DOI:
10.1039/C9FO02476J
(Paper)
Food Funct., 2020,
11, 270-284
Human-origin Lactobacillus salivarius AR809 protects against immunosuppression in S. aureus-induced pharyngitis via Akt-mediated NF-κB and autophagy signaling pathways
Received
23rd October 2019
, Accepted 2nd January 2020
First published on 7th January 2020
Abstract
Lactobacillus salivarius AR809 is a newly discovered probiotic strain from a healthy human pharynx and has potential ability to adhere to the pharyngeal epithelium and inhibit Staphylococcus aureus (S. aureus)-induced inflammatory response. Pharyngeal spray administration of AR809 exhibited protective effects in a S. aureus-induced mouse model of pharyngitis. The inhibitory effect and underlying molecular mechanism of AR809 on S. aureus-stimulated pharyngitis were further investigated. AR809 significantly increased phagocytosis and bactericidal activity, reduced the production of inflammatory mediators (intracellular reactive oxygen species (ROS), prostaglandin E2 (PGE2), cyclooxygenase-2 (COX-2), nitric oxide (NO), inducible NOS (iNOS)) and the expression of inflammatory cytokines (tumor necrosis factor-α (TNF-α) and interleukin-1β (IL-1β)), and induced macrophages to adopt the M2 phenotype. AR809 also attenuated S. aureus-induced phosphorylations of protein kinase B (Akt) and rapamycin (mTOR), and elevated the autophagic protein (light chain 3 from II (LC3-II) and Beclin-1) level. Furthermore, AR809 inhibited nuclear transcription factor kappa-B (NF-κB) activation by suppressing the nuclear translocation of NF-κB p65. Likewise, 740Y-P (a PI3K activator) decreased the anti-inflammatory effect of AR809 against S. aureus-induced inflammatory response, while AR809 treatments with wortmannin (a PI3K inhibitor) markedly reversed this inflammatory response. AR809 prevents S. aureus-induced pharyngeal inflammatory response, possibly by regulating TLR/PI3K/Akt/mTOR signalling pathway-related autophagy and TLR/PI3K/Akt/IκB/NF-κB pathway activity, and therefore has potential for use in preventing pharyngitis and other inflammatory diseases.
1. Introduction
Pharyngitis, commonly referred to as a sore throat, is an inflammation of the oropharynx.1 Inflammatory response is the primary manner of host defense against pharyngitis, which enables the removal of pathogens and facilitates antigen presentation via a series of complex interplays among immune cells.2,3 However, prolonged high levels of inflammatory cytokines in body circulation or in local inflammatory sites will result in the occurrence of severe progressive diseases, such as chronic asthma, oral diseases, respiratory tract infections, gastrointestinal diseases and cardiovascular disease.4–8 All of these diseases are particularly debilitating to the patient's functional capacity and quality of life.9
Macrophages are key mediators in the innate immune response against invading microorganisms and play a significant role in the modulation of an inflammatory environment in tissues.10 When infection occurs, macrophages are activated by recognizing pathogen-associated molecular patterns via a series of pattern-recognition receptors and releasing large amounts of pro-inflammatory mediators and cytokines.11,12 Therefore, controlling the inflammatory responses by modulating these inflammatory mediators and cytokines is important to prevent tissue injury.13
Staphylococcus aureus (S. aureus) recognized as a significant pathogen in pharyngitis infection can stimulate macrophages and initiate the defense response and inflammatory response.14–16 During inflammatory processes, S. aureus induces the production of pro-inflammatory cytokines (TNF-α, IL-1β, etc.) and molecular mediators (COX-2, NO, PGE2, etc.).17,18 Toll-like receptors (TLRs) are type I transmembrane receptors which are most predominantly expressed by immune cells (such as macrophages).19 TLRs as pattern-recognition receptors (PRRs) can activate innate immunity via sensing the invasion of microbial pathogens of which, Toll-like receptor 2 (TLR2) is recruited to macrophages for orchestrating innate immune response and19,20 has been identified as a pathogen recognition receptor that responds to pharyngeal pathogens such as S. aureus. Meanwhile, TLR2 plays a pivotal role in airway inflammation via regulating the PI3K/Akt signaling pathway-related autophagy.21–23
Previous studies reported that resveratrol, luteolin and polysaccharides from Citrus grandis relieved acute pharyngitis and chronic pharyngitis by anti-inflammatory responses via suppressing the TLR/IκB/NF-κB pathway and the polarization of M1 macrophages in vitro and in vivo.24,25 The flavonoids of Lonicera japonica could also inhibit the oropharyngeal inflammatory mediators TNF-α and IL-1β through the PI3K/Akt/NF-κB signaling pathway.26 The TLR/PI3K/Akt signaling pathway is an upstream activator of the NF-κB signaling cascade.27 Activation of the TLR/PI3K/Akt signaling pathway contributes to the activation of NF-κB by inducing the phosphorylation level of IKKα/β and IκBα.23 While P65, the subunit of NFκB, could translocate into the nucleus, evoking the transcription of pro-inflammatory mediators (such as TNF-α, IL-1β, etc.). These inflammatory stimuli can accelerate the development of inflammatory diseases such as pharyngitis.
Probiotics, defined as live microorganisms, confer health benefits on the host when administered in adequate amounts.28 They affect the inflammatory processes and exhibit anti-inflammatory responses as well as immunomodulatory activities in vitro and in vivo.29,30 Clinical and experimental studies reported that probiotic Lactobacillus strains could efficiently prevent and treat infections caused by S. aureus and other pathogens.31–33 Recently, we have isolated an antibacterial strain Lactobacillus salivarius (L. salivarius) AR809 (GenBank accession number MK680189) from the human oropharynx tract and demonstrated that this strain could adhere to pharyngeal epithelial FaDu cells, antagonize S. aureus, adapt to the oral environment, and modulate host innate immunity by inducing potentially protective effects.34 Particularly, AR809 grew efficiently when cultured in milk and could be applied in novel milk-based probiotic-fermented food products.34 Because of its unique functional properties, AR809 has the potential to enhance the immunity of the human oropharyngeal tract against invading pathogenic microbes for the prevention and treatment of pharyngeal infections.34 However, the relationship between probiotic bacteria AR809, pharyngeal pathogenic bacteria S. aureus, and immune responses is not completely clear yet. Mechanisms underlying the immunological protective effect of AR809 remain to be elucidated. Thus, the aim of this study was to explore the effects of AR809 on S. aureus-induced pharyngitis injury in vivo and in vitro, and confirm whether AR809 could inhibit inflammatory responses via the regulation of TLR/PI3K/Akt/mTOR signalling pathway-related autophagy and the TLR/PI3K/Akt/NF-κB pathway.
2. Materials and methods
2.1 Animal experiments
ICR male mice weighing 20–22 g were purchased from Shanghai Jiesijie Laboratory Animal Co., Ltd (SPF grade, Certificate no. SCXK20130006). All mice were housed in a humidity-controlled environment with 12 h dark/light cycle, temperature (22 ± 1 °C) and had unlimited access to water and food. All protocols of animal experiments were approved by the Institutional Animal Care and Use Committee at Jiangnan University (no. 20170915b1920115). The procedures were performed in accordance with the Guidance of the Care and Use of Laboratory Animals in China.
The S. aureus-induced pharyngitis mice were prepared by injecting 0.1 mL of S. aureus broth culture (1 × 108 CFUs mL−1) into the pharynx mucosa 2 times daily, for a period of 3 days. 18 mice were randomly assigned to 3 groups (n = 6 mice per group) as follows: (a) the control group (CG), (b) the model group (MG), and (c) the pre-treated group (PG). At 1–14 days, the mice in CG and MG were sprayed with 0.2 mL of saline twice daily, whereas the mice in PG were sprayed with 0.2 mL of AR809 suspension (1 × 108 CFUs mL−1) into the pharynx mucosa twice daily. At 15–17 days, the mice in MG and the mice pre-treated with AR809 in PG were injected with S. aureus, and the mice in CG were injected with an equal volume of saline. At 18–31 days, the mice in CG and MG were sprayed with saline and the mice in PG were sprayed with AR809 suspension into the pharynx mucosa again. All mice were fasted overnight before being killed. The clinical signs of pharyngitis were assessed: (a) body weight, (b) cough, (c) redness of the throat, (d) athletic ability, and (e) fur color.26,35,36 The statistical scores of each group were determined by the severity of clinical manifestations, and were graded into asymptomatic (score 0), mild (score 1), moderate (score 2) and severe (score 3). The total scores of each group were compared. Pharynx mucosal tissues and sera were collected for further analysis.
2.2 Bacterial strains and cell culture
The L. salivarius AR809 strain (CGMCC 17206) isolated from the oropharynx tract of healthy individuals was initially cultured on the de Man, Rogosa and Sharpe (MRS) medium (BD, Heidelberg, Germany) in an anaerobic incubator (85% N2, 10% H2, and 5% CO2) (Thermo Fisher, Waltham, USA) at 37 °C for 24 h. The S. aureus strain (GenBank accession number MK811090.1) isolated from the oropharynx tract of patients with pharyngitis was plated onto the brain heart infusion (BHI) medium (BD, Heidelberg, Germany) and incubated overnight at 37 °C under aerobic conditions.
For cell assays, RAW264.7 cells were cultured with Dulbecco's Modified Eagle (HyClone, Utah, USA) medium (DMEM) containing 10% (vol/vol) fetal bovine serum (FBS) (HyClone, Utah, USA) and 100 U mL−1 penicillin/streptomycin (Sigma-Aldrich, St Louis, USA) at 37 °C under 5% CO2. The medium was renewed every two days. Cells were sub-cultured once per week with a split ratio of 1
:
3 using 0.25% trypsin–EDTA solution (Sigma-Aldrich, St Louis, USA). The experiment was divided into three groups: the control group (CG): the RAW264.7 cells were cultured in fresh medium without treatment, the model group (MG): the RAW264.7 cells treated with S. aureus acted as a pharyngitis model, and the pre-treatment group (PG): the RAW264.7 cells were treated with AR809. After incubation, the cells were washed with sterile phosphate-buffered saline and then exposed to S. aureus.
2.3 Cell viability assay
A cell viability assay was performed using a previous method with little modifications.23 Based on our recent study, the suitable dose of AR809 was selected as a MOI of 2.34 RAW264.7 cells were seeded at 1 × 104 cells per well in 96-well plates (Corning Costar, Massachusetts, USA) and then pre-treated with AR809 at a multiplicity of infection (MOI, bacteria: macrophages) of 2
:
1 for 12 or 24 hours and then exposed to S. aureus (MOI 2) for 2 h. Ten microliters of cell counting kit-8 solution (Beyotime, Shanghai, China) was added for 1 h. Cell viability was determined by measuring the absorbance at 450 nm using a multi-function microplate reader (Molecular Devices, Silicon Valley, USA). Cell viability was calculated using the following equation: Cell viability (%) = (Asample/Acontrol) × 100, where Asample is the absorbance of RAW264.7 cells co-incubated with AR809 and Acontrol is the absorbance of RAW264.7 cells alone.37 Each experiment was performed in triplicate.
2.4 LDH release assay
The activity of extracellular lactate dehydrogenase (LDH) was investigated using a Cytotoxicity LDH Assay Kit (Beyotime, Shanghai, China) according to the manufacturer's protocol. Briefly, the cells (1 × 104 cells per well) were seeded in 96-well plates and then subjected to the indicated treatments. For analysis, the supernatant was extracted from each well, and catalyst solutions were added to each well and incubated for 30 min at 25 °C. The absorbance in each well was measured at 490 nm using a multi-function microplate reader.
2.5 Macrophage phagocytosis assay
The phagocytic ability of RAW264.7 cells was investigated using a previous method.31 Macrophages (1 × 105 cells per well) in DMEM were placed in 12-well tissue culture plates (Corning Costar, Massachusetts, USA) and incubated for 24 h at 5% CO2. After counting, macrophages in separate wells were pre-treated with AR809 at a MOI of 2
:
1 for 24 h at 37 °C and subsequently challenged with FITC-labeled S. aureus at a MOI of 5
:
1 for 1 h at 37 °C. At the end of the phagocytosis, culture plates were removed from the incubator and kept on ice for 20–30 s to stop the phagocytosis. The cells were washed with Dulbecco's phosphate-buffered saline (DPBS) and then fixed with 4% paraformaldehyde (pH 7.4). Subsequently, the nuclei of the cells were stained with 4′6-diamidino-2-phenylindole (DAPI, 1 mg mL−1). Glass coverslips were air-dried and mounted onto glass microscope slides. The phagocytic bacteria were visualized using a fluorescence microscope (Leica, München, Germany). Measurements were conducted using a Becton Dickinson FACS caliber flow cytometer (BD Bioscience, California, USA).
2.6 Intracellular bacterial killing assay
An intracellular bacterial killing assay was performed using a gentamicin protection assay.38 RAW264.7 cells (1 × 105 cells per well) were cultured in a 12-well plate (Corning Costar, Massachusetts, USA), and then pre-treated with AR809 at a MOI of 2
:
1 for 24 h at 37 °C. The cells were challenged with S. aureus (MOI = 2
:
1) for 1 h and 2 h, respectively. Subsequently, the well was washed and treated with media containing 200 μg ml−1 gentamicin for 30 min at 37 °C to kill any extracellular S. aureus. After being washed with DPBS three times, the cells were incubated for another 1 h and then lysed with sterile water containing 1% Triton-X-100. Dilutions of the resulting lysates were plated on BHI agar plates and incubated overnight at 37 °C. The efficiency of the intracellular bacterial killing assay was calculated using the following equation: Intracellular bacterial killing = [colony forming units (CFU) (1 h) − CFU (2 h)]/CFU (1 h) × 100%.
2.7 Measurement of ROS generation
The concentration of intracellular reactive oxygen species (ROS) in RAW264.7 cells was assayed using a Reactive Oxygen Species Assay Kit (Beyotime, Shanghai, China). The cells were seeded in 96-well plates at a density of 1 × 104 cells per well and pre-treated with or without AR809 (MOI 2) for 24 h before being exposed to S. aureus (MOI 2) for 2 h. Following incubation, the cells were treated with 10 μM 2′,7′-dichlorodihydrofluorescein diacetate and further incubated for 30 min. The cells were harvested using trypsin–EDTA into 3 mL tubes and washed using DPBS. Signals were monitored by using a multi-function microplate reader at excitation and emission wavelengths of 488 and 525 nm, respectively.
2.8 NO measurement
The production of nitric oxide (NO) in the culture medium was measured using the Griess reaction.39 The cells (1 × 104 cells per well) were seeded in 96-well plates and then subjected to the indicated treatments. 50 μL of the cell culture medium was mixed with 50 μL of Griess reagent I and 50 μL of Griess reagent II (Beyotime, Shanghai, China). After incubation at 25 °C for 5 min, the absorbance of the cell culture medium at 540 nm was measured using a multi-function microplate reader. The amount of nitrite in the samples was measured from the sodium nitrite serial dilution standard curve.
2.9 Flow cytometry analysis
Flow cytometry analysis was performed as described previously.40 The cells were plated as 1 × 105 cells per well in 12-well culture plates. After being cultured overnight, the cells were counted and pre-incubated with and without the indicated concentrations of AR809 (MOI 2) for 24 h, and then incubated with S. aureus (MOI 2) for 2 h. To evaluate the CD16/32+ and CD206+ protein expression, the cultured cells after treatments were harvested and incubated with a PE-conjugated rat anti-mouse CD16/CD32+ monoclonal antibody (mAb) (BD Bioscience, California, USA) or APC-conjugated rat anti-mouse CD206+ mAb (Thermo Fisher, Massachusetts, USA) on ice for 30 min. The cells were finally washed, fixed with 1% paraformaldehyde, and the fluorescence intensity was analyzed using a Becton Dickinson FACS caliber flow cytometer (BD Bioscience, California, USA) and FlowJo software V7.6.1.
2.10 Western blot analysis
The cells were seeded in the wells of a 6-well plate at a density of 1 × 106 cells per well. After being cultured overnight, the cells were counted and pre-treated with or without AR809 (MOI 2) for 24 h before being exposed to S. aureus (MOI 2) for 2 h. The cells were collected and lysed in lysis buffer (Beyotime Biotechnology, Shanghai, China) and the protein content of the cell lysates was then determined using the Bradford reagent. The proteins in each sample were determined by sodium dodecyl sulfate polyacrylamide gel electrophoresis, transferred to a polyvinylidene difluoride membrane, and exposed to an appropriate antibody (the dilution rates are as follows: anti-TLR2, anti-PI3K, anti-Akt, anti-phospho-Akt (ser473), anti-mTOR, anti-phospho-mTOR, anti-IKB α, anti-phospho-IKB α, anti-NF-κB p65, anti-phospho-NF-κB p65, anti-COX-2, anti-Beclin-1, and anti-β-actin, 1
:
1000) (Beyotime, Shanghai, China). The proteins were visualized by using an enhanced chemiluminescence detection system (Amersham Biosciences, Piscataway, NJ) with a horseradish peroxidase-conjugated anti-rabbit or anti-mouse secondary antibody. Images were acquired using a ChemiDoc MP Imaging System (Bio-Rad, Hercules, CA).
2.11 RT-qPCR
The cells were seeded in the wells of a 12-well plate at a density of 1 × 105 cells per well. After being cultured overnight, the cells were counted and pre-treated with or without AR809 (MOI 2) for 24 h before being exposed to S. aureus (MOI 2) for 2 h. After incubation, total RNA was isolated from RAW264.7 cells according to the manufacturer's instructions using Trizol reagent (Invitrogen, USA) and its reverse transcription was performed according to our previous reports.34 PCR primer sequences of the genes are shown in Table 1. Real-time PCR was performed on a LightCycler® Nano thermal cycler (Roche Applied Science, Penzberg, Germany) using a SYBR Green FastStart kit (Roche, Basel, Switzerland). PCR cycles are as follows: 1 cycle at 50 °C for 2 min, 1 cycle at 95 °C for 10 min, followed by 40 cycles at 95 °C for 15 s and at 60 °C for 1 min. Relative expression was calculated using the 2−ΔΔCt method.
Table 1 Primer sequences used for RT-qPCR
Gene |
Forward primer |
Reverse primer |
TNF-α
|
ATGAGCACAGAAAGCATGATC |
TACAGGCTTGTCACTCGAATT |
IL-1β
|
TGCAGAGTTCCCCAACTGGTACATC |
GTGCTGCCTAATGTCCCCTTGAATC |
IL-4
|
GCCATATCCACGGATGCGAC |
TCCATCTCCGTGCATGGCGTC |
IL-10
|
TGGCCTTGTAGACACCTTGGTCTT |
CAGAGAAGCATGGCCCAGAAATCA |
Agr1
|
CCTGAAGGAACTGAAAGGAAAG |
TTGGCAGATATGCAGGGAGT |
Mrc1
|
GCAAATGGAGCCGTCTGTGC |
CTCGTGGATCTCCGTGACAC |
iNOS
|
AATGGCAACATCAGGTCGGCCATCACT |
GCTGTGTGTCACAGAAGTCTCGAACTC |
PGE2
|
GCCATTATGACCATCACCTTCG |
GCCATTATGACCATCACCTTCG |
TLR2
|
TTTGCTCCTGCGAACTCC |
GCCACGCCCACATCATTC |
PI3K
|
ATGCCAGAAAGGAGAATG |
TGTTGGACTCAGCAATAC |
Akt
|
AGTCCCCACTCAACAACTTCT |
GAAGGTGCGCTCAATGACTG |
mTOR
|
ACACCCTCCATCCACCTCAT |
TAGCGGATATCAGGGTCAGGA |
NF-κB p65
|
GTATTGCTGTGCCTACCCGAAAC |
GTTTGAGATCTGCCCTGATGGTAA |
LC3-II
|
AAACGCATTTGCCATCACA |
GGACCTTCAGCAGTTTACAGTCAG |
Beclin-1
|
TGCTCCTTAGGGGATGTTTG |
CATGGGGCTGAGTCAAAACT |
Atg5
|
CTTGCATCAAGTTCAGCTCTTCC |
AAGTGAGCCTCAACCGCATCCT |
Atg7
|
CCTGTGAGCTTGGATCAAAGGC |
GAGCAAGGAGACCAGAACAGTG |
Atg12
|
GAAGGCTGTAGGAGACACTCCT |
GGAAGGGGCAAAGGACTGATTC |
β-Actin
|
GTGCTATGTTGCTCTAGACTTCG |
ATGCCACAGGATTCCATACC |
2.12 Statistical analysis
Statistical package for social sciences (SPSS) software version 22.0 (SPSS Inc., Chicago, IL, USA) was used to perform statistical analysis. The significance of differences among data was assessed using the ANOVA program with a level of significance at p < 0.05.
3. Results
3.1 AR809 attenuates S. aureus-induced pharyngitis in mice
To verify the potential effect of AR809 on S. aureus-induced pharyngitis in vivo, mice were administered with AR809 for 31 days. Compared with the mice in CG, the body weights of mice in MG were significantly decreased at the end of the experiment on day 31, whereas AR809 contributed to a significant change in weight gain (p < 0.05). The symptoms of pharyngitis were evaluated by the pharyngitis clinical sign score. As shown in Fig. 1, CG showed no signs of pharyngitis (score 0). At the end of the experiment on day 31, the average score of pharyngitis clinical signs for the MG reached 8.7 ± 0.37, while the oral administration of AR809 remarkably decreased the pharyngitis clinical sign score to 3.5 ± 0.24 (p < 0.05, vs.MG).
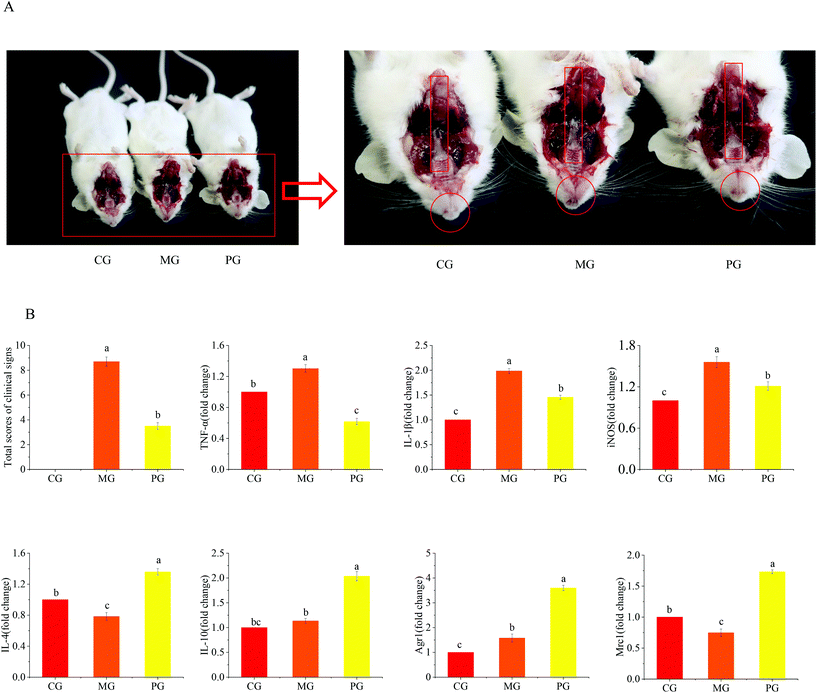 |
| Fig. 1 The in vivo anti-inflammatory effects of AR809 on the S. aureus-induced animal model of pharyngitis. (A) Clinical pathological features. (B) Statistical scores of clinical manifestations and the mRNA expressions of Agr1, Mrc1, IL-4, IL-10, TNF-α, IL-1β, and iNOS. | |
S. aureus-induced pharyngitis is associated with the high levels of pro-inflammatory cytokines. Thus, the pro-inflammatory M1 phenotype and anti-inflammatory M2 phenotype were measured in the sera of mice using RT-qPCR. Consistent with our previous data, AR809 obviously reduced the pharyngitis inflammation reaction induced by S. aureus by suppressing the gene expressions of pro-inflammatory (M1) markers (TNF-α, IL-1β, and iNOS) while upregulating the expressions of anti-inflammatory (M2) markers (Agr1, Mrc1, IL-4, and IL-10).
3.2 AR809 protects the cell viability of RAW264.7 cells with S. aureus treatment
To evaluate the effect of AR809 on cell viability, the cells were pre-treated with AR809 for 12 h or 24 h, and then exposed to S. aureus for 2 h. Interestingly, we observed that AR809 significantly increased the cell viability after incubation for 24 h in S. aureus-induced RAW264.7 cells (Fig. 2A, p < 0.05). In order to observe the toxicity of AR809, the LDH assay was performed. AR809 decreased LDH release in S. aureus-induced RAW264.7 cells, resulting in a notable reduction (17.16%, p < 0.05) (Fig. 2B).
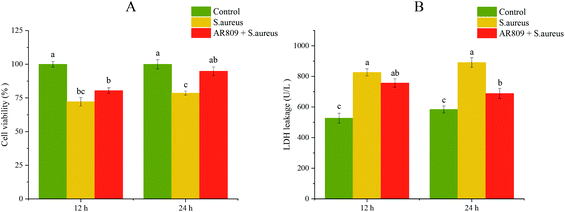 |
| Fig. 2 Effect of AR809 on cell viability and LDH release in RAW264.7 cells. Cells are treated with the indicated concentrations of AR809 for 12 and 24 h, and then exposed to S. aureus for 2 h. Cell viability (A) and LDH release (B) are determined in RAW264.7 cells. Values are expressed as mean ± SD of three independent determinations. Mean values with different letters differ significantly (p < 0.05). | |
3.3 Effects of AR809 on phagocytosis in S. aureus-induced RAW264.7 cells
The phagocytosis of bacteria is an effective mechanism employed by the innate immune response. We therefore pre-incubated macrophages with probiotic AR809 to determine their ability to increase phagocytosis in response to challenge with S. aureus (Fig. 3(A and B), p < 0.05). In the cells challenged with FITC-labeled phagocytic stimuli, the rate of ingestion of phagocytized S. aureus cells was markedly higher than that in CG (p < 0.05). In addition, pre-treatment with AR809 resulted in a significantly higher efficiency of macrophage phagocytosis (46.0%) compared with that in MG (32.0%) (p < 0.05). Lactobacillus AR809 strongly activated the phagocytic functions of the S. aureus-induced macrophages.
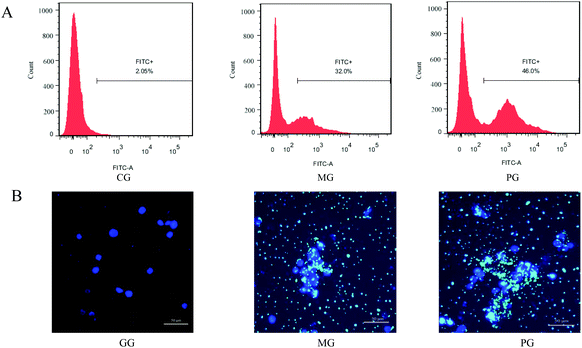 |
| Fig. 3 Effect of AR809 on phagocytosis in RAW264.7 macrophages. (A) Flow cytometry analysis and (B) DAPI fluorescence microscopy images. Nuclei are shown in blue, and green colour represents “FITC-labelled S. aureus”. | |
3.4 Effect of AR809 on the expression of CD16/32+ and CD206+ in S. aureus-stimulated RAW264.7 cells
The effect of AR809 on the expression of CD16/32+ (M1 phenotypic marker) and CD206+ (M2 phenotypic marker) was examined by flow cytometry. Fig. 4A shows that the application of S. aureus resulted in a significant increase in the expression of CD16/32+. The pre-treatment of AR809 led to an increase in the expression of CD206+. These data suggested that AR809 could make macrophages adopt the M2 phenotype.
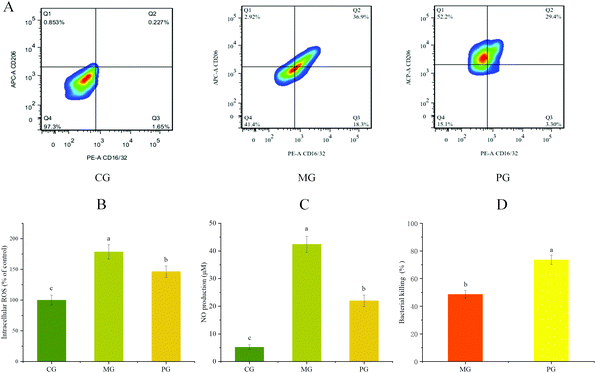 |
| Fig. 4 Effects of AR809 on the M1/M2 phenotype, ROS/NO production and bactericidal activity in RAW264.7 cells. (A) AR809 promoted the polarization of the M1 phenotype to the M2 phenotype in RAW264.7 cells. (B and C) AR809 reduced ROS and NO production in S. aureus-induced RAW264.7 cells. (D) Effect of AR809 on the bacterial activity of macrophages against the pathogen S. aureus. | |
3.5
S. aureus-induced ROS/NO production and bactericidal activity
We examined the effects of AR809 on S. aureus-induced ROS and NO production in RAW264.7 cells. A marked increase was observed in the S. aureus-stimulated group, while 24 h pre-incubation with AR809 significantly inhibited the S. aureus-induced NO and intracellular ROS (Fig. 4(B and C)). To confirm S. aureus survival during infection along with AR809 pre-treatment, we assessed the macrophage-mediated intracellular killing activity. As shown in Fig. 4D, the pre-incubation of RAW264.7 cells for 24 h with AR809 (MOI 2) induced a decrease in the number of viable intracellular S. aureus. These data confirmed that AR809, but not ROS and NO production, enhanced macrophage-mediated intracellular killing and restricted the survival of the pathogen S. aureus.
3.6 Effects of AR809 on the expression of inflammatory mediators and inflammatory cytokines in S. aureus-induced RAW264.7 cells
We evaluated the effects of pre-treatment with AR809 on the production of inflammatory mediators (COX-2, iNOS and PGE2) and the expression of inflammatory cytokines (TNF-α, IL-1β, IL-4 and IL-10) in S. aureus-induced RAW264.7 cells. As shown in Fig. 5 and 7(A, B), the mRNA or protein expression levels of COX-2, iNOS, PGE2, TNF-α and IL-1β dramatically (p < 0.05) increased following stimulation of macrophages with S. aureus. However, the expression levels of pro-inflammatory mediators/pro-inflammatory cytokines were significantly (p < 0.05) downregulated and those of anti-inflammatory cytokines (IL-4 and IL-10) were upregulated by the macrophages pre-treated with AR809 for 24 h.
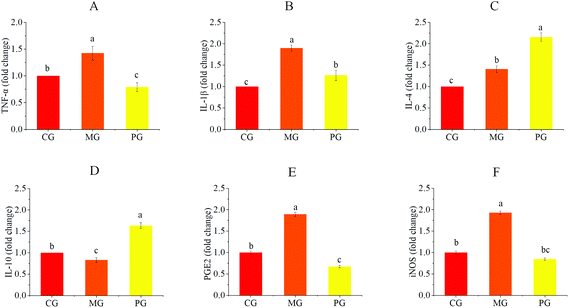 |
| Fig. 5 Effects of AR809 on the expression of inflammatory mediators and inflammatory cytokines in S. aureus-induced RAW264.7 cells. The mRNA expressions of TNF-α (A), IL-1β (B), IL-4 (C), IL-10 (D), PGE2 (E) and iNOS (F) are assayed by RT-qPCR. Values are expressed as mean ± SD of three independent determinations. Mean values with different letters differ significantly (p < 0.05). | |
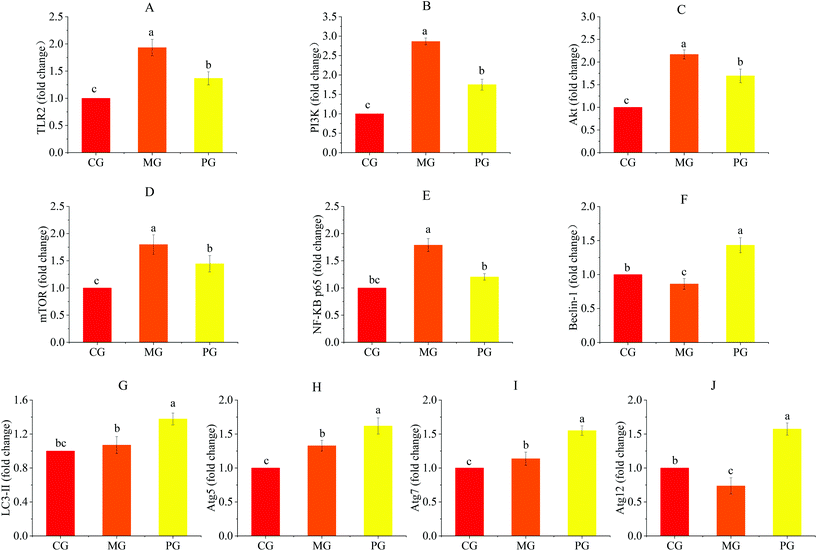 |
| Fig. 6 AR809 inhibited S. aureus-induced TLR2/PI3K/Akt/mTOR and NF-κB pathway activation in RAW264.7 cells. The mRNA expressions of TLR2 (A), PI3K (B), Akt (C), mTOR (D), NF-κB p65 (E), Beclin-1 (F), LC3-II (G), Atg5 (H), Atg7 (I), and Atg12 (J) are assayed by RT-qPCR. Values are expressed as mean ± SD of three independent determinations. Mean values with different letters differ significantly (p < 0.05). | |
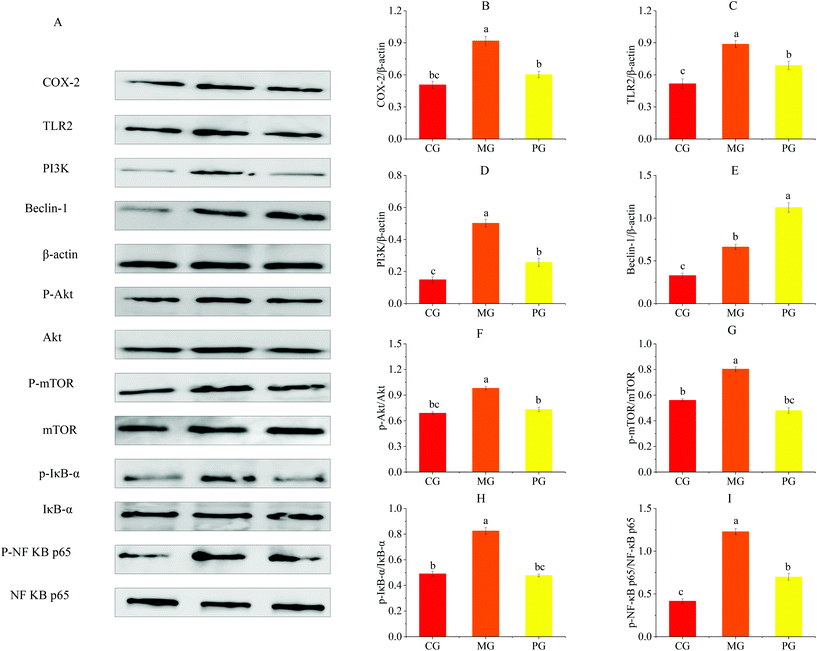 |
| Fig. 7 AR809 inhibited S. aureus-induced TLR2/PI3K/Akt/mTOR and NF-κB pathway activation in RAW264.7 cells. (A) Western blot analysis of COX-2, TLR2, PI3K, Beclin-1, p-Akt, p-mTOR, p-IκB and p-NF-κB p65 expression in RAW264.7 cells. The right bar graphs show the statistical results for the relative quantitative expression of COX-2 (B), TLR2 (C), PI3K (D), Beclin-1 (E), p-Akt (F), p-mTOR (G), p-IκB (H) and p-NF-κB p65 (I). Values are expressed as mean ± SD of three independent determinations. Mean values with different letters differ significantly (p < 0.05). | |
3.7 Effects of AR809 on S. aureus-induced activation of the TLR2/PI3K/Akt/mTOR pathway
To determine the mechanism by which AR809 induced the inhibitory effect on S. aureus-induced inflammatory response, TLR/PI3K/Akt/mTOR signaling pathways were investigated by western blot and RT-qPCR. As shown in Fig. 6(A–D) and 7(C, D, F and G), the phosphorylation levels of Akt and mTOR in macrophages were increased by about 0.42- and 0.44-fold by S. aureus treatment, respectively, compared with the control groups. However, this effect was attenuated by AR809 treatment and the ratio of TLR2/β-actin, PI3K/β-actin, p-Akt/Akt and p-mTOR/mTOR was decreased by 22.52%, 48.67%, 25.33% and 40.28%, respectively. This result suggested that AR809 inhibited S. aureus-induced TLR2/PI3K/Akt/mTOR activation in RAW264.7 macrophages.
3.8 Effects of AR809 on S. aureus-induced activation of the NF-κB pathway
To determine the effect of AR809 on NF-κB activity, we investigated the related proteins and genes of this signaling pathway using western blot and RT-qPCR. As shown in Fig. 6(E) and 7(H, I), significant (p < 0.05) degradation of IκB-α and decreased expression levels of p-IκB-α and p-NF-κB p65 were observed in the cells after S. aureus exposure for 2 h, which indicated increased NF-κB activity. However, AR809 significantly inhibited the S. aureus-induced phosphorylation of NF-κB p65, as well as phosphorylation and degradation of IκB-α. These data showed that the NF-κB activity in S. aureus-induced RAW264.7 cells was significantly (p < 0.05) inhibited by AR809.
3.9 Inhibition of TLR2/PI3K/Akt/mTOR signaling pathway-activated cell autophagy
Inhibition of PI3K/Akt/mTOR is known to induce autophagy activation.23 As shown in Fig. 6(F–J) and 7(E), with the inhibition of the PI3K/Akt/mTOR signaling pathway, the autophagy marker protein level (Beclin-1) in PG was higher than that in CG and MG (p < 0.05) (Fig. 7E). The mRNA expressions of autophagy-related factors (LC3 II, Beclin-1, Atg5, Atg7 and Atg12) in PG were significantly increased compared with those in CG and MG (p < 0.05) (Fig. 6F–J).
3.10 Effects of AR809, wortmannin and 740Y-P on the TLR/PI3K/Akt/mTOR signalling pathway-related autophagy and TLR/PI3K/Akt/IκB/NF-κB pathway
To further validate the role of the NF-κB and mTOR signalling pathway in the regulation of inflammation by AR809, the PI3K inhibitor (wortmannin, 50 μM) and PI3K activator (740Y-P, 50 μg mL−1) were added to macrophages treated with AR809. As shown in Fig. 8(A and B), the 740Y-P + S. aureus group induced a significant upregulation of TLR2, PI3K, p-IκBα/IκBα, p-NF-κB p65/NF-κB p65, p-mTOR/mTOR and p-Akt/Akt expressions both in protein and mRNA levels. The mRNA levels of Beclin-1 and LC3 II were significantly lower in the 740Y-P + S. aureus group than that in the control group. However, this effect could be reversed by AR809. The wortmannin + S. aureus group and the AR809 + wortmannin + S. aureus group also confirmed that the PI3K/Akt signaling pathway was involved in the protective effect of AR809.
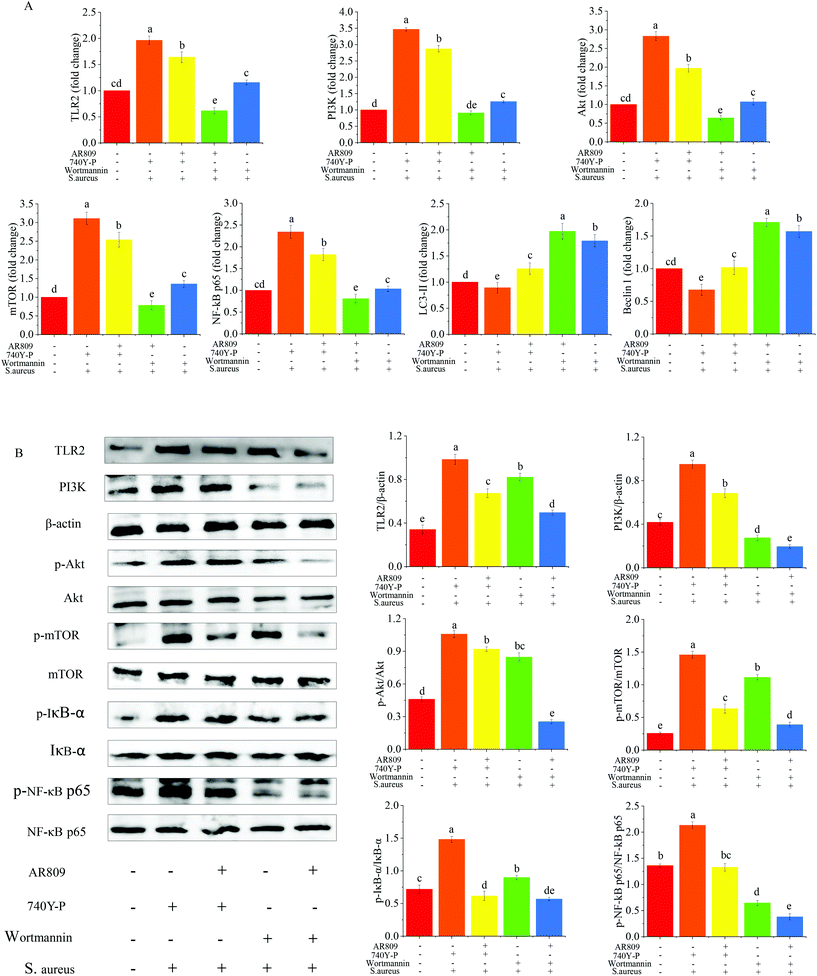 |
| Fig. 8 The role of the TLR/PI3K/Akt/mTOR signalling pathway-related autophagy and TLR/PI3K/Akt/IκB/NF-κB pathways in the regulation of inflammation by the AR809/PI3K inhibitor (wortmannin)/PI3K activator (740Y-P) is further validated. Mean values with different letters differ significantly (p < 0.05). (A) The mRNA expression of the pathway-related proteins is assayed by RT-qPCR and (B) western blotting assay reveals the expression of pathway-related proteins in RAW264.7 cells from each group. β-Actin is used as an endogenous reference. Values are expressed as mean ± SD of three independent determinations. Mean values with different letters differ significantly (p < 0.05). | |
4. Discussion
Inflammation is a host response to foreign pathogens or tissue injury by the organism to eliminate harmful stimuli, such as infection and noxious stimuli, as well as to initiate the healing and repair process of the damaged tissue.41 Chronic inflammation causes the increase in pro-inflammatory mediators and various cytokines which play key roles in the pathogenesis of various chronic inflammatory diseases. Thus, blocking the effects of pro-inflammatory mediators offers an attractive precautionary or therapeutic strategy. In this study, we demonstrated the immunological protective effects of probiotic AR809 in a S. aureus-induced pharyngitis model. AR809 significantly inhibited the expression levels of inflammatory mediators and pro-inflammatory cytokines. The mechanism might involve the innate immune response via modulating the TLR/PI3K/Akt/mTOR signaling pathway-related autophagy and TLR/PI3K/Akt/NF-κB pathways by AR809 in S. aureus-induced RAW264.7 macrophages (Fig. 9).
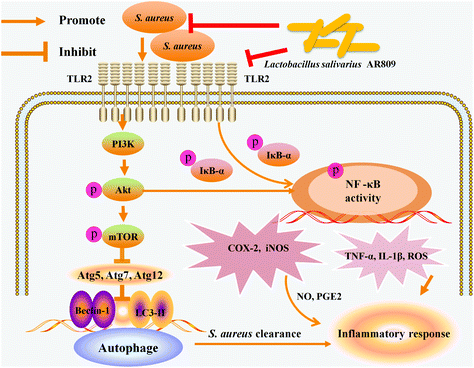 |
| Fig. 9 Schematic representation of the molecular mechanism underlying the immunological protective effect of AR809. The PI3K/Akt signaling pathway is involved in AR809-induced autophagy and immune responses. | |
The potential probiotic bacterium of the human pharynx should have promising antagonistic and immunomodulatory properties. Previous studies demonstrated that Lactobacillus helveticus MIMLh5 had the potential abilities of controlling Streptococcus pyogenes infections at the pharyngeal level. MIMLh5 efficiently reduced the induction of interleukin-6, interleukin-8, and TNF-α.42 Compared with MIMLh5, the probiotic L. salivarius AR809 had a high ability to inhibit the growth of S. aureus, prevent S. aureus adhesion on the pharyngeal epithelium and modulate host innate immunity.34 Basically, S. aureus is a significant pathogen that is associated with aggressive respiratory diseases. S. aureus produces immunosuppressive factors, inhibits phagocytosis activity and the production of cytokines, and promotes the apoptosis of macrophages. Thus, S. aureus as an infection source is important for evaluating the effectiveness of RAW264.7 cells activated by AR809.
A primary function of macrophages is to phagocytize and degrade invading pathogens.19 However, weakened phagocytosis might occur due to the lack of a key phagocytic component to increase the susceptibility to bacterial infection. An important step for the immunomodulation by probiotic lactobacilli is to see the ability to enhance phagocytic activity by the RAW264.7 cells. Our results showed that the phagocytosis of the activated RAW264.7 macrophages was significantly stimulated and demonstrated the ability of AR809 to activate macrophages and kill microbes, and to improve a host response against infection with S. aureus.
Upon being challenged by inflammatory stimuli, several types of cells within the immune system, including macrophages, lymphocytes, and polymorph nuclear leukocytes, are induced to synthesize and secrete inflammatory mediators and pro-inflammatory cytokines/chemokines which play important roles in mediating inflammatory responses.43 INOS is the key enzyme for NO synthesis and the induction of iNOS leads to the generation of NO.44 The overexpression of iNOS likely results in the production of excessive NO, which generates reactive nitrogen species and causes cell inflammatory damage, augmenting the pathological activities of NO.45,46 COX-2 plays a crucial role in the development of various inflammatory responses.47 Previous studies have also revealed the elevated expression of COX-2 in various inflammatory diseases and human cancers, including acute pharyngitis and endometrial cancer.48,49 PGE2 is derived from arachidonic acid (AA) as a result of the sequential enzymatic reactions of COX-2 and PGE synthase.48 Overproduction of NO and PGE2 is associated with the upregulation of iNOS and COX-2.50 We demonstrated that AR809 inhibited the close crosstalk between NO/iNOS and PGE2/COX-2, decreased the synergic effects of this crosstalk in S. aureus-induced RAW264.7 cells and promoted macrophage polarization toward the M2 phenotype.
In addition, we manifested that AR809 also potently suppressed S. aureus-induced inflammation via modulating the TLR/PI3K/Akt/mTOR signaling pathway-related autophagy and NF-κB pathways. Toll-like receptors (TLRs), a major connection between innate and adaptive mucosal immune responses, act as transmembrane receptors in the cellular response to insults.51 It has been known that TLR2, which serves as the primary mediator of S. aureus cell signaling, is associated with the activation of NF-κB and PI3K/Akt/mTOR.21 The transcription factor NF-κB plays a key role in the regulation of many genes responsible for the generation of inflammatory mediators. NF-κB activation involves the rapid phosphorylation of IκBs in response to pro-inflammatory stimuli.52 Meanwhile, the NF-κB pathway is also activated via the PI3K/Akt/mTOR signal, leading to the production of inflammatory mediators and secretion of pro-inflammatory cytokines.53 mTOR and NF-κB are the major downstream effectors of Akt, and the mTOR or NF-κB signaling pathway through Akt can potentially proceed in two mutually exclusive and independent directions:43 (1) mTOR regulates Akt via a feedback mechanism, and this can help in the regulation of NF-κB by mTOR through the regulation of Akt via the mTOR-associated protein receptor, and (2) a more direct regulation of NF-κB by mTOR involves IKK. In the activation of NF-κB, IKK plays an important role by phosphorylating the inhibitory IκBα leading to its dissociation from NF-κB, resulting in an activated NF-κB that translocates to the nucleus. The mTOR-mediated induction of IKK leads to the activation of NF-κB.43 In this process, AR809 inhibited the translocation of NF-κB from the cytoplasm to the nucleus via the TLR2/PI3K/Akt signaling pathway, and decreased the mRNA expressions of iNOS, TNF-α, IL-1β, and PGE2 and protein production of COX-2.
Autophagy refers to a dynamic process by which cytoplasmic organelles and proteins are sequestered into autophagosomes that subsequently fuse with lysosomes, leading to the degradation of cargo by lysosomal hydrolases.54 It has important regulatory effects on inflammation, and affects the pathological progression of inflammatory diseases.55 Autophagy can be inhibited by activating mTOR, while an inactive mTOR pathway can induce autophagy.55LC3-II, Beclin-1, Atg5, Atg7 and Atg12 are key regulators of autophagy, and their abnormally low expression levels may lead to lower autophagic activity. It was also found that the Akt/mTOR signaling pathway was upregulated, and cell autophagy was suppressed. What set forth above has consistency with our results.55 It can be concluded that AR809 could enhance autophagy by suppressing the PI3K/Akt/mTOR signaling pathway, and kill pathogens to protect cells from pathogens.
The in vivo anti-inflammatory effects of AR809 were also evaluated using a S. aureus-induced animal model of pharyngitis. Pharyngeal spray administration of AR809 significantly ameliorated pharyngitis induced by S. aureus, which included preventing a decrease in body weight and the symptoms of pharyngitis. AR809 could inhibit S. aureus-induced pharyngitis by preventing the overproduction of pro-inflammatory factors (TNF-α, IL-1β, and iNOS), and shifting the macrophage phenotype from pro-inflammatory M1 to anti-inflammatory M2 both in vivo and in vitro. These data suggest that AR809 has therapeutic potential for treating pharyngitis.
5. Conclusions
The current study demonstrated the protective effect of oral probiotic AR809 on S. aureus-induced pharyngitis inflammation injury in vivo and in vitro. AR809 inhibited S. aureus-induced iNOS and COX-2 expressions, as well as PGE2 and ROS productions, in the macrophages. Similarly, it also inhibited the expression of pro-inflammatory cytokines (TNF-α and IL-1β) and promoted macrophage M2 polarization. These effects corresponded to the inhibition of NF-κB phosphorylation and activation of an autophagy pathway through the downregulation of PI3K/Akt signaling. Understanding these mechanisms will provide new insights into the immune applications of AR809. Further investigations on the effects of the PI3K/Akt/mTOR signaling pathway in pharyngitis disease are needed to fully comprehend the regulating mechanism and pharyngitis disease progression diagnosis, which is very important for the development of new therapeutic approaches.
Conflicts of interest
There are no conflicts to declare.
Acknowledgements
This work was supported by the National Key Research and Development Program of China (No. 2018YFD0501600), the National Natural Science Foundation of China (No. 31771956), the Shanghai Agriculture Applied Technology Development Program, China (No. 201902080007F01152), and the Shanghai Engineering Research Center of Food Microbiology (No. 19DZ2281100). This study was also approved by the Ethics Committee of Tongji Hospital (KYSB-2016-97). We are grateful to the Department of Otolaryngology, Tongji Hospital of Tongji University, for technical support.
References
- J. Uemura, R. Nagpal, N. Zerbinati, B. Singh, M. Marcellino, D. Mohania, F. Marotta, F. He, A. Ayala and Y. Kasugai, Effect of VBC-1814/7J, a poly-phytocompound, on a non-infectious model of pharyngitis, Exp. Ther. Med., 2017, 13, 3075–3080 CrossRef CAS PubMed.
- Q. Xu, M. Liu, Q. Liu, W. Wang, Y. Du, H. Yin, Q. Xu, M. Liu, Q. Liu and W. Wang, The inhibition of LPS-induced inflammation in RAW264.7 macrophages via the PI3K/Akt pathway by highly N-acetylated chitooligosaccharide, Carbohydr. Polym., 2017, 174, 1138–1143 CrossRef CAS PubMed.
- M. Harding and P. Kubes, Innate immunity in the vasculature: interactions with pathogenic bacteria, Curr. Opin. Microbiol., 2012, 15, 85–91 CrossRef CAS PubMed.
- K. Atarashi, W. Suda, C. Luo, T. Kawaguchi, I. Motoo, S. Narushima, Y. Kiguchi, K. Yasuma, E. Watanabe and T. Tanoue, Ectopic colonization of oral bacteria in the intestine drives TH1 cell induction and inflammation, Science, 2017, 358, 359–365 CrossRef CAS PubMed.
- N. Dutzan, T. Kajikawa, L. Abusleme, T. Greenwell-Wild, C. E. Zuazo, T. Ikeuchi, L. Brenchley, T. Abe, C. Hurabielle, D. Martin, R. J. Morell, A. F. Freeman, V. Lazarevic, G. Trinchieri, P. I. Diaz, S. M. Holland, Y. Belkaid, G. Hajishengallis and N. M. Moutsopoulos, A dysbiotic microbiome triggers TH17 cells to mediate oral mucosal immunopathology in mice and humans, Sci. Transl. Med., 2018, 10, eaat0797 CrossRef PubMed.
- J. M. Allaire, S. M. Crowley, T. L. Hong, S. Y. Chang, H. J. Ko and B. A. Vallance, The intestinal epithelium: central coordinator of mucosal immunity, Trends Immunol., 2018, 39, 677–696 CrossRef CAS.
- L. Ferrucci and E. Fabbri, Inflammageing: chronic inflammation in ageing, cardiovascular disease, and frailty, Nat. Rev. Cardiol., 2018, 15, 505–522 CrossRef CAS.
- I. D. Pavord, R. Beasley, A. Agusti, G. P. Anderson, E. Bel, G. Brusselle, P. Cullinan, A. Custovic, F. M. Ducharme and J. V. Fahy, After asthma: redefining airways diseases, Lancet, 2017, 391, 350–400 CrossRef.
- M. Neri, L. Sansone, L. Pietrasanta, A. Kisialiou, E. Cabano, M. Martini, M. A. Russo, D. Ugolini, M. Tafani and S. Bonassi, Gene and protein expression of CXCR4 in adult and elderly patients with chronic rhinitis, pharyngitis or sinusitis undergoing thermal water nasal inhalations, Immun. Ageing, 2018, 15, 10 CrossRef PubMed.
- N. Jaffar, T. Okinaga, T. Nishihara and T. Maeda, Enhanced phagocytosis of Aggregatibacter actinomycetemcomitans cells by macrophages activated by a probiotic Lactobacillus strain, J. Dairy Sci., 2018, 101, 5789–5798 CrossRef CAS PubMed.
- C. Guo, L. Yang, J. Luo, C. Zhang, Y. Xia, T. Ma and L. Kong, Sophoraflavanone G from Sophora alopecuroides inhibits lipopolysaccharide-induced inflammation in RAW264.7 cells by targeting PI3K/Akt, JAK/STAT and Nrf2/HO-1 pathways, Int. Immunopharmacol., 2016, 38, 349–356 CrossRef CAS PubMed.
- C. Bi, H. Wang, Y. Wang, J. Sun, J. Dong, X. Meng and J. Li, Selenium inhibits Staphylococcus aureus -induced inflammation by suppressing the activation of the NF-κB and MAPK signalling pathways in RAW264.7 macrophages, Eur. J. Pharmacol., 2016, 780, 159–165 CrossRef CAS PubMed.
- Y. W. Liu, W. K. Ong, Y. W. Su, C. C. Hsu, T. H. Cheng and Y. C. Tsai, Anti-inflammatory effects of Lactobacillus brevis K65 on RAW 264.7 cells and in mice with dextran sulphate sodium-induced ulcerative colitis, Benefic. Microbes, 2016, 7, 387–396 CrossRef CAS PubMed.
- S. Gowrishankar, R. Thenmozhi, K. Balaji and S. K. Pandian, Emergence of methicillin-resistant, vancomycin-intermediate Staphylococcus aureus among patients associated with group A streptococcal pharyngitis infection in southern India, Infect., Genet. Evol., 2013, 14, 383–389 CrossRef PubMed.
- M. S. Chen, W. C. Lin, H. T. Yeh, C. L. Hu and S. M. Sheu, Propofol specifically suppresses IL-1β secretion but increases bacterial survival in Staphylococcus aureus -infected RAW264.7 cells, Mol. Cell. Biochem., 2018, 449, 117–125 CrossRef CAS.
- S. Gowrishankar, A. Kamaladevi, K. Balamurugan and S. K. Pandian, In vitro and In vivo biofilm characterization of methicillin-resistant staphylococcus aureus from patients associated with pharyngitis infection, BioMed Res. Int., 2016, 2016, 1289157 Search PubMed.
- Y. Wang, B. Ren, X. Zhou, S. Liu, Y. Zhou, B. Li, Y. Jiang, M. Li, M. Feng and L. Cheng, Growth and adherence of Staphylococcus aureus were enhanced through the PGE2 produced by the activated COX-2/PGE2 pathway of infected oral epithelial cells, PLoS One, 2017, 12, e0177166 CrossRef PubMed.
- B. Krismer, C. Weidenmaier, A. Zipperer and A. Peschel, The commensal lifestyle of Staphylococcus aureus and its interactions with the nasal microbiota, Nat. Rev. Microbiol., 2017, 15, 675–687 CrossRef CAS PubMed.
- L. Fang, H. M. Wu, P. S. Ding and R. Y. Liu, TLR2 mediates phagocytosis and autophagy through JNK signaling pathway in Staphylococcus aureus-stimulated RAW264.7 cells, Cell. Signalling, 2014, 26, 806–814 CrossRef CAS PubMed.
- C. Bi, H. Wang, Y. Wang, J. Sun, J. Dong, X. Meng and J. Li, Selenium inhibits Staphylococcus aureus-induced inflammation by suppressing the activation of the NF-κB and MAPK signalling pathways in RAW264.7 macrophages, Eur. J. Pharmacol., 2016, 780, 159–165 CrossRef CAS.
- X. Jiang, L. Fang, H. Wu, X. Mei, F. He, P. Ding and R. Liu, TLR2 regulates allergic airway inflammation and autophagy through PI3K/Akt signaling pathway, Inflammation, 2017, 40, 1382–1392 CrossRef CAS PubMed.
- I. T. Lee, C. W. Lee, W. H. Tung, S. W. Wang, C. C. Lin, J. C. Shu and C. M. Yang, Cooperation of TLR2 with MyD88, PI3K, and Rac1 in lipoteichoic acid–induced cPLA2/COX-2–dependent airway inflammatory responses, Am. J. Pathol., 2010, 176, 1671–1684 CrossRef CAS PubMed.
- Q. Zhang, J. Sun, Y. Wang, W. He, L. Wang, Y. Zheng, J. Wu, Y. Zhang and X. Jiang, Antimycobacterial and anti-inflammatory mechanisms of baicalin via induced autophagy in macrophages infected with mycobacterium tuberculosis, Front. Microbiol., 2017, 8, 2142 CrossRef PubMed.
- Z. X. Zhou, S. F. Mou, X. Q. Chen, L. L. Gong and W. S. Ge, Anti-inflammatory activity of resveratrol prevents inflammation by inhibiting NF-κB in animal models of acute pharyngitis, Mol. Med. Rep., 2018, 17, 1269–1274 CAS.
- X. Chen, Y. Lai, X. Song, J. Wu, L. Wang, H. Zhang, Z. Liu and Y. Wang, Polysaccharides from Citrus grandis associate with luteolin relieves chronic pharyngitis by anti-inflammatory via suppressing NF-κB pathway and the polarization of M1 macrophages, Int. J. Immunopathol. Pharmacol., 2018, 32, 1–7 Search PubMed.
- C. Li, F. Wu, W. Yuan, Q. Ding, M. Wang, Q. Zhang, J. Zhang, J. Xing and S. Wang, Systematic review of Herbal Tea (a traditional Chinese treatment method) in the therapy of chronic simple pharyngitis and preliminary exploration about its medication rules, Evid. Based Complement. Alternat. Med., 2019, 2019, 9458676 Search PubMed.
- L. Zha, J. Chen, S. Sun, L. Mao, X. Chu, H. Deng, J. Cai, X. Li, Z. Liu and W. Cao, Soyasaponins can blunt inflammation by inhibiting the reactive oxygen species-mediated activation of PI3K/Akt/NF-kB pathway, PLoS One, 2014, 9, e107655 CrossRef PubMed.
- Y. Wang, Y. Wu, Y. Wang, A. Fu, L. Gong, W. Li and Y. Li, Bacillus amyloliquefaciens SC06 alleviates the oxidative stress of IPEC-1 via modulating Nrf2/Keap1 signaling pathway and decreasing ROS production, Appl. Microbiol. Biotechnol., 2017, 101, 3015–3026 CrossRef CAS.
- R. Ai, J. Wei, D. Ma, L. Jiang, H. Dan, Y. Zhou, N. Ji, X. Zeng and Q. Chen, A meta-analysis of randomized trials assessing the effects of probiotic preparations on oral candidiasis in the elderly, Arch. Oral Biol., 2017, 83, 187–192 CrossRef PubMed.
- P. Rungsri, N. Akkarachaneeyakorn, M. Wongsuwanlert, S. Piwat, P. Nantarakchaikul and R. Teanpaisan, Effect of fermented milk containing Lactobacillus rhamnosus SD11 on oral microbiota of healthy volunteers: A randomized clinical trial, J. Dairy Sci., 2017, 100, 7780–7787 CrossRef CAS.
- L. M. Rocha-Ramírez, R. A. Pérez-Solano, S. L. Castañón-Alonso, S. S. Moreno Guerrero, P. A. Ramírez, G. M. García and C. Eslava, Probiotic Lactobacillus strains stimulate the inflammatory response and activate human macrophages, J. Immunol. Res., 2017, 2017, 4607491 Search PubMed.
- A. López-lópez, A. Camelo-castillo, M. D. Ferrer, Á. Simon-soro and A. Mira, Health-associated niche inhabitants as oral probiotics: The case of streptococcus dentisani, Front. Microbiol., 2017, 8, 379 Search PubMed.
- T. H. Lin, C. H. Lin and T. M. Pan, The implication of probiotics in the prevention of dental caries, Appl. Microbiol. Biotechnol., 2018, 102, 577–586 CrossRef CAS PubMed.
- G. Jia, N. Che, Y. Xia, P. F.-H. Lai, Z. Xiong, G. Wang, H. Zhang and L. Ai, Adhesion to pharyngeal epithelium and modulation of immune response: Lactobacillus salivarius AR809, a potential probiotic strain isolated from the human oral cavity, J. Dairy Sci., 2019, 102, 6738–6749 CrossRef CAS PubMed.
- C. Lu, Y. Song, J. Zhang, Y. Du, T. Wang, Y. Xue, F. Fu and L. Zhang, Yanshu spraying agent, a traditional Chinese medicine, relieves chronic pharyngitis in animals by anti-inflammatory and antibacterial effects, Exp. Ther. Med., 2014, 7, 990–994 CrossRef PubMed.
- M. S. Miao, B. J. Chang, M. Bai and L. Bai, Specification for preparation of animal models of acute pharyngitis (Draft), Pharmacol. Clin. Chin. Mater. Med., 2018, 34, 175–178 Search PubMed.
- J. Y. Huang, C. Y. Kao, W. S. Liu and T. J. Fang, Characterization of high exopolysaccharide-producing Lactobacillus strains isolated from mustard pickles for potential probiotic applications, Int. Microbiol., 2017, 20, 75–84 CAS.
- K. Chen, Q. Fu, D. Li, Y. Wu, S. Sun and X. Zhang, Wnt3a suppresses Pseudomonas aeruginosa-induced inflammation and promotes bacterial killing in macrophages, Mol. Med. Rep., 2016, 13, 2439–2446 CrossRef CAS PubMed.
- N. S. Oh, J. Y. Joung, J. Y. Lee and Y. Kim, Probiotic and anti-inflammatory potential of Lactobacillus rhamnosus 4B15 and Lactobacillus gasseri 4 M13 isolated from infant feces, PLoS One, 2018, 13, e0192021 CrossRef PubMed.
- T. Zhou, Z. Huang, X. Zhu, X. Sun, Y. Liu, B. Cheng, M. Li, Y. Liu, C. He and X. Liu, Alpha-1 antitrypsin attenuates M1 microglia-mediated neuroinflammation in retinal degeneration, Front. Immunol., 2018, 9, 1202 CrossRef PubMed.
- D. Lin, J. Li, X. Zhang, L. Wang and W. Zhang, Pomegranate peel polyphenols inhibits inflammation in LPS-induced RAW264.7 macrophages via the suppression of MAPKs activation, J. Funct. Foods, 2018, 43, 62–69 CrossRef.
- S. Guglielmetti, V. Taverniti, M. Minuzzo, S. Arioli, I. Zanoni, M. Stuknyte, F. Granucci, M. Karp and D. Mora, A dairy bacterium displays in vitro probiotic properties for the pharyngeal mucosa by antagonizing group A streptococci and modulating the immune response, Infect. Immun., 2010, 78, 4734–4743 CrossRef CAS PubMed.
- J. Wang, P. Zhang, H. He, X. Se, W. Sun, B. Chen, L. Zhang, X. Yan and K. Zou, Eburicoic acid from Laetiporus sulphureus (Bull.: Fr.) Murrill attenuates inflammatory responses through inhibiting LPS-induced activation of PI3K/Akt/mTOR/NF-κB pathways in RAW264. 7 cells, Naunyn Schmiedebergs Arch. Pharmacol., 2017, 390, 845–856 CrossRef CAS PubMed.
- L. Wang, Y. Xia, T. Chen, Y. Zeng, L. Li, Y. Hou, W. Li and Z. Liu, Sanyang Xuedai enhances the radiosensitivity of human non-small cell lung cancer cells via increasing iNOS/NO production, Biomed. Pharmacother., 2018, 102, 618–625 CrossRef CAS PubMed.
- B. P. Su, G. H. Park, Y. Um, H. N. Kim, H. M. Song, N. Kim, H. S. Kim and B. J. Jin, Wood-cultivated ginseng exerts anti-inflammatory effect in LPS-stimulated RAW264.7 cells, Int. J. Biol. Macromol., 2018, 116, 327–334 CrossRef PubMed.
- J. T. Lin, Y. Y. Chang, Y. C. Chen, B. Y. Shen and D. J. Yang, Molecular mechanisms of ethanolic extract from Muntingia calabura Linn. fruit against lipopolysaccharide-induced pro-inflammatory mediators in macrophages, Food Funct., 2017, 8, 1245–1253 RSC.
- T. Li, D. Gao, M. Du, X. Cheng and X. Mao, Casein glycomacropeptide hydrolysates inhibit PGE2 production and COX2 expression in LPS-stimulated RAW 264.7 macrophage cells via Akt mediated NF-κB and MAPK pathways, Food Funct., 2018, 9, 2524–2532 RSC.
- J. Pan, L. Cheng, X. Bi, Z. Xin, S. Liu, X. Bai, F. Li and A. Z. Zhao, Elevation of ω-3 polyunsaturated fatty acids attenuates PTEN-deficiency induced endometrial cancer development through regulation of COX-2 and PGE2 production, Sci. Rep., 2015, 5, 14958 CrossRef CAS PubMed.
- Z. Yu, X. Wei and H. Wang, Resveratrol treatment inhibits acute pharyngitis in the mice model through inhibition of PGE2/COX-2 expression, Saudi J. Biol. Sci., 2017, 25, 1468–1472 CrossRef PubMed.
- L. T. Zhou, K. J. Wang, L. Li, H. Li and M. Geng, Pinocembrin inhibits lipopolysaccharide-induced inflammatory mediators production in BV2 microglial cells through suppression of PI3K/Akt/NF-κB pathway, Eur. J. Pharmacol., 2015, 761, 211–216 CrossRef CAS PubMed.
- S. R. Hyam, I. A. Lee, G. Wan, K. A. Kim, J. J. Jeong, S. E. Jang, M. J. Han and D. H. Kim, Arctigenin ameliorates inflammation in vitro and in vivo by inhibiting the PI3K/AKT pathway and polarizing M1 macrophages to M2-like macrophages, Eur. J. Pharmacol., 2013, 708, 21–29 CrossRef CAS PubMed.
- G. W. Fan, Y. Zhang, X. Jiang, Y. Zhu, B. Wang, L. Su, W. Cao, H. Zhang and X. Gao, Anti-inflammatory activity of baicalein in LPS-stimulated RAW264.7 macrophages via estrogen receptor and NF-κB-dependent pathways, Inflammation, 2013, 36, 1584–1591 CrossRef CAS PubMed.
- L. Meng, L. Li, S. Lu, K. Li, Z. Su, Y. Wang, X. Fan, X. Li and G. Zhao, The protective effect of dexmedetomidine on LPS-induced acute lung injury through the HMGB1-mediated TLR4/NF-κB and PI3K/Akt/mTOR pathways, Mol. Immunol., 2018, 94, 7–17 CrossRef CAS PubMed.
- R. Su, X. Jin, W. Zhang, Z. Li, X. Liu and J. Ren, Particulate matter exposure induces the autophagy
of macrophages via oxidative stress-mediated PI3K/AKT/mTOR pathway, Chemosphere, 2017, 167, 444–453 CrossRef CAS.
- J. F. Xue, Z. M. Shi, J. Zou and X. L. Li, Inhibition of PI3K/AKT/mTOR signaling pathway promotes autophagy of articular chondrocytes and attenuates inflammatory response in rats with osteoarthritis, Biomed. Pharmacother., 2017, 89, 1252–1261 CrossRef CAS.
Footnote |
† These authors contributed equally to this article. |
|
This journal is © The Royal Society of Chemistry 2020 |
Click here to see how this site uses Cookies. View our privacy policy here.