillai, M. K. Seery, P. Falaras, A. G. Kontos, P. S. M. Dunlop, J. W. J. Hamilton, J. A. Byrne, K. O'Shea, M. H. Entezari and D. D. Dionysiou, A review on the visible light active titanium dioxide photocatalysts for environmental applications, Appl. Catal., B, 2012, 125, 331–349 CrossRef CAS.
- R. Daghrir, P. Drogui and D. Robert, Modified TiO2 for environmental photocatalytic applications: a review, Ind. Eng. Chem. Res., 2013, 52, 3581–3599 CrossRef CAS.
- W. Choi, A. Termin and M. R. Hoffmann, The role of metal ion dopants in quantum-sized TiO2: correlation between photoreactivity and charge carrier recombination dynamics, J. Phys. Chem., 1994, 98, 13669–13679 CrossRef.
- H. Irie, Y. Watanabe and K. Hashimoto, Carbon-doped anatase TiO2 powders as a visible-light sensitive photocatalyst, Chem. Lett., 2003, 32, 772–773 CrossRef CAS.
- S. J. Singh and R. V. Jayaram, Oxidation of alkylaromatics to benzylic ketones using TBHP as an oxidant over LaMO3 (M = Cr, Co, Fe, Mn, Ni) perovskites, Catal. Commun., 2009, 10, 2004–2007 CrossRef CAS.
- Y. Mao, Facile molten-salt synthesis of double perovskite La2BMnO6 nanoparticles, RSC Adv., 2012, 2, 12675–12678 RSC.
- Z. Yang, L. Yang, C. Ji, D. Xu, C. Zhang, H. Bu, X. Tan, X. Yun and J. Sun, Studies on luminescence properties of double perovskite deep red phosphor La2ZnTiO6:Mn4+ for indoor plant growth LED applications, J. Alloys Compd., 2019, 802, 628–635 CrossRef CAS.
- K. Peng, L. Fu, H. Yang and J. Ouyang, Perovskite LaFeO3/montmorillonite nanocomposites: synthesis, interface characteristics and enhanced photocatalytic activity, Sci. Rep., 2016, 6, 19723 CrossRef CAS PubMed.
- H. Sabeeh, S. Musaddiq, M. Shahid, M. A. Khan, M. Sher and M. F. Warsi, Rare earth substituted nanocrystalline LaFeO3 perovskites and their composites with reduced graphene oxide for enhanced photocatalytic and other potential applications, Mater. Res. Express, 2018, 5, 065062 CrossRef.
- Y. Bai, Y. Xia, H. Li, L. Han, Z. Wang, X. Wu, S. Lv, X. Liu and J. Meng, A-Site-Doping Enhanced B-Site Ordering and Correlated Magnetic Property in La2−xBix CoMnO6, J. Phys. Chem. C, 2012, 116, 16841–16847 CrossRef CAS.
- D. Choudhury, A. Hazarika, A. Venimadhav, C. Kakarla, K. T. Delaney, P. S. Devi, P. Mondal, R. Nirmala, J. Gopalakrishnan and N. A. Spaldin, Electric and magnetic polarizabilities of hexagonal Ln2CuTiO6 (Ln = Y, Dy, Ho, Er, and Yb), Phys. Rev. B: Condens. Matter Mater. Phys., 2010, 82, 134203 CrossRef.
- M. Ghiasi and A. Malekzadeh, Solar photocatalytic degradation of methyl orange over La0.7Sr0.3MnO3 nano-perovskite, Sep. Purif. Technol., 2014, 134, 12–19 CrossRef CAS.
- S. Feraru, A. I. Borhan, P. Samoila, C. Mita, S. Cucu-Man, A. R. Iordan and M. N. Palamaru, Development of visible-light-driven Ca2Fe1−xSmxBiO6 double perovskites for decomposition of rhodamine 6G dye, J. Photochem. Photobiol., A, 2015, 307–308, 1–8 CrossRef CAS.
- J. Luan, M. Li, K. Ma, Y. Li and Z. Zou, Photocatalytic activity of novel Y2InSbO7 and Y2GdSbO7 nanocatalysts for degradation of environmental pollutant rhodamine B under visible light irradiation, Chem. Eng. J., 2011, 167, 162–171 CrossRef CAS.
- M. Valian, F. Beshkar and M. Salavati-Niasari, Urchin-like Dy2CoMnO6 double perovskite nanostructures: new simple fabrication and investigation of their photocatalytic properties, J. Mater. Sci.: Mater. Electron., 2017, 28, 12440–12447 CrossRef CAS.
- M. R. Palacín, J. Bassas, J. Rodríguez-Carvajal and P. Gómez-Romero, Syntheses of the perovskite La2CuTiO6 by the ceramic, oxide precursors and sol–gel methods, and study of the structure and Cu–Ti distribution by X-ray and neutron diffraction, J. Mater. Chem., 1993, 3, 1171–1177 RSC.
- J. Krishna Murthy and A. Venimadhav, Magnetodielectric behavior in La2CoMnO6 nanoparticles, J. Appl. Phys., 2012, 111, 024102 CrossRef.
- H. Khalilian, M. Behpour, V. Atouf and S. N. Hosseini, Immobilization of S, N-codoped TiO2 nanoparticles on glass beads for photocatalytic degradation of methyl orange by fixed bed photoreactor under visible and sunlight irradiation, Sol. Energy, 2015, 112, 239–245 CrossRef CAS.
- V. Uvarov and I. Popov, Metrological characterization of X-ray diffraction methods at different acquisition geometries for determination of crystallite size in nano-scale materials, Mater. Charact., 2013, 85, 111–123 CrossRef CAS.
- P. M. Kumar, S. Badrinarayanan and M. Sastry, Nanocrystalline TiO2 studied by optical, FTIR and X-ray photoelectron spectroscopy: correlation to presence of surface states, Thin Solid Films, 2000, 358, 122–130 CrossRef CAS.
- S. Thirumalairajan, K. Girija, I. Ganesh, D. Mangalaraj, C. Viswanathan, A. Balamurugan and N. Ponpandian, Controlled synthesis of perovskite LaFeO3 microsphere composed of nanoparticles via self-assembly process and their associated photocatalytic activity, Chem. Eng. J., 2012, 209, 420–428 CrossRef CAS.
-
M. Mylarappa, V. V. Lakshmi, K. V. Mahesh, H. Nagaswarupa and N. Raghavendra, A facile hydrothermal recovery of nano sealed MnO2 particle from waste batteries: An advanced material for electrochemical and environmental applications, IOP Conference Series: Materials Science and Engineering, IOP Publishing, 2016, p. 012178.
- M. Viana, V. Soares and N. Mohallem, Synthesis and characterization of TiO2 nanoparticles, Ceram. Int., 2010, 36, 2047–2053 CrossRef CAS.
- A. Abdul-Kader, Modification of the optical band gap of polyethylene by irradiation with electrons and gamma rays, Philos. Mag. Lett., 2009, 89, 162–169 CrossRef CAS.
- H. Eskandarloo, A. Badiei and M. A. Behnajady, TiO2/CeO2 hybrid photocatalyst with enhanced photocatalytic activity: optimization of synthesis variables, Ind. Eng. Chem. Res., 2014, 53, 7847–7855 CrossRef CAS.
- S. Anandan, P. S. Kumar, N. Pugazhenthiran, J. Madhavan and P. Maruthamuthu, Effect of loaded silver nanoparticles on TiO2 for photocatalytic degradation of Acid Red 88, Sol. Energy Mater. Sol. Cells, 2008, 92, 929–937 CrossRef CAS.
- X. Van Doorslaer, K. Demeestere, P. M. Heynderickx, H. Van Langenhove and J. Dewulf, UV-A and UV-C induced photolytic and photocatalytic degradation of aqueous ciprofloxacin and moxifloxacin: reaction kinetics and role of adsorption, Appl. Catal., B, 2011, 101, 540–547 CrossRef CAS.
- V. K. Gupta, B. Gupta, A. Rastogi, S. Agarwal and A. Nayak, A comparative investigation on adsorption performances of mesoporous activated carbon prepared from waste rubber tire and activated carbon for a hazardous azo dye—Acid Blue 113, J. Hazard. Mater., 2011, 186, 891–901 CrossRef CAS PubMed.
- J. Fu, Y. Tian, B. Chang, F. Xi and X. Dong, BiOBr–carbon nitride heterojunctions: synthesis, enhanced activity and photocatalytic mechanism, J. Mater. Chem., 2012, 22, 21159–21166 RSC.
- M. Amini, M. Ashrafi, S. Gautam and K. H. Chae, Rapid oxidative degradation of methylene blue by various metal oxides doped with vanadium, RSC Adv., 2015, 5, 37469–37475 RSC.
- Y. Tian, B. Chang, J. Lu, J. Fu, F. Xi and X. Dong, Hydrothermal Synthesis of Graphitic Carbon Nitride–Bi2WO6 Heterojunctions with Enhanced Visible Light Photocatalytic Activities, ACS Appl. Mater. Interfaces, 2013, 5, 7079–7085 CrossRef CAS PubMed.
Footnote |
† Electronic supplementary information (ESI) available. See DOI: 10.1039/c9nj04932k |
|
This journal is © The Royal Society of Chemistry and the Centre National de la Recherche Scientifique 2020 |