DOI:
10.1039/C9NJ04511B
(Paper)
New J. Chem., 2020,
44, 194-199
Can the HRP–ACAC binary system initiate acrylamide polymerisation?
Received
1st September 2019
, Accepted 21st November 2019
First published on 26th November 2019
Abstract
In this communication, acrylamide (AM) polymerisation to poly(acrylamide) was initiated by horseradish peroxidase (HRP) and acetylacetone (ACAC) without hydrogen peroxide (H2O2). Electron spin resonance (ESR) spectroscopy showed that free radicals of ACAC and AM were present in the reaction. Gel Permeation Chromatography (GPC), FT-IR, 1H-NMR and relative viscosity indicated that the product was PAM. Furthermore, the enzymatic cycling mechanism of the HRP–ACAC–H2O2 ternary system was also explained.
Introduction
In the last few decades, new methods of acrylamide (AM) polymerisation have been continuously explored. Initiators such as azobisisobutyronitrile,1,2 benzoyl peroxide3,4 and persulphate5,6 are commonly used for polymerisation of AM. Reversible addition–fragmentation chain transfer (RAFT) was used to achieve controllable and rapid synthesis of poly(acrylamide) (PAM). However, the toxicity and environmental pollution of traditional initiators greatly limit their application. Therefore, enzymes are increasingly used for the polymerization of vinyl monomers by virtue of their high selectivity to specific functional groups and their environmental friendliness.7 Enzymatic controlled radical polymerisation is a typical example of the application of enzymes in controlled polymerisation.8–10
Horseradish peroxidase (HRP) has high substrate specificity and extremely high catalytic efficiency under mild catalytic conditions. Therefore, HRP catalysed polymerisation of AM has several advantages compared with other polymerisation methods. HRP, obtained from renewable resources, can improve the conversion rate of the target substrate, because of its high selectivity to specific functional groups. HRP-catalysed AM polymerisation avoids the use of organic solvents and toxic catalysts.
HRP was first used for initiating AM polymerisation along with H2O2.11 In this experiment, PAM was obtained using a large amount of H2O2 and without the use of acetylacetone (ACAC). However, other researchers could not obtain PAM under the same experimental conditions. β-diketone was found to be the key substrate, when the HRP/H2O2/β-diketone ternary system was used to initiate AM polymerisation.12–16 This was also verified by Uyama, who used HRP to initiate the polymerisation of methyl methacrylate in water at room temperature.17,18 Many experimental studies have concluded that HRP, ACAC and H2O2 are all indispensable as initiators for AM polymerisation. Cai also proved experimentally that neither of the two initiator combinations can initiate AM polymerisation.19
The mechanism of the HRP ternary initiation system is shown in Fig. 1 red.20 Structurally, HRP consists of a protoporphyrin ring with an iron centre. HRP brings about the splitting of H2O2 molecules to form ([(–Fe(III)–)H+][˙O2H]) transition states. Then, a two-electron oxidation reaction occurs at the active site of the enzyme resulting in the formation of HRP-I and H2O. HRP-I undergoes a single electron redox reaction with acetylacetone producing two types of free radicals of ACAC (carbon free radical and alkoxyl radical) and HRP-II. HRP-II is then restored to its original state by a similar single electron redox reaction. Two carbon radicals are generated in one enzymatic cycle. In theory, this method can be used to generate primary free radicals to initiate the addition polymerisation of vinyl monomers. However, Berglund proposed that HRP reacts with a reductive substrate to form ferrous enzyme without the addition of H2O2 (Fig. 1 blue).20 So far, this theory has not been applied in experiments. Can the HRP–ACAC binary system initiate the polymerisation of acrylamide in the absence of H2O2? If it can be proved experimentally that HRP/ACAC initiates AM polymerisation, then it can meaningfully explain the mechanism of HRP enzyme catalysis. A possible reaction was proposed as shown in Table 1.
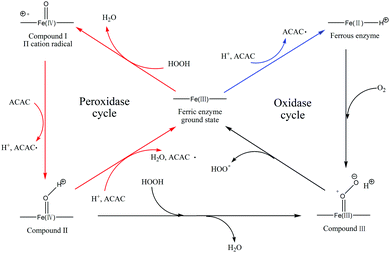 |
| Fig. 1 The cyclic mechanism of formation of intermediaries in the HRP initiation system. | |
Table 1 The process of AM polymerization initiated by the HRP/ACAC binary system
No. |
Reaction |
1 |
HRP + H+ + ACAC → Ferrous enzyme + ACAC˙ |
2 |
ACAC˙ + AM → AM˙ |
3 |
nAM˙ → PAM |
Polymerisation of AM by HRP initiation can be divided into several stages (initiation, growth, transfer and termination), similar to other initiation systems of multicomponent free radical polymerisations.14 Polymerisation kinetics is an effective method to study the polymerisation mechanism. The rate equation for polymerisation was obtained from the time graph of transformation to study the polymerisation mechanism.19 Furthermore, electron spin resonance (ESR) spectroscopy is also an important tool for exploring this mechanism. Free radicals present in the reaction system can be captured by the capture agent and detected by ESR. Free radicals were captured by α-(4-pyridyl-1-oxide)-N-tert-butylnitrone (POBN) and detected by ESR while studying the mechanism of AM polymerisation initiated by the manganese peroxidase/H2O2/ACAC ternary system.21 It was found that first ACAC radicals were formed in the ternary initiation system, which further induced acrylamide to generate radicals and polymerise. The site of formation of free radicals as well as the species can be detected by ESR. Ikkai could determine the free radicals produced in UV-induced gelation of acryloyl-type monomer and polymer solutions.22 ESR data revealed that a UV-induced cross-linking reaction occurred at the α-carbon atoms in the acryloyl-type monomers and polymers.
In this report, the ESR technique was used for the detection of different types of free radicals in the HRP enzymatic initiation system, which clearly suggested a cyclic enzymatic mechanism. While studying this mechanism, it became evident that the HRP–ACAC initiation system could also produce ACAC radicals without the addition of H2O2. This was further verified by conducting the AM polymerisation with the HRP–ACAC initiator system. It was found that PAM could also be generated without the addition of H2O2.
Materials and methods
Horseradish peroxidase (HRP) (>300 units mg−1), 5,5-dimethyl-1-pyrroline N-oxide (DMPO) (97%), acetylacetone (ACAC) (≥99.6%) and acrylamide (AM) (99.9%) were purchased from Aladdin Chemical Company (Shanghai, China). POBN (>98.0%) was purchased from TCI Chemical Company (Shanghai, China). H2O2 (≥30%) was purchased from Sinopharm Chemical Reagent Company (Shanghai, China).
The ESR spectra were recorded on a JES-FR30 JEOL spectrometer (JEOL, Japan) that operated at 9.425 GHz and 100 kHz modulation frequency using DMPO and POBN as capture agents. The types of free radicals that these two capture agents can capture are shown in Table 2. 1H-nuclear magnetic resonance (NMR) spectroscopic analysis of the polymer in D2O solvent was conducted using a JNM-LA400MK instrument (JEOL, Japan). The structure of the polymer was also characterised by infrared analysis using a NICOLET560 Fourier transform infrared spectrometer. The powdered polymer was mixed with KBr powder and compressed into a pellet for analysis. The real-time viscosity of the reaction solution was measured at 40 min intervals using an Ubbelohde viscometer.
Table 2 Types of free radicals that the two capture agents can capture
|
C˙ |
HO˙ |
RO˙ |
DMPO
|
✓ |
✓ |
✓ |
POBN
|
✓ |
✗ |
✗ |
The chromatograph system (Shimadzu Corp., Tokyo, Japan) contained an LC-20AT pump, a SIL-10A injection valve with a 10 mL loop and RID-10A differential refractive index detector (Shimadzu Corp., Tokyo, Japan). The analysis of the BLD was achieved on a Shodex OHpak SB-804 HQ (8.0 × 300 mm, i.d. 6 mm) column (Shodex China Co., Ltd, Shanghai, China). The molecular weights of the products were recorded on a Waters Alliance e2695 GPC system using water as the eluent and glucan as the standard at a flow rate of 1.0 1.0 mL min−1 after the samples were dissolved in water at a concentration of 4.0 mg mL−1 and passed through microfilters with an average pore size of 0.2 μm.
During the reaction initiated by HRP/ACAC/H2O2, dissolved oxygen in the solution was flushed with N2 until the end of the reaction. Polymerisation was started by adding H2O2 solution (0.5 mL, 40 mM) (no addition in the reaction initiated by HRP/ACAC) to a 20 mL solution containing HRP (3750 units), 20 mM phosphate buffer solution (pH 6.0), 0.17 mM acetylacetone and 700 mM acrylamide (acrylic acid and methyl acrylate). The reaction was maintained at 35 °C for 4 h. Subsequently, the reaction mixture was precipitated by 2 times the volume of absolute alcohol. The precipitate formed was collected by filtration, washed with ethanol and dried under vacuum at 45 °C. DMPO and POBN were added to the solution before the reaction began. The free radicals generated in the reaction were captured by DMPO and POBN to form adducts for ESR detection (Fig. 2).
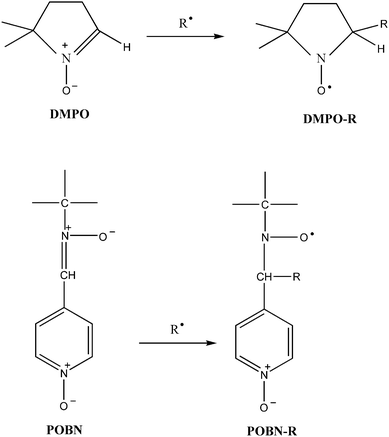 |
| Fig. 2 Spin trapping of the free radical R by DMPO and POBN spin traps to form DMPO-R and POBN-R adducts. | |
Results and discussion
Types of free radicals in the HRP/ACAC/H2O2 system
To investigate whether HRP–ACAC could initiate the AM polymerisation, the free radicals in the HRP–ACAC–H2O2 ternary system and HRP–ACAC binary system should be determined. Free radicals in the HRP–ACAC–H2O2 ternary system were captured by DMPO and detected by ESR. The ESR spectra are shown in Fig. 3. (A) is the test spectrum, (B) is the fitted spectrum obtained by fitting the test spectrum, and (C–E) are the characteristic peaks present in the fitted spectrum. (C) shows the characteristic spectrum of carbon radicals and the hyperfine splitting constants of the adduct were αN = 14.3, αHβ = 21.08, and g = 2.0059. The hyperfine splitting constants of alkoxy radicals (D) were αN = 13.15, αHβ = 7.45, and g = 2.0059. The hyperfine splitting constants of hydroxyl radicals (E) were αN = 14.80, αHβ = 14.80, and g = 2.0059. In Fig. 3, the characteristic peaks of carbon radicals were marked by “*”, whereas the characteristic peaks of hydroxyl radicals were marked by “o”. Both these peaks appeared in the fitted spectrum individually. The other characteristic peaks appeared in the fitted spectrum by superimposition. Hydroxyl radicals were produced from H2O2. It is known that the ternary initiation system contains two types of acetylacetone radicals (C˙ and RO˙). This was totally consistent with the types of free radicals in the HRP–ACAC–H2O2 cycle (Fig. 1 red). The next step would be to explore whether the free radicals also exist in the binary initiation system and induce acrylamide to produce free radicals.
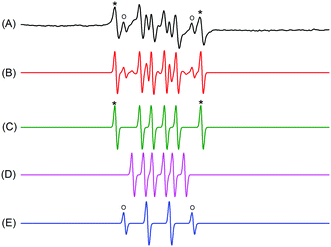 |
| Fig. 3 ESR spectrum and the fitted spectrum of the DMPO radical adduct formed in the HRP–ACAC–H2O2 initiation system. (A) Original spectrum, (B) fitting spectrum, (C) characteristic spectrum of carbon radicals, (D) characteristic spectrum of alkoxy radicals, and (E) characteristic spectrum of hydroxyl radicals. | |
Types of free radicals in the HRP–ACAC binary systems
In order to investigate whether free radicals existed in these three binary initiation systems, three combinations of HRP, ACAC and H2O2 as binary systems were chosen. The free radicals in these three groups were captured by POBN and detected by ESR spectroscopy (Fig. 3). In Fig. 4, free radicals were detected in the HRP–ACAC system. The ESR spectrum showed a doublet of triplet characteristic peaks of the acetylacetone radical, with hyperfine splitting constants of αN = 14.3 G, αHβ = 1.6 G and g = 2.0044. However, no free radicals were detected in the HRP–H2O2 and H2O2–ACAC systems. This suggested that the free radicals of acetylacetone could also be produced in an initiation system, without the participation of hydrogen peroxide. However, this was inconsistent with the previous reports.19 To verify this further, polymerisation of acrylamide was conducted using the HRP–ACAC initiation system. The free radicals in the reaction were captured by POBN for ESR spectral analysis (Fig. 5(C)). The reaction system contained not only ACAC radicals but also AM radicals.21 Thus, it was demonstrated that the HRP–ACAC system could also induce acrylamide to generate free radicals. It is necessary to further investigate the mechanism of AM polymerisation initiated by HRP–ACAC.
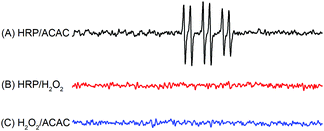 |
| Fig. 4 ESR spectrum of the POBN radical adduct formed in the binary initiation systems. (A) HRP/ACAC, (B) HRP/H2O2, and (C) ACAC/H2O2. | |
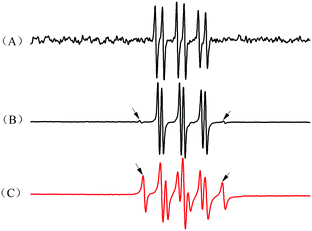 |
| Fig. 5 ESR spectra of POBN radical adducts formed during AM polymerisation initiated by HRP–ACAC. (A) Without added AM, (B) with added AM after 15 min of the reaction, and (C) with added AM after 4 h of the reaction. | |
ESR analysis of AM polymerisation initiated by HRP–ACAC
ESR study demonstrated that the addition of ACAC promoted the polymerisation of acrylamide in the peroxidation of horseradish peroxidase to lipids. It indicated that the polymerisation of acrylamide started with the formation of free radicals. 14, 15POBN was used to capture the free radicals in the AM polymerisation. The POBN adducts were detected by ESR to analyse the changes in free radical species (Fig. 5). When no AM was added, the ultrafine splitting constants of the POBN adduct were αN = 14.3 G, αHβ = 1.6 G and g = 2.0044. It indicated that this spectrum was a doublet of triplet peaks of carbon radicals generated by acetylacetone (Fig. 5(A)). An additional new peak (shown by black arrow in Fig. 4(B)) appeared in the ESR spectrum after the addition of acrylamide to the reaction system after 15 min. The hyperfine splitting constants of the new peak were αN = 15.3 G, αHβ = 2.8 G and g = 2.0056. After 4 h of reaction, the intensity of the new peak increased, and the intensity of the carbon radical generated by acetylacetone decreased (Fig. 5(C)). The appearance of the new peak is similar to that previously report.21 This proved that the carbon radicals of acetylacetone produced in the system promoted the polymerisation of acrylamide.
Characterization of products produced by HRP/ACAC/AM
FT-IR and 1H-NMR spectroscopic analyses characterised the structure of the product of AM polymerisation, initiated by HRP–ACAC. Fig. 6 shows the infrared spectra of the products. The peaks of C–H bonds of acrylamide (which appeared at 3035 cm−1 and 3096 cm−1) in the range of 3000 to 3100 cm−1. The reaction products did not show these two characteristic peaks. The peak at 2934 cm−1 was characteristic of methylene anti-symmetric stretching vibration. The peak at 2852 cm−1 was characteristic of methylene symmetric stretching vibration. The absorption peak of methylene deformation appeared at 1453 cm−1. These three peaks were all unique to PAM, and were absent in AM. As shown in Fig. 8, peaks 1 and 2 located between δ 5.6–6.4 were of olefinic hydrogen of AM (Fig. 8(A)). Peaks 3 and 4 were attributed to methylene protons, the appearance of which proved that the product was PAM. As the reaction time increases, the signal of the characteristic peak of the products also became strong (Fig. 8(B)–(D)). And Fig. 6(A) and Fig. 8(A) also proved that HRP/H2O2 and H2O2/ACAC cannot initiate the AM polymerisation. The GPC curves of the product were correlated with the changes in relative viscosity of the reaction solution (Fig. 10). The relative viscosity of the reaction mixture gradually increased with the passage of time, which could be due to a gradual increase in relative molecular weight of the polymer in the reaction. The Mn and Mw, which increased in proportion to the reaction time, were much higher than the molecular weight of AM (71.08). These results demonstrated that HRP–ACAC could initiate the AM polymerisation without the addition of H2O2. In order to prove that acrylamide is not a special monomer which can be initiated by HRP/ACAC, acrylic acid and methyl acrylate were used to be initiated by HRP/ACAC. And the products were detected by FT-IR and 1H-NMR (Fig. 7 and Fig. 9). As shown in Fig. 7, 1722 cm−1 is the stretching vibration peak of C
O in polymethyl acrylate and 1580 cm−1 is the stretching vibration peak of C
O in polyacrylic acid. And the characteristic peaks (Fig. 9 (1–5)) also showed that HRP/ACAC can initiate the polymerisation of AA and MA.
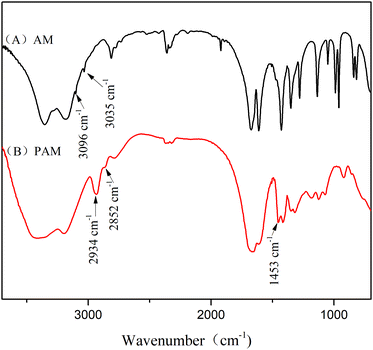 |
| Fig. 6 FT-IR spectra of the products. (A) Initiated by HRP/H2O2 and H2O2/ACAC, and (B) initiated by HRP/ACAC. | |
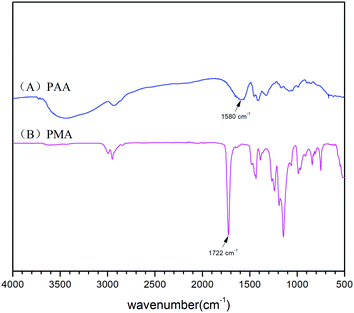 |
| Fig. 7 FT-IR spectra of the products initiated by HRP/ACAC. (A) PAA, and (B) PMA. | |
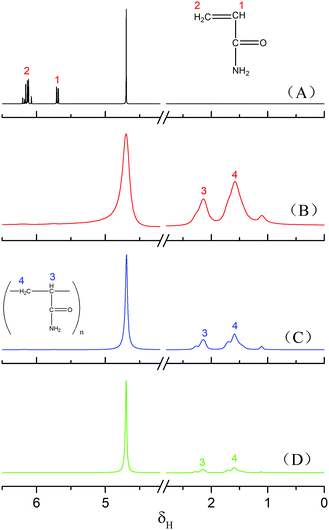 |
| Fig. 8
1H-NMR spectra of the products. (A) Initiated by HRP/H2O2 and H2O2/ACAC, (B) after 4 h of the reaction initiated by HRP/ACAC, (C) after 2 h of the reaction initiated by HRP/ACAC, and (D) after 1 h of the reaction initiated by HRP/ACAC. | |
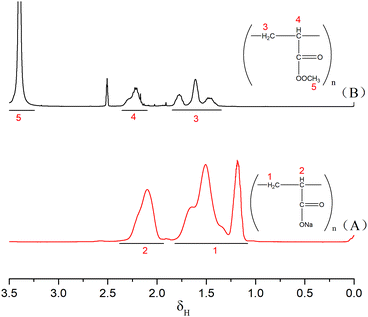 |
| Fig. 9
1H-NMR spectra of the products initiated by HRP/ACAC. (A) PAA, and (B) PMA. | |
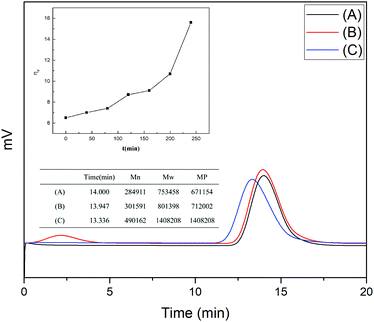 |
| Fig. 10 GPC spectra of the products initiated by HRP/ACAC and the change in the relative viscosity of the reaction liquid. (A) After 1 h of the reaction, (B) after 2 h of the reaction, and (C) after 4 h of the reaction. | |
Conclusions
Free radicals produced in the HRP/ACAC/H2O2 ternary initiation system were captured for ESR testing by DMPO. The ESR spectral analysis showed that alkoxy radicals, carbon radicals and hydroxyl radicals were present in the initiation system. This finding was consistent with the cyclic enzymatic mechanism, which was proposed. It also demonstrated the mechanism of the enzyme cycle, in terms of free radical species. At the same time, it also proved that acetylacetone radicals were also produced in the HRP/ACAC binary system without the addition of H2O2, which further induced acrylamide to generate free radicals. Therefore, free radicals in the AM polymerisation initiated by HRP–ACAC were captured for ESR analysis by POBN. The acetylacetone radicals were first produced in the initiation system, which further induced acrylamide to generate free radicals. Through a series of characterizations of the product, such as FT-IR, 1H-NMR, GPC and the relative viscosity, it was proved that HRP–ACAC could also initiate AM polymerisation without the addition of H2O2. This finding is inconsistent with the existing conclusions from the literature and the cyclic enzymatic mechanism of HRP–ACAC needs to be further researched.
Conflicts of interest
There are no conflicts to declare.
Acknowledgements
This work was supported by the National Natural Science Foundation of China (No. 21674043), the Fundamental Research Funds for the Central Universities (JUSRP51717A) and the Postgraduate Research & Practice Innovation Program of Jiangsu Province (KYCX17_1436).
References
- B. Ray and B. M. Mandal, J. Polym. Sci., Part A: Polym. Chem., 1999, 37, 493–499 CrossRef CAS.
- M. Celik and M. Sacak, J. Appl. Polym. Sci., 1996, 59, 609–617 CrossRef CAS.
- O. Sanli and E. Pulat, J. Appl. Polym. Sci., 1993, 47, 1–6 CrossRef CAS.
- R. Coskun, M. Sacak and M. Karakisla, Appl. Polym. Sci., 2005, 97, 1795–1803 CrossRef CAS.
- D. Hunkeler, Macromolecules, 1991, 24, 2160–2171 CrossRef CAS.
- H. R. Lin, Eur. Polym. J., 2001, 37, 1507–1510 CrossRef CAS.
- S. J. Sigg, F. Seidi, K. Renggli, T. B. Silva, G. Kali and N. Bruns, Macromol. Rapid Commun., 2011, 32, 1710–1715 CrossRef CAS PubMed.
- B. Zhang, X. Wang, A. Zhu, K. Ma, Y. Lv, X. Wang and Z. An, Macromolecules, 2005, 46, 3515–3521 Search PubMed.
- A. P. Danielson, D. B. Van Kuren, M. E. Lucius, K. Makaroff, C. Williams, R. C. Page, J. A. Berberich and D. Konkolewicz, Macromol. Rapid Commun., 2016, 37, 362–367 CrossRef CAS PubMed.
- S. J. Sigg, F. Seidi, K. Renggli, T. B. Silva, G. Kali and N. Bruns, Macromol. Rapid Commun., 2011, 32, 1710–1715 CrossRef CAS PubMed.
- R. A. Derango, L. C. Chiang, R. Dowbenko and J. G. Lasch, Biotechnol. Tech., 1992, 6, 523–526 CrossRef CAS.
- A. Durand, T. Lalot, M. Brigodiot and E. Marechal, Polymer, 2000, 41, 8183–8192 CrossRef CAS.
- O. Emery, T. Lalot, M. Brigodiot and E. Marechal, J. Polym. Sci., Part A: Polym. Chem., 1997, 35, 3331–3333 CrossRef CAS.
- D. Teixeira, T. Lalot, M. Brigodiot and E. Marechal, Macromolecules, 1999, 32, 70–72 CrossRef CAS.
- T. Lalot, M. Brigodiot and E. Marechal, Polym. Int., 1999, 48, 288–292 CrossRef CAS.
- A. Durand, T. Lalot, M. Brigodiot and E. Marechal, Polymer, 2001, 42, 5515–5521 CrossRef CAS.
- H. Uyama, C. Lohavisavapanich, R. Ikeda and S. Kobayashi, Macromolecules, 1998, 31, 554–556 CrossRef CAS.
- R. Ikeda, H. Tanaka, H. Uyama and S. Kobayashi, Macromol. Rapid Commun., 1998, 19, 423–425 CrossRef CAS.
- Z. Q. Cai, J. Z. Sun, Q. Y. Zhou and P. Zhang, J. Funct. Polym., 2003, 8, 15 Search PubMed.
- G. I. Berglund, G. H. Carlsson, A. T. Smith, H. Szoke, A. Henriksen and J. Hajdu, Nature, 2002, 417, 463–468 CrossRef CAS PubMed.
- K. Iwahara, M. Hirata, Y. Honda, T. Watanabe and M. Kuwahara, Biotechnol. Lett., 2000, 22, 1355–1361 CrossRef CAS.
- F. Ikkai and E. Adachi, Macromol. Chem. Phys., 2010, 208, 271–276 CrossRef.
|
This journal is © The Royal Society of Chemistry and the Centre National de la Recherche Scientifique 2020 |