DOI:
10.1039/C9FO02654A
(Paper)
Food Funct., 2020,
11, 253-269
Soyasaponins A1 and A2 exert anti-atherosclerotic functionalities by decreasing hypercholesterolemia and inflammation in high fat diet (HFD)-fed ApoE−/− mice
Received
11th November 2019
, Accepted 23rd December 2019
First published on 26th December 2019
Abstract
Atherosclerosis is a chronic inflammatory disease causing coronary heart attacks and strokes. Soyasaponins (SS), the phytochemicals naturally existing in soybeans and their products, have been shown to reduce hypercholesterolemia and inflammation, which are intimately related to the genesis and development of atherosclerosis. However, the anti-atherosclerotic functionality of soyasaponins remains unknown. The aim of this study was to investigate the effects of the supplementation of two types of soyasaponin monomers (A1 and A2) on atherosclerotic plaque formation, serum lipid profiles, and inflammation in ApoE gene knockout (ApoE−/−) mice. Sixty 5-week-old ApoE−/− male mice were fed with a high-fat diet (HFD) and intervened by SSA1 and SSA2 (10 and 20 μmol per kg BW, respectively) or simvastatin (10 μmol per kg BW) for 24 weeks. The atherosclerotic lesions in the aorta, aortic root, and innominate artery, lipid profile and inflammatory markers in serum, and TLR4/MyD88/NF-κB signaling in arterial tissues were determined. SSA1 and SSA2 decreased the plaque ratio in the aortic root and innominate artery but not in the entire aorta. In serum, SSA1 reduced TG, TC, and LDL-C but increased HDL-C; SSA2 decreased TC, TG, and LDL-C but did not affect HDL-C. Meanwhile, SSA1 increased TG, SSA2 increased TC, and both of them increased bile acids in the feces. SSA1 and SSA2 lowered TNF-α, MCP-1, and hs-crp in serum. Furthermore, SSA1 and SSA2 reduced the TLR4 and MyD88 expressions in the aorta and innominate artery and inhibited NF-κB p65 and IκBα phosphorylation in the aorta. These results suggest that SSA1 and SSA2 exert anti-atherosclerotic functionalities by decreasing hypercholesterolemia and inflammation in HFD-fed ApoE−/− mice.
1 Introduction
Cardiovascular diseases (CVDs) are one of the leading causes of premature death in the world; according to the latest report by the World Health Organization (WHO), CVDs have caused an estimated 17.9 million deaths in 2016.1 Out of these deaths, 85% were due to heart attack and stroke, which are primarily caused by atherosclerosis.1,2 Atherosclerosis involves a buildup of fatty deposits (atheroma or plaque) in the large arteries.3 Nowadays, it is well recognized that atherosclerosis is a progressive disease involving lipid dysfunction, oxidative stress, inflammation, foam cell formation, fibrous cap coverage, and eventually the production of severe lesions.3,4 Many genetic and environmental risk factors are associated with the genesis and progression of atherosclerosis. In the past several decades, epidemiological, clinical, and experimental studies have demonstrated that food plays a central role in the prevention of atherosclerosis because of the presence of not only specific nutrients such as unsaturated fatty acids, zinc, and choline, but also bioactive compounds such as phytochemicals.5–9 Phytochemicals, the plant-food-borne secondary metabolites, have particularly attracted considerable interest in recent years because of their significant roles in the prevention of non-communicable chronic diseases including atherosclerosis.5,10
Soy and its products are popular foods in Asian countries. The intake of soy products is associated with a lower incidence of CVDs in Asian populations.11 This beneficial effect has been attributed to the reduction in cholesterol levels, oxidative stress, and inflammation, which are inherently induced by the proteins, fibers, and phytochemicals contained in soy and its products.12,13 Various phytochemicals naturally exist in soybeans and their products. Generally, the major phytochemicals in soybeans are saponins (0.17%–6.16%), phytic acid (1.0%–2.2%), sterols (0.23%–0.46%), isoflavones (0.1%–0.3%), and lignans (0.02%).14 Among all these phytochemicals in soybeans and their products, saponins (often referred to as soyasaponins) are relatively more abundant.15,16 Soyasaponins are triterpenoid glycosides that possess an oleanane-type aglycone with sugar chains. According to the aglycone (soyasapogenol) structure, soyasaponins can be classified into groups A, B, E, and DDMP soyasaponins.16,17 Group A soyasaponins comprise 16 members (A1, A2, A3, A4, A5, A6, Aa or acetyl A4, Ab or acetyl A1, Ac, Ad, Ae or acetyl A5, Af or acetyl A2, Ag or acetyl A6, Ah or acetyl A3, AX, and A3) and group B soyasaponins contain 7 main members (Ba or V, Bb or I, Bc or II, Bb′ or III, Bc′ or BX, IV, and Bh).14In vitro and in vivo studies have revealed that soyasaponins have a wide range of health-promoting benefits, such as anticarcinogenic, antimutagenic, hypoglycemic, hypocholesterolemic, hepatoprotective, immunomodulatory, neuroprotective, anticoagulant, antioxidant, and antiinflammatory bioactivities.14–17 In particular, the hypocholesterolemic, anticoagulant, and antiinflammatory bioactivities of soyasaponins reveal that they may have potential antiatherosclerotic effects because lipid dysfunction, intravascular coagulation, and inflammation are involved in the genesis and development of atherosclerosis.2,5
Firstly, saponins are important in our diets as they reduce the risk of coronary heart disease (CHD) by lowering the cholesterol levels in plasma.16,18 Saponin-containing alfalfa meals promoted the regression of atherosclerosis by reducing cholesterolemia and plasma phospholipid levels in cholesterol-fed monkeys.19 Saponins from Panax ginseng C. A. Mey (Ginsenoside Rb1) inhibited early atherosclerosis by reducing serum lipids (total cholesterol, TC, low-density lipoprotein cholesterol, LDL-C, and triglycerides, TG) and inflammation, as well as reversing the imbalance between apoptosis and autophagy in ApoE−/− mice.20 Further, clinical studies have suggested that the presence of saponins in food was beneficial in lowering blood cholesterol in humans by inhibiting cholesterol absorption or bile acid reabsorption in the body.16,21 The hypocholesterolemic effects of soyasaponins have been recognized since a long time.22–24 Total soyasaponin extracts have been shown to reduce the serum TC, LDL-C, and TG levels, as well as increase high-density lipoprotein cholesterol (HDL-C) in high-fat diet (HFD)-fed mice.25 Group B soyasaponin extracts could decrease plasma TC by 20%, non-HDL-C by 33%, TG by 18%, and ratio of TC to HDL-C by 13% in golden Syrian hamsters through a mechanism involving the enhanced excretion of fecal bile acids and neutral sterols.26 Therefore, it has been suggested that the hypocholesterolemic functionality of soyasaponins could be attributed to their ability to bind cholesterol and bile acids in the digestive tract and then promote their excretion in feces.14,16,23 The oral administration of soyasaponins (90.2% crude saponin content) was found to lower not only blood pressure but also plasma LDL-C (by 29.4%) in spontaneously hypertensive rats.27 Recently, we investigated the effects of three types of purified soyasaponin monomers (A1, A2, and I) on the lipid profiles in HFD-induced obese mice and found that soyasaponins A1 and A2 decreased TC, TG, and LDL-C and soyasaponin I reduced TG and LDL-C; further, all the three soyasaponin monomers (A1, A2, and I) increased HDL-C.17 These studies suggest that both total soyasaponin crude extracts and some soyasaponin monomers have hypocholesterolemic effects; furthermore, different soyasaponin monomers exhibit varied abilities.
Secondly, existing evidence suggests that soyasaponins induce antithrombotic action. Total soyasaponins were shown to prevent a decrease in blood platelets and fibrinogen, as well as an increase in fibrin degradation products in the model of disseminated intravascular coagulation (DIC) induced by the infusion of endotoxin or thrombin in rats.14,28 Moreover, both total soyasaponins and soyasaponin monomers (I, II, A1, and A2) inhibited the conversion of fibrinogen to fibrin.14,28
Thirdly, inflammation plays an important role in the genesis and progression of atherosclerosis.3 A number of in vivo29,30 and in vitro31,32 investigations have shown that both total soyasaponin extracts and soyasaponin monomers (A1, A2, A3, Ab, or I) exhibit antiinflammatory activities by reducing proinflammatory cytokines (tumor necrosis factor a, TNF-a; interleukin 1β, IL-1β; interleukin 6, IL-6; monocyte chemoattractant protein 1, MCP-1; and intercellular adhesion molecule 1, ICAM-1) and/or mediators (nitric oxide, NO; inducible nitric oxide synthase, iNOS; cyclooxygenase 2, COX-2; and prostaglandin E2, PGE2), which have been effectively characterized to be associated with the development of atherosclerosis. Soyasaponins have also been revealed to inhibit the inflammatory toll-like receptor 4 (TLR4)/nuclear factor kappa B (NF-κB) signaling, which is highly involved in atherosclerosis.33–36
However, the antiatherosclerotic functionality of soyasaponins (particularly different monomers) still remains unknown, although the above existing evidence indicates that they may possess this functionality. Therefore, the aim of the present study was to investigate the effects of the supplementation of two types of soyasaponin monomers (A1 and A2) on atherosclerotic plaque formation, serum lipid profiles, and inflammation in ApoE−/− mice.
2 Materials and methods
2.1 Reagents
Soyasaponin monomers (A1 and A2) were prepared and purified by using the methods described earlier.32 Antibodies for myeloid differentiation primary response protein 88 (MyD88), β-actin, IκBα, phosphorylated IκBα (p-IκBα), NF-κB p65, and phosphorylated NF-κB p65 (p-p65Ser536) were purchased from Cell Signaling Technology (Danvers, MA, USA) and TLR4 was obtained from Sigma (Saint Louis, MO, USA). Rabbit anti-GAPDH was obtained from Good Here (Hangzhou, Zhejiang, China), and simvastatin was purchased from Solarbio Technology (Beijing, China). All the secondary antibodies used for western blotting were obtained from Rockland Immunochemical (Gilbertsville, PA, USA).
2.2 Animals
Sixty 5-week-old male ApoE gene knockout (ApoE−/−) C57BL/6J (B6) mice (no. 312024300001195) were purchased from Shanghai Southern Model Biological Research Center (Shanghai, China). After 1 week of adaptive feeding, the mice were randomly assigned to 6 groups (n = 10 each) and all the mice were fed with HFD containing 45% fat and providing energy of 4.73 kcal g−1 (no. D12451). The 1st group served as the control (HFD). Mice in the 2nd and 3rd groups were fed with HFD added with 10 μmol per kg BW and 20 μmol per kg BW of soyasaponin A1, respectively. Mice in the 4th and 5th groups were fed with HFD supplemented with 10 μmol per kg BW and 20 μmol per kg BW of soyasaponin A2, respectively. Mice in the 6th group were fed with HFD supplemented with 10 μmol per kg BW of simvastatin. The intervention dosage of soyasaponins was based on our earlier study, where the supplementation of soyasaponins with 10 μmol per kg BW or 20 μmol per kg BW in mice exhibited antiinflammatory and lipid profile improvement effects.17 The choice of simvastatin dosage was made by referring to the study by Song et al. (2011).37 The HFD was purchased from Guangdong Medical Laboratory Animal Center (Guangzhou, China) and did not contain any soy-based materials in order to avoid disturbance by any natural source of soyasaponins. Both simvastatin and soyasaponins were premixed into the feed. The experimental period was 24 weeks. All the mice were housed in standard cages, with 5 mice in each cage in a room maintained at 22 ± 1 °C on a 12 h/12 h light/dark cycle. Diets and ultrapure water were provided ad libitum. The body weight and feed consumption were recorded weekly. All the procedures involving animals and their care were approved by the Southern Medical University Animal Care and Use Committee (no. SMUA2017003).
2.3 Intraperitoneal glucose tolerance test (IPGTT)
One week before the end of the experiment, mice were fasted overnight and the IPGTT was administered. Each mouse was intraperitoneally injected with 2 mg g−1 BW of glucose. The blood glucose level was then measured at 0, 15, 30, 60, 90, and 120 min by using the Roche ACCU-CHEK Performa glucometer (Indianapolis, IN, USA).
2.4 Oil Red O staining for lesions in aortic root and aorta
At the end of the intervention experiment, following overnight fasting, the mice were anesthetized by inhaling ether in a closed box with a concentration-controlled valve and then sacrificed for sample collection. The heart was fully perfused by phosphate-buffered solution (PBS) and then carefully removed for the analysis of aortic root lesions. Cryostat sections across the aortic root were prepared and embedded in an optimal cutting temperature (OCT) compound. Serial sections (5 μm thick) were cut and collected until three valves appeared under the microscope. Five sections were placed onto each slide for a total of 4 slides. These slices were stained with Oil Red O and counterstained with Lillie–Mayer hematoxylin. An Olympus microscope was used to observe the pathological changes, and Image-Pro Plus 6.0 software was used for quantifying the atherosclerotic lesions.
The entire aorta was carefully separated after the removal of the heart under the dissecting microscope and then fixed with 4% paraformaldehyde for 48–72 h. The adipose tissues around the aorta were carefully peeled off. Then, the aorta was longitudinally opened with ophthalmic scissors, horizontally tiled, and fixed by using steel needles on the wax base of a black dish. The aorta was carefully washed three times by using PBS to remove any floating impurities. The aorta was stained with Oil Red O for 1 h at room temperature, rinsed with PBS thrice, and photographed with a Canon microscope. The Image-Pro Plus 6.0 software was used to analyze the proportion of red lipid plaques in the entire aorta.
2.5 Hematoxylin–eosin (HE) staining for innominate artery
After sacrifice, the innominate artery was carefully extracted from the aortic arch, fixed in 4% paraformaldehyde for more than 48 h, and then embedded in paraffin to make serial sections (thickness: 4 μm). A total of 24 sections (4 sections on each slide for a total of 6 slides) from the innominate arterial tissue sample of each mouse were chosen at regular intervals for HE staining. The pathological lesions in the innominate artery were observed by using an Olympus microscope and analyzed by the Image-Pro Plus 6.0 software.
2.6 Analysis of serum parameters
Following anesthetization, blood was collected from the retro-orbital plexus, and the serum was separated and stored at −80 °C. Commercially available enzyme-linked immunosorbent assay (ELISA) kits were employed to determine the fasting values for MCP-1 (NeoBioscience, China), TNF-a (Genetimes Technology, Shanghai, China), and high-sensitivity C-reactive protein (hs-crp, Nanjing Jiancheng Bioengineering Institute, China) in serum. The TC, TG, LDL-C, and HDL-C levels in serum were determined by using the corresponding commercial kits purchased from Nanjing Jiancheng Bioengineering Institute (Nanjing, China) according to the manufacturer's instructions.
2.7 Western blotting
Vascular tissues from each specimen were placed in a 1.5 mL EP tube. Then, the tissue was immersed into 0.5 mL PBS containing 1% phenylmethylsulfonyl fluoride (PMSF) and cut into pieces of about 3 mm × 3 mm by using ophthalmic scissors. The tissues in the tube were stirred 10–15 times and concentrated with the discarded PBS. The protocol was repeated 3 times. The tissue was then transferred to a glass homogenizer added with 150 μL cold radioimmunoprecipitation assay (RIPA) lysate containing 1% PMSF and 0.4% phosphatase inhibitor and then manually homogenized 15 times on ice until it was fully lysed. The tissue homogenate was then transferred to a pre-cooled 1.5 mL EP tube and centrifuged at 10
000 rpm for 5 min at 4 °C. The supernatant was retained. The bicinchoninic acid (BCA) method was used to determine the protein concentration.33
Western blotting was performed as described earlier.33 Briefly, a 1.0% thick Tris-glycine gel was prepared, and the tissue protein samples were sequentially added to each lane at loading of 20–40 μg; thereafter, the marker was added. Electrophoresis was carried out using a voltage of 80–120 V. The protein strip of the gel was then charged to a nitrocellulose membrane (Bio-Rad) at a voltage of 100 V. Proteins were quantified by immune blotting with primary and secondary antibodies. Fluorescent bands were visualized using an Odyssey infrared imaging system (Odyssey Fc, LI-COR).
2.8 Immunohistochemistry and immunofluorescence in tissue sections
A total of innominate artery paraffin sections (4 sections on each slide) from each mouse were used for immunohistochemistry staining to analyze the protein expression of TLR4 and MyD88. All the sections were placed in a drying oven for 2 h at 65 °C, dewaxed 3 times with xylene, dehydrated by gradient alcohol, and antigen-repaired by citrate buffer, tris-ethylenediaminetetraacetic acid (Tris-EDTA), pepsin, and trypsin. The primary and secondary antibodies were then added to induce the reaction. Following washing by PBS, slices were added with diaminobenzidine (DAB) for color development, hematoxylin for counterstaining, and alcohol containing 1% hydrochloric acid for differentiation. Then, the slices were dehydrated and made transparent by using gradient alcohol and xylene. The slides were finally sealed by neutral gum and dried by cold wind. Microscopy was used for observing the target protein that was stained in brown, while the nucleus was stained in blue. Image-Pro Plus 6.0 software was used to calculate the integrated optical density (IOD) and the sum of area (Area). The average optical density (AOD) was determined by using the formula AOD = IOD/Area.
The aortic root frozen sections from 4 mice (each mouse had a total of 16 sections on 4 slides with 4 sections each) of each group were used to analyze the protein expressions of TLR4 and MyD88 by using the immunofluorescence staining technology. After washing with PBS, slices were reacted with the primary antibody and then the corresponding fluorescent secondary antibody. 4,6-Diamidino-2-phenylindole (DAPI) was used to stain the nucleus. Under an Olympus fluorescence microscope, the target protein was stained in red and the nucleus, blue. Image-Pro Plus 6.0 software was used to characterize the pictures.
2.9 Statistical analysis
Statistical analyses were performed using the one-way analysis of variance (one-way ANOVA) by using SPSS 20.0 statistical software (SPSS, Chicago, IL, USA). All the results were expressed as means ± standard deviation (means ± S.D.). A p value less than 0.05 (p < 0.05) was considered to be statistically significant.
3 Results
3.1 Animal growth and feed consumption
ApoE−/− mice are a well-established model for atherosclerosis research. In this study, we fed all the mice with HFD and intervened some with soyasaponins. As shown in Fig. 1A, the mice gradually grew throughout the experimental period. Interestingly, intervention with 20 μmol per kg BW of soyasaponin A2 induced slower growth. At weeks 2, 4, 5, 6, 14, and 19, the mice fed with 20 μmol per kg BW of soyasaponin A2 had significantly lower BW than those in the control group (p < 0.05). However, no statistical differences involving the initial body weight (IBW), final body weight (FBW), and body weight gain (BWG) were found between the groups (Fig. 1B) (p > 0.05). As shown in Fig. 1C, the feed consumptions in both g per d per mice and kcal per d per mice were not statistically different between the different groups (p > 0.05). These results indicate that soyasaponin A1 and simvastatin did not affect the growth and feed intake of HFD-fed ApoE−/− mice. However, soyasaponin A2 may potentially cause weight loss without changing the food intake.
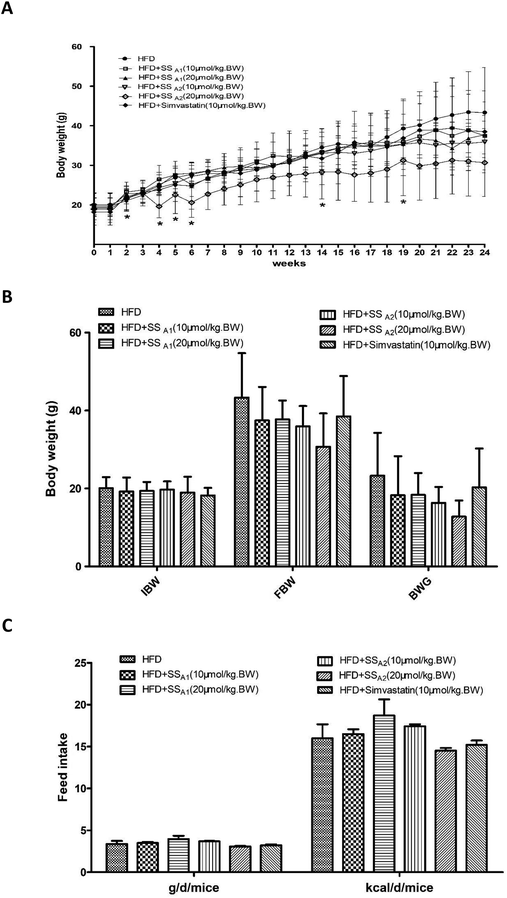 |
| Fig. 1 Effects of soyasaponins (SS) on animal growth and feed consumption in HFD-fed ApoE−/− mice. Body weight and feed intake were monitored once a week. (A) The growth curve was plotted. (B) The initial body weight (IBW), final body weight (FBW), and body weight gain (BWG) were calculated and presented. (C) The feed intake throughout the experimental period was calculated. Data were statistically analyzed by using one-way ANOVA with the SPSS 20.0 software. Results presented are expressed as means ± SD of the samples for ten (n = 10) mice in each group. The asterisk (*) means a significant difference as compared to the control (HFD) group (p < 0.05). | |
3.2 Soyasaponins reduce atherosclerotic plaques in innominate artery and aortic roots
ApoE−/− mice fed with HFD develop obvious atherosclerotic plaques in the large arteries. In this study, we characterized the atherosclerotic plaques in the aorta, innominate artery, and aortic root, which are the most important sites for the direct observation of atherosclerotic lesions. Atherosclerotic plaques in the innominate artery and aortic root of ApoE−/− mice were significantly reduced after intervention by soyasaponins (A1 and A2) for 24 weeks when compared to those in the mice from the control group (p < 0.05) (Fig. 2B–D). The ratios of atherosclerotic plaques among the different groups were not statistically different (Fig. 2A and D). Unexpectedly, simvastatin, a lipid-lowering drug that can be used to lower the risk of CVDs and manage abnormal lipid levels by inhibiting the endogenous cholesterol production in the liver, did not reduce atherosclerotic plaques in the aorta and innominate artery (p > 0.05) (Fig. 2A, B, and D), although it decreased that in the aortic root (p < 0.05) (Fig. 2C and D). These results suggest that soyasaponins (A1 and A2) exert antiatherosclerotic functionality in HFD-fed ApoE−/− mice.
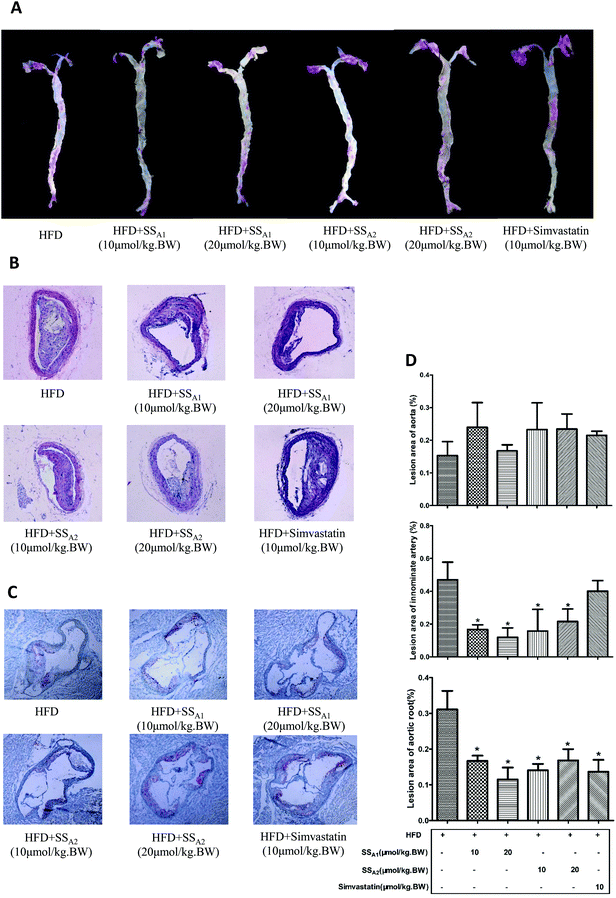 |
| Fig. 2 Effects of soyasaponins (SS) on atherosclerotic plaques in aorta, aortic root, and innominate artery in HFD-fed ApoE−/− mice. (A) Aorta was stained with Oil Red O and representative pictures are shown. (B) Innominate arterial tissues were paraffin-embedded, sliced, and then stained with hematoxylin–eosin (HE). (C) The aortic roots were OCT-embedded, sliced after being frozen, and then stained with Oil Red O. (D) The atherosclerotic lesions in the aorta, innominate artery, and aortic root were quantified by using methods as described in the Materials and methods section. Data were statistically analyzed by using one-way ANOVA with the SPSS 20.0 software. Results presented are expressed as means ± SD of the samples from four (n = 4) mice in each group. The asterisk (*) means a significant difference as compared to the control (HFD) group (p < 0.05). | |
3.3 Soyasaponins decrease hypercholesterolemia and promote fecal lipid excretion
It is well established that HFD-induced disorder of lipid metabolism pivotally contributes toward atherosclerosis. Here, we analyzed the lipid profiles (TC, TG, LDL-C, and HDL-C) in serum and lipids excretion (TG, TC, and bile acids) in feces. Soyasaponin A1 (10 and 20 μmol per kg BW) significantly decreased the TG, TC, and LDL-C levels (p < 0.05) and increased the HDL-C (p < 0.05) level in serum (Fig. 3A). Soyasaponin A2 (10 and 20 μmol per kg BW) significantly decreased the TC and LDL-C levels (p < 0.05); further, only 20 μmol per kg BW of soyasaponin A2 reduced the TG level (p < 0.05) in serum. Soyasaponin A2 did not affect the HDL-C level in serum (p > 0.05) (Fig. 3A). Simvastatin significantly reduced the LDL-C level (p < 0.05) and increased the HDL-C level (p < 0.05) in serum. However, it did not affect the TG and TC levels in serum (p > 0.05) (Fig. 3A). A further analysis of the lipids in feces (Fig. 3B) revealed that 20 μmol per kg BW of soyasaponin A1 significantly increased the level of TG in feces (p < 0.05). Further, 10 μmol per kg BW of soyasaponin A2 significantly enhanced the TC level in feces as compared to that in the control (p < 0.05). Moreover, 20 μmol per kg BW of soyasaponin A1 and A2 significantly increased the level of bile acids in feces (p < 0.05). Simvastatin did not induce a significant change (p > 0.05) in the TG, TC, and bile acid levels in feces, although it had the tendency (p = 0.13) to increase the TC level in feces. These results show that soyasaponin (A1 and A2) intervention can decrease hypercholesterolemia probably by promoting fecal lipids excretion in HFD-fed ApoE−/− mice.
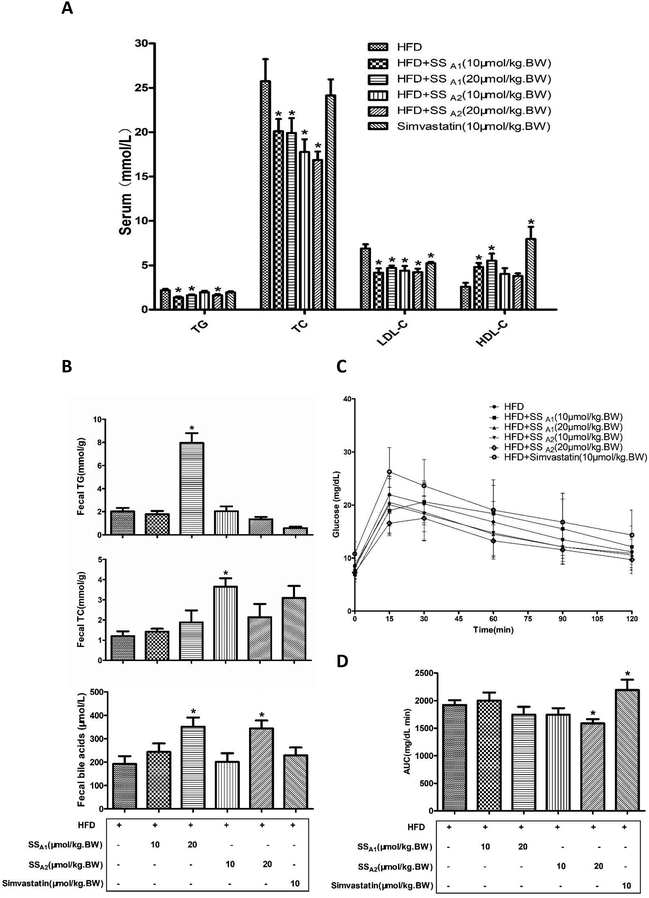 |
| Fig. 3 Effects of soyasaponins (SS) on serum lipid profile, fecal lipid excretion, and blood glucose level in HFD-fed ApoE−/− mice. The levels of lipid profile (TG, TC, LDL-C, and HDL-C) in serum (A) and fecal excretion of lipids (TG, TC, and bile acids) (B) were determined by commercial kits. Intraperitoneal glucose tolerance test (IPGTT) (C) was performed and the area under the curve (AUC) (D) was, therefore, calculated. Data were statistically analyzed by using one-way ANOVA with the SPSS 20.0 software. Results presented are expressed as means ± SD of the samples from ten (n = 10) mice in each group. The asterisk (*) means a significant difference as compared to the control (HFD) group (p < 0.05). | |
We further performed IPGTT to measure the blood glucose metabolism in mice, since glucose metabolic dysfunction also plays a very important role in atherosclerosis. As shown in Fig. 3C and D, 20 μmol per kg BW of soyasaponin A2 significantly reduced the area under the curve (AUC) of IPGTT in HFD-fed ApoE−/− mice (p < 0.05). Interestingly, simvastatin significantly increased the AUC in HFD-fed ApoE−/− mice as compared to that in the control (p < 0.05).
3.4 Soyasaponins reduce inflammatory markers in serum
It is well recognized that atherosclerosis is a chronic inflammatory disease that occurs within the arterial wall. In this study, the inflammatory markers (TNF-a, MCP-1, and hs-crp) in serum were analyzed by using commercial ELISA kits. As shown in Fig. 4A, soyasaponin A1 (10 and 20 μmol per kg BW) and soyasaponin A2 (20 μmol per kg BW) significantly decreased the TNF-α level in serum as compared to that in the control group (p < 0.05). However, simvastatin did not significantly affect the TNF-α level in serum (p > 0.05). Moreover, both soyasaponins (A1 and A2) and simvastatin lowered the levels of MCP-1 and hs-crp (Fig. 4B and C) in serum (p < 0.05). These results indicate that soyasaponins can reduce inflammation in HFD-fed ApoE−/− mice.
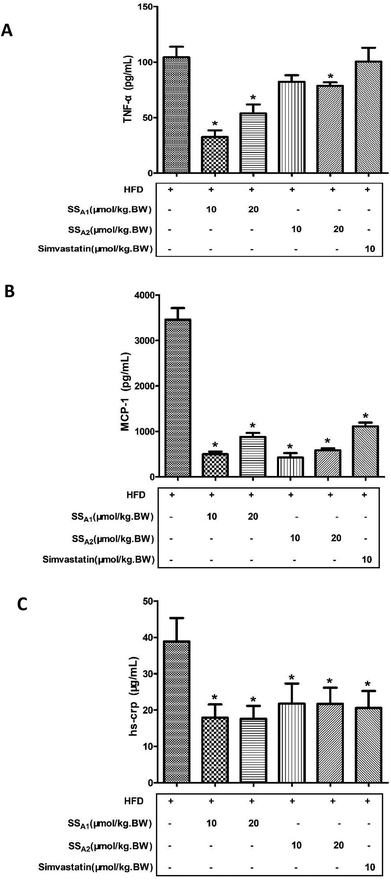 |
| Fig. 4 Effects of soyasaponins (SS) on inflammatory markers in serum. The levels of TNF-α (A), MCP-1 (B), and hs-crp (C) were determined by using commercial ELISA kits. Data were statistically analyzed by using one-way ANOVA with the SPSS 20.0 software. Results presented are expressed as means ± SD of the serum samples from seven (n = 7) mice in each group. The asterisk (*) means a significant difference as compared to the control (HFD) group (p < 0.05). | |
3.5 Soyasaponins downregulate TLR4/MyD88/NF-κB signaling in aorta and innominate artery
TLR4/MyD88/NF-κB is a classical signaling pathway that mediates the inflammatory response and is closely related to the development of atherosclerosis. Here, we detected the expression of key molecules in the TLR4/MyD88/NF-κB signaling pathways in the aorta, aortic root, and innominate artery. As shown in Fig. 5(A and B), soyasaponin A1 (10 and 20 μmol per kg BW) and soyasaponin A2 (20 μmol per kg BW) significantly reduced (p < 0.05) the protein expression levels of TLR4 and MyD88 in the aortic tissues as compared to those in the control group. However, 10 μmol per kg BW of soyasaponin A2 did not significantly change the TLR4 and MyD88 levels (p > 0.05). Simvastatin lowered the MyD88 level (p < 0.05), while it did not affect the TLR4 expression in aortic tissues (p > 0.05). Furthermore, both soyasaponins (A1 and A2) and simvastatin reduced (p < 0.05) the phosphorylation of IκBα and NF-κB p65 in the aortic tissues (Fig. 5C and D).
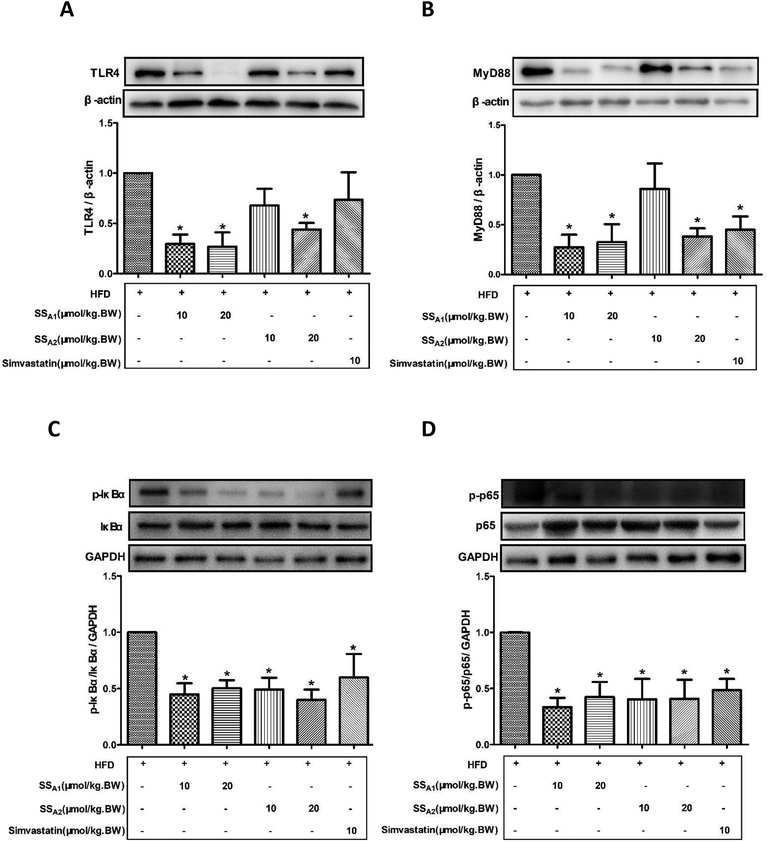 |
| Fig. 5 Effects of soyasaponins (SS) on TLR4/MyD88/NF-κB inflammatory signaling in aorta. The protein expressions of key molecules (TLR4, MyD88, p-IκBα, IκBα, p-p65, and p65) in the TLR4/MyD88/NF-κB inflammatory signaling pathway in aortic tissues were determined by western blotting. Data were statistically analyzed by using one-way ANOVA with the SPSS 20.0 software. Results presented are expressed as means ± SD of the aortic tissue samples from four (n = 4) mice in each group. The asterisk (*) means a significant difference as compared to the control (HFD) group (p < 0.05). | |
Fig. 6 shows the TLR4 and MyD88 expressions in the innominate artery as determined by using immunohistochemical staining. Both soyasaponins (A1 and A2) and simvastatin reduced (p < 0.05) the expressions of TLR4 (Fig. 5A and C) and MyD88 (Fig. 5B and D) in the innominate artery.
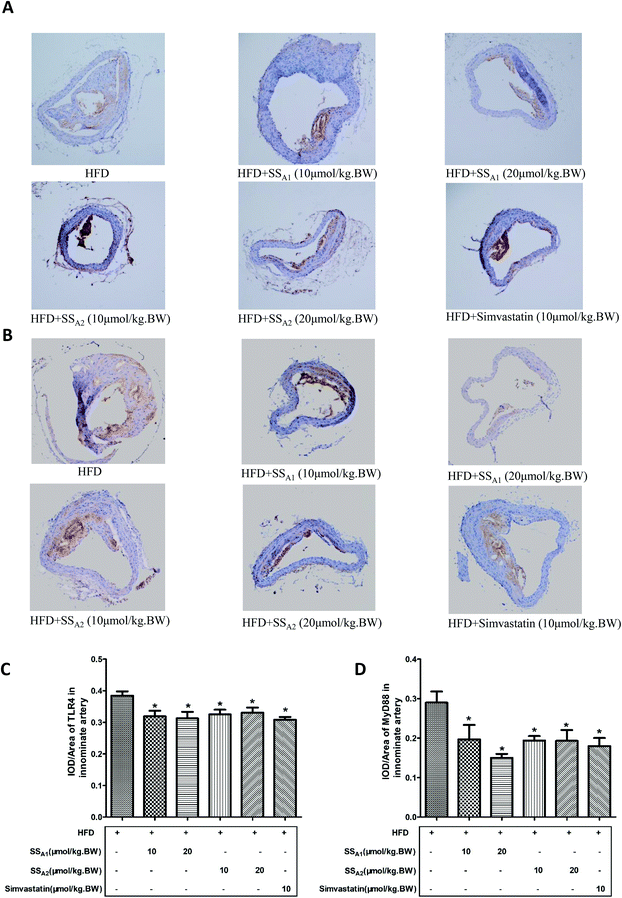 |
| Fig. 6 Effects of soyasaponins (SS) on the expressions of TLR4 and MyD88 in the innominate artery. The protein expressions of TLR4 (A and C) and MyD88 (B and D) in the TLR4/MyD88/NF-κB inflammatory signaling pathway in paraffin tissue slices of the innominate artery was determined by immunohistochemical staining. Data were statistically analyzed by using one-way ANOVA with the SPSS 20.0 software. Results presented are expressed as means ± SD of the innominate arterial tissue samples from four (n = 4) mice in each group. The asterisk (*) means a significant difference as compared to the control (HFD) group (p < 0.05). | |
As shown in Fig. 7, the expression levels of TLR4 and MyD88 in the aortic root were determined by the immunofluorescence technique. Soyasaponins (A1 and A2) and simvastatin had the tendency to reduce the expressions of TLR4 (Fig. 7A) and MyD88 (Fig. 7B) in the aortic root, although an accurate quantification was not made because of the different immunofluorescence backgrounds on every slice.
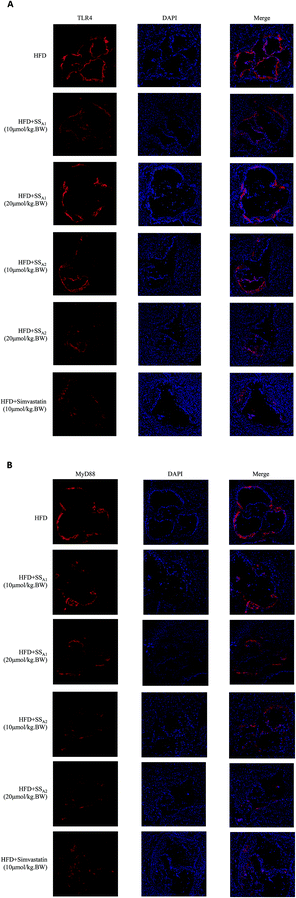 |
| Fig. 7 Effects of soyasaponins (SS) on the expressions of TLR4 and MyD88 in the aortic root. The protein expressions of TLR4 (A) and MyD88 (B) in the TLR4/MyD88/NF-κB inflammatory signaling pathway in frozen sections of the aortic root tissues were determined by means of the immunofluorescence technique. Representative pictures are shown from all the pictures of the aortic root tissues from four (n = 4) mice in each group. | |
Taken together, these results show that soyasaponins (A1 and A2) can downregulate TLR4/MyD88/NF-κB-mediated inflammation in the arterial tissues of HFD-fed ApoE−/− mice.
4 Discussions
Atherosclerosis is a progressive chronic inflammatory disease characterized by the accumulation of lipids and fibrous elements in the large arteries.38 In the recent years, food-derived phytochemicals have been shown to play effective roles in the prevention of atherosclerosis.20,39 Soyasaponins are important phytochemicals rich in soy and its products.33 Existing evidence has shown that soyasaponins have hypocholesterolemic and antiinflammatory bioactivities, which may contribute toward its potential for preventing atherosclerosis.14,30–33 However, till date, no direct evidence is available that can support the antiatherosclerotic bioactivities of soyasaponins. Here, we investigated the effects of soyasaponins on atherosclerosis by using the well-established ApoE−/− mice model and made some novel findings.
The primary finding of the present study is that soyasaponins (A1 and A2) can decrease plaque formation in the HFD-fed ApoE−/− mice model of atherosclerosis. It is well known that HFD feeding can induce the formation of wide and typical atherosclerotic plaques in ApoE−/− mice.40 In the present study, we characterized the atherosclerotic plaques in the aorta, innominate artery, and aortic root, which are the three most important sites for assessing atherosclerosis in ApoE−/− mice.41 Soyasaponin (A1 and A2) intervention on HFD-fed ApoE−/− mice for 24 weeks significantly reduced the atherosclerotic plaques in the innominate artery and aortic root. However, soyasaponins did not significantly decrease the plaques in the aorta. In HFD-fed ApoE−/− mice, atherosclerotic plaques usually appear earlier in the innominate artery and aortic roots than in that in the aorta, and the appearance of plaques in the innominate artery is even ahead of that in the aortic roots.42,43 Aortic lesions often appear in advanced atherosclerotic lesions, and the plaques are mainly distributed in the aortic arch, lumbar artery opening, and left and right common iliac arteries.44 Here, following soyasaponin intervention for 24 weeks, there was a significant reduction in plaque formations in the innominate artery and aortic root, but not in the aorta. This is probably because the plaque lesions formed in ApoE−/− mice are still at an early stage in the present study. Furthermore, the quantification of plaques in the entire aorta—if advanced plaques are not developed—is usually not as accurate as that in the innominate artery and aortic root.45 Moreover, the present results show that soyasaponins (A1 and A2) can indeed reduce the formation of atherosclerotic plaques in HFD-fed ApoE−/− mice. To the best of our knowledge, this is the first in vivo study that provides direct evidence for supporting the antiatherosclerotic bioactivities of soyasaponins. Earlier studies have shown that saponins from other sources prevent atherosclerosis.20,46 Simvastatin is the most famous antithrombotic medicine usually used for clinically preventing atherosclerosis.47 Unexpectedly, in this research, it decreased plaques in the aortic root, while did not reduce atherosclerotic plaques in the aorta and innominate artery. This may be because of two possible reasons. On one hand, it has been shown that the effect of simvastatin is highly correlated with its absorption efficiency.48 In the current study, a simvastatin dosage of 10 μmol per kg BW was administrated to the mice by mixing it into the feed and not by intragastric gavage, which may not guarantee good absorption to achieve its effective concentration in vivo. A high dosage of simvastatin warrants further testing so that we can observe its significant effects in the future. In the early and intermediate stages of atherosclerosis, low-dose simvastatin intervention may not be sufficient to reduce plaque formation in the aorta within 24 weeks. At the same time, the quantification of plaques in the entire aorta is usually not as accurate as those in the innominate artery and aortic root if advanced plaques are not developed.49 On the other hand, it has been shown that the innominate artery is a common site of plaque rupture, and simvastatin may promote the stability of atherosclerotic plaque in ApoE−/− mice, resulting in no quantitative changes in the formation of plaques.44,50,51 Therefore, a study on soyasaponin and simvastatin interventions using intragastric administration for a longer experimental period can be worthwhile. In addition, we found that soyasaponin A2 might have the potential to decrease the body weight of HFD-fed ApoE−/− mice. A recent study suggested that group B soyasaponin aglycone exhibited an antiobesity effect by lowering the weight of fat adipose tissue and increasing the ratio of muscle to whole body weight in HFD-fed C57/BL6 mice.52 The decrease in body weight (particularly fat mass) contributes toward improving metabolism and reducing inflammation, facilitating atherosclerosis prevention. Therefore, whether the antiatherosclerotic effect of soyasaponins is associated with their antiobesity function or not requires further investigations.
Second, soyasaponins prevent atherosclerosis by improving the serum lipid profile in HFD-fed ApoE−/− mice. It is well established that lipid metabolic disorder is an important risk factor for atherosclerosis.53 Cholesterol crystals and cell debris are the major components of atherosclerotic plaque. Lowering the levels of cholesterol and fat in serum by a dietary or pharmaceutical method has been proven to be an effective prerequisite for reducing atherosclerotic plaque formation in ApoE−/− mice.54 A series of large-scale clinical trials have revealed that correcting hypercholesterolemia profoundly reduces morbidity and mortality from atherosclerosis-associated CHD.55,56 In this study, soyasaponins (A1 and A2) improved the serum lipid profile by decreasing the TG, TC, and LDL-C levels, as well as increasing HDL-C levels, in HFD-fed ApoE−/− mice, which may contribute toward their antiatherosclerotic effects. This is in accordance with earlier studies from our lab and others regarding the hypocholesterolemic effects of soyasaponins. Xiao et al. (2005) revealed that the total soyasaponins significantly decreased the TC, LDL-C, and TG levels, as well as increased the HDL-C level, in serum in mice.25 Lee et al. (2005) found that hamsters fed with group B soyasaponins lowered the plasma TC by 20%, non-HDL-C by 33%, and TG by 18% as compared to those fed with casein.26 Our recent study found that in HFD-fed obese mice, soyasaponins A1 and A2 decreased TC by 33.3% and 27.4%, TG by 55.7% and 61.2%, and LDL-C by 41.1% and 52.1%, respectively. Soyasaponin I reduced TG by 66.1% and LDL-C by 49.3%. Soyasaponins A1, A2, and I increased HDL-C by 132.5%, 87.5%, and 120.6%, respectively.17 According to many clinical studies, the presence of soyasaponins in food was shown to be effective in reducing cholesterol in humans.22,57 The abilities of soyasaponins to improve the blood lipid profile have been, to a large extent, attributable to their characteristic to form insoluble complexes with cholesterol, as well as to interfere with bile acid metabolism in the gastrointestinal tract, and therefore, to promote the excretion of cholesterol in feces.23,24,26,58 Our study, along with previously published investigations,17,22,23 supports the fact that soyasaponins can increase the fecal excretion of cholesterol and bile acids. However, other mechanisms may possibly be involved in the abilities of soyasaponins to improve the serum lipid profile since the study by Xiao et al. revealed that soyasaponins increased the HFD-induced reduction of lipoprotein lipase (LPL) mRNA expression in mice.25 Simvastatin is a competitive inhibitor of the rate-limiting enzyme 3-hydroxy-3-methyl-glutaryl coenzyme A (HMG-CoA) reductase. It restricts the endogenous synthesis of cholesterol in the liver and therefore reduces LDL and raises HDL; it has been widely used in the clinical therapy of hyperlipidemia and atherosclerosis.59 Song et al. (2011) found simvastatin significantly decreased the plaque area of the aortic arch and aortic root in ApoE−/− mice by reversing cholesterol transport and improving HDL-C level.37 However, Sparrow et al. (2001) found that simvastatin exhibited antiinflammatory and antiatherosclerotic activities without lowering plasma cholesterol.48 In addition, Liu et al. (2013) found that simvastatin markedly attenuated vascular inflammation and atherosclerotic lesion areas in ApoE−/− mice by decreasing the aortic expression of VCAM-1 and MCP-1, while it did not affect the abnormal TC and TG levels in serum.60,77 This is consistent with our current findings, which indicates that simvastatin induces an antiatherosclerotic activity by reducing LDL-C and independent of the reduction in the TC and TG levels.
Interestingly, 20 μmol per kg BW of soyasaponin A2 improved glucose tolerance in ApoE−/− mice in this study. This is consistent with our recent research in HFD-induced obese C57BL/6J (B6) mice.17 Several studies have shown that total soyasaponins60,61 and groups B, E, and DDMP soyasaponins62,63 exhibited bioactivities to improve glucose homeostasis. However, the hypoglycemic effects of different soyasaponin monomers and whether such effects contribute toward atherosclerosis prevention or not necessitate further detailed investigations.
The last finding of this study is that soyasaponins prevent atherosclerosis by inhibiting the TLR4/MyD88/NF-κB-mediated inflammation in HFD-fed ApoE−/− mice. Atherosclerosis is a progressive disease that induces oxidative stress, inflammation, foam cell formation, and fibrous cap coverage.2–4,38 It is well known that LDL is the main carrier for the transport of endogenous cholesterol in the body. LDL accumulation in blood makes it easier to be oxidized to produce excessive Ox-LDL.4 Ox-LDL, which has been widely recognized as an independent risk factor of atherosclerosis, injures the vascular endothelium and initiates inflammatory responses, thereby recruiting mononuclear macrophages to phagocytose Ox-LDL to form foam cells and deposit in the intima of blood vessels, initiating atherosclerosis.2–4 In these complicated processes, a large number of inflammatory mediators (TNF-a, MCP-1, hs-crp, VCAM-1, etc.) are produced and involved in the vascular endothelial injury formation of plaques.3,64 Following the proliferation and migration of smooth muscle cells, the fiber caps increasingly thicken, and the atherosclerotic lesions advance and finally block the blood vessels.2,3,38 All these studies suggest that inflammation plays a very important role in the development of atherosclerosis. In this study, soyasaponins (A1 and A2) decreased the levels of TNF-α, MCP-1 and hs-crp in serum for HFD-fed ApoE−/− mice. TNF-α is a potent inflammatory cytokine, mainly produced by activated macrophages and T-cells, with a broad range of biological activities.64,65 TNF-α is highly involved in several aspects during the development of atherosclerosis. For example, TNF-α is one of the cytokines that can promote foam cell formation in vivo.64 Further, hs-crp is an inflammatory marker that responds to damage to arterial wall tissues. It is closely related to the development of atherosclerosis and an independent risk factor for CVDs.66 MCP-1 is an important cytokine secreted by chemotactic monocytes and macrophages. It plays important roles in the phagocytosis of macrophages and the recruitment of macrophages to the endothelium in the initiation of atherosclerosis.67 Here, the abilities of soyasaponins to decrease TNF-α, MCP-1, and hs-crp in serum of HFD-fed ApoE−/− mice indicate that soyasaponins prevent atherosclerosis by inhibiting inflammation. In the recent years, the antiinflammatory bioactivities of soyasaponins have been documented by means of both in vitro and in vivo studies.29–33 Soyasaponins can reduce the production of proinflammatory cytokines (TNF-a, IL-6, and IL-1β), and/or inhibit the inflammatory enzymes/mediators (iNOS, NO, COX-2, and PGE2),29–34 or repress the antigen-specific activation of T helper cells.68 Total soyasaponins have been shown to inhibit TNF-α and MCP-1 in LPS-stimulated macrophages.31 Soyasaponin I can reduce TNF-α in LPS-stimulated macrophages32 and TNBS-induced colitic mice.29 Soyasaponin A3 and soyasapogenols (B and C) can inhibit the TNF-α-induced expression of ICAM-1 in THP-1 monocytic leukemia cells.69 The uncovered mechanisms underlying the antiinflammatory properties of soyasaponins are associated with their inhibitory modulation of the signaling pathways of TLR4/NF-κB,29,30,32,33 phosphoinositide 3-kinase/protein kinase B (PI3K/Akt),33 and mitogen-activated protein kinases (MAPKs).29,30,34 Recently, we revealed a novel antiinflammatory mechanism of soyasaponin Bb, which can inhibit the recruitment of TLR4 into lipid rafts and its signaling pathway by suppressing the nicotinamide adenine dinucleotide phosphate (NADPH)-oxidase-dependent generation of reactive oxygen species (ROS).34
The TLR4/MyD88/NF-κB signaling pathway has been shown to play important roles in chronic inflammation and atherosclerosis.70 Toll-like receptors (TLRs), particularly TLR4, are the most widely and extensively studied pattern recognition receptors. TLR4 signaling plays a critical role in promoting atherosclerosis, as evidenced by the studies that reveal that the whole-body deletion of TLR4 confers protection from hypercholesterolemia-induced atherosclerosis.70 TLR4 is activated in response to ligands like lipopolysaccharide (LPS) from Gram-negative bacteria, glycoproteins, saturated fatty acids, etc. MyD88 is one of the major adaptor molecules channeling the signaling of TLR4.34,71 The inactivation of MyD88 has been shown to reduce atherosclerosis by reducing macrophage recruitment to the artery wall and decreasing chemokine levels. The whole-body genetic ablation of MyD88 has also been shown to inhibit atherosclerosis development in hypercholesterolemic mice.72 During inflammation, TLR4/MyD88 signaling results in the downstream activation of NF-κB. NF-κB is a transcription factor that regulates inflammation and many other immune responses. In the resting situation, NF-κB is located in the cytoplasm and is bound to IκB (a and β), which prevents it from entering the nuclei. Following stimulation, IκB is phosphorylated and rapidly degraded by proteasomes. The NF-κB subunits (p65, p50, or others) are released from IκB. Then, p65 is phosphorylated and translocated into the nuclei, where it binds to specific sequences in the promoter regions of the target genes.73 Activated NF-κB has been detected in situ in human atherosclerotic plaques, but it is absent or nearly absent in healthy vessels.72 Numerous studies have shown that phytochemicals exert antiatherosclerotic activities by regulating the TLR4/MyD88/NF-κB signaling pathways.74,75 Here, we examined the key proteins in the TLR4/MyD88/NF-κB signaling pathway in the aorta, innominate artery, and aortic root in order to reveal the possible mechanisms underlying the antiatherosclerotic activities of soyasaponins. Soyasaponins (A1 and A2) significantly reduce the protein expression levels of TLR4 and MyD88 and reduce the phosphorylation of IκBα and NF-κB p65 in aortic tissues in HFD-fed ApoE−/− mice. Furthermore, soyasaponins decreased the expressions of TLR4 and MyD88 in the innominate artery and exhibited a tendency to reduce them in the aortic root. These results indicate that soyasaponins can downregulate TLR4/MyD88/NF-κB signaling in arterial tissues in HFD-fed ApoE−/− mice. The regulation of various soyasaponins on TLR4/MyD88/NF-κB signaling has been previously revealed by a number of studies. Total soyasaponins have been shown to inhibit IκBa degradation in LPS-stimulated macrophages31 and in colon cancer HT-29 cells.76 Lee et al. showed that soyasaponin Ab ameliorated colitis—a chronic inflammatory disease—by inhibiting the expression of TLR4 and the phosphorylation of IRAK1, IKKβ, and p65 in TNBS-induced colitic mice.30 In LPS-stimulated macrophages, soyasaponin Ab inhibited IκBa degradation and the binding of LPS to TLR430 and soyasaponin I repressed IκBa phosphorylation and NF-κB activity.29,32 Recently, we found that soyasaponin Bb inhibited the recruitments of TLR4, MyD88, and TRIF into lipid raft fractions as well as TLR4 interaction with MyD88 or TRIF in the LPS-stimulated RAW264.7 macrophages.34 Our unpublished data also show that soyasaponins can repress LPS- or palmitate-stimulated MyD88 expressions in macrophages.
In summary, this study shows that soyasaponins (A1 and A2) exert antiatherosclerotic functionality by decreasing hypercholesterolemia and inflammation in HFD-fed ApoE−/− mice. This study contributes toward the novel understanding of the bioactivities of soyasaponins.
Abbreviations
Akt | Protein kinase B |
ApoE−/− | Apolipoprotein E knockout |
COX-2 | Cyclooxygenase 2 |
DAB | Diaminobenzidine |
DDMP | 2,3-Dihydro-2,5-dihydroxy-6-methyl-4H-pyran-4-one |
HDL-C | High-density lipoprotein cholesterol |
HE | Hematoxylin–eosin |
HFD | High-fat diet |
Hs-crp | Hypersensitive C-reactive protein |
ICAM-1 | Intercellular adhesion molecule 1 |
IκBα | Nuclear factor of kappa light polypeptide gene enhancer in B-cells inhibitor, alpha |
IL-1β | Interleukin 1 beta |
iNOS | Inducible nitric oxide synthase |
IPGTT | Intraperitoneal glucose tolerance test |
LDL-C | Low-density lipoprotein cholesterol |
LPS | Lipopolysaccharide |
MAPKs | Mitogen-activated protein kinases |
MCP-1 | Monocyte chemoattractant protein 1 |
NADPH | Nicotinamide adenine dinucleotide phosphate |
NF-κB | Nuclear factor kappa B |
MyD88 | Myeloid differentiation factor 88 |
Ox-LDL | Oxidized low-density lipoprotein |
PBS | Phosphate-buffered solution |
RIPA | Radioimmunoprecipitation assay |
TLR4 | Toll-like receptor 4 |
TNF-α | Tumor necrosis factor a |
VCAM-1 | Vascular cell adhesion molecule-1 |
Author contributions
The contributions of the authors to this study are as follows: L. Zha was responsible for the study concept and design; Q. Xie performed the histological, immunohistochemical and immunofluorescent analysis of artery tissues; X. Wu did the ELISA analysis of inflammatory markers; F. Xiong conducted the western blotting; J. Chen determined the levels of cholesterol, bile acids and triglycerides in serum and feces; X. Gu did the Oil red staining; C. Su, L. Xiao, and Z. Zheng performed the animal experiments (feeding, growth monitoring and soyasaponins intervention); Y. Wei and H. Ullah did the IPGTT; Q. Xie, J. Chen, and L. Zha carried out the statistical analysis and interpretation of data; Q. Xie and L. Zha wrote the manuscript; all authors read and approved the final manuscript.
Conflicts of interest
The authors declare no conflict of interest in the manuscript.
Acknowledgements
This work was supported by grants from National Natural Science Foundation of China (NSFC) (No. 81573125, 81872621 and 81102130), the Natural Science Foundation of Guangdong Province, China (No. 2014A030313313), the Research Start-up Plan of Southern Medical University (No. CX2016N012), and the Scientific Enlightenment Plan of Southern Medical University (No. B219339024).
References
- World Health Organization, Cardiovascular diseases (CVDs): Key facts. 2017, https://www.who.int/en/news-room/fact-sheets/detail/cardiovascular-diseases-(cvds).
- A. J. Lusis, Atherosclerosis, Nature, 2000, 407(6801), 233–241 CrossRef CAS PubMed.
- S. Taleb, Inflammation in atherosclerosis, Arch. Cardiovasc. Dis., 2016, 109(12), 708–715 CrossRef.
- A. J. Kattoor, N. V. Pothineni, D. Palagiri and J. L. Mehta, Oxidative stress in atherosclerosis, Curr. Atheroscler. Rep., 2017, 19(11), 42 CrossRef PubMed.
- N. Torres, M. Guevara-Cruz, L. A. Velázquez-Villegas and A. R. Tovar, Nutrition and atherosclerosis, Arch. Med. Res., 2015, 46, 408–426 CrossRef CAS PubMed.
- L. Y. Zhang, L. Ding, H. H. Shi, J. Xu, C. H. Xue, T. T. Zhang and Y. M. Wang, Eicosapentaenoic aicd in the form of phospholipids exerts superior anti-atherosclerosis effects to its triglyceride form in ApoE-/- mice, Food Funct., 2019, 10, 4177 RSC.
- T. T. Zhang, J. Xu, Y. M. Wang and C. H. Xue, Health benefits of dietary marine DHA/EPA-enriched glycerophospholipids, Prog. Lipid Res., 2019, 75, 100997 CrossRef CAS PubMed.
- T. Wang, C. Xue, T. Zhang and Y. Wang, The improvement of functional ingredients from marine foods in lipid metabolism, Trends Food Sci. Technol., 2018, 81, 74–89 CrossRef CAS.
- L. Ding, T. T. Zhang, H. X. Che, L. Y. Zhang, C. H. Xue, Y. G. Chang and Y. M. Wang, Saponins of sea cucumber attenuate atherosclerosis in ApoE-/- mice via lipid-lowering and anti-inflammatory properties, J. Funct. Foods, 2018, 48, 490–497 CrossRef CAS.
- A. M. Ruiz-León, M. Lapuente, R. Estruch and R. Casas, Clinical advances in immunonutrition and atherosclerosis: a review, Front. Immunol., 2019, 10, 837 CrossRef PubMed.
- K. D. Setchell, Phytoestrogens: the biochemistry, physiology, and implications for human health of soy isoflavones, Am. J. Clin. Nutr., 1998, 68(6 suppl), 1333S–1346S CrossRef CAS PubMed.
- I. Torre-Villalvazo, F. Gonzalez, C. A. Aguilar-Salinas, A. R. Tovar and N. Torres, Dietary soy protein reduces cardiac lipid accumulation and the ceramide concentration in high-fat diet-fed rats and ob/ob mice, J. Nutr., 2009, 139(12), 2237–2243 CrossRef CAS PubMed.
- S. Nagarajan, Mechanisms of anti-atherosclerotic functions of soy-based diets, J. Nutr. Biochem., 2010, 21(4), 255–260 CrossRef CAS.
- J. Kang, T. M. Badger, M. J. J. Ronis and X. Wu, Non-isoflavone phytochemicals in soy and their health effects, J. Agric. Food Chem., 2010, 58, 8119–8133 CrossRef CAS.
- O. Güçlü-Ustündağ and G. Mazza, Saponins: properties, applications and processing, Crit. Rev. Food Sci. Nutr., 2007, 47(3), 231–258 CrossRef PubMed.
- B. Singh, J. P. Singh, N. Singh and A. Kaur, Saponins in pulses and their health promoting activities: a review, Food Chem., 2017, 233, 540–549 CrossRef CAS PubMed.
- Q. Y. Xie, X. F. Gu, J. B. Chen, M. S. Liu, F. Xiong, X. L. Wu, Y. J. Zhang, F. P. Chen, H. R. Chen, M. J. Li, S. X. Sun, X. W. Chu and L. Y. Zha, Soyasaponins reduce inflammation and improve lipid and glucose homeostasis in high fat diet-induced obese mice, Mol. Nutr. Food Res., 2018, 62(19), e1800205 CrossRef.
- D. G. Oakenfull and G. S. Sidhu, Prevention of dietary hypercholesterolaemia by chickpea saponins and navy beans, Proc. Nutr. Soc. Aust., 1984, 9, 104–106 Search PubMed.
- M. R. Malinow, P. McLaughlin, H. K. Naito, L. A. Lewis and W. P. McNulty, Effects of alfalfa meal on shrinkage (regression) of atherosclerotic plaques during cholesterol feeding in monkeys, Atherosclerosis, 1978, 30, 27–43 CrossRef CAS.
- P. Zhou, W. Xie, Y. Luo, S. Lu, Z. Dai, R. Wang, X. Zhang, G. Li, G. Sun and X. Sun, Inhibitory Effects of Ginsenoside Rb1 on Early Atherosclerosis in ApoE-/- Mice via Inhibition of Apoptosis and Enhancing Autophagy, Molecules, 2018, 23, 2912 CrossRef PubMed.
- P. Leterme, Recommendation by health organizations for pulse consumption, Br. J. Nutr., 2002, 88(3), S239–S242 CrossRef CAS PubMed.
- J. D. Potter, D. L. Topping and D. Oakenfull, Soy, saponins, and plasma cholesterol, Lancet, 1979, 1, 223 CrossRef CAS.
- D. G. Oakenfull and D. L. Topping, Saponins and plasma cholesterol, Atherosclerosis, 1983, 48, 301–303 CrossRef CAS.
- D. Oakenfull and G. S. Sidhu, Could saponins be a useful treatment for hypercholesterolaemia, Eur. J. Clin. Nutr., 1990, 44, 79–88 CAS.
- J. X. Xiao, G. H. Peng and S. H. Zhang, Prevention effects of soyasaponins on hyperlipidemia mice and its molecular mechanism, Acta Nutr. Sin., 2005, 27, 147–150 CAS.
- S. O. Lee, A. L. Simons, P. A. Murphy and S. Hendrich, Soyasaponins lowered plasma cholesterol and increased fecal bile acids in female golden Syrian hamsters, Exp. Biol. Med., 2005, 230, 472–478 CrossRef CAS.
- K. Hiwatashi, H. Shirakawa, K. Hori, Y. Yoshiki, N. Suzuki, M. Hokari, M. Komai and S. Takahashi, Reduction of blood pressure by soybean saponins, renin inhibitors from soybean, in spontaneously hypertensive rats, Biosci. Biotechnol. Biochem., 2010, 74, 2310–2312 CrossRef CAS PubMed.
- M. Kubo, H. Matsuda, T. Tani, K. Namba, S. Arichi and I. Kitagawa, Effects of soyasaponin on experimental disseminated intravascular coagulation, Chem. Pharm. Bull., 1984, 32, 1467–1471 CrossRef CAS PubMed.
- I. A. Lee, Y. J. Park, H. K. Yeo, M. J. Han and D. H. Kim, Soyasaponin I attenuates TNBS-induced colitis in mice by inhibiting NF-κB pathway, J. Agric. Food Chem., 2010, 58, 10929–10934 CrossRef CAS PubMed.
- I. A. Lee, Y. J. Park, E. H. Joh and D. H. Kim, Soyasaponin Ab ameliorates colitis by inhibiting the binding of lipopolysacchardie (LPS) to toll-like receptor (TLR) 4 on macrophages, J. Agric. Food Chem., 2011, 59, 13165–13172 CrossRef CAS.
- J. H. Kang, M. K. Sung, T. Kawada, H. Yoo, Y. K. Kim, J. S. Kim and R. Yu, Soybean saponins suppress the release of pro-inflammatory mediators by LPS-stimulated peritoneal macrophages, Cancer Lett., 2005, 230, 219–227 CrossRef CAS.
- L. Y. Zha, L. M. Mao, X. C. Lu, H. Deng, J. F. Ye, X. W. Chu, S. X. Sun and H. J. Luo, Anti-inflammatory effect of soyasaponins through suppressing nitric oxide production in LPS-stimulated RAW264.7 cells by attenuation of NF-κB-mediated nitric oxide synthase expression, Bioorg. Med. Chem. Lett., 2011, 21, 2415–2418 CrossRef CAS PubMed.
- L. Y. Zha, J. D. Chen, S. X. Sun, L. M. Mao, X. W. Chu, H. Deng, J. W. Cai, X. F. Li, Z. Q. Liu and W. H. Cao, Soyasaponins can blunt inflammation by inhibiting reactive oxygen species (ROS)-mediated activation of PI3 K/Akt/NF-κB pathway, PLoS One, 2014, 9, e107655 CrossRef PubMed.
- Y. J. Zhang, F. P. Chen, J. D. Chen, S. Q. Huang, J. B. Chen, J. Huang, N. Li, S. X. Sun, X. W. Chu and L. Y. Zha, Soyasaponin Bb inhibits the recruitment of toll-like receptor 4 (TLR4) into lipid rafts and its signaling pathway by suppressing the nicotinamide adenine dinucleotide phosphate (NADPH) oxidase-dependent generation of reactive oxygen species, Mol. Nutr. Food Res., 2016, 60, 1532–1543 CrossRef CAS PubMed.
- C. Erridge, The roles of toll-like receptors in atherosclerosis, J. Innate Immun., 2009, 1, 340–349 CrossRef CAS PubMed.
- T. Collins and M. I. Cybulsky, NF-κB: pivotal mediator or innocent bystander in atherogenesis, J. Clin. Invest., 2001, 107(3), 255–264 CrossRef CAS PubMed.
- G. Song, J. Liu, Z. Zhao, Y. Yu, H. Tian, S. Yao, G. Li and S. Qin, Simvastatin reduces atherogenesis and promotes the expression of hepatic genes associated with reverse cholesterol transport in apoE-knockout mice fed high-fat diet, Lipids Health Dis., 2011, 10, 8 CrossRef CAS PubMed.
- W. Jin, W. Yan, Z. Li, L. Chen, L. Cao, M. Wang and Q. Fu, A decrease in the percentage of circulating mDC precursors in patients with coronary heart disease: a relation to the severity and extent of coronary artery lesions, Heart Vessels, 2013, 28, 135–142 CrossRef PubMed.
- Y. W. Hu, X. Ma, J. L. Huang, X. R. Mao, J. Y. Yang, J. Y. Zhao, S. F. Li, Y. R. Qiu, J. Yang, L. Zheng and Q. Wang, Dihydrocapsaicin attenuates plaque formation through a PPAR gamma/LXR alpha pathway in apoE(-/-) mice fed a high-fat/high-cholesterol diet, PLoS One, 2013, 8(6), e66876 CrossRef CAS PubMed.
- Y. Li, C. G. Zhang, X. H. Wang and D. H. Liu, Progression of atherosclerosis in ApoE-knockout mice fed on a high-fat diet, J. Eur. Rev. Med. Pharmacol. Sci., 2016, 20, 3863–3867 CAS.
- J. D. Smith and J. L. Breslow, The emergence of mouse models of atherosclerosis and their relevance to clinical research, J. Intern. Med., 1997, 242, 99–109 CrossRef CAS.
- J. L. Johnson, A. H. Baker, K. Oka, L. Chan, A. C. Newby, C. L. Jackson and S. J. George, Suppression of atherosclerotic plaque progression and instability by tissue inhibitor of metalloproteinase-2: involvement of macrophage migration and apoptosis, Circulation, 2006, 113, 2435–2444 CrossRef CAS.
- J. Johnson, K. Carson, H. Williams, S. Karanam, A. Newby, G. Angelini, S. George and C. Jackson, Plaque rupture after short periods of fat feeding in the apolipoprotein E-knockout mouse: model characterization and effects of pravastatin treatment, Circulation, 2005, 111, 1422 CrossRef CAS PubMed.
- R. Coleman, T. Hayek, S. Keidar and M. A. Aviram, Mouse model for human atherosclerosis: long-term histopathological study of lesion development in the aortic arch of apolipoprotein E-deficient (E0) mice, Acta Histochem., 2006, 108, 415–424 CrossRef CAS PubMed.
- Y. Nakashima, A. S. Plump, E. W. Raines, J. L. Breslow and R. Ross, ApoE-deficient mice develop lesions of all phases of atherosclerosis throughout the arterial tree, Arterioscler. Thromb., 1994, 14, 133–140 CrossRef CAS PubMed.
- M. Qin, Y. Luo, S. Lu, J. Sun, K. Yang, G. Sun and X. Sun, Ginsenoside F1 ameliorates endothelial cell inflammatory injury and prevents atherosclerosis in mice through A20-mediated suppression of NF-κB signaling, Front. Pharmacol., 2017, 8, 953 CrossRef PubMed.
- R. A. Davidson, E. Rosenberg and J. J. Brugts, Review: Statins reduce mortality and cardiovascular events in adults at risk for cardiovascular disease, Ann. Intern. Med., 2009, 151, JC4–J14 CrossRef PubMed.
- C. P. Sparrow, C. A. Burton, M. Hernandez, S. Mundt, H. Hassing, S. Patel, R. Rosa, A. Hermanowski-Vosatka, P. R. Wang, D. Zhang, L. Peterson, P. A. Detmers, Y. S. Chao and S. D. Wright, Simvastatin has anti-inflammatory and antiatherosclerotic activities independent of plasma cholesterol lowering, Arterioscler. Thromb. Vasc. Biol., 2001, 21, 115–121 CrossRef CAS PubMed.
- R. L. Reddick, S. H. Zhang and N. Maeda, Atherosclerosis in mice lacking apo E. Evaluation of lesional development and progression, J. Arterioscler. Thromb., 1994, 14, 141–147 CrossRef CAS PubMed.
- F. Borthwick, R. Mangat, S. Warnakula, M. Jacome-Sosa, D. F. Vine and S. D. Proctor, Simvastatin treatment upregulates intestinal lipid secretion pathways in a rodent model of the metabolic syndrome, Atherosclerosis, 2014, 232, 141–148 CrossRef CAS PubMed.
- F. Bea, E. Blessing, B. Bennett, M. Levitz, E. P. Wallace and M. E. Rosenfeld, Simvastatin promotes atherosclerotic plaque stability in apoE-deficient mice independently of lipid lowering, Arterioscler. Thromb. Vasc. Biol., 2002, 22, 1832 CrossRef CAS.
- S. Kamo, Y. Takada, T. Yamashita, T. Sato, E. Yano, N. Zaima and T. Moriyama, Group B soyasaponin aglycone suppresses body weight gain and fat levels in high fat-fed mice, J. Nutr. Sci. Vitaminol., 2018, 64, 222–228 CrossRef CAS PubMed.
- G. Fredman, J. Hellmann, J. D. Proto, G. Kuriakose, R. Colas, B. Dorweiler, E. Connolly, R. Solomon, D. Jones, E. Heyer, M. Spite and I. Tabas, An imbalance between specialized pro-resolving lipid mediators and pro-inflammatory leukotrienes promotes instability of atherosclerotic plaques, Nat. Commun., 2016, 23, 12859 CrossRef PubMed.
- L. Badimón, G. Vilahur and T. Padró, Lipoproteins, Platelets, and Atherothrombosis, J. Rev. Esp. Cardiol., 2009, 62, 1161–1178 CrossRef.
- B. Nordestgaard, M. Chapman, S. Humphries, H. Ginsberg, L. Masana, O. Descamps, O. Wiklund, R. Hegele, F. Raal and J. Defesche, Familial hypercholesterolaemia is underdiagnosed and undertreated in the general population: guidance for clinicians to prevent coronary heart disease: consensus statement of the European atherosclerosis society, Eur. Heart J., 2013, 34, 3478–3490 CrossRef CAS.
- American College of Cardiology/American Heart Association Task Force on Practice Guidelines, 2013 ACC/AHA guideline on the assessment of cardiovascular risk: a report of the American College of Cardiology/American Heart Association Task Force on Practice Guidelines, Circulation, 2014, 129, 49–73 CrossRef PubMed.
- W. Harris, C. Dujovne, S. Windsor, L. Gerrond, F. Newton and R. Gelfand, Inhibiting cholesterol absorption with CP-88,818 (beta-tigogenin cellobioside; tiqueside): studies in normal and hyperlipidemic subjects, Cardiovasc. Pharmacol., 1997, 30, 55–60 CrossRef CAS.
- G. Sidhu and D. G. Oakenfull, A mechanism for the hypocholesterolaemic activity of saponins, Br. J. Nutr., 1986, 55, 643–649 CrossRef CAS.
- Heart Protection Study Collaborative Group, MRC/BHF Heart Protection Study of cholesterol lowering with simvastatin in 20,536 high-risk individuals: a randomised placebo-controlled trial, Lancet, 2002, 360, 7–22 CrossRef.
- Y. P. Wang, J. X. Wu, F. L. Zhang and X. R. Wang, Effect of soyasaponin and ginsenoside (stem-leave saponin) on SOD and LPO in diabetic rats, J. Baiqiuen Med. Univ., 1993, 19, 122–123 CAS.
- J. S. Quan, X. Z. Yin, M. Tanaka and T. Kanazawa, The hypoglycemic effects of soybean hypocotyl extract in diabetic rats and their mechanism, Acta Nutr. Sin., 2004, 26, 207–210 CAS.
- H. J. Yang, D. Y. Kwon, H. J. Kim, M. J. Kim, D. Y. Jung, H. J. Kang, D. S. Kim, S. Kang, N. R. Moon, B. K. Shin and S. Park, Fermenting soybeans with Bacillus licheniformis potentiates their capacity to improve cognitive function and glucose homeostasis in diabetic rats with experimental Alzheimer's type dementia, Eur. J. Nutr., 2015, 54, 77–88 CrossRef CAS.
- D. Y. Kwon, J. W. Daily, H. J. Kim and S. Park, Antidiabetic effects of fermented soybean products on type 2 diabetes, J. Nutr. Res., 2010, 30, 1–13 CrossRef CAS.
- D. P. Ramji and T. S. Davies, Cytokines in atherosclerosis: key players in all stages of disease and promising therapeutic targets, Cytokine Growth Factor Rev., 2015, 26, 673–683 CrossRef CAS.
- S. J. Ha, J. Lee, K. M. Song, Y. H. Kim, N. H. Lee, Y. E. Kim and S. K. Jung, Ultrasonicated Lespedeza cuneata extract prevents TNF-α-induced early atherosclerosis in vitro and in vivo, Food
Funct., 2018, 9, 2090–2101 RSC.
- Y. B. Zhang, Z. Yin, X. Han, Q. Wang, Z. Zhang and J. Geng, Association of circulating high-sensitivity C-reactive protein with late recurrence after ischemic stroke, J. Neuroreport., 2017, 28, 598–603 CrossRef CAS.
- J. Lin, V. Kakkar and X. Lu, Impact of MCP-1 in atherosclerosis, J. Curr. Pharm. Des., 2014, 20, 4580–4588 CrossRef CAS.
- S. J. Lee, J. Bae, S. Kim, S. Jeong, C. Y. Choi, S. P. Choi, H. S. Kim, W. W. Jung, J. Y. Imm, S. H. Kim and T. Chun, Saponins from soy bean and mung bean inhibit the antigen specific activation of helper T cells by blocking cell cycle progression, Biotechnol. Lett., 2013, 35, 165–173 CrossRef CAS.
- K. Ahn, J. Kim, S. Oh, B. Min, J. Kinjo and H. Lee, Effects of oleanane-type triterpenoids from fabaceous plants on the expression of ICAM-1, Biol. Pham. Bull., 2002, 25, 1105–1107 CrossRef CAS PubMed.
- J. J. Fuster, TLR4 in Atherogenesis: Paying the Toll for Antimicrobial Defense, J. Am. Coll. Cardiol., 2018, 71, 1571–1573 CrossRef PubMed.
- A. Abdelsadik and A. Trad, Toll-like receptors on the fork roads between innate and adaptive immunity, J. Hum. Immunol., 2011, 72, 1188–1193 CrossRef CAS PubMed.
- M. Falck-Hansen, C. Kassiteridi and C. Monaco, Toll-like receptors in atherosclerosis, Int. J. Mol. Sci., 2013, 14, 14008–14023 CrossRef.
- P. J. Barnes and M. Karin, Nuclear factor-κB: a pivotal transcription factor in chronic inflammatory diseases, N. Engl. J. Med., 1997, 336(15), 1066–1071 CrossRef CAS PubMed.
- J. Wang, R. Zhang, Y. Xu, H. Zhou, B. Wang and S. Li, Genistein inhibits the development of atherosclerosis via inhibiting NF-κB and VCAM-1 expression in LDLR knockout mice, Can. J. Physiol. Pharmacol., 2008, 86, 777–784 CrossRef CAS.
- H. Li, Y. Jiao and M. Xie, Paeoniflorin ameliorates atherosclerosis by suppressing TLR4-Mediated NF-κB activation, Inflammation, 2017, 40, 2042–2051 CrossRef CAS.
- H. Kim, R. Yu, J. Kim, Y. Kim and M. Sung, Antiproliferative crude soy saponin extract modulates the expression of IκB-α, protein kinase C, and cyclooxygenase-2 in human colon cancer cells, Cancer Lett., 2004, 210, 1–6 CrossRef CAS.
- M. Liu, Y. Yu, H. Jiang, L. Zhang, P. P. Zhang, P. Yu, J. G. Jia, R. Z. Chen, Y. Z. Zou and J. B. Ge, Simvastatin suppresses vascular inflammation and atherosclerosis in ApoE(−/−) mice by downregulating the HMGB1-RAGE axis, Acta Pharmacol. Sin., 2013, 34, 830–836 CrossRef CAS.
Footnote |
† These authors contributed equally to this work. |
|
This journal is © The Royal Society of Chemistry 2020 |