DOI:
10.1039/C9GC03692J
(Paper)
Green Chem., 2020,
22, 256-264
Multi-component solvent-free cascade reaction of 2-cyanoacetamides: regioselective synthesis of pyridin-2-ones bearing quaternary centers†
Received
28th October 2019
, Accepted 24th November 2019
First published on 25th November 2019
Abstract
A novel protocol has been constructed for the synthesis of four types of pyridin-2-ones via solvent-free cascade reactions of 2-cyanoacetamides, various ketones, and acetone via multicomponent reactions to prepare target compounds with good to excellent yields. As a result, functionalized pyridin-2-ones bearing quaternary centers, specifically, spiropyridin-2-ones, were generated by piperidine under solvent-free conditions. Also, a double bond has been introduced into the target compounds through aryl substituted 2-cyanoacetamides under the same conditions. The advantages of this approach include no solvents, simple and practical operation (multi-component one-pot), high yields (up to 95%), and a product with potential biological activity.
Introduction
The chemical industry is known to affect the entire ecological environment. With this, more policies have been developed in favor of sustainable chemical development.1 Green chemistry encompasses all aspects and types of chemical processes that reduce negative impacts on human health and the environment.2 Among them, seeking solvent-free conditions instead of traditional organic solvents is a crucial aspect.3 Also, multicomponent reactions (MCRs)4 are an alternative approach that can effectively avoid tedious separation and purification operation and the use of a large number of solvents and eluents. This approach can also maximize the efficiency of the synthesis of the same molecular skeleton with molecular diversity of the compound library to meet the needs of drug screening.
Pyridine derivatives are not only common moieties in natural products and drug molecules but also play important roles in functional materials, coordination chemistry, and organic catalysis. Pyridines have been widely used in organic and pharmaceutical chemistries.5–8 Among them, pyridin-2-ones have been used because pyridin-2-one contains an important heterocyclic skeleton structure and widely exists in numerous synthetic and natural products possessing a broad range of biological activities including antitumor9 (Fig. 1, A
9a), anti-HIV10 (Fig. 1, LAM-trans10a,b), anticonvulsant such as perampanel,11 schizophrenia, for example JNJ40411813,12 vasodilator (Fig. 1, milrinone and amrinone),13 and antidiabetic activities.14 Consequently, pyridin-2-ones have been widely studied and more methods have been constructed for the synthesis of this type of compound, including the Vilsmeier reaction of cyclopropane derivatives,15 the Vilsmeier–Haack reaction of cyclopropane derivatives,16 aldol-like cyclocondensation of aryl aminoketones,17 [2 + 2] cyclization,18 [1 + 2 + 3] cyclization, [3 + 3] cyclization, and rearrangement or aryl migration,19 and so on.20–22 Although these methods have contributed to the synthesis of pyridin-2-ones, they usually have some shortcomings. Some methods use toxic reagents, such as POCl3 and PBr3, and obtain lower yields in the Vilsmeier reaction,15,16 and some methods need expensive transition metal additives,18,21a harsh reaction conditions,17 or tedious workup procedures. In addition, the construction of carbon–carbon bonds, along with the creation of quaternary centers, continues to be an appealing and demanding area of research. Until now, there were not many efficient and practical methods for the synthesis of pyridin-2-ones bearing quaternary centers.23a Therefore, it is desirable to develop an environmentally friendly, multi-component, solvent-free approach to efficiently synthesize pyridin-2-ones bearing quaternary centers, with the goal of achieving diversity in molecular structures from available building blocks and under mild reaction conditions.
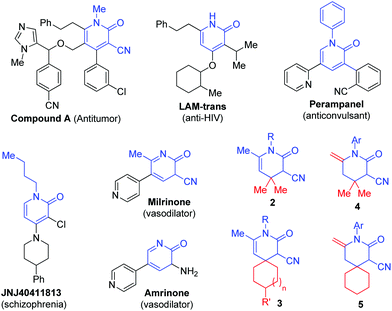 |
| Fig. 1 Bioactive pyridin-2-ones and the target compounds 2–5. | |
Herein, target compounds based on three-component 2-cyanoacetamides 1, various ketones, and acetone have been designed for the synthesis of functional pyridin-2-ones bearing quaternary centers. This has been accomplished by reacting various 2-cyanoacetamides 1 with different ketones and acetone via the Knoevenagel reaction, Michael addition reaction, and intramolecular cyclization, via solvent-free reactions at 50 °C. This method is simple, efficient, environmentally friendly, and produces excellent yields (Scheme 1).
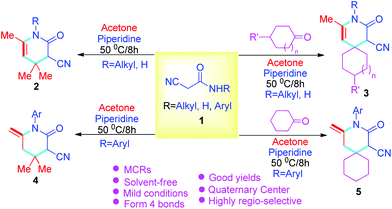 |
| Scheme 1 Methods for the synthesis of pyridin-2-ones bearing quaternary centers. | |
Results and discussion
Reaction condition optimization was a key focus in this paper. In the initial experiment, 2-cyanoacetamide 1a was used as the substrate and reacted with acetone in methanol. No reaction occurred without the addition of a promoter at reflux for 6 h (Table 1, entry 1), then, basic catalysts including K2CO3, Cs2CO3, KOH, Et3N, piperidine, and DBU, and acidic catalysts such as AcOH, were assessed (Table 1, entries 2–8). In the catalyst screening process, it was determined that most of the alkali can promote Knoevenagel condensation reactions and provide intermediate 9 (Scheme 2). However, piperidine is the optimal catalyst for the Michael addition reaction and intramolecular cyclization and gives the target compound 2a. The reaction promoted by DBU can only obtain compound 2a with very low yield (<5%) (Table 1, entry 7). It is believed that piperidine further promotes acetone to form intermediate 10 instead of DBU (Scheme 2). The results showed that this reaction does not efficiently proceed except when using piperidine as the promoter, which produced the desired compound 2a in 67% yield (Table 1, entry 6). Based on this result and the principles of environmental friendliness and simple post-treatments, other green or commonly available solvents were tested in this reaction, including EtOH, MeCN, CHCl3, DCM, 1,4-dioxane, and H2O (Table 1, entries 9–14). The results showed that although this reaction proceeded in all solvents at reflux, EtOH was the most suitable solvent and produced the target compound 2a in 72% yield. Next, solvent-free conditions were investigated. The yield of target compound 2a significantly improved under solvent-free conditions with an 84% yield (Table 1, entry 15). Reaction temperature was then evaluated and it was revealed that the yield decreased as the temperature decreased to room temperature (Table 1, entry 16). When the temperature was decreased to 50 °C, the yield did not change (Table 1, entry 17). The reaction time was optimized, and the results suggested that at 8 h, the yield of 2a was the highest (Table 1, entry 17 vs. 18–19). For further optimization, the concentration of piperidine was varied (Table 1, entries 20–22), and at 0.5 equiv., the reaction performed the best with a 95% yield of compound 2a. Finally, microwave radiation was incorporated into this reaction, and the reaction catalyzed by piperidine under microwave radiation (1000 W) at 50 °C for 15 minutes achieved compound 2a in only 39% yield (Table 1, entry 23). As the reaction time increased to 30 minutes, the reaction was catalyzed by piperidine under microwave radiation (1000 W) at 50 °C to obtain 2a with 84% yield (Table 1, entry 24). Accordingly, it was concluded that the optimal conditions were a piperidine concentration of 0.5 equiv. as the catalyst under solvent-free conditions and at 50 °C for 8 hours.
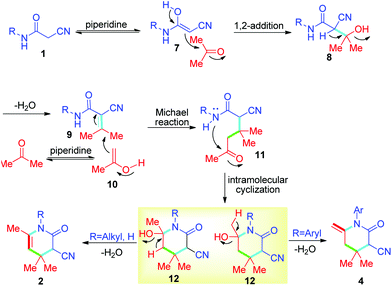 |
| Scheme 2 The proposed mechanism for the formation of compound 4. | |
Table 1 Optimization of the reaction conditions for the model reactiona
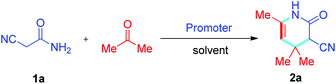
|
Entry |
Solvent |
Additive |
T (°C) |
Time/h |
Yieldb (%) |
Reagents and conditions: 2-Cyanoacetamide 1a (1.0 mmol) and solvent (5 mL) or solvent-free.
Isolated yield based on 1a.
Microwave irradiation, 1000 W.
|
1 |
CH3OH |
— |
Reflux |
6 |
n.r |
2 |
CH3OH |
K2CO3 (1.0 equiv.) |
Reflux |
6 |
Trace |
3 |
CH3OH |
Cs2CO3 (1.0 equiv.) |
Reflux |
6 |
n.r |
4 |
CH3OH |
KOH (1.0 equiv.) |
Reflux |
6 |
n.r |
5 |
CH3OH |
Et3N (1.0 equiv.) |
Reflux |
6 |
Trace |
6 |
CH3OH |
Piperidine (1.0 equiv.) |
Reflux |
6 |
67 |
7 |
CH3OH |
DBU (1.0 equiv.) |
Reflux |
6 |
Trace |
8 |
CH3OH |
AcOH (1.0 equiv.) |
Reflux |
6 |
n.r |
9 |
EtOH |
Piperidine (1.0 equiv.) |
Reflux |
6 |
72 |
10 |
MeCN |
Piperidine (1.0 equiv.) |
Reflux |
6 |
59 |
11 |
CHCl3 |
Piperidine (1.0 equiv.) |
Reflux |
6 |
48 |
12 |
DCM |
Piperidine (1.0 equiv.) |
Reflux |
6 |
37 |
13 |
1,4-Dioxane |
Piperidine (1.0 equiv.) |
Reflux |
6 |
66 |
14 |
H2O |
Piperidine (1.0 equiv.) |
Reflux |
6 |
42 |
15 |
— |
Piperidine (1.0 equiv.) |
Reflux |
6 |
84 |
16 |
— |
Piperidine (1.0 equiv.) |
r.t |
6 |
63 |
17 |
— |
Piperidine (1.0 equiv.) |
50 |
6 |
84 |
18 |
— |
Piperidine (1.0 equiv.) |
50 |
4 |
70 |
19 |
— |
Piperidine (1.0 equiv.) |
50 |
8 |
89 |
20 |
— |
Piperidine (2.0 equiv.) |
50 |
8 |
79 |
21 |
— |
Piperidine (0.5 equiv.) |
50 |
8 |
95 |
22 |
— |
Piperidine (0.25 equiv.) |
50 |
8 |
90 |
23 |
— |
Piperidine (0.5 equiv.) |
50 |
0.25 |
39c |
24 |
— |
Piperidine (0.5 equiv.) |
50 |
0.5 |
84c |
With the prescribed optimized conditions, the scope of substrates was further explored. Various R substituents in cyanoacetamide 1 including CH3, C2H5, C6H5CH2, p-ClC6H4CH2, p-MeC6H4CH2, and p-FC6H4CH2 were used as substrates for reaction with acetone (Table 2, 2b–2g). The results revealed that the substituted groups of cyanoacetamide 1 have little influence on the reaction, where only R = Me, Et showed a decreased yield. In the end, cyanoacetamide 1 reacted well with acetone and produced good to excellent yields. To further expand the diversity of target compounds, cyclohexanone or cyclopentanone were used to react with cyanoacetamide 1 to form the intermediate of the Knoevenagel condensation reaction and further react with acetone to obtain the expected product. Based on this idea, first, the intermediate of the Knoevenagel condensation reaction was synthesized from cyclic ketone and cyanoacetamide 1via piperidine as the catalyst at 50 °C for 2 hours under solvent-free conditions. Acetone was immediately added to the reaction solution, and target compounds 3a–3j were efficiently synthesized in high yields by further reaction of the intermediate with acetone under optimal conditions (Table 2, 3a–3j). A similar qualitative result was shown where as before, when R = CH3, CH2CH3, the yield decreased (Table 2, 3avs.3b–3c; 3dvs.3e–3f; 3gvs.3h). Additionally, the chain length of the cyclic ketone also affects the yield of the target compounds, where shorter chain cyclic ketones produce target compounds with higher yields (Table 2, 3a–3cvs.3d–3h) than those with longer chains. Generally, the yield of the reaction depends on the size of the cyclic ketone, and the yield of cyclohexanone is higher than that of cyclopentanone (Table 2, 3a–3hvs.3i–3j). The yield of compound 2 is generally higher than that of compound 3 (Table 2, 2a–2gvs.3a–3j).
Table 2 Synthesis of pyridin-2-ones bearing quaternary centers 2–3
a,b,c
Reagents and conditions: 2-Cyanoacetamide 1 (1.0 mmol), ketone (0.5 mL) and acetone (1.0 mL).
Piperidine (0.5 mmol).
Isolated yield based on 1.
|
|
Next, more substrates were tested to include cyano-N-phenylacetamide, which was subsequently reacted with acetone under solvent-free conditions promoted by piperidine at 50 °C for 8 hours. A series of novel structure types, 4a–4e, with exocyclic double bonds were obtained and produced excellent yields. The aryl group of the target compound 4 and the exocyclic double bonds easily form π–π conjugation, which is conducive to the structural stability of compound 4. In addition, the results showed that the substituted groups on the phenyl ring have only minor influence on the reaction (Table 3, 4a–4e). On this basis, cyano-N-phenylacetamide 1 was further expanded, and compounds 5a–5d were synthesized in excellent yields using the solvent-free, multicomponent, one-pot method. The effects of substituents are similar to those of previous reactions (Table 3, 5a–5d), but, in total, the yield is slightly lower than that of compound 4 (Table 3, 4a–4evs.5a–5d).
Table 3 Synthesis of pyridin-2-ones bearing quaternary centers 4–5
a,b,c
Reagents and conditions: 2-Cyanoacetamide 1 (1.0 mmol), ketone (0.5 mL) and acetone (1.0 mL).
Piperidine (0.5 mmol).
Isolated yield.
|
|
Finally, different structures of aromatic aldehydes were used as substrates to react with 2-cyanoacetamides 1 and acetone. The results showed that the aromatic aldehydes can also form target compounds 6, albeit with lower yields than that of cyclic ketones (Tables 2–3vs.Table 4).
Table 4 Synthesis of pyridin-2-ones 6
a,b,c
Reagents and conditions: 2-Cyanoacetamide 1 (1.0 mmol), benzaldehyde (0.5 mL) and acetone (1.0 mL).
Piperidine (0.5 mmol).
Isolated yield.
|
|
To verify the structure of the target product, compounds 2a and 4a were selected as representative compounds and characterized by X-ray crystallography (Fig. 2).
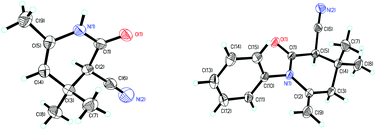 |
| Fig. 2 X-Ray crystal structure of 2a (left) and 4a (right). | |
The proposed mechanism is outlined in Scheme 2. Initially, 2-cyanoacetamide 1 was ketone-enol tautomerization catalyzed by piperidine to form intermediate 7. Then, intermediate 7 reacted with acetone via 1,2-addition, eliminating a molecule of water to form the intermediate 9via the Knoevenagel condensation reaction. Similarly, acetone was changed to intermediate 10 catalyzed by piperidine through ketone-enol tautomerization. Next, intermediate 10 reacted with compound 9 through the Michael addition reaction to form intermediate 11. Next, intermediate 12 was formed via the intramolecular cyclization reaction of intermediate 11. Finally, there were two possible reaction directions. If substrate 1 was alkyl- or hydrogen-substituted 2-cyanoacetamide (R = alkyl, H), the dehydration leading to the final product was target compound 2. However, if substrate 1 was aryl substituted 2-cyanoacetamide (R = aryl), the dehydration leading to the final product was target compound 4. In summary, this reaction is a regioselective dehydration reaction that depends on the stability of the target compounds. Common characterization methods, IR, 1H NMR, 13C NMR, and HRMS, were used to distinguish the structure of the product (2 or 4).
Conclusions
An environmentally friendly and practical strategy for the synthesis of four types of pyridin-2-ones (2–5) via the solvent-free cascade reaction of 2-cyanoacetamides 1, different ketones and acetone via multicomponent reactions has been developed. As a result, functionalized pyridin-2-ones bearing quaternary centers (2–5), specifically spiropyridin-2-ones (3 and 5), have been produced using piperidine under solvent-free conditions. Also, a double bond has been introduced into target compounds 4 and 5 only through aryl substituted 2-cyanoacetamides under the same conditions. The advantages of this approach include no solvents, simple and practical operation (multi-component one-pot), and high yields (up to 95%). More importantly, acetone/cyclic ketone with low reactivity was used to synthesize compounds with quaternary carbon centers or spirocompound libraries.
Experimental section
General information
All compounds were fully characterised by spectroscopic data. The NMR spectra were recorded on a Bruker DRX500 & DRX600. Chemical shifts (δ) are expressed in ppm, J values are given in Hz, and deuterated CDCl3 was used as the solvent. IR spectra were recorded on a FT-IR Thermo Nicolet Avatar 360 using a KBr pellet. The reactions were monitored by thin layer chromatography (TLC) using silica gel GF254. The melting points were determined on an XT-4A melting point apparatus and are uncorrected. HRMs were performed on an Agilent LC/Msd TOF instrument.
The materials used were purchased from Adamas-Beta Corporation Limited. All chemicals and solvents were used as received without further purification unless otherwise stated. Column chromatography was performed on silica gel (200–300 mesh). 2-Cyanoacetamides 1a–1c were commercially available reagents, and 2-cyanoacetamides 1d–1g were prepared according to the literature.23b
General procedure for the synthesis of 2 and 4
First, cyanoacetamide 1 (1.0 mmol) and acetone (1.5 mL) were charged into a round-bottom flask. Then, piperidine (0.5 mmol) was added to the mixture. The mixture was stirred at 50 °C for about 8 hours and monitored by TLC until the intermediate was completely consumed. The reaction mixture was poured into 20 mL of water and 10 mL of ethyl acetate for extraction and separation. The crude product was purified by column chromatography (petroleum ether/EtOAc = 6
:
1), and a series of compounds 2 or 4 were obtained with 71–95% yield.
General procedure for the synthesis of 3 and 5
First, cyanoacetamide 1 (1.0 mmol) and cyclic ketone (0.5 mL) were charged into a round-bottom flask. Then, piperidine (0.5 mmol) was added to the mixture. The mixture was stirred at 50 °C for about 2 hours. Next, acetone (1.0 mL) was charged into the reaction mixture. The mixture was continually stirred at 50 °C for 6 hours and monitored by TLC until the intermediate of the condensation reaction substrate was completely consumed. The reaction mixture was poured into 20 mL of water and 10 mL of ethyl acetate for extraction and separation. The crude product was purified by column chromatography (petroleum ether/EtOAc = 10
:
1), and a series of compounds 3 or 5 were obtained with 68–90% yield.
4,4,6-Trimethyl-2-oxo-1,2,3,4-tetrahydropyridine-3-carbonitrile (2a).
White solid; mp: 138.8–139.4 °C; IR (KBr): 3438, 2969, 1670, 1630, 1497, 1399, 1325, 1167, 1079, 762, 702, 664, 584, 527, 437 cm−1; 1H NMR (500 MHz, CDCl3): δ = 1.20 (s, 3H, CH3), 1.28 (s, 3H, CH3), 1.85 (s, 3H, CH3), 3.46 (s, 1H, CH), 4.77 (s, 1H, CH), 8.42 (s, 1H, NH); 13C NMR (125 MHz, CDCl3): δ = 18.6, 23.2, 27.6, 35.1, 47.5, 111.4, 115.0, 131.2, 164.0. HRMS (TOF ES+): m/z calcd for C9H13N2O [(M + H)+], 165.1022; found, 165.1025.
1,4,4,6-Tetramethyl-2-oxo-1,2,3,4-tetrahydropyridine-3-carbonitrile (2b).
White solid; mp: 148.8–149.6 °C; IR (KBr): 3430, 1626, 1368, 1114, 760, 695, 607, 564 cm−1; 1H NMR (600 MHz, CDCl3): δ = 1.15 (s, 3H, CH3), 1.23 (s, 3H, CH3), 1.95 (s, 3H, CH3), 3.15 (s, 3H, CH3), 3.46 (s, 1H, CH), 4.92 (s, 1H, CH); 13C NMR (150 MHz, CDCl3): δ = 19.0, 23.0, 27.2, 29.5, 33.7, 48.1, 114.5, 115.3, 134.6, 162.5. HRMS (TOF ES+): m/z calcd for C10H15N2O [(M + H)+], 179.1179; found, 179.1180.
1-Ethyl-4,4,6-trimethyl-2-oxo-1,2,3,4-tetrahydropyridine-3-carbonitrile (2c).
White solid; mp: 83.7–84.5 °C; IR (KBr): 2974, 1668, 1625, 1390, 1262, 1119, 689, 610, 543 cm−1; 1H NMR (600 MHz, CDCl3): δ = 1.13 (t, J = 6.4 Hz, 6H, CH3), 1.23 (s, 3H, CH3), 1.95 (s, 3H, CH3), 3.45 (s, 1H, CH), 3.52–3.86 (m, 2H, CH2), 4.95 (s, 1H, CH); 13C NMR (150 MHz, CDCl3): δ = 13.6, 18.6, 22.8, 27.1, 33.7, 37.4, 48.2, 115.4, 115.8, 133.9, 162.0. HRMS (TOF ES+): m/z calcd for C11H17N2O [(M + H)+], 193.1335; found, 193.1334.
1-Benzyl-4,4,6-trimethyl-2-oxo-1,2,3,4-tetrahydropyridine-3-carbonitrile (2d).
White solid; mp: 117.1–117.6 °C; IR (KBr): 3442, 2966, 2883, 2837, 1676, 1623, 1498, 1445, 1384, 1238, 1118, 750, 705, 660, 612, 564, 441 cm−1; 1H NMR (600 MHz, CDCl3): δ = 1.16 (s, 3H, CH3), 1.25 (s, 3H, CH3), 1.91 (s, 3H, CH3), 3.55 (s, 1H, CH), 4.63–5.11 (m, 2H, CH2), 4.92 (d, J = 1.0 Hz, 1H, CH), 7.21–7.33 (m, 5H, ArH); 13C NMR (150 MHz, CDCl3): δ = 19.1, 23.0, 27.4, 33.9, 45.8, 48.3, 115.4, 126.8, 127.0, 127.5, 128.8, 134.4, 137.3, 163.0. HRMS (TOF ES+): m/z calcd for C16H19N2O [(M + H)+], 255.1492; found, 255.1496.
1-(4-Chlorobenzyl)-4,4,6-trimethyl-2-oxo-1,2,3,4-tetrahydro-pyridine-3-carbonitrile (2e).
White solid; mp: 114.5–115.0 °C; IR (KBr): 3440, 2967, 1672, 1629, 1492, 1392, 1237, 1100, 1016, 832, 787, 714, 669, 605, 566, 516 cm−1; 1H NMR (600 MHz, CDCl3): δ = 1.15 (s, 3H, CH3), 1.25 (s, 3H, CH3), 1.91 (s, 3H, CH3), 3.55 (s, 1H, CH), 4.59–5.06 (m, 2H, CH2), 4.92 (s, 1H, CH), 7.17 (d, J = 8.3 Hz, 2H, ArH), 7.29 (d, J = 6.4 Hz, 2H, ArH); 13C NMR (150 MHz, CDCl3): δ = 19.1, 23.0, 27.3, 33.9, 45.2, 48.2, 115.2, 115.7, 128.3, 128.5, 128.9, 129.0, 133.4, 134.0, 135.8, 163.0. HRMS (TOF ES+): m/z calcd for C16H18ClN2O [(M + H)+], 289.1102; found, 289.1100.
4,4,6-Trimethyl-1-(4-methylbenzyl)-2-oxo-1,2,3,4-tetrahydro-pyridine-3-carbonitrile (2f).
White solid; mp: 100.7–101.3 °C; IR (KBr): 3443, 2966, 1668, 1627, 1388, 1234, 1116, 789, 673, 596, 539, 443 cm−1; 1H NMR (600 MHz, CDCl3): δ = 1.15 (s, 3H, CH3), 1.24 (s, 3H, CH3), 1.91 (s, 3H, CH3), 2.32 (s, 3H, CH3), 3.54 (s, 1H, CH), 4.58–5.08 (m, 2H, CH2), 4.90 (s, 1H, CH), 7.17 (d, J = 8.3 Hz, 2H, ArH), 7.29 (d, J = 6.4 Hz, 2H, ArH); 13C NMR (150 MHz, CDCl3): δ = 19.1, 21.1, 23.0, 27.4, 33.9, 45.6, 48.3, 115.3, 126.8, 127.0, 129.4, 134.3, 134.4, 137.2, 162.9. HRMS (TOF ES+): m/z calcd for C17H21N2O [(M + H)+], 269.1648; found, 269.1651.
1-(4-Fluorobenzyl)-4,4,6-trimethyl-2-oxo-1,2,3,4-tetrahydro-pyridine-3-carbonitrile (2g).
White solid; mp: 88.9–89.3 °C; IR (KBr): 3432, 2972, 1683, 1619, 1512, 1435, 1388, 1227, 1152, 1101, 842, 808, 675, 611, 545, 485 cm−1; 1H NMR (600 MHz, CDCl3): δ = 1.13 (s, 3H, CH3), 1.24 (s, 3H, CH3), 1.92 (s, 3H, CH3), 3.55 (s, 1H, CH), 4.59–5.07(m, 2H, CH2), 4.94 (s, 1H, CH), 6.99–7.28 (m, 4H, ArH); 13C NMR (150 MHz, CDCl3): δ = 19.0, 23.0, 27.3, 33.9, 45.2, 48.2, 115.2, 115.7 (t, J = 10.5 Hz), 115.2, 128.9 (d, J = 9.0 Hz), 133.1, 134.1, 161.3, 162.9. HRMS (TOF ES+): m/z calcd for C16H18FN2O [(M + H)+], 273.1398; found, 273.1395.
4-Methyl-2-oxo-3-azaspiro[5.5]undec-4-ene-1-carbonitrile (3a).
White solid; mp: 87.4–88.3 °C; IR (KBr): 3434, 2974, 1664, 1625, 1374, 1196, 1118, 770, 670, 610, 441 cm−1; 1H NMR (600 MHz, CDCl3): δ = 1.18–1.34 (m, 2H, CH2), 1.43–1.67 (m, 8H, CH2), 1.82 (d, J = 9.0 Hz, 3H, CH3), 3.35 (s, 1H, CH), 4.97 (s, 1H, CH), 8.23 (s, 1H, NH); 13C NMR (150 MHz, CDCl3): δ = 19.2, 21.5, 21.8, 25.2, 29.4, 32.1, 34.3, 36.4, 48.5, 111.3, 115.4, 134.8, 162.5 HRMS (TOF ES+): m/z calcd for C12H17N2O [(M + H)+], 205.1335; found, 205.1331.
3,4-Dimethyl-2-oxo-3-azaspiro[5.5]undec-4-ene-1-carbonitrile (3b).
White solid; mp: 100.9–101.4 °C; IR (KBr): 3422, 2923, 1618, 1384, 1331, 1115, 784, 654, 615 cm−1; 1H NMR (600 MHz, CDCl3): δ = 1.14–1.35 (m, 2H, CH2), 1.39–1.45 (m, 4H, CH2), 1.51–1.57 (m, 4H, CH2), 1.92 (s, 3H, CH3), 3.07 (s, 3H, CH3), 3.38 (s, 1H, CH), 5.09 (s, 1H, CH); 13C NMR (150 MHz, CDCl3): δ = 19.2, 21.5, 21.8, 25.2, 29.4, 32.1, 34.3, 36.4, 47.8, 111.3, 115.4, 134.8, 162.5. HRMS (TOF ES+): m/z calcd for C13H19N2O [(M + H)+], 219.1492; found, 219.1490.
3-Ethyl-4-methyl-2-oxo-3-azaspiro[5.5]undec-4-ene-1-carbonitrile (3c).
White solid, 216 mg; mp: 99.7–100.3 °C; IR (KBr): 3435, 2934, 2855, 1675, 1622, 1451, 1392, 1260, 1119, 770, 677, 605, 554 cm−1; 1H NMR (600 MHz, CDCl3): δ = 1.13 (t, J = 7.1 Hz, 3H, CH3), 1.25–1.42 (m, 2H, CH2), 1.47–1.52 (m, 4H, CH2), 1.58–1.72 (m, 4H, CH2), 1.98 (s, 3H, CH3), 3.42 (s, 3H, CH3), 3.60–3.76 (m, 2H, CH2), 5.16 (s, 1H, CH); 13C NMR (150 MHz, CDCl3): δ = 13.6, 18.9, 21.6, 21.8, 25.2, 32.3, 34.3, 36.5, 37.4, 47.8, 112.7, 115.4, 134.1, 162.0. HRMS (TOF ES+): m/z calcd for C14H21N2O [(M + H)+], 233.1648; found, 233.1649.
4,9-Dimethyl-2-oxo-3-azaspiro[5.5]undec-4-ene-1-carbonitrile (3d).
White solid; mp: 107.7–108.1 °C; IR (KBr): 3429, 3229, 3170, 3113, 2931, 2867, 1708, 1684, 1620, 1452, 1376, 1119, 829, 679, 504 cm−1; 1H NMR (600 MHz, CDCl3): δ = 0.94 (d, J = 1.8 Hz, 3H, CH3), 0.95–1.17 (m, 2H, CH2), 1.34–1.50 (m, 2H, CH2), 1.56–1.72 (m, 4H, CH2), 1.88 (s, 3H, CH3), 2.00–2.02 (m, 1H, CH), 3.61 (s, 1H, CH), 4.78 (s, 1H, CH), 8.50 (s, 1H, NH); 13C NMR (150 MHz, CDCl3): δ = 18.7, 20.7, 28.8, 29.0, 30.2, 31.0, 32.3, 37.5, 42.1, 110.4, 115.3, 131.6, 163.8. HRMS (TOF ES+): m/z calcd for C13H19ClN2O [(M + H)+], 219.1492; found, 219.1490.
3,4,9-Trimethyl-2-oxo-3-azaspiro[5.5]undec-4-ene-1-carbonitrile (3e).
White solid; mp: 109.3–109.9 °C; IR (KBr): 3436, 2935, 1669, 1624, 1441, 1371, 1111, 778, 657, 607, 557 cm−1; 1H NMR (600 MHz, CDCl3): δ = 0.93 (q, J = 3.2 Hz, 3H, CH3), 1.08–1.14 (m, 2H, CH2), 1.31–1.45 (m, 2H, CH2), 1.58–1.72 (m, 4H, CH2), 1.99 (s, 4H, CH3, CH), 3.13 (s, 3H, CH3), 3.68 (s, 1H, CH), 4.90 (s, 1H, CH); 13C NMR (150 MHz, CDCl3): δ = 19.1, 20.9, 28.0, 29.1, 29.5, 30.3, 31.0, 32.5, 36.1, 42.4, 113.9, 115.6, 135.0, 162.2. HRMS (TOF ES+): m/z calcd for C14H21N2O [(M + H)+], 233.1648; found, 233.1650.
3-Ethyl-4,9-dimethyl-2-oxo-3-azaspiro[5.5]undec-4-ene-1-carbonitrile (3f).
White solid; mp: 110.0–110.5 °C; IR (KBr): 3437, 2936, 2893, 1675, 1624, 1459, 1391, 1265, 1215, 1119, 780, 664, 604 cm−1; 1H NMR (600 MHz, CDCl3): δ = 0.93 (d, J = 6.7 Hz, 3H, CH3), 1.08–1.15 (m, 5H, CH2, CH3), 1.31–1.44 (m, 2H, CH2), 1.57–1.65 (m, 4H, CH2), 1.99 (s, 3H, CH3), 2.00 (s, 1H, CH), 3.50 (q, J = 7.0 Hz, 1H, CH2), 3.65 (s, 1H, CH), 3.84 (q, J = 7.1 Hz, 1H, CH2), 4.92 (s, 1H, CH); 13C NMR (150 MHz, CDCl3): δ = 13.4, 18.7, 20.9, 28.8, 29.1, 29.7, 30.6, 30.9, 32.6, 36.2, 37.3, 42.5, 115.0, 115.7, 134.2, 161.7. HRMS (TOF ES+): m/z calcd for C15H23N2O [(M + H)+], 247.1805; found, 247.1803.
9-Ethyl-3,4-dimethyl-2-oxo-3-azaspiro[5.5]undec-4-ene-1-carbonitrile (3g).
White solid; mp: 131.7–132 °C; IR (KBr): 3422, 3231, 2923, 2899, 1618, 1384, 1331, 1115, 615 cm−1; 1H NMR (600 MHz, CDCl3): δ = 0.89 (t, J = 7.4 Hz, 3H, CH3), 1.00–1.27 (m, 4H, CH2), 1.43–1.58 (m, 6H, CH2), 1.66–1.72 (m, 1H, CH), 1.98 (s, 3H, CH3), 3.14 (s, 3H, CH3), 3.39 (s, 1H, CH), 5.17 (s, 1H, CH); 13C NMR (150 MHz, CDCl3): δ = 11.4, 19.3, 28.0, 28.2, 29.5, 31.9, 34.7, 36.5, 38.4, 48.4, 111.0, 115.3, 134.8, 162.6. HRMS (TOF ES+): m/z calcd for C15H23N2O [(M + H)+], 247.1805; found, 247.1803.
9-Ethyl-4-methyl-2-oxo-3-azaspiro[5.5]undec-4-ene-1-carbonitrile (3h).
White solid; mp: 148.8–149.3 °C; IR (KBr): 3431, 3236, 3121, 2934, 2865, 1688, 1624, 1450, 1373, 1123, 816, 549, 509 cm−1; 1H NMR (600 MHz, CDCl3): δ = 0.88 (t, J = 6.7 Hz, 3H, CH3), 1.12–1.19 (m, 2H, CH2), 1.29–1.37 (m, 4H, CH2), 1.44–1.49 (m, 1H, CH), 1.66–1.72 (m, 3H, CH2), 1.88 (s, 3H, CH3), 2.00–2.02 (m, 1H, CH2), 3.61 (s, 1H, CH), 4.79 (s, 1H, CH), 8.43 (s, 1H, NH); 13C NMR (150 MHz, CDCl3): δ = 11.6, 18.7, 26.5, 26.7, 27.7, 31.1, 32.4, 37.1, 37.8, 42.1, 110.5, 115.3, 131.5, 163.8. HRMS (TOF ES+): m/z calcd for C14H21N2O [(M + H)+], 233.1648; found, 233.1649.
9-Methyl-7-oxo-8-azaspiro[4.5]dec-9-ene-6-carbonitrile (3i).
White solid; mp: 165.2–165.8 °C; IR (KBr): 3281, 2960, 2870, 1672, 1447, 1385, 1264, 1114, 786, 611 cm−1; 1H NMR (600 MHz, CDCl3): δ = 1.63–1.74 (m, 8H, CH2), 1.81 (s, 3H, CH3), 3.50 (s, 1H, CH), 4.82 (s, 1H, CH), 8.38 (s, 1H, NH); 13C NMR (150 MHz, CDCl3): δ = 18.6, 23.9, 24.2, 36.0, 37.3, 44.8, 45.2, 111.5, 115.6, 131.1, 164.1. HRMS (TOF ES+): m/z calcd for C11H15N2O [(M + H)+], 191.1179; found, 191.1183.
8,9-Dimethyl-7-oxo-8-azaspiro[4.5]dec-9-ene-6-carbonitrile (3j).
White solid; mp: 144.2–144.9 °C; IR (KBr): 3434, 2962, 1679, 1626, 1447, 1370, 1259, 1121, 774, 658, 609, 566 cm−1; 1H NMR (600 MHz, CDCl3): δ = 1.65–1.86 (m, 8H, CH2), 1.96 (s, 3H, CH3), 3.15 (s, 3H, CH3), 3.55 (s, 1H, CH), 5.05 (s, 1H, CH); 13C NMR (150 MHz, CDCl3): δ = 19.0, 23.9, 24.1, 29.5, 35.9, 37.0, 44.0, 45.3, 114.9, 115.9, 134.5, 162.6. HRMS (TOF ES+): m/z calcd for C12H17N2O [(M + H)+], 205.1335; found, 205.1335.
4,4-Dimethyl-6-methylene-2-oxo-1-phenylpiperidine-3-carbonitrile (4a).
White solid; mp: 166.6–166.9 °C; IR (KBr): 3435, 2968, 2836, 1668, 1628, 1498, 1375, 1325, 1123, 762, 700, 600 cm−1; 1H NMR (600 MHz, CDCl3): δ = 1.29 (s, 6H, CH3), 2.55–2.66 (m, 2H, CH2), 3.62 (s, 1H, CH), 3.81 (s, 1H, CH2), 4.23 (s, 1H, CH2), 7.13 (d, J = 7.6 Hz, 2H, ArH), 7.40–7.48 (m, 3H, ArH); 13C NMR (150 MHz, CDCl3): δ = 22.8, 27.6, 32.7, 42.3, 48.7, 97.6, 115.3, 128.4, 128.6, 129.8, 137.7, 142.4, 161.2. HRMS (TOF ES+): m/z calcd for C15H17N2O [(M + H)+], 241.1335; found, 241.1335.
1-(4-Chlorophenyl)-4,4-dimethyl-6-methylene-2-oxopiperidine-3-carbonitrile (4b).
White solid; mp: 179.2–179.9 °C; IR (KBr): 3442, 2961, 1676, 1628, 1487, 1372, 1291, 1096, 843, 792, 724, 599, 517 cm−1; 1H NMR (600 MHz, CDCl3): δ = 1.28 (s, 3H, CH3), 1.29 (s, 3H, CH3), 2.55–2.66 (m, 2H, CH2), 3.62 (s, 1H, CH), 3.83 (s, 1H, CH2), 4.26 (s, 1H, CH2), 7.08 (d, J = 8.5 Hz, 2H, ArH), 7.45 (d, J = 8.5 Hz, 2H, ArH); 13C NMR (150 MHz, CDCl3): δ = 22.8, 27.7, 32.8, 42.2, 48.7, 97.8, 115.1, 129.9, 130.1, 134.6, 136.1, 142.3, 161.3. HRMS (TOF ES+): m/z calcd for C15H16ClN2O [(M + H)+], 275.0946; found, 275.0940.
4,4-Dimethyl-6-methylene-2-oxo-1-(p-tolyl)piperidine-3-carbonitrile (4c).
White solid; mp: 188.1–188.8 °C; IR (KBr): 3442, 2971, 2835, 1625, 1510, 1366, 1120, 768, 660, 602, 554, 522, 498, 436 cm−1; 1H NMR (600 MHz, CDCl3): δ = 1.28 (s, 6H, CH3), 2.39 (s, 3H, CH3), 2.54–2.65 (m, 2H, CH2), 3.60 (s, 1H, CH), 3.85 (s, 1H, CH2), 4.22 (s, 1H, CH2), 7.00 (d, J = 8.2 Hz, 2H, ArH), 7.27 (d, J = 8.0 Hz, 2H, ArH); 13C NMR (150 MHz, CDCl3): δ = 21.2, 22.8, 27.7, 32.7, 42.2, 48.7, 97.5, 115.4, 128.0, 130.4, 135.0, 138.5, 142.5, 161.2. HRMS (TOF ES+): m/z calcd for C16H19N2O [(M + H)+], 255.1492; found, 255.1490.
1-(4-Fluorophenyl)-4,4-dimethyl-6-methylene-2-oxopiperidine-3-carbonitrile (4d).
White solid; mp: 182.6–183.0 °C; IR (KBr): 3442, 2966, 1626, 1513, 1370, 1114, 836, 766, 688, 601, 533 cm−1; 1H NMR (600 MHz, CDCl3): δ = 1.28 (s, 3H, CH3), 1.29 (s, 3H, CH3), 2.56–2.66 (m, 2H, CH2), 3.62 (s, 1H, CH), 3.82 (s, 1H, CH2), 4.26 (s, 1H, CH2), 7.10–7.27 (m, 4H, ArH); 13C NMR (150 MHz, CDCl3): δ = 22.8, 27.7, 32.8, 42.2, 48.7, 97.6, 116.8 (d, J = 22.5 Hz), 130.2 (d, J = 9.0 Hz), 133.4, 142.5, 161.4 (d, J = 12.0 Hz), 163.1. HRMS (TOF ES+): m/z calcd for C15H16FN2O [(M + H)+], 259.1241; found, 259.1240.
1-(4-Methoxyphenyl)-4,4-dimethyl-6-methylene-2-oxopiperidine-3-carbonitrile (4e).
White solid; mp: 144.4–144.8 °C; IR (KBr): 3441, 2964, 1677, 1624, 1512, 1369, 1255, 1111, 867, 768, 660, 606, 556 cm−1; 1H NMR (600 MHz, CDCl3): δ = 1.28 (s, 6H, CH3), 2.54–2.64 (m, 2H, CH2), 3.61 (s, 1H, CH), 3.86 (s, 3H, OCH3), 4.23 (s, 1H, CH2), 6.97 (d, J = 8.8 Hz, 2H, ArH), 7.03 (d, J = 8.8 Hz, 2H, ArH); 13C NMR (150 MHz, CDCl3): δ = 22.8, 27.7, 32.7, 42.2, 48.7, 55.5, 97.5, 115.0, 115.4, 129.3, 130.2, 142.6, 159.4, 161.4. HRMS (TOF ES+): m/z calcd for C16H19N2O2 [(M + H)+], 271.1441; found, 271.1439.
4-Methylene-2-oxo-3-phenyl-3-azaspiro[5.5]undecane-1-carbonitrile (5a).
White solid; mp: 162.4–162.8 °C; IR (KBr): 3440, 2962, 2931, 2877, 1674, 1627, 1477, 1364, 1266, 1199, 1124, 799, 723, 690, 606, 528, 436 cm−1; 1H NMR (600 MHz, CDCl3): δ = 1.26–1.71 (m, 10H, CH2), 2.49–3.01 (m, 2H, CH2), 3.66 (s, 1H, CH), 3.82 (s, 1H, CH2), 4.27 (s, 1H, CH2), 7.13 (d, J = 7.5 Hz, 2H, ArH), 7.39–7.49 (m, 3H, ArH); 13C NMR (150 MHz, CDCl3): δ = 21.3, 21.5, 25.3, 31.9, 34.6, 35.2, 36.9, 97.8, 115.3, 128.4, 128.6, 129.8, 137.7, 142.1, 161.1. HRMS (TOF ES+): m/z calcd for C18H21N2O [(M + H)+], 281.1648; found, 281.1644.
3-(4-Fluorophenyl)-4-methylene-2-oxo-3-azaspiro[5.5]undecane-1-carbonitrile (5b).
White solid; mp: 167.9–168.6 °C; IR (KBr): 3439, 2937, 1631, 1508, 1368, 1214, 1119, 672, 605, 543 cm−1; 1H NMR (600 MHz, CDCl3): δ = 1.30–1.70 (m, 10H, CH2), 2.45–3.01 (m, 2H, CH2), 3.65 (s, 1H, CH), 3.83 (s, 1H, CH2), 4.28 (s, 1H, CH2), 7.01 (d, J = 8.2 Hz, 2H, ArH), 7.26 (t, J = 4.0 Hz, 2H, ArH); 13C NMR (150 MHz, CDCl3): δ = 21.2, 21.3, 21.5, 25.3, 31.9, 34.5, 35.1, 36.9, 48.1, 97.6, 115.4, 128.0, 130.4, 135.0, 138.5, 142.1, 161.1. HRMS (TOF ES+): m/z calcd for C18H20FN2O [(M + H)+], 299.1554; found, 299.1549.
4-Methylene-2-oxo-3-(p-tolyl)-3-azaspiro[5.5]undecane-1-carbonitrile (5c).
White solid; mp: 174.4–174.7 °C; IR (KBr): 3441, 2936, 2867, 1677, 1630, 1514, 1454, 1372, 1302, 1112, 836, 767, 706, 661, 602, 558 cm−1; 1H NMR (600 MHz, CDCl3): δ = 1.52–1.70 (m, 10H, CH2), 2.39 (s, 3H, CH3), 2.44–2.99 (m, 2H, CH2), 3.64 (s, 1H, CH), 3.85 (s, 1H, CH2), 4.25 (s, 1H, CH2), 7.10–7.18 (m, 4H, ArH); 13C NMR (150 MHz, CDCl3): δ = 21.3, 21.4, 25.3, 31.9, 34.6, 35.2, 36.9, 48.1, 97.8, 115.1, 116.8 (d, J = 22.5 Hz), 130.2 (d, J = 7.5 Hz), 133.4, 142.1, 161.4 (d, J = 30.0 Hz), 163.1. HRMS (TOF ES+): m/z calcd for C19H23N2O [(M + H)+], 295.1805; found, 295.1800.
3-(4-Methoxyphenyl)-4-methylene-2-oxo-3-azaspiro[5.5]undecane-1-carbonitrile (5d).
White solid; mp: 171.9–172.3 °C; IR (KBr): 3436, 2972, 1683, 1614, 1512, 1435, 1390, 1342, 1276, 1227, 1154, 841, 808, 761, 671, 544 cm−1; 1H NMR (600 MHz, CDCl3): δ = 1.52–1.70 (m, 10H, CH2), 2.44–2.99 (m, 2H, CH2), 3.64 (s, 1H, CH), 3.83 (s, 3H, OCH3), 3.87 (s, 1H, CH2), 4.26 (s, 1H, CH2), 6.97 (d, J = 8.8 Hz, 2H, ArH), 7.04 (t, J = 8.9 Hz, 2H, ArH); 13C NMR (150 MHz, CDCl3): δ = 21.3, 21.5, 25.3, 31.9, 34.5, 35.1, 36.9, 48.1, 55.5, 97.6, 115.0, 115.3, 129.3, 130.2, 142.3, 159.4, 161.3. HRMS (TOF ES+): m/z calcd for C19H23N2O2 [(M + H)+], 311.1754; found, 311.1746.
6-Methyl-2-oxo-4-phenyl-1,2,3,4-tetrahydropyridine-3-carbonitrile (6a).
White solid; mp: 162.2–163.7 °C; IR (KBr): 3434, 3235, 3172, 3121, 2962, 1678, 1622, 1389, 1273, 1193, 1121, 767, 700, 612, 561, 521 cm−1; 1H NMR (600 MHz, CDCl3): δ = 1.96 (s, 3H, CH3), 3.93 (t, J = 5.6 Hz, 1H, CH), 3.98 (d, J = 6.8 Hz, 1H, CH), 5.13 (d, J = 5.2 Hz, 1H, CH), 7.26–7.38 (m, 5H, ArH), 7.92 (s, 1H, NH); 13C NMR (150 MHz, CDCl3): δ = 19.0, 41.1, 41.4, 103.7, 114.8, 128.0, 128.4, 129.1, 134.1, 136.9, 162.7. HRMS (TOF ES+): m/z calcd for C13H13N2O [(M + H)+], 213.1022; found, 213.1024.
6-Methyl-2-oxo-4-(p-tolyl)-1,2,3,4-tetrahydropyridine-3-carbonitrile (6b).
White solid; mp: 160.5–161.0 °C; IR (KBr): 3439, 3233, 1618, 1457, 1339, 1145, 675 cm−1; 1H NMR (600 MHz, CDCl3): δ = 1.93 (s, 3H, CH3), 2.34 (d, J = 9.0 Hz, 3H, CH3), 3.65 (t, J = 12.0 Hz, 1H, CH), 3.96 (d, J = 11.9 Hz, 1H, CH), 4.94 (s, 1H, CH), 7.17–7.18 (m, 4H, ArH), 7.50 (s, 1H, NH); 13C NMR (150 MHz, CDCl3): δ = 18.9, 21.1, 42.7, 42.8, 104.3, 115.9, 127.5, 129.7, 129.8, 133.8, 136.5, 138.0, 163.3. HRMS (TOF ES+): m/z calcd for C14H15N2O [(M + H)+], 227.1179; found, 227.1180.
1,6-Dimethyl-2-oxo-4-phenyl-1,2,3,4-tetrahydropyridine-3-carbonitrile (6c).
White solid; mp: 102.5–103.1 °C; IR (KBr): 3440, 1671, 1454, 1371, 1134, 771, 710, 668, 508 cm−1; 1H NMR (600 MHz, CDCl3): δ = 2.06 (d, J = 1.2 Hz, 3H, CH3), 3.20 (t, J = 3.6 Hz, 3H, CH3), 3.71 (d, J = 11.6 Hz, 1H, CH), 3.90 (d, J = 11.6 Hz, 1H, CH), 5.13 (d, J = 1.3 Hz, 1H, CH), 7.27 (q, J = 2.7 Hz, 2H, ArH), 7.30–7.33 (m, 1H, ArH), 7.37 (t, J = 7.5 Hz, 2H, ArH); 13C NMR (150 MHz, CDCl3): δ = 19.4, 29.8, 41.7, 43.2, 107.2, 116.0, 127.5, 128.2, 129.2, 137.3, 139.2, 161.8. HRMS (TOF ES+): m/z calcd for C14H15N2O [(M + H)+], 227.1179; found, 227.1181.
Conflicts of interest
There are no conflicts to declare.
Acknowledgements
This work was supported by the Program for Changjiang Scholars and Innovative Research Team in University (IRT17R94), the National Natural Science Foundation of China (No. 21662042, 81760621), the Natural Science Foundation of Yunnan Province (2017FA003), the Joint Fund of Yunnan Science and Technology Department-Yunnan University (2019FY003003), and the Scientific and Technological Innovation Team of Green Synthesis and Activity Research of Natural-like Heterocyclic Compound Libraries in Universities of Yunnan Province.
Notes and references
-
(a)
M. Doble and A. K. Kruthiventi, Green Chemistry & Engineering, Academic Press is an imprint of Elsevier, 2007 Search PubMed;
(b) M. C. Bryan, B. Dillon, L. G. Hamann, G. J. Hughes, M. E. Kopach, E. A. Peterson, M. Pourashraf, I. Raheem, P. Richardson, D. Richter and H. F. Sneddon, J. Med. Chem., 2013, 56, 6007–6021 CrossRef CAS PubMed
W. Zhang and B. W. Cue Jr., Green Techniques for Organic Synthesis and Medicinal Chemistry, John Wiley & Sons, Ltd., 2012 Search PubMed
(c) Q.-W. Song, Z.-H. Zhou and L.-N. He, Green Chem., 2017, 19, 3707–3728 RSC.
- G. An, C. Seifert and G. Li, Org. Biomol. Chem., 2015, 13, 1600–1617 RSC.
-
(a) F. Yu, R. Huang, H. Ni, J. Fan, S. Yan and J. Lin, Green Chem., 2013, 15, 453–462 RSC;
(b) B. Zhou, Z.-C. Liu, W.-W. Yang, R. Yang, X.-R. Lin, S.-J. Yan and J. Lin, Green Chem., 2014, 16, 4359–4370 RSC.
- For reviews see:
(a)
Multicomponent Reactions, ed. J. P. Zhu and H. Bienayme, Wiley-VCH, Weinheim, Germany, 2005 Search PubMed;
(b) B. Ganem, Acc. Chem. Res., 2009, 42, 463–472 CrossRef CAS;
(c) A. Dömling, Chem. Rev., 2006, 106, 17–89 CrossRef PubMed.
-
(a) S. Basit, Z. Ashraf, K. Lee and M. Latif, Eur. J. Med. Chem., 2017, 134, 348–356 CrossRef CAS PubMed;
(b) R. L. Dow, M. Ammirati, S. W. Bagley, S. K. Bhattacharya, L. Buckbinder, C. Cortes, A. F. EI-Kattan, K. Ford, G. B. Freeman, C. R. W. Guimaraes, S.-P. Liu, M. Niosi, A. Skoura and D. Tess, J. Med. Chem., 2018, 61, 3114–3125 CrossRef CAS;
(c) M. Wei, X. Peng, L. Xing, Y. Dai, R.-M. Huang, M.-Y. Geng, A. Zhang, J. Ai and Z.-L. Song, Eur. J. Med. Chem., 2018, 154, 9–28 CrossRef CAS PubMed;
(d) W.-S. Guo, Q. Dou, J. Hou, L.-R. Wen and M. Li, J. Org. Chem., 2017, 82, 7015–7022 CrossRef CAS PubMed;
(e) L.-Y. Xie, Y.-J. Li, J. Qu, Y. Duan, J. Hu, K.-J. Liu, Z. Cao and W.-M. He, Green Chem., 2017, 19, 5642–5646 RSC.
-
(a) Y. Wei and N. Yoshikai, J. Am. Chem. Soc., 2013, 135, 3756–3759 CrossRef CAS;
(b) Z. Zhao, H. Wei, K. Xiao, B. Cheng, H. Zhai and Y. Li, Angew. Chem., Int. Ed., 2019, 58, 1148–1152 CrossRef CAS;
(c) C. R. Reddy, R. Ranjan and S. K. Prajapti, Org. Lett., 2019, 21, 623–626 CrossRef CAS PubMed;
(d) H. Andersson, R. Olsson and F. Almqvist, Org. Biomol. Chem., 2011, 9, 337–346 RSC.
-
(a) J.-L. Zhan, M.-W. Wu, D. Wei, B.-Y. Wei, Y. Jiang, W. Yu and B. Han, ACS Catal., 2019, 9, 4179–4188 CrossRef CAS;
(b) X.-X. Du, Q.-X. Zi, Y.-M. Wu, Y. Jin, J. Lin and S.-J. Yan, Green Chem., 2019, 21, 1505–1516 RSC;
(c) L. Chen, R. Huang, X.-X. Du, S.-J. Yan and J. Lin, ACS Sustainable Chem. Eng., 2017, 5, 1899–1905 CrossRef CAS;
(d) B.-Q. Wang, C.-H. Zhang, X.-X. Tian, J. Lin and S.-J. Yan, Org. Lett., 2018, 20, 660–663 CrossRef CAS PubMed.
-
(a) H. Xu, B. Zhou, P. Zhou, J. Zhou, Y. Sen, F.-C. Yu and L.-L. Lu, Chem. Commun., 2016, 52, 8002–8005 RSC;
(b) Q. Luo, R. Huang, Q. Xiao, Y. Yao, J. Lin and S.-J. Yan, J. Org. Chem., 2019, 84, 1999–2011 CrossRef CAS PubMed;
(c) K. Li, L. Chen, Y.-X. Fan, Y. Wei and S.-J. Yan, J. Org. Chem., 2019, 84, 11971–11982 CrossRef CAS PubMed;
(d) F.-C. Yu, B. Zhou, H. Xu, K.-J. Chang and Y. Sen, Tetrahedron Lett., 2015, 56, 837–841 CrossRef CAS.
-
(a) J. F. Blake, M. Burkard, J. Chan, H. Chen, K.-J. Chou, D. Diaz, D. A. Dudley, J. J. Gaudino, S. E. Gould, J. Grina, T. Hunsaker, L. Liu, M. Martinson, D. Moreno, L. Mueller, C. Orr, P. Pacheco, A. Qin, K. Rasor, L. Ren, K. Robarge, S. Shahidi-Latham, J. Stults, F. Sullivan, W. Wang, J. Yin, A. Zhou, M. Belvin, M. Merchant, J. Moffat and J. B. Schwarz, J. Med. Chem., 2016, 59, 5650–5660 CrossRef CAS;
(b) T. Yogo, H. Nagamiya, M. Seto, S. Sasaki, H. Shih-Chung, Y. Ohba, N. Tokunaga, G. N. Lee, C. Y. Rhim, C. H. Yoon, S. Y. Cho, R. Skene, S. Yamamoto, Y. Satou, M. Kuno, T. Miyazaki, H. Nakagawa, A. Okabe, S. Marui, K. Aso and M. Yoshida, J. Med. Chem., 2016, 59, 733–749 CrossRef CAS;
(c) K. Piska, A. Gunia-Krzyżak, P. Koczurkiewicz, K. Wójcik-Pszczoła and E. Pękala, Eur. J. Med. Chem., 2018, 156, 13–20 CrossRef CAS;
(d) L. Li, Y. Zhao, R. Cao, L. Li, G.-H. Cai, J.-J. Li, X.-B. Qi, S. Chena and Z.-Y. Zhang, Chem. Commun., 2019, 55, 4407–4410 RSC;
(e) X.-X. Du, R. Huang, C.-L. Yang, J. Lin and S.-J. Yan, RSC Adv., 2017, 7, 40067–40073 RSC.
-
(a) S.-T. Wu, Q.-Q. Yin, L. Zhao, N.-N. Fan, X.-W. Tang, J.-X. Zhao, T. Sheng, Y. Guo, C. Tian, Z.-L. Zhang, W.-X. Xu, Z.-M. Liu, S.-B. Jiang, L.-Y. Ma, J.-Y. Liu and X.-W. Wang, Org. Biomol. Chem., 2016, 14, 1413–1420 RSC;
(b) H. Vite-Caritino, O. Méndez-Lucio, H. Reyes, A. Cabrera, D. Chávezc and J. L. Medina-Franco, RSC Adv., 2016, 6, 2119–2130 RSC;
(c) M. Okello, M. Nishonov, P. Singh, S. Mishra, N. Mangu, B. Seo, M. Gund and V. Nair, Org. Biomol. Chem., 2013, 11, 7852–7858 RSC;
(d) J. Guillemont, A. Benjahad, S. Oumouch, L. Decrane, P. Palandjian, D. Vernier, L. Queguiner, K. Andries, M.-P. D. Béthune, K. Hertogs, D. S. Grierson and C. H. Nguyen, J. Med. Chem., 2009, 52, 7473–7487 CrossRef CAS PubMed.
- S. Hibi, K. Ueno, S. Nagato, K. Kawano, K. Ito, Y. Norimine, O. Takenaka, T. Hanada and M. Yonaga, J. Med. Chem., 2012, 55, 10584–10600 CrossRef CAS.
- J. M. Cid, G. Tresadern, G. Duvey, R. Lütjens, T. Finn, J.-P. Rocher, S. Poli, J. A. Vega, A. I. D. Lucas, E. Matesanz, M. L. Linares, J. I. Andrés, J. Alcazar, J. M. l. Alonso, G. J. Macdonald, D. Oehlrich, H. Lavreysen, A. Ahnaou, W. Drinkenburg, C. Mackie, S. Pype, D. Gallacher and A. A. Trabanco, J. Med. Chem., 2014, 57, 6495–6512 CrossRef CAS.
-
(a) D. W. Robertson, E. E. Beedle, J. K. Swartzendruber, N. D. Jones, T. K. Elzey, R. F. Kauffman, H. Wilson and J. S. Hayes, J. Med. Chem., 1986, 29, 635–640 CrossRef CAS;
(b) J.-D. Charrier, A. Miller, D. P. Kay, G. Brenchley, H. C. Twin, P. N. Collier, S. Ramaya, S. B. Keily, S. J. Durrant, R. M. A. Knegtel, A. J. Tanner, K. Brown, A. P. Curnock and J.-M. Jimenez, J. Med. Chem., 2011, 54, 2341–2350 CrossRef CAS.
-
(a) D. A. Wacker, Y. W. M. Broekema, K. Rossi, S. O'Connor, Z. Hong, G. Wu, S. E. Malmstrom, C.-P. Hung, L. LaMarre, A. Chimalakonda, L. Zhang, L. Xin, H. Cai, C.-X. Chu, S. Boehm, J. Zalaznick, R. Ponticiello, L. Sereda, S.-P. Han, R. Zebo, B. Zinker, C. E. Luk, R. Wong, G. Everlof, Y.-X. Li, C. K. Wu, M. Lee, S. Griffen, K. J. Miller, J. Krupinski and J. A. Robl, J. Med. Chem., 2014, 57, 7499–7508 CrossRef CAS;
(b) H. Igawa, M. Takahashi, K. Kakegawa, A. Kina, M. Ikoma, J. Aida, T. Yasuma, Y. Kawata, S. Ashina, S. Yamamoto, M. Kundu, U. Khamrai, H. Hirabayashi, M. Nakayama, Y. Nagisa, S. Kasai and T. Maekawa, J. Med. Chem., 2016, 59, 1116–1139 CrossRef CAS;
(c) V. S. P. Lingam, D. H. Dahale, V. E. Rathi, Y. B. Shingote, R. R. Thakur, A. S. Mindhe, S. Kummari, N. Khairatkar-Joshi, M. Bajpai, D. M. Shah, R. S. Sapalya, S. Gullapalli, P. K. Gupta, G. S. Gudi, S. B. Jadhav, R. Pattem and A. Thomas, J. Med. Chem., 2015, 58, 8292–8308 CrossRef CAS;
(d) F. X. Talamas, S. C. Abbot, S. Anand, K. A. Brameld, D. S. Carter, J. Chen, D. Davis, J. d. Vicente, A. D. Fung, L. Gong, S. F. Harris, P. Inbar, S. S. Labadie, E. K. Lee, R. Lemoine, S. L. Pogam, V. Leveque, J. Li, J. McIntosh, I. Nájera, J. Park, A. Railkar, S. Rajyaguru, M. Sangi, R. C. Schoenfeld, L. R. Staben, Y. Tan, J. P. Taygerly, A. G. Villaseñor and P. E. Weller, J. Med. Chem., 2014, 57, 1914–1931 CrossRef CAS.
-
(a) P. Huang, N. Zhang, R. Zhang and D.-W. Dong, Org. Lett., 2012, 14, 370–373 CrossRef CAS PubMed;
(b) D.-X. Xiang, K.-W. Wang, Y.-Y. Liang, G.-Y. Zhou and D.-W. Dong, Org. Lett., 2008, 10, 345–348 CrossRef CAS.
- W. Pan, D.-W. Dong, K.-W. Wang, J. Zhang, R. Wu, D.-X. Xiang and Q. Liu, Org. Lett., 2007, 9, 2421–2423 CrossRef CAS PubMed.
- T. Apsunde and R. P. Wurz, J. Org. Chem., 2014, 79, 3260–3266 CrossRef CAS PubMed.
- R. Shintani, R. Takano and K. Nozaki, Chem. Sci., 2016, 7, 1205–1211 RSC.
- H. Yi, L.-P. Song, W. Wang, J.-N. Liu, S.-Z. Zhu, H.-M. Deng and M. Shao, Chem. Commun., 2010, 46, 6941–6943 RSC.
-
(a) V. Nagaraju, D. Purnachander, N. S. V. M. Rao Mangina, b. Suresh, B. Sridhard and G. V. Karunakar, Org. Biomol. Chem., 2015, 13, 3011–3023 RSC;
(b) P.-Z. Zhang, J.-A. Li, L. Zhang, A. Shoberu, J.-P. Zou and W. Zhang, Green Chem., 2017, 19, 919–923 RSC;
(c) V. Krishnamurti, S. B. Munoz, X. Ispizua-Rodriguez, J. Vickerman, T. Mathew and G. K. S. Prakash, Chem. Commun., 2018, 54, 10574–10577 RSC;
(d) M. Ando, T. Wada and N. Sato, Org. Lett., 2006, 8, 3805–3808 CrossRef CAS PubMed.
-
(a) K. A. Kumar, P. Kannaboinaa and P. Das, Org. Biomol. Chem., 2017, 15, 5457–5461 RSC;
(b) G. Hu, J.-X. Xu and P.-F. Li, Org. Biomol. Chem., 2018, 16, 4151–4158 RSC.
-
(a) X.-X. Du, R. Huang, C.-L. Yang, J. Lin and S.-J. Yan, RSC Adv., 2017, 7, 40067–40073 RSC;
(b) J. Liu, S.-J. Yan, Z.-M. Cao, S.-S. Cui and J. Lin, RSC Adv., 2016, 6, 103057–103064 RSC.
-
(a) S.-X. Lin, Y. Wei, F.-H. Liang, B.-Z. Zhao, Y.-L. Liu and P.-J. Liu, Org. Biomol.
Chem., 2012, 10, 4571–4576 RSC;
(b) K. M. Al-Zaydi, R. M. Borik and M. H. Elnagdi, Green Chem. Lett. Rev., 2012, 5, 241–250 CrossRef CAS.
Footnotes |
† Electronic supplementary information (ESI) available. CCDC 1960650 and 1960651. For ESI and crystallographic data in CIF or other electronic format see DOI: 10.1039/c9gc03692j |
‡ These authors contributed equally to this paper. |
|
This journal is © The Royal Society of Chemistry 2020 |
Click here to see how this site uses Cookies. View our privacy policy here.