DOI:
10.1039/C9FO02322D
(Paper)
Food Funct., 2020,
11, 662-679
Total triterpenoids from the fruits of Chaenomeles speciosa exerted gastroprotective activities on indomethacin-induced gastric damage via modulating microRNA-423-5p-mediated TFF/NAG-1 and apoptotic pathways
Received
4th October 2019
, Accepted 18th December 2019
First published on 19th December 2019
Abstract
Our previous studies have demonstrated that the total triterpenes from the fruits of Chaenomeles speciosa (CSTT) exhibit effective therapeutic effects on gastric ulcer patients and animals. The present aim is to further investigate the mechanisms involved. The results indicated that CSTT could ameliorate IND-induced gastric injury, which was related to promoting IND-damaged GES-1 cell proliferation and migration, improving the IND-damaged rat GBF, ulcer area, inhibition rate and pathologic changes of gastric mucous tissue, increasing the amount of adhered gastric mucus, attenuating the volume and total acidity of the gastric effluents, and augmenting the gastric pH; further studies showed that CSTT obviously downregulated miR-423-5p mRNA, NAG-1 mRNA and protein expression, Bax, Bad, cytosol cytochrome C, Apaf-1, cleaved-caspase-3, and cleaved-caspase-9 protein expression and cytosol cytochrome C concentration, and upregulated TFF1, TFF2 and TFF3 mRNA and protein expression, Bcl-2, Bcl-xl, pro-caspase-3, and pro-caspase-9 protein expression, mitochondrial viability, mitochondrial cytochrome C concentration and Bcl-2/Bax, Bcl-xl/Bad ratios. These findings demonstrated that CSTT protected against IND-induced gastric damage by depressing miR-423-5p expression and modulating the TFF/NAG-1 pathway, which in turn restrained mitochondrion-mediated apoptosis.
1. Introduction
Gastric ulcers affect people of all ages and half of the world's population, and are being considered as the new “plague of the 21st century”.1 As is well known, the gastric mucosa is known as the first guard to protect the stomach from ulcer injury, while the aetiology of gastric ulcer is related to imbalances between gastric mucosal protective and aggressive factors.2 Therefore, reducing or eliminating the aggressive factors, returning to the balance of gastric ulcer between mucosal protective and aggressive factors, and then restoring the normal functions of the gastric mucosal barrier could be crucial for treating gastric ulcer.
MicroRNAs (miRs) are considered as pivotal post-transcriptional regulators, which are concerned with cell growth, differentiation and disappearance, as well as many physiological and pathological processes.3 Recent evidence indicates that miR-423-5p is widely involved in the pathological processes of peptic ulcer, gastrointestinal malignancies, diabetes mellitus and heart failure.4 Liu and his colleagues5 demonstrated that miR-423-5p might negatively adjust the trefoil factor family 1 (TFF1, a member of the trefoil factor family) expression, and involve in the proliferation and invasion-related processes of gastric cancer in a dose-dependent manner. Simultaneously, our previous studies also confirmed that it negatively regulated the mRNA and protein expression levels of TFF1 and participated in the development of indomethacin (IND)-induced gastric damage.6 Accumulated evidence indicates that TFF, including TFF1, TFF2 and TFF3, plays central roles in protecting the gastric mucosa and promoting repair of the damaged gastric mucosa by triggering its mediated signaling cascades.7 On the one hand, it forms a “mucus cap” with the residual nucleus of mucus and epithelial cells, covers the lesion and protects the “bare wound”; on the other hand, it can activate normal epithelial cells around the lesion through the comprehensive regulation of TFF triggering signaling cascades, promote their migration to the lesion site, accelerate the healing of the damaged gastric mucosa through cell migration, proliferation, differentiation, and angiogenesis, and reconstruct the mucosal barrier.8,9
Nonsteroidal antiinflammatory drug (NSAID)-activated gene-1 (NAG-1) is a divergent member of the transforming growth factor-β superfamily, which is also an important target gene mediated by NSAIDs.10 Colucci et al.11 indicated that the healing of gastric ulcer induced by acetic acid in rats was obviously delayed, and the NAG-1 expression was significantly increased after IND administration. Concurrently, we also confirmed that the NAG-1 expression in IND-damaged GES-1 cells and mouse gastric tissues was evidently elevated.12 The data showed that the gastric injury was closely related to the activation of the apoptosis pathway mediated by NAG-1. With the aforementioned analysis, we can easily discover that miR-423-5p, TFF and NAG-1 play an extremely important role in the processes of gastric damage and healing. Therefore, targeting miR-423-5p and its downstream TFF/NAG-1 signaling pathway will provide a new therapeutic tool for the prevention and treatment of gastric injury.
The fruits of Chaenomeles speciosa (Sweet) Nakai, also known as “mugua”, might be not only used directly for food, but also processed into edible and health care derived products (for example jam, fruit jelly, fruit liquor, fruit vinegar, candy, yogurt, cosmetics and skincare, etc.), and have been a commonly used traditional medicine in China for thousands of years. Studies on their chemical constituents indicated that their main constituents are triterpenoids, flavonoids, phenolic and phenylpropionic acids, etc., and they possess the traditional efficacy to relax channels and activate collaterals, harmonize the stomach and eliminate dampness.13,14 Modern pharmacological studies have confirmed that “mugua” might prevent and treat several clinical conditions, such as rheumatism, cholera, dysentery, enteritis, beriberi, vitamin C deficiency syndrome, neuralgia, migraine, stroke and depression.13,15 Our previous studies demonstrated that the total triterpenes from the fruits of Chaenomeles speciosa (CSTT) exhibit effective therapeutic effects on gastric ulcer patients and animals, and further confirmed that TFF1 and the apoptotic pathway are closely interrelated with its exertion of gastroprotection.12,16–18 However, its underlying molecular mechanisms have not been fully elucidated.
Based on the core role of miR-423-5p and TFF/NAG-1 and apoptotic pathways in ameliorating the damaged gastric mucosal repair and healing, and gastroprotection of CSTT in our previous studies, the current study was performed to further investigate its protective effect on IND-damaged GES-1 cells and rats, and its underlying mechanisms through modulating microRNA-423-5p-mediated TFF/NAG-1 and apoptotic pathways.
2. Materials and methods
2.1. Plant material
The fruits of Chaenomeles speciosa (Sweet) Nakai harvested in July 2018 were purchased from Langping Mugua Planting Base (Yichang, China), and were identified by professor Junzhi Wang, China Three Gorges University. Voucher specimens (2018-0728) are deposited in the College of Biological and Pharmaceutical Sciences, China Three Gorges University.
2.2. Reagents
All solvents used for the extraction and analysis of CSTT in the present study were of analytical grade and purchased from Sinopharm Chemical Reagent Co., Ltd (Shanghai, China). AB-8 macroporous resin was obtained from Wako Pure Chemical Industries, Ltd (Osaka, Japan). Maslinic acid, betulin, betulinic acid, oleanolic acid, ursolic acid and 3-O-acetyl ursolic acid were purchased from the National Institutes for Food and Drug Control (Beijing, China). All the reagents used for cell culture were purchased from Gibco (Carlsbad CA, USA). Hoechst 33258, dimethyl sulfoxide (DMSO), IND, 3-(4,5-dimethylthiazol-2-yl)-2,5-diphenyltetrazolium bromide (MTT) and 5,5′,6,6′-tetrachloro-1,1′,3,3′-tetraethylbenzimidazolcarbocyanine iodide (JC-1) were purchased from Sigma-Aldrich (St Louis, USA). An Annexin V-FITC/Propidium Iodide (PI) apoptosis detection kit was obtained from BD Biosciences (Bedford, MA, USA). A Trizol isolation kit was obtained from Invitrogen Corporation (Carlsbad, CA, USA), a Luciferase Reporter Assay System, EasyScript First-Strand cDNA Synthesis SuperMix and SYBR Green real-time PCR Master Mix reagents were purchased from Promega Corporation (Madison, WI, USA). An miR-423-5p mimic, an miR-423-5p inhibitor, and an miR mimic negative control (miR-NC) were obtained from GenePharma Company (Shanghai, China). siRNA for control, NAG-1, and Lipofectamine 3000 were purchased from Invitrogen Corporation (Carlsbad, CA, USA). Primary antibodies and secondary antibodies were purchased from Cell Signaling Technology, Inc. (Danvers, MA, USA). WesternBright ECL prime detection reagent was obtained from Boshide Biotechnology Company (Wuhan, China). Other chemical reagents used in this study were of analytical grade.
2.3. Extraction and analysis of CSTT
The extraction and separation of CSTT from the fruits of Chaenomeles speciosa (Sweet) Nakai were put into effect according to the method described in our previous article.19 Briefly, fresh fruits of Chaenomeles speciosa (Sweet) Nakai (10.0 kg) were sliced and extracted with 15-fold (w/v) 95% ethanol by the ultrasonic extraction method twice, 30 min each time. The extract was evaporated in vacuo at 50 °C to remove ethanol. The residue was first defatted with petroleum ether, and then extracted with ethyl acetate to obtain the crude triterpenoids. After evaporating ethyl acetate in vacuo, the crude extract was resuspended in 1.5 L of water and placed in an AB-8 macroporous resin column (50 × 1000 mm), and successively eluted with 4.0 L of water, 4.0 L of 60% ethanol, and 4.0 L of 95% ethanol. The 95% ethanol elute was concentrated in vacuo and lyophilized to obtain light brown CSTT (32.0 g, 0.32% yield from the fresh fruits of Chaenomeles speciosa). The total triterpenoid content of the extract expressed as an oleanolic acid (OA) equivalent (mg OA per g fresh weight) was determined by colorimetric methods as previously described.20 The chemical profile of CSTT, as well as contents of 6 marked components in it, was analyzed by high performance liquid chromatography (HPLC) using a Waters system equipped with a Delta 600 pump and a diode array detector (DAD) (Milford, MA, USA) on an (MP) F51103 column (4.6 × 250 mm, 5 μm) (Shiseido, Tokyo, Japan); the detection wavelength range of the DAD was 190–400 nm with the monitoring wavelength set at 210 nm. The mobile phase was a binary eluent of methanol (A) and 0.5% (v/v) phosphoric acid water (B) with the following gradient program: 0–5 min changed from 15% A to 35% A, 5–15 min from 35% A to 45% A, 15–20 min from 45% A to 75% A, and 20–25 min from 75% A to 90% A. The flow rate was 1.0 mL min−1, and the column temperature was set to 35 °C. The injection volume was set to 10 μL.
2.4. Cell culture and transfection
The nonmalignant gastric epithelial GES-1 cell lines were obtained from the American Type Culture Collection (ATCC) and grown in RPMI-1640 medium containing 10% phosphate buffered saline (PBS) and 1% antibiotic (100 μg mL−1 streptomycin and 100 units per mL penicillin) at 37 °C under a humidified 5% CO2 atmosphere. ① Transfection of miR-423-5p: miR-423-5p mimics (5′-UGAG-GGGCAGAGAGCGAGACUUU-3′), anti-miR-423-5p (5′-AAAGUCUCGCUCUCUGCCCCU-CA-3′) and miR-NC (5′-CAGUACUUUUGUGUAGUA-3′) were ordered from GenePharma Company (Shanghai, China). GES-1 cells were transfected with 20 nM miR-423-5p mimics, an miR-423-5p inhibitor or scrambled miR-NC for 24 h. ② Transfection of NAG-1 complementary DNA and siRNA: NAG-1 complementary DNA (cDNA) transfection was performed as previously described.20 siRNA for control and NAG-1 (sense: 5′-CUCAGUUGUCCUGCCCUGUdTdT-3′, antisense: 5′-ACAGGGC-AGGACAACUGAGdTdT-3′) were purchased from Invitrogen Corporation (Carlsbad, CA, USA). All transfection experiments were performed using the Lipofectamine 3000 reagent obtained from Invitrogen Corporation (Carlsbad, CA, USA).
2.5. Viability assay
The GES-1 cells (1 × 105 cells per well) were seeded in a 96-well plate to adhere for 24 h, and then pretreated with CSTT (2.5, 5, 10, 20, 40, and 80 μg mL−1) for 6 h, and then IND (700 μM, final concentration) was added whose concentration induced GES-1 cell injury was confirmed according to the method described by Qin et al.,21 and continuously for 18 h and 42 h. MTT assay was also used to evaluate the proliferation of CSTT in the presence or absence of an IND environment. The cell viability assays of GES-1 cells pretreated with CSTT (20 μg mL−1) or combined with the miR-423-5p mimics, miR-423-5p inhibitor, and NAG-1 siRNA were also similar to it.
2.6. Colony-forming assay
The cell colony formation experiment was carried out as previously described with some modifications.22 Briefly, the GES-1 cells (300 cells per well) were seeded in 6-well plates to adhere for 24 h, and then treated with various concentrations of CSTT (2.5, 5, 10, 20, 40, and 80 μg mL−1), respectively. The culture media were replaced every 3 days. 18 h before the end of the experiment, the test groups were added with or without 700 μM IND (final concentration). 14 days after incubation, the cells were rinsed twice with PBS, and stained with 0.1% crystal violet for 15 min. After being rinsed twice with PBS to remove the residual dye, the colonies were counted under an inverted light microscope (Olympus, Tokyo, Japan), and the clone formation rate was calculated.
2.7. Wound-healing migration assay
The wound-healing migration assay was performed as previously described with minor modifications.2 In brief, the GES-1 cells (1 × 105 cells per well) were seeded in 24-well plates to adhere for 24 h, and then the scratch-wounds were made by scraping the confluent cell monolayer across every culture plate using a 200 μL micropipette tip. After being rinsed with PBS to remove the floating cells, different concentrations of CSTT (5, 10, and 20 μg mL−1) were then added, and the cells were unceasingly cultured. Phase contrast images were recorded (0, 12 and 24 h after scratching) under the inverted light microscope, and analyzed using an Image Pro software system (Bethesda, MD, USA). In order to ensure recording the same region, marks were made on the wells across the scratch-wound area.
2.8. Hoechst staining
After the GES-1 cells (1 × 105 cells per well) were seeded in 12-well plates to adhere for 24 h, the cells were pretreated with CSTT (20 μg mL−1) or combined with the miR-423-5p mimics and miR-423-5p inhibitor for 6 h, and then 700 μM IND (final concentration) was added except for the control group. 18 h after continuous incubation, the cells were rinsed twice with PBS, and stained using Hoechst 33258 dye at 37 °C for 10 min. After being rinsed with PBS two times to remove the residual dye, the cell morphological evaluations of nuclear condensation and fragmentation were performed under a fluorescence microscope (Olympus, Tokyo, Japan).
2.9. Annexin V-FITC/PI cytometric assay
The adherent cells were pretreated with CSTT (20 μg mL−1) or combined with the miR-423-5p mimics and miR-423-5p inhibitor for 6 h, and then 700 μM IND (final concentration) was added except for the control group. 18 h after continuous incubation, the cells were harvested, rinsed with cold PBS and stained with Annexin V-FITC/PI double-staining. The apoptosis was detected by using a flow cytometer obtained from BD Biosciences (Bedford, MA, USA) with the excitation and emission settings at 488 and 530 nm, respectively.
2.10. Mitochondrial viability assay
The adherent GES-1 cells were pretreated with CSTT (20 μg mL−1) or combined with the miR-423-5p mimics and NAG-1 siRNA for 6 h, and then 700 μM IND (final concentration) was added except for the control group. 18 h after continuous cultivation, the cells were harvested, and the viability of mitochondria was measured using a JC-1-Mitochondrial Membrane Potential Assay Kit according to the method introduced in our previous article.23
2.11. Luciferase reporter assay
The predicted binding site in the 3′-UTR of TFF was amplified by PCR, and inserted into the pmir GLO Luciferase miRNA Target Expression Vector (Promega, Madison, USA), which was referred to as TFF wild type (TFF-WT). The predicted binding sites were replaced to form the corresponding negative controls, such as, TFF mutated type (TFF-MUT). The vectors were transfected with the miR-423-5p mimic or the mimic NCs by using the Lipofectamine 3000 reagent obtained from Invitrogen Corporation (Carlsbad, CA, USA). 48 h after transfection, luciferase activities were assessed by using the Luciferase Reporter Assay System (Promega, Madison, USA).
2.12. Animal and treatments
Male Sprague-Dawley rats (age 6–7 weeks) were obtained from the Experimental Animal Center of China Three Gorges University (Yichang, China), which were raised under a 12 h light/dark cycle and humidity and temperature controlled environment. The rats had free access to food and water ad libitum. All animal experimental procedures in our study were conducted according to the guidelines of the National Institutes of Health (Bethesda, Maryland, USA). The experimental protocols were approved by the Experimental Animal Care and Use Committee of China Three Gorges University (Permission number: CTGUEAC-2018-056).
Rat gastric mucosal damage was carried out according to the modified method of Luo et al.24 After one week of acclimatization, the experimental rats were randomly divided into five groups (n = 20 per group). (1) Control group; (2) IND group; (3) CSTT-L (25 mg kg−1) + IND group; (4) CSTT-M (50 mg kg−1) + IND group; and (5) CSTT-H (100 mg kg−1) + IND group. The rats were pretreated orally with CSTT once a day for 21 consecutive days in the CSTT groups except for control and IND groups which were administered a vehicle (0.5% carboxymethylcellulose sodium). On the last day of treatment, the experimental rats were deprived of food but allowed free access to water for 24 h after completing the final administration, and then IND (100 mg kg−1) was administered to all groups by gavage except for the control group. The experiment was carried out 2 h and 6 h after IND administration, respectively.
2.13. Gastric juice parameter determination
2 h after IND administration, the pylorus ligation of 10 rats randomly selected in each group was performed under diethyl ether anesthesia, and then drinking water was withheld. After 4 h, the rat ligated pylorus was anaesthetized with urethane (1.2 g kg−1, i.p.). The abdomen was opened and the stomach was removed. The stomach was opened along the greater curvature, the gastric content was collected and drained into a graduated centrifuge tube, and then centrifuged at 3000 rpm for 15 min. The supernatant obtained was used for the determination of gastric juice parameters (volume, pH and total acidity) as previously described.25
2.14. Gastric ulcer analysis
After the gastric contents were removed, the whole stomachs were rinsed with ice-cold normal saline, and then laid flat on clean white paper and photographed. The ulcer area was analyzed using the ImageJ Morphology Analysis System (National Institutes of Health, Bethesda, Maryland, USA) by an experienced and board-certified pathologist who was blinded to the treatment. The inhibition percentage was calculated using the following formula: (ulcer area of IND group − ulcer area of CSTT group)/(ulcer area of IND group) × 100%. After being photographed, the gastric tissue sample (0.5 cm × 0.5 cm) obtained from each stomach was immersed in 4% paraformaldehyde for histopathological assay, and the residual gastric tissues were stored at −80 °C for biochemical and molecular analyses.
2.15. Gastric blood flow (GBF) measurement
6 h after administrating IND, the remaining rats in each group were anesthetized with urethane (1.2 g kg−1, i.p.), and the stomachs were opened along the greater curvature for the GBF measurement at the ulcer margin using a BPM403A laser Doppler flowmeter (Vasamedics, St Paul, MN, USA) according to the method described by Magierowski et al.26
2.16. Gastric wall mucus content evaluation
After the GBF was measured, the rats were sacrificed, and the stomach was excised. The gastric wall mucus content of the experimental rats was evaluated by using the Alcian blue dying method according to a previous description.25
2.17. Histopathological analysis
Gastric tissues fixed in 4% paraformaldehyde were embedded in paraffin, and sectioned at 5.0 μm, and then stained with hematoxylin and eosin. Under a light microscope (Olympus, Tokyo, Japan), the sections were assessed by an experienced and board-certified pathologist in a blinded manner according to the criteria established by Laine and Weinstein.27
2.18. Immunohistochemical staining
Formaldehyde-fixed gastric tissue was embedded in paraffin for TFF1 immunohistochemical examination. Immunohistochemical staining was performed according to the method described by Cheng et al.28
2.19. Quantitative real-time polymerase chain reaction (qRT-PCR) analysis
Total RNA was extracted from GES-1 cells and gastric tissue samples with the Trizol reagent (Invitrogen, Carlsbad, CA, USA) according to the manufacturer's protocols, and then total RNA was reverse transcribed into cDNA. miRNA and mRNA expression levels were quantified by using qPCR with SYBR Green real-time PCR Master Mix reagents. U6 and glyceraldehyde-3-phosphate dehydrogenase (GAPDH) were used as loading controls, respectively. The primer sequences employed in qRT-PCR are listed in Table 1, and synthesized by Shanghai Sangon Biotech Company, China.
Table 1 Primer sequences used in qRT-PCR
Genes |
Forward primer (5′–3′) |
Reverse primer (5′–3′) |
hsa-miR-423-5p |
ATGGTTCGTGGGTGAGGGGCAGAGAGCGAGAGC |
GTGCAGGGTCCGAGGT |
AGGGTCCGAGGTATTCG |
hTFF1 |
GCAAATAAGGGCTGCTGTTTC |
GAAGCGTGTCTGAGGTGTCC |
hTFF2 |
CCCCCATAACAGGACGAAC |
ATGAAGTTGGAGAAGCAGCAC |
hTFF3 |
TCCCCAAGCAAACAATCC |
TGAAACACCAAGGCACTCC |
hNAG-1 |
CTCCAGATTCCGAGAGTTGC |
AGAGATACGCAGGTGCAGGT |
rno-miR-423-5p |
CATGAGCCACTGCCCACAGC |
ATCCAGTGCAGGGTCCGAGG |
rTFF1 |
TTGCCCAGAACCAGGAAG |
GTGCCGAGTCTTGATGTAACC |
rTFF2 |
GTGCCCCTCTCTTGGTAGTG |
GACGCTTGGTTTGGAAGTG |
rTFF3 |
ATGGAGACCAGAGCCTTCT |
GGATGCTGGAGTCAAAACAG |
rNAG-1 |
CCCAGCTGTCCGGATACTC |
ATCATAAGTCTGCAGTGACA |
U6 |
CTCGCTTCGGCAGCACA |
AACGCTTCACGAATTTGCGT |
GAPDH |
GACAACTTTGGCATCGTGGA |
ATGCAGGGATGATGTTCTGG |
2.20. Western blot analysis
The total protein and cytosol protein in the GES-1 cells and gastric tissues were isolated by using appropriate separation kits. The protein content was detected using a nucleic acid analyzer (Thermo scientific, USA). The protein expression levels of TFF (TFF1, TFF2 and TFF3) and apoptosis-related proteins (Bax, Bad, Bcl-2, Bcl-xl, cytosol cytochrome C, apoptotic protease activating factor 1 (Apaf-1), pro-caspase-9, cleaved-caspase-9, pro-caspase-3, cleaved-caspase-3 and NAG-1) in GES-1 cells and gastric tissues were determined by using western blot analysis, which was performed according to our previous description.6,21
2.21. Mitochondrial cytochrome C release analysis
Mitochondria in the GES-1 cells and rat gastric tissues were isolated with a mitochondrial separation kit. The cytochrome C levels of mitochondria and cytoplasm were detected according to our previous description.29
2.22. Statistical analysis
The results were presented as mean ± SD, and analyzed statistically by one-way analysis of variance (ANOVA), followed by using the 21.0 statistical software package (SPSS Inc., Chicago, IL, USA) and Student's t-tests. Probability P values less than 0.05 were considered statistically significant.
3. Results
3.1. Chemical composition of CSTT and content determination of 6 marked components
In the present extraction and purification, the content of total triterpenoids obtained was 90.02 ± 3.16% (mg OA per g fresh weight) by using the colorimetric method. Ten chromatogram peaks were marked in the HPLC fingerprint chromatogram of CSTT, and six of them were identified by comparison with the retention time (tR) and UV spectrum of the corresponding peaks in the standard solution (Fig. 1A), and these were peaks 5 (maslinic acid, tR = 8.38 min), 6 (betulin, tR = 13.25 min), 7 (betulinic acid, tR = 13.58 min), 8 (oleanolic acid, tR = 15.92 min), 9 (ursolic acid, tR = 16.74 min) and 10 (3-O-acetyl ursolic acid), tR = 18.46 min, and their contents were determined by an external standard method to be 2.49 ± 0.23%, 10.57 ± 0.86%, 5.63 ± 0.39%, 21.94 ± 1.25%, 6.36 ± 0.73% and 3.89 ± 0.28%, respectively. These triterpenoids were divided into oleanane-type triterpenoids (maslinic acid and oleanolic acid), lupane-type triterpenoids (betulin and betulinic acid) and ursane-type triterpenoids (ursolic acid and 3-O-acetyl ursolic acid) according to their molecular skeleton. The chemical structures of the triterpenoid compounds are shown in Fig. 1B.
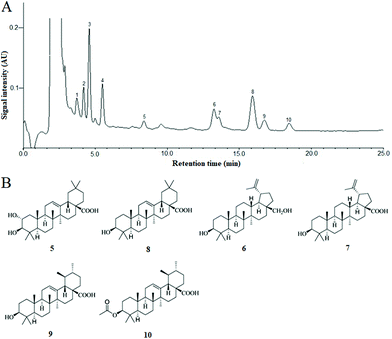 |
| Fig. 1 The chromatogram and chemical structures of total triterpenoids obtained from fresh fruits of Chaenomeles speciosa (Sweet) Nakai. (A) The chromatogarm of total triterpenoids. (B) Chemical structures of triterpenoids identified (oleanane-type triterpenoids: 5, maslinic acid and 8, oleanolic acid; lupane-type triterpenoids: 6, betulin and 7, betulinic acid and Ursane-type triterpenoids: 9, ursolic acid and 10, 3-O-acetyl ursolic acid). | |
3.2. CSTT promoted GES-1 cell proliferation
To evaluate the influence of CSTT on the cytotoxicity and proliferation on normal GES-1 cells and IND-damaged GES-1 cells, the cells were treated with CSTT (2.5–80 μg mL−1) in the presence or absence of 700 μM IND for 24 or 48 h. Following 24 and 48 h of treatment with CSTT in normal GES-1 cells, the proliferation of GES-1 cells was dose–time dependently promoted by 2.5, 5, 10, 20 and 40 μg mL−1 CSTT, and significantly inhibited at a dosage of 80 μg mL−1 CSTT (P < 0.05 or P < 0.01, respectively) (Fig. 2A). However, in the IND-damaged GES-1 cells, the concentrations of 2.5, 5, 10 and 20 μg mL−1 of CSTT boosted GES-1 cell proliferation, and 40 and 80 μg mL−1 of CSTT depressed the GES-1 cell proliferation (P < 0.05 or P < 0.01, respectively) (Fig. 2B). This implied that CSTT might promote GES-1 cell proliferation at low concentrations of CSTT, and the proliferation activity could be suppressed at high concentrations of CSTT. In harmony with the aforementioned MTT assay data, there were similar effects of colony formation by CSTT (Fig. 2D–F). Based on existing research, the appropriate concentrations of CSTT (under 20 μg mL−1) and treatment time (24 h) were, for further in vitro studies, identified.
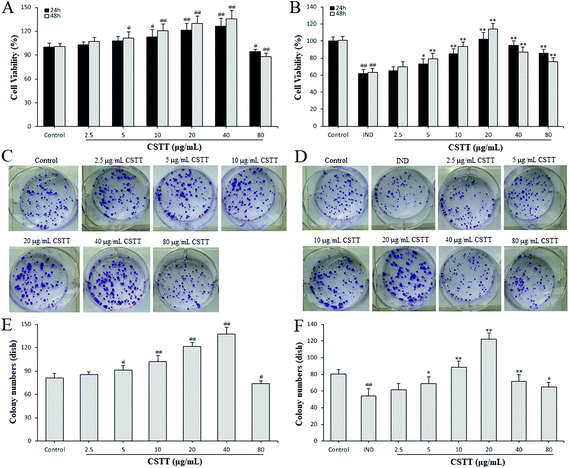 |
| Fig. 2 CSTT promoted GES-1 cell proliferation. (A and B) Cell viability in the presence or absence of 700 μM IND for 24 and 48 h. (C, D) Microscopic appearances of colony formation in the presence or absence of 700 μM IND for consecutive 14 d. (E and F) Colony number assay. The data are shown as the mean ± SD (n = 4). #P < 0.05, ##P < 0.01 compared with the control group; *P < 0.05, **P < 0.01 compared with the IND group. | |
3.3. CSTT ameliorated GES-1 cell wound healing
The gastric epithelium is the first significant barrier, and continuous epithelium renewal by proliferation and migration is very important in repairing the damaged gastric mucosa and maintaining mucosal structural integrity.30 After analyzing the promoting proliferation of CSTT, we further studied its effect on the migration of damaged GES-1 cells by scraping the wound. The different concentrations of CSTT (5, 10 and 20 μg mL−1) used for treating GES-1 cells for 0, 12 and 24 h obviously ameliorated cell wound healing by promoting migration and proliferation, and indicated dose–effect and time–effect relationships compared with the control group (P < 0.05 or P < 0.01, respectively) (Fig. 3).
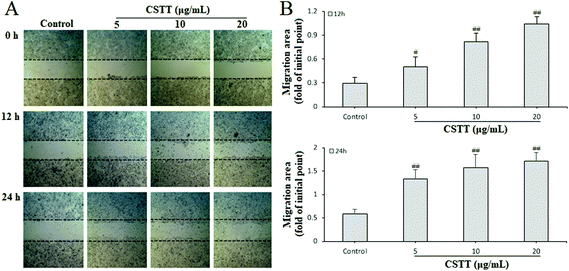 |
| Fig. 3 CSTT ameliorated GES-1 cell wound healing. (A) Microscopic appearances of cells migrating into the wounded area. (B) Cell migration ability. The cell migration assay was performed in the presence of CSTT (5, 10, and 20 μg mL−1) for 12 and 24 h. The data are shown as the mean ± SD (n = 4). #P < 0.05, ##P < 0.01 compared with the control group. | |
3.4. CSTT suppressed IND-induced GES-1 cell apoptosis via downregulation of miR-423-5p and modulation of the mitochondrion-dependent apoptotic pathway
In order to investigate the protective effect and potential mechanisms of CSTT on the IND-induced GES-1 cell injury, we detected the viability and apoptosis of CSTT on IND-damaged GES-1 cells after transfection with the miR-423-5p mimic through MTT, Hoechst-staining and flow cytometry assays, respectively. As shown in Fig. 4A–C, CSTT (20 μg mL−1) significantly promoted IND-damaged GES-1 cell proliferation, and repressed its apoptosis. In the Hoechst-staining assays, the number of condensed and fragmented nuclei of the CSTT group was remarkably reduced compared with that of the IND group, which was also confirmed by flow cytometry assay. To test whether miR-423-5p was involved in the protective effects of CSTT on IND-damaged GES-1 cells, we analyzed the miR-423-5p expression. As shown Fig. 4E, the expression level of miR-423-5p in IND-damaged GES-1 cells was obviously elevated compared with that of the control group (P < 0.01), and the miR-423-5p expression further increased in the miR-423-5p mimic-transfected GES-1 cells compared with that of the mimic NC group (P < 0.01). Interestingly, IND-damaged GES-1 and miR-423-5p mimic-transfected GES-1 cells pretreated with CSTT (20 μg mL−1) indicated obviously decreased miR-423-5p expression compared with the IND and mimic NC groups (P < 0.01, respectively). The data were consistent with CSTT increasing the cell viability and decreasing apoptotic cell rate (Fig. 4A–C). The above-mentioned results indicated that the cytoprotective effect of CSTT was related to suppressing miR-423-5p expression.
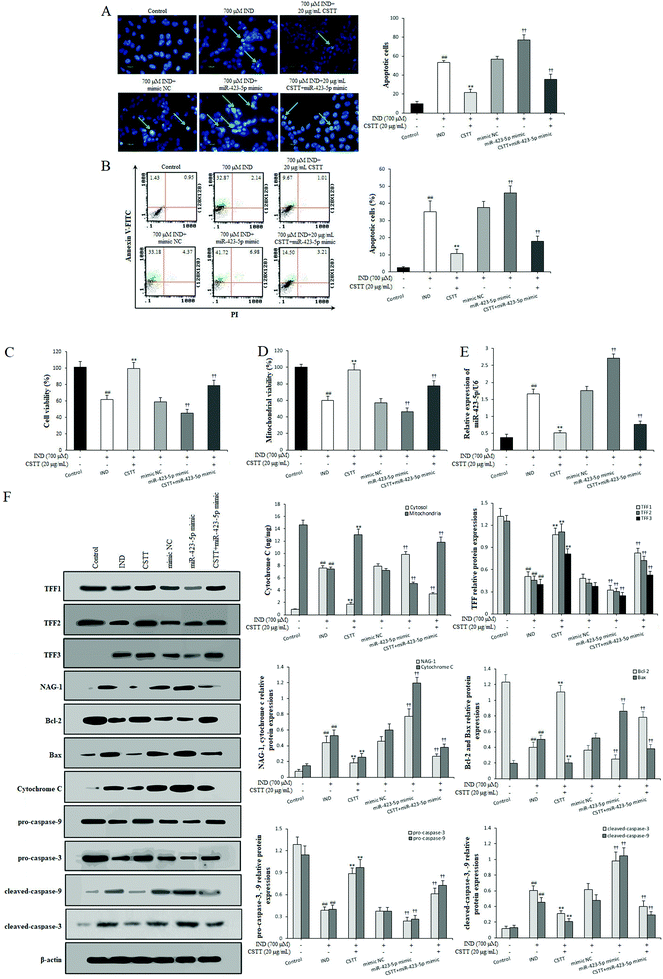 |
| Fig. 4 CSTT suppressed IND-induced GES-1 cell apoptosis via downregulation of miR-423-5p and modulation of the TFF/NAG-1 pathway. (A) Apoptotic cells by Hoechst-staining analysis. (B) Apoptotic cells by flow cytometry analysis. (C) Cell viability by MTT analysis. (D) Mitochondrial viability by flow cytometry analysis. (E) miR-423-5p mRNA expression. (F) Protein expression of the mitochondrial apoptosis pathway. The IND-damaged GES-1 cells were pretreated with CSTT and transfection with miR-423-5p alone or in combination. Apoptotic cells are shown by a green arrow head. All data are shown as the mean ± SD (n = 4). #P < 0.05, ##P < 0.01 compared with the control group; *P < 0.05, **P < 0.01 compared with the IND group; †P < 0.05, ††P < 0.01 compared with the mimic NC group. | |
The mitochondria are the center of apoptotic regulation, and the attenuation of mitochondrial viability causes membrane depolarization and triggers a cascade of mitochondrion-mediated apoptotic processes.31,32 In the present study, the mitochondrial viabilities were apparently attenuated in the IND and miR-423-5p mimic-transfected groups compared with those in the control and mimic NC groups (P < 0.01, respectively); conversely, CSTT pretreatment induced the increase of mitochondrial viability compared with the IND and mimic NC groups (P < 0.01, respectively) (Fig. 4D). Subsequently, we also found that the decreased mitochondrial viability caused the release of cytochrome C from the mitochondria into the cytosol and increased the cytosol cytochrome C protein expression in the IND and miR-423-5p mimic-transfected groups, which in turn resulted in the obvious increase of proapoptotic Bax, cleaved-caspase-9, and cleaved-caspase-3 protein expression levels and the significant reduction of antiapoptotic Bcl-2, pro-caspase-9, and pro-caspase-3 protein expression levels and the ratio of Bcl-2/Bax compared with the control and mimic NC groups (P < 0.01, respectively). As expected, CSTT pretreatment dramatically reversed the anomalous concentrations of cytochrome C in the mitochondria and cytosol and the abnormal expression levels of proapoptotic and antiapoptotic proteins, elevated the Bcl-2/Bax ratio and reduced the cytosol cytochrome C protein expression compared with the IND and mimic NC groups (P < 0.01, respectively), which in turn suppressed IND-induced GES-1 cell apoptosis (Fig. 4F).
3.5. miR-423-5p knockdown modulated TFF/NAG-1 pathway by targeting the TFF1 gene
To further confirm whether miR-423-5p was extended to the IND-induced GES-1 cell apoptosis, after the GES-1 cells were deleted miR-423-5p gene by transfecting with miR-423-5p inhibitor, we analyzed the GES-1 cell viability, apoptosis and TFF/NAG-1 pathway related protein expressions. As Fig. 5A–F show, the knockdown of miR-423-5p not only boosted the proliferation and restrained apoptosis, but also reduced the miR-423-5p expression in the miR-423-5p inhibitor-transfected GES-1 cells. Meanwhile, western blot analytical results also indicated that the TFF/NAG-1 pathway was heavily influenced in the miR-423-5p mimic-transfected GES-1 cells. As shown in Fig. 4F, the TFF1 and TFF2 protein expression levels were obviously debased in the miR-423-5p mimic-transfected GES-1 cells compared with those in the GES-1 cells transfected with mimic NC (P < 0.01, respectively); pretreatment with CSTT (20 μg mL−1) significantly elevated TFF1 and TFF2 expression levels. In contrast, the downregulation of protein expression levels of TFF1 and TFF2 induced by IND was also attenuated after knockdown of miR-423-5p (Fig. 5G), and the expression of NAG-1 was just the opposite to TFF1 and TFF2 expression. Interestingly, the protein expression of TFF3 was negative in the control GES-1 cells, and its expression was observed in the IND-damaged GES-1 cells, but pretreatment with CSTT or miR-423-5p knockdown further promoted TFF3 expression compared with the IND group or inhibitor NC group (P < 0.01, respectively) (Fig. 4F and 5G).
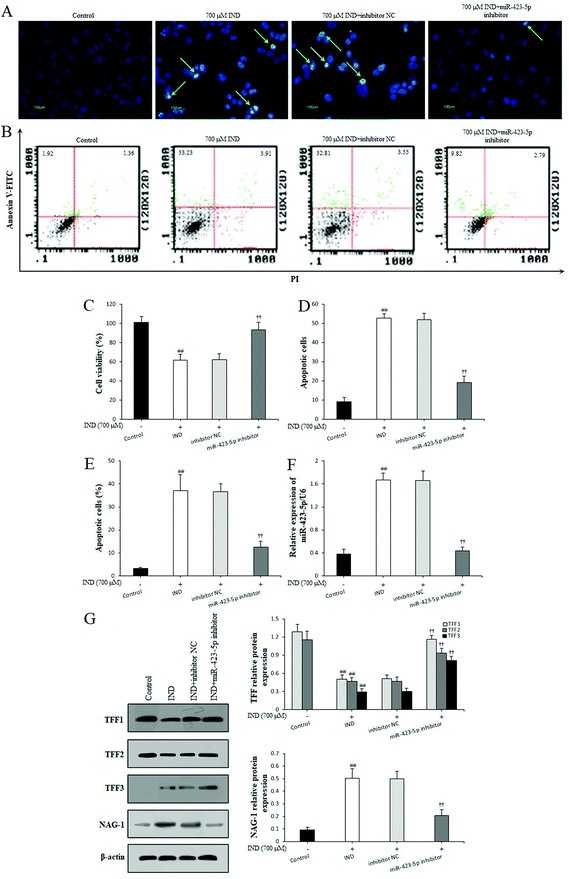 |
| Fig. 5 Knockdown of the miR-423-5p modulated TFF/NAG-1 pathway. (A and D) Apoptotic cells by Hoechst-staining analysis. (B and E) Apoptotic cells by flow cytometry analysis. (C) Cell viability by MTT analysis. (F) miR-423-5p mRNA expression. (G) Protein expression of the TFF/NAG-1 pathway. The IND-damaged GES-1 cells were treated with miR-423-5p inhibitor transfection. Apoptotic cells are shown by a green arrow head. The data are shown as the mean ± SD (n = 4). #P < 0.05, ##P < 0.01 compared with the control group; †P < 0.05, ††P < 0.01 compared with the inhibitor NC group. | |
Through the aforementioned analysis, we found that miR-423-5p performed the negative regulation of TFF in IND-damaged GES-1 cells. Nevertheless, whether TFF or one of them is the potential target gene of miR-423-5p is still unclear. To illustrate this, we predicted them using bioinformatics analysis on the online database. As shown in Fig. 6A, TFF1 was predicted to be a presumptive target gene of miR-423-5p. To further confirm this, the luciferase reporter assay was performed after transfection with the WT or MUT 3′-UTR sequence of TFF1 combined with mimic NC or the miR-423-5p mimic in GES-1 cells. The results showed that transfection with the miR-423-5p mimic significantly decreased the luciferase enzyme activity when co-transfected with WT 3′-UTR of TFF1, but caused no change in luciferase enzyme activity in MUT 3′-UTR of TFF1 (Fig. 6B). Meanwhile, we also found out that the mRNA and protein expression levels of TFF1 was obviously restrained by the miR-423-5p mimic, and remarkably ameliorated by the miR-423-5p inhibitor in GES-1 cells (Fig. 6C and D). In our study, miR-423-5p binding sites in the 3′-UTR of TFF2 and TFF3 were not predicted by using an online database (data not shown). Thus, TFF1 was a direct target gene of miR-423-5p, and the knockdown of miR-423-5p readily modulated the TFF/NAG-1 pathway.
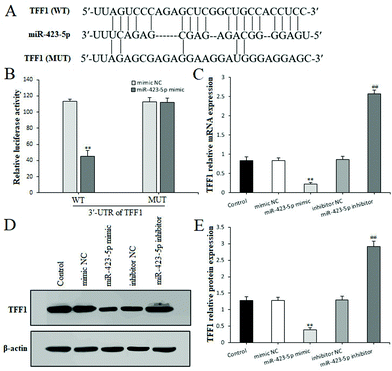 |
| Fig. 6 TFF1 was a direct target of miR-423-5p. (A) miR-423-5p binding site in the 3′-UTR of TFF1 predicted by bioinformatics analysis on the online database. (B) The luciferase activity assay. (C) TFF1 mRNA expression. (D and E) TFF1 protein expression. The GES-1 cells were subjected to miR-423-5p mimic or inhibitor transfection. The data are shown as the mean ± SD (n = 4). *P < 0.05, **P < 0.01 compared with the mimic NC group; #P < 0.05, ##P < 0.01 compared with the inhibitor NC group. | |
3.6. NAG-1 knockdown strengthened the protection of CSTT against IND–damaged GES-1 cells
To strengthen the evidence linking CSTT protection with suppression of NAG-1, NAG-1 siRNA was transfected in GES-1 cells to knockdown the NAG-1 expression. Fig. 7A–C indicate that the mRNA and protein expression levels of NAG-1 in GES-1 cells was significantly decreased by siRNA transfection compared with that in the siRNA NC and control groups (P < 0.01, respectively). In IND-damaged GES-1 cells, transfection with NAG-1 siRNA significantly elevated GES-1 cell proliferation, augmented the mitochondrial viabilities and restrained the release of cytochrome C from the mitochondria into the cytosol compared with the IND group (P < 0.01, respectively). After pretreatment with CSTT, the above amelioration had further been firmed up compared with the NAG-1 siRNA group (P < 0.01). In addition, qRT-PCR and western blot data also confirmed that the NAG-1 knockdown evidently depressed the release of cytochrome C from the mitochondria into the cytosol, attenuated the mRNA and protein expression levels of NAG-1, and the Bax, cytosol cytochrome C, cleaved-caspase-9, and cleaved-caspase-3 protein expression levels, and elevated Bcl-2, pro-caspase-9, and pro-caspase-3 protein expression levels compared with the IND group (P < 0.01, respectively) (Fig. 7F and G). CSTT pretreatment further strengthened the aforementioned efficacies on IND-damaged GES-1 cells after transfection with NAG-1 siRNA (P < 0.01, respectively), which were also coincident with CSTT's protective effect on the IND-damaged GES-1 cells (Fig. 4F). Taken together, NAG-1 knockdown results added to the evidence that NAG-1 siRNA and CSTT pretreatment could generate a synergistic protective effect on IND-induced GES-1 cell injury. The present data suggested that NAG-1 was negatively involved in CSTT mediated cytoprotection.
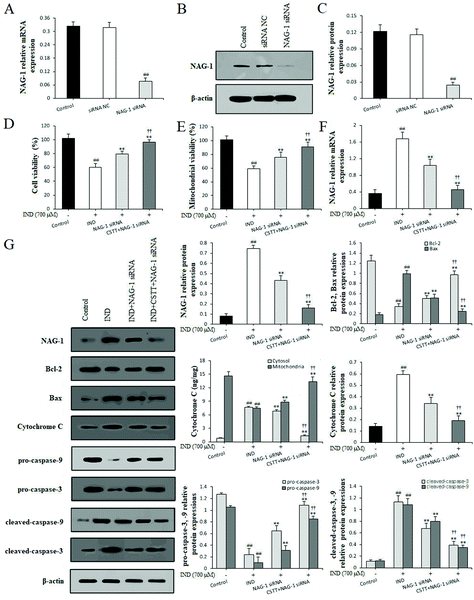 |
| Fig. 7 NAG-1 knockdown strengthened the cytoprotection of CSTT against IND-induced GES-1 cell injury. (A) NAG-1 mRNA expression in GES-1 cells. (B and C) NAG-1 protein expression in GES-1 cells. (D) Cell viability of IND-damaged GES-1 cells. (E) Mitochondrial viability of IND-damaged GES-1 cells. (F) NAG-1 mRNA expression in IND-damaged GES-1 cells. (G) Protein expression of the mitochondrial apoptosis pathway of IND-damaged GES-1 cells. The GES-1 and IND-damaged GES-1 cells were transfected with NAG-1 siRNA. The data are shown as the mean ± SD (n = 4). #P < 0.05, ##P < 0.01 compared with the control group; *P < 0.05, **P < 0.01 compared with the IND group; †P < 0.05, ††P < 0.01 compared with the NAG-1 siRNA group. | |
3.7. CSTT administration exerted a protective effect on IND-induced gastric damage in rats
Finally, the in vivo gastroprotective effect of CSTT on IND-induced gastric damage in rats was evaluated. As shown in Fig. 8A, there is an obvious decrease of the GBF at the ulcer margin in the IND group, which is as low as 38.23% compared with that of the control group (P < 0.01). After pretreatment with CSTT (25, 50 and 100 mg kg−1), the GBF at the ulcer margin was significantly ameliorated compared with the IND group (P < 0.05 or P < 0.01, respectively); specifically, the GBF at the ulcer margin in the CSTT-H group (100 mg kg−1) was dramatically raised relative to that of the IND group (30.21 ± 3.39 vs. 21.78 ± 3.42, P < 0.01).
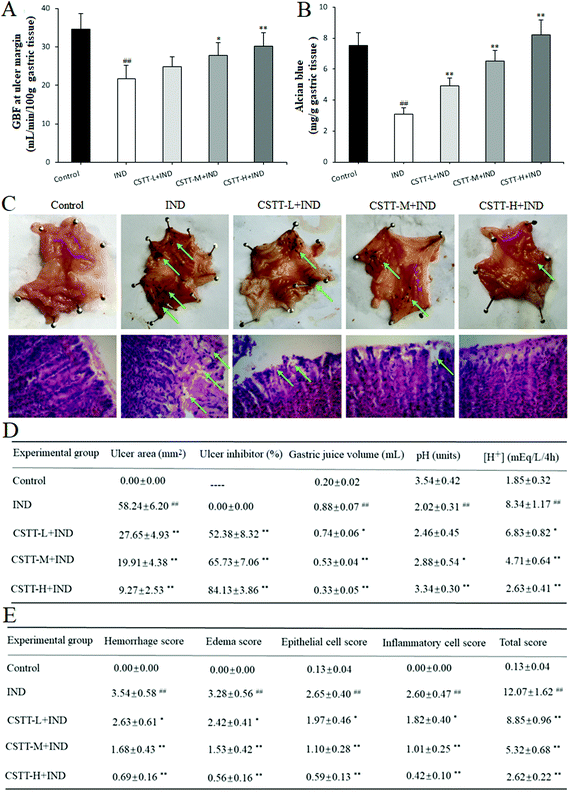 |
| Fig. 8 CSTT administration exerted a protective effect on IND-induced gastric damage in rats. (A) GBF at the ulcer margin. (B) Mucus level in the gastric mucosa. (C) Macroscopic and microscopic appearances of the representative gastric mucosa. (D) Gastric ulcer and gastric acid secretions. (E) Gastric mucosal damage score. Ulcer and lesions are shown by a green arrow head. The data are shown as the mean ± SD (n = 10). #P < 0.05, ##P < 0.01 compared with the control group; *P < 0.05, **P < 0.01 compared with the IND group. | |
Gastric mucus together with bicarbonate and phospholipids forms the first protective barrier of the gastric mucosa, which efficiently reduces the harmful effects of aggressive factors on the gastric mucosa.33 So the amount of adhered gastric mucus was an important index for evaluating the gastric mucosal action of drugs. As shown in Fig. 8B, oral administration of IND resulted in a significant depletion of rat gastric mucus compared with that in the control group (P < 0.01). Pretreatment with CSTT (25, 50 and 100 mg kg−1) readily caused mucus depletion, and increased the amount of adhered gastric mucus by 59.44%, 111.96% and 165.87% compared with the IND group (P < 0.01, respectively). Since the primary role of the gastric adherent mucus layer is to act against gastric acid damage, the current therapy in the clinical treatment of gastric ulcer is based on antisecretory drugs.28 In our experiment, the effects of CSTT on gastric juice parameters after a pylorus ligation test in IND-induced gastric mucosal injury rats were also evaluated. Fig. 8D indicates that oral administration of IND evidently increases the volume and total acidity of the gastric effluents, and reduces the gastric pH compared with the control group (P < 0.01, respectively), whereas CSTT (25, 50 and 100 mg kg−1) significantly attenuates the volume and total acidity of the gastric effluents by 15.91%, 39.77%, 62.50% and 18.11%, 43.53%, 68.47%, and augments the gastric pH by 20.30%, 42.57% and 65.35% compared with the IND group (P < 0.05 or P < 0.01, respectively).
Macroscopically, visual inspection showed that oral administration of IND induced serious hemorrhage in the gastric mucosal lining. The blood oozing out contained hemoglobin, which made the ulcerative surface appear black due to an acidic environment of the stomach. By gastric ulcer analysis, we found that the ulcer area had reached 58.24 ± 6.20 mm2 in the IND group; pretreatment with CSTT (25, 50 and 100 mg kg−1) efficiently attenuated the aforementioned abnormal varieties (27.65 ± 4.93, 19.91 ± 4.38, 9.27 ± 2.53 mm2) in a concentration-dependent manner, and there were obvious differences compared with the IND group (P < 0.01, respectively) (Fig. 8C and D). Microscopic analysis by H&E staining further confirmed that the structure of the stomach was intact, gastric glands were arrayed evenly, and there was no infiltration of the mucosa by inflammatory cells in the control group. In contrast, the IND group showed the typical characteristics of gastric mucosal damage, such as intense hemorrhage, submucosal edema, epithelial cell loss and inflammatory cell infiltration, and its mucosal damage scores of hemorrhage, edema, epithelial cell loss and inflammatory cell infiltration touched 3.54 ± 0.58, 3.28 ± 0.56, 2.65 ± 0.40 and 2.60 ± 0.47, respectively; CSTT (25, 50 and 100 mg kg−1) protected the gastric tissues from haemorrhagic lesions along with obvious attenuation of hemorrhage, edema, epithelial cell loss and inflammatory cell infiltration, and whose improvement rates were as much as 25.71%, 52.54%, 80.51%; 26.22%, 53.35%, 82.93%; 25.66%, 58.49%, 77.74% and 30.00%, 61.15%, 83.85% (P < 0.05 or P < 0.01, respectively) (Fig. 8C and E).
3.8. CSTT inhibited miR-423-5p expression and modulated the TFF/NAG-1 pathway in IND-damaged rats
After fully confirming the gastroprotective effect of CSTT on IND-induced gastric mucosal damage in rats, we next explored its potential mechanism. First, miR-423-5p expression in gastric tissues was analyzed by qRT-PCR. In the IND group, miR-423-5p expression significantly increased compared with the control group (P < 0.01, respectively); in contrast, CSTT (25, 50 and 100 mg kg−1) remarkably restrained miR-423-5p expression compared with the IND group (P < 0.01, respectively) (Fig. 9B), which was consistent with the in vitro data.
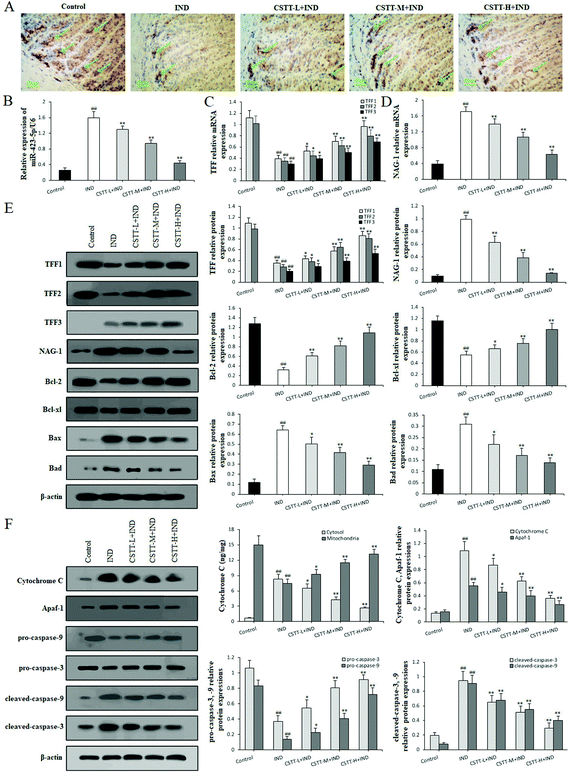 |
| Fig. 9 CSTT inhibited miR-423-5p expression and modulated the TFF/NAG-1 pathway in IND-damaged rats. (A) TFF1 immunohistochemical analysis. (B) miR-423-5p mRNA expression. (C) TFF mRNA expression. (D) NAG-1 mRNA expression. (E) Protein expression levels of TFF, NAG-1, Bcl-2, Bcl-xl, Bax and Bad. (F) Protein expression levels of cytosol cytochrome C, Apaf, caspase-9 and caspase-3. TFF1 positive expression levels in cytosol are shown by a green arrow head. The data are shown as the means ± SD (n = 4). #P < 0.05, ##P < 0.01 compared with the control group; *P < 0.05, **P < 0.01 compared with the IND group. | |
We next analyzed the related protein expression levels of the TFF/NAG-1 pathway. Immunohistochemical staining of the rat gastric mucosa illustrated that the TFF1 immunoreactivity of the IND group noticeably reduced compared to that of the control group (7.62 ± 1.27 vs. 31.73 ± 3.61 area percentage of immune-positive cells, P < 0.01). CSTT pretreatment significantly promoted TFF1 immunoreactivities by 44.11%, 116.15% and 234.10% (P < 0.05 or P < 0.01, respectively) (Fig. 9A). Meanwhile, qRT-PCR and western blot data showed that CSTT caused dose-dependent augments in TFF1 and TFF2 expression levels in gastric tissues compared to the IND group (P < 0.05 or P < 0.01, respectively). While no mRNA and protein expression of TFF3 was found in the gastric tissue of control rats, and low expression of TFF3 in the IND group was observed. Pretreatment with CSTT further enhanced their mRNA and protein expression levels by 32.61%, 71.07%, 133.90% and 46.80%, 98.68%, 170.46% compared with the IND group (P < 0.05 or P < 0.01, respectively) (Fig. 9C and E). Additionally, their downstream NAG-1 expression was also detected, Fig. 9D and E indicate that NAG-1 mRNA and protein expression levels of the IND group were noticeably elevated compared to those of the control group (P < 0.01, respectively). Conversely, CSTT pretreatment effectively reversed their expression levels compared with the IND group (P < 0.01, respectively).
After confirming that CSTT depressed apoptosis through the mitochondrion-mediated apoptotic pathway in vitro, we wanted to identify whether CSTT also had the same effect in vivo. As shown in Fig. 9E and F, the release of cytochrome C from the mitochondria into the cytosol was dramatically elevated, the protein expression levels of Bcl-2, Bcl-xl, pro-caspase-9, and pro-caspase-3 appreciably reduced, the Bax, Bad, cytosol cytochrome C, Apaf-1, cleaved-caspase-9, and cleaved-caspase-3 protein expression levels obviously increased, and the ratios of Bcl-2/Bax and Bcl-xl/Bad were as low as 0.514 ± 0.153 and 1.808 ± 0.331 in the IND group compared with the control group (11.148 ± 2.835, 11.130 ± 3.451) (P < 0.01, respectively); after pretreatment with CSTT, the abnormal release of cytochrome C, protein expression levels of Bcl-2, Bcl-xl, Bax, Bad, cytosol cytochrome C, Apaf-1, pro-caspase-9, pro-caspase-3, cleaved-caspase-9, and cleaved-caspase-3 and the ratios Bcl-2/Bax and Bcl-xl/Bad were evidently reversed compared with the IND group (P < 0.05 or P < 0.01, respectively). The aforementioned results suggested that miR-423-5p and its mediated downstream TFF/NAG-1 pathway were involved in the CSTT-suppressed mitochondrion-mediated apoptotic process in the IND-induced gastric damage rats, which was consistent with the results of in vitro experiments.
4. Discussion
Tujia nationality inhabitants in Chaenomeles speciosa production areas of Southwestern China should have rheumatic diseases and peptic ulcers for living in the damp environments and bingeing on spicy and pungent foods. Owing to the existence of the fruit derived products of Chaenomeles speciosa as their complementary foods or snacks, the customs described above make them rarely suffer from the two kinds of diseases. Enlightened by these, we investigated the structure–activity relationships and screened CSTT for gastroprotective activity,12,16–18 and found that CSTT was the most abundant ingredient and major active component in fruits of Chaenomeles speciosa, which was divided into oleanane-type triterpenoids (maslinic acid and oleanolic acid), lupane-type triterpenoids (betulin and betulinic acid) and ursane-type triterpenoids (ursolic acid and 3-O-acetyl ursolic acid). In order to further verify its gastroprotective function, as well as the mechanisms involved, we conducted the current research. In this study, our results demonstrated that CSTT had a protective effect on gastric injury, which was related to promoting IND-damaged GES-1 cell proliferation and migration, ameliorating the IND-damaged rat GBF at the ulcer margin, ulcer area and inhibition rate, increasing the amount of adhered gastric mucus, attenuating the volume and total acidity of the gastric effluents, augmenting the gastric pH, alleviating the hemorrhage, submucosal edema, epithelial cell loss and inflammatory cell infiltration of the gastric mucosa, upregulating TFF1, TFF2 and TFF3 mRNA and protein expression levels, Bcl-2, Bcl-xl, pro-caspase-3 and pro-caspase-9 protein expression levels, mitochondrial viability, concentration of cytochrome C in the mitochondria and ratios of Bcl-2/Bax and Bcl-xl/Bad, downregulating miR-423-5p mRNA expression, NAG-1 mRNA and protein expression levels, Bax, Bad, cytosol cytochrome C, Apaf-1, cleaved-caspase-3, and cleaved-caspase-9 protein expression levels and concentration of cytochrome C in the cytosol. Our present study demonstrated that the gastroprotective effect of CSTT was closely connected with depressing miR-423-5p expression and modulating the TFF/NAG-1 pathway, which in turn restrained mitochondrion-mediated apoptosis.
In recent years, miR-423-5p has been extensively studied in many human diseases such as diabetes, heart failure, and gastric and liver cancer.34 Liu and his colleagues5 verified that it was involved in the proliferation and invasion-related processes in gastric cancer, and more importantly it negatively regulated TFF1 expression. Interestingly, we first found that the miR-423-5p expression was obviously elevated and the mRNA and protein expression levels of TFF1 were dramatically reduced in the IND-damaged mouse gastric tissue.6 Meanwhile, we also confirmed that the high expression of miR-423-5p was closely related to the occurrence and prognosis of gastric ulcer patients.35 In the present study, the miR-423-5p mRNA expression levels in the IND-damaged GES-1 cells and rat gastric tissues showed an obvious elevating trend compared with the IND-damaged GES-1 cells and rats, and pretreatment with CSTT obviously restrained its expression. In addition, the overexpression of miR-423-5p resulting from miR-423-5p mimic transfection was observed to further aggravate the IND-induced GES-1 cell damage, which was associated with cell growth inhibition, mitochondrial viability reduction, the release of cytochrome C from mitochondria to cytosol and apoptosis increase. Conversely, the knockdown of miR-423-5p by transfecting with the miR-423-5p inhibitor or pretreatment with CSTT might significantly promote the cell proliferation and suppress the release of cytochrome C from mitochondria to cytosol and apoptosis; the miR-423-5p changes of the rat gastric tissues in the CSTT pretreated groups were also similar to it.
It is generally known that miRs function by regulating their target genes, which meant that further research on miR-423-5p target genes was necessary. To accomplish this, bioinformatics prediction analysis and luciferase reporter gene assay were used in the present study, and the data indicated that miR-423-5p was able to directly bind to the 3′-UTR region of TFF1 mRNA, and consequently, TFF1 could be identified as a target gene of miR-423-5p. TFF is an important mucin-associated protein involved in migration, restitution and reepithelialization of mucosal epithelial cells and the maintenance of mucosal barrier integrity.36 Normally, it exists in specific expression regions in the gastrointestinal tract. For example, TFF1 mainly localizes in the gastric surface epithelium, TFF2 is presents abundantly in the gastric neck cells and antral glands, and TFF3 is expressed in the intestinal goblet cells. They are usually cosecreted with glycoprotein mucins (MUC5AC, MUC6 and MUC2, respectively), constituting the first line of protection: the mucus–bicarbonate–phospholipid “barrier” which tenaciously adheres to the epithelial surfaces.8,37 Interestingly, when mucosal defense barrier integrity is disrupted, their regionally specific expression disappears at once, and the three of them are observed in the damaged gastric epithelial cells and gastric tissues. TFF1–3, then, act as “motogenes” to stimulate epithelial cell survival, protect cells from apoptosis and anoikis, and promote migration of viable cells at the transition zone toward the area of damage.37 For instance, application of recombinant TFF1 protected gastrointestinal tract cells from chemical-, Bad- or anchorage-dependent apoptosis accelerated the healing of the damaged gastric mucosa.38 In the current study, TFF1 mRNA and protein expression levels were significantly suppressed in IND-damaged GES-1 cells and rat gastric tissues, and their negative regulatory relationship with miR-423-5p was further confirmed by miR-423-5p mimic and miR-423-5p inhibitor experiments in IND-damaged GES-1 cells. Although TFF2 and TFF3 are not the target genes of miR-423-5p, they are equally important as TFF1 in the gastric epithelial repair and gastric mucosal wound healing. Aihara and Babyatsky et al.8,39 discovered that exogenous TFF2 or TFF3 resisted IND- and ethanol-induced gastric injury in rats, and rescued the delayed gastric epithelial repair in TFF2 KO mice. In the present study, we found that the TFF2 mRNA and protein expression levels were restrained and the TFF3 expression emerged in the IND-damaged GES-1 cells and rat gastric tissues. Interestingly, pretreatment with CSTT or miR-423-5p knockdown might effectively reverse TFF2 expression and further strengthen TFF3 expression. Based on the elucidated content above, we concluded that miR-423-5p negatively regulated its downstream TFF1 target, whilst TFF2 and TFF3 were not the direct target genes of miR-423-5p, and they were still indirectly affected by miR-423-5p. These findings indicated that the gastroprotective effect of CSTT on IND-damaged GES-1 cells and rats was closely connected with restraining miR-423-5p expression and elevating TFF1–3 expression.
It has been well-documented that NAG-1 plays a crucial role in the formation and development of ulcer.40 Although human and rodent epithelial cells show a low level of NAG-1 expression under normal physiological conditions, apoptotic mucosal epithelial cells for inflammation and antiinflammatory agents used presented relatively high levels of NAG-1.41 Colucci et al.11 showed that NAG-1 induction, followed by activating proapoptotic pathways, contributed to the delaying effects of NSAIDs on the gastric epithelial repair and gastric mucosal wound healing. Zhang et al.42 further indicated that an important element contributing to the activation of the proapoptotic pathway was that NAG-1 localized in mitochondria was induced, thus dissipating the mitochondrial membrane potential, leading to lipid peroxidation, subsequently triggering apoptosis. All of these indicated that the gastric injury healing was closely related to the activation of the apoptosis pathway mediated by NAG-1. In the current study, we found that the mRNA and protein expression levels of NAG-1 in IND-damaged GES-1 cells were significantly elevated, mitochondrial viabilities were remarkably suppressed, the release of cytochrome C from mitochondria to cytosol was increased and apoptosis was triggered. Conversely, pretreatment with CSTT and the miR-423-5p inhibitor or knockdown NAG-1 might efficiently restrain NAG-1 expression, augment the mitochondrial viabilities and depress the release of cytochrome C from mitochondria to cytosol and apoptosis; simultaneously, our in vivo experimental data also confirmed this fact. The above results indicated that CSTT attenuated NAG-1 expression and restrained IND-induced GES-1 cell apoptosis and rat gastric mucosal injury, thereby accelerating the gastric epithelial repair and gastric mucosal wound healing.
Mitochondria recognized as the bioenergetic, metabolic and apoptotic regulation centers are tightly modulated by a variety of external and internal signals, such as chemicals, ROS, NAG-1, Bcl-2 and caspase families, etc.43,44 The mitochondrial apoptosis is the primary cell-death type which arises after opening of a large number of mitochondrial permeabilization transition pores (mPTPs).45 In general, the process is tightly regulated by Bcl-2 family proteins, including antiapoptotic proteins (e.g., Bcl-2 and Bcl-xl) and proapoptotic proteins (such as Bax and Bad). Antiapoptotic proteins are usually distributed in the inner mitochondrial membrane, and proapoptotic proteins are primarily located on the outer mitochondrial membrane. Their relative balance between the antiapoptotic and proapoptotic proteins directly influences the physiological function of mitochondria.46,47 Normally, antiapoptotic proteins preserve mitochondrial membrane integrity and restrain the massive opening of mPTPs, which in turn suppress cytochrome C and other proapoptotic factors from the mitochondria releasing into the cytosol, while proapoptotic proteins reduce the mitochondrial membrane potential, increase mitochondrial outer membrane permeabilization and open mPTPs, resulting in the increased release of cytochrome C and other proapoptotic factors from the mitochondria into the cytosol, activate Apaf-1, forming an intermediary complex apoptosome together with cytochrome C, Apaf-1 and pro-caspase-9, and then activate caspace-3, triggering apoptosis.48 In our study, reduced mitochondrial viability, Bcl-2 and Bcl-xl protein expression levels, ratios of Bcl-2/Bax and Bcl-xl/Bad and concentration of cytochrome C in the mitochondria and elevated protein expression levels of Bax, Bad, and cytosol cytochrome C and concentration of cytochrome C in the cytosol were found in the IND-damaged, miR-423-5p mimic-transfected treated GES-1 cells and gastric tissues of IND-damaged rats. Pretreatment with CSTT, the miR-423-5p inhibitor or NAG-1 knockdown might successfully recover the mitochondrial viabilities, reverse the anomalous protein expression levels of Bcl-2, Bcl-xl, Bax, Bad, and cytosol cytochrome C and the concentrations of cytochrome C in the mitochondria and cytosol, and elevate the ratios Bcl-2/Bax and Bcl-xl/Bad. All the above aspects coincided with the amelioration of IND-damaged GES-1 cell proliferation, migration results and the IND-damaged rat GBF, ulcer area, inhibition rate, amount of adhered gastric mucus, volume and total acidity of the gastric effluents, gastric pH, and pathologic changes of gastric mucous tissue. The present results demonstrated that CSTT exerted a protective effect in restoring mitochondrial function. Caspases are another family known as aspartate-specific cysteine proteases, which also play important roles in modulating apoptosis. Normally, the number of intermediary complex apoptosome made up of cytochrome C, Apaf-1 and pro-caspase-9 is relatively small. Abnormal stimuli such as ROS, excessive hydrochloric acid, NSAID use, and so forth result in mitochondrial dysfunction and promote opening of mPTPs, bring out increased cytochrome C from the mitochondria into the cytosol, and boost the formation of intermediary complex apoptosome, which in turn cleaves pro-caspase-9 into cleaved-caspase-9 and pro-caspase-3 into cleaved-caspase-3 in turn, ultimately causing apoptosis.3,49 So the activation of caspase-9 and caspase-3 is considered as the another major cytopathologic hallmark of the apoptotic process. To illustrate this, we detected the Apaf-1, pro-caspase-9, pro-caspase-3, cleaved-caspase-9, and cleaved-caspase-3 protein expression levels. The results indicated that the pro-caspase-9 and pro-caspase-3 protein expression levels were dramatically depressed, and Apaf-1, cleaved-caspase-9, and cleaved-caspase-3 protein expression levels were significantly increased in the IND-damaged, miR-423-5p mimic-transfected GES-1 cells and IND-damaged rat gastric tissue. Conversely, CSTT, the miR-423-5p inhibitor or NAG-1 knockdown might efficiently ameliorate the aforementioned expression levels. These data indicated that the protective effect of CSTT on IND-damaged GES-1 cells and rats occurred through modulation of the mitochondrion-mediated apoptotic pathway.
5. Conclusion
Our results firstly demonstrated that CSTT possessed protective property against IND-induced gastric damage in vitro and in vivo, and its gastroprotective effects were closely related to restraining miR-423-5p expression and modulating the TFF/NAG-1 pathway, thereby reducing mitochondrion-mediated apoptosis (Fig. 10). These data provided a new insight for interpreting the potential mechanism of the gastroprotective effect of CSTT, and it showed promise in acting as a candidate agent to treat gastric injury.
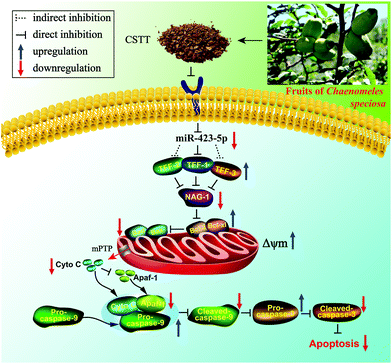 |
| Fig. 10 Potential molecular mechanism of the CSTT-mediated gastroprotective effect on IND-induced gastric damage. | |
Abbreviations
Apaf-1 | Apoptotic protease activating factor 1 |
CSTT | Total triterpenes from the fruits of Chaenomeles speciosa |
DAD | Diode array detector |
DMSO | Dimethyl sulfoxide |
FBS | Fetal bovine serum |
GAPDH | Glyceraldehyde-3-phosphate dehydrogenase |
t
R
| Retention time |
GBF | Gastric blood flow |
HPLC | High performance liquid chromatography |
IND | Indomethacin |
JC-1 | 5,5′,6,6′-Tetrachloro-1,1′,3,3′-tetraethylbenzimidazolcarbocyanine iodide |
KO | Knockout |
miR-NC | miR mimic negative control |
miRs | microRNAs |
mPTPs | Mitochondrial permeabilization transition pores |
MTT | 3-(4,5-Dimethylthiazol-2-yl)-2,5-diphenyltetrazolium bromide |
MUT | Mutated type |
NAG-1 | NSAID-activated gene-1 |
NSAIDs | Nonsteroidal antiinflammatory drugs |
OA | Oleanolic acid |
PBS | Phosphate buffered saline |
PI | Propidium iodide |
qRT-PCR | Quantitative real-time polymerase chain reaction |
ROS | Reactive oxygen species |
TFF1 | Trefoil factor family 1 |
WT | Wild type |
Conflicts of interest
The authors declare that there are no conflicts of interest.
Acknowledgements
This work was supported by the Research Foundation of Hubei Biological Enzyme Engineering Technology Research Center (JS2018-06), the Research Foundation of Yichang City Science and Technology Bureau (A18-302-a02), the Open Foundation of Hubei Key Laboratory of Tumor Microenvironment and Immunotherapy (2018KZL01) and the Master Dissertation Foundation of China Three Gorges University (2019SSPY159).
References
- P. O'Malley, Gastric ulcers and GERD: the new “plagues” of the 21st century update for the clinical nurse specialist, Clin. Nurse Spec., 2003, 17, 286–289 CrossRef PubMed.
- P. S. Tu, Y. T. Tung, W. T. Lee and G. C. Yen, Protective effect of camellia oil (Camellia oleifera Abel.) against ethanol-induced acute oxidative injury of the gastric mucosa in mice, J. Agric. Food Chem., 2017, 65, 4932–4941 CrossRef CAS PubMed.
- L. Chen, Y. Gao, L. H. Zhu, H. J. Song, L. L. Zhao, A. H. Liu, G. L. Zhang and G. Y. Shi, Establishment and characterization of a GES-1 human gastric epithelial cell line stably expressing miR-23a, Oncol. Lett., 2018, 16, 977–983 Search PubMed.
- R. Liu, C. Z. Zhang, Z. B. Hu, G. Li, C. Wang, C. H. Yang, D. Z. Huang, X. Chen, H. Y. Zhang, R. Zhuang, T. Deng, H. Liu, J. J. Yin, S. F. Wang, K. Zen, Y. Ba and C. Y. Zhang, A five microRNA signature identified from genome-wide serum microRNA expression profiling serves as a fingerprint for gastric cancer diagnosis, Eur. J. Cancer, 2011, 47, 784–791 CrossRef CAS PubMed.
- J. J. Liu, X. Wang, X. N. Yang, Y. P. Liu, Y. Shi, J. L. Ren and B. Y. S. Guleng, miRNA423-5p regulates cell proliferation and invasion by targeting trefoil factor 1 in gastric cancer cells, Cancer Lett., 2014, 347, 98–104 CrossRef CAS PubMed.
- H. L. Qin, Y. F. Zhang, X. Y. Wang, H. B. Tang, Q. Q. Lu, W. H. Yan, H. B. He and J. Z. Wang, Effect of ethyl acetate extract from Chaenomeles speciosa (Sweet) Nakaion on the expression of miR-423-5p, TFF1 and p53 in acute gastric ulcer mice, Pharmacol. Clin. Chin. Mater. Med., 2016, 32, 80–82 Search PubMed.
- M. Busch and N. Dünker, Trefoil factor family peptides-friends or foes?, Biomol. Concepts, 2015, 6, 343–359 CAS.
- E. Aihara, K. A. Engevik and M. H. Montrose, Trefoil factor peptides and gastrointestinal function, Annu. Rev. Physiol., 2017, 79, 357–380 CrossRef CAS PubMed.
- W. Hoffmann, Trefoil factors TFF (trefoil factor family) peptide-triggered signals promoting mucosal restitution, Cell. Mol. Life Sci., 2005, 62, 2932–2938 CrossRef CAS PubMed.
- J. L. Liggett, X. B. Zhang, T. E. Eling and S. J. Baeka, Anti-tumor activity of non-steroidal anti-inflammatory drugs: cyclooxygenase-independent targets, Cancer Lett., 2014, 346, 217–224 CrossRef CAS PubMed.
- R. Colucci, L. Antonioli, N. Bernardini, C. Ippolito, C. Segnani, O. Awwad, M. Tuccori, C. Blandizzi, C. Scarpignato and M. Fornai, Nonsteroidal anti-inflammatory drug-activated gene-1 plays a role in the impairing effects of cyclooxygenase inhibitors on gastric ulcer healing, J. Pharmacol. Exp. Ther., 2012, 342, 140–149 CrossRef CAS PubMed.
- H. B. He, Y. F. Zhang, X. M. Li, X. Q. Li, T. Luo, J. H. Zhang, Q. Zhou and S. J. Cai, Clinical study of total triterpenoids of Chaenomeles speciosa (Sweet) Nakai combined with omeprazole capsule in treatment of upper gastrointestinal bleeding caused by nonsteroidal anti-inflammatory drugs, Pharmacol. Clin. Chin. Mater. Med., 2017, 33, 209–212 Search PubMed.
- S. Y. Zhang, L. Y. Han, H. Zhang and H. L. Xin, Chaenomeles speciosa: A review of chemistry and pharmacology, Biomed. Rep., 2014, 2, 12–18 CrossRef CAS PubMed.
-
China Pharmacopoeia Committee, Chinese Pharmacopoeia (I), China Medical Science and Technology Press, Beijing, 2015, p. 61 Search PubMed.
- J. Miao, C. C. Zhao, X. Li, X. T. Chen, X. H. Mao, H. H. Huang, T. T. Wang and W. Y. Gao, Chemical composition and bioactivities of two common chaenomeles fruits in China: Chaenomeles speciosa and Chaenomeles sinensis, J. Food Sci., 2016, 81, H2049–H2058 CrossRef CAS PubMed.
- H. B. He, Y. F. Zhang, X. M. Li, X. Q. Li, H. L. Qin, C. X. Liu, H. B. Tang, J. Z. Wang and K. Zou, Effect of triterpenoids from Chaenomeles speciosa (Sweet) Nakai on gastric acid secretion and gastric mucosal barrier function in indomethacin induced mice, Biotic Resour., 2017, 39, 211–216 Search PubMed.
- J. H. Zhang, X. M. Li, M. Q. Shi, X. J. Xiong, X. Q. Li, M. L. Feng, H. Y. Xu, W. Y. Yang, H. L. Qin and T. Luo, Effects of total triterpenoids of Chaenomeles speciosa combined with mesalazine on the clinical efficacy, inflammatory and intestinal barrier protective factors of ulcerative colitis, Chin. J. Clin. Pharmacol. Ther., 2018, 23, 1165–1173 Search PubMed.
- H. B. He, X. Q. Li, X. M. Li, Y. M. He, H. L. Qin, Y. F. Zhang, X. J. Xiong, J. Z. Wang and K. Zou, Therapeutic effect of total triterpenoids of Chaenomeles speciosa combined with omeprazole on gastric ulcer induced by indomethacin in rats, China J. Chin. Mater. Med., 2019, 44, 2338–2247 Search PubMed.
- M. Q. Shi, H. L. Qin, Y. F. Zhang, Y. Liu, W. Deng, W. Y. Yang, J. Z. Wang and X. C. Lu, Effect of total triterpene from Chaenomeles speciosa (sweet) Nakaion on cytokines in LPS-stimulated RAW264.7 cells, Pharmacol. Clin. Chin. Mater. Med., 2016, 32, 76–80 Search PubMed.
- S. J. Baek, K. S. Kim, J. B. Nixon, L. C. Wilson and T. E. Eling, Cyclooxygenase inhibitors regulate the expression of a TGF-beta superfamily member that has proapoptotic and antitumorigenic activities, Mol. Pharmacol., 2001, 59, 901–908 CrossRef CAS.
- H. L. Qin, Y. F. Zhang, H. B. He, H. B. Tang, C. X. Liu, J. Z. Wang, W. H. Yan and H. J. Luo, Anti-apoptotic effect of triterpene from Chaenomeles speciosa (Sweet) Nakaion in indomethacin induced RGM-1 cells, Chin. J. Clin. Pharmacol. Ther., 2016, 21, 1347–1353 Search PubMed.
- X. X. Chen, X. Wu, W. Ouyang, M. Gu, Z. L. Gao, M. Y. Song, Y. J. Chen, Y. Y. Lin, Y. Cao and H. Xiao, Novel ent-kaurane diterpenoid from Rubus corchorifolius L. f. inhibits human colon cancer cell growth via inducing cell cycle arrest and apoptosis, J. Agric. Food Chem., 2017, 65, 1566–1573 CrossRef CAS PubMed.
- F. Deng, S. Wang, L. Zhang, X. Xie, S. Cai, H. Li, G. L. Xie, H. L. Miao, C. Yang, X. Liu and Z. Xia, Propofol through upregulating Caveolin-3 attenuates post-hypoxic mitochondrial damage and cell death in H9C2 cardiomyocytes during hyperglycemia, Cell. Physiol. Biochem., 2017, 44, 279–292 CrossRef PubMed.
- C. D. Luo, H. B. Chen, Y. F. Wang, G. S. Lin, C. L. Li, L. H. Tan, Z. R. Su, X. P. Lai, J. H. Xie and H. F. Zeng, Protective effect of coptisine free base on indomethacin-induced gastric ulcers in rats: Characterization of potential molecular mechanisms, Life Sci., 2018, 193, 47–56 CrossRef CAS PubMed.
- S. M. Dhiyaaldeen, Z. A. Amin, P. H. Darvish, I. F. Mustafa, M. M. Jamil, E. Rouhollahi and M. A. Abdulla, Protective effects of (1-(4-hydroxy-phenyl)-3-mtolyl-propenone chalcone in indomethacin induced gastric erosive damage in rats, BMC Vet. Res., 2014, 10, 961, DOI:10.1186/s12917-014-0303-7.
- M. Magierowski, K. Magierowska, M. Hubalewska-Mazgaj, Z. Sliwowski, G. Ginter, R. Pajdo, A. Chmura, S. Kwiecien and T. Brzozowski, Carbon monoxide released from its pharmacological donor, tricarbonyldichlororuthenium(II) dimer, accelerates the healing of pre-existing gastric ulcers, Br. J. Pharmacol., 2017, 174, 3654–3668 CrossRef CAS PubMed.
- L. Laine and W. M. Weinstein, Histology of alcoholic hemorrhagic “gastritis”:
a prospective evaluation, Gastroenterology, 1988, 94, 1254–1262 CrossRef CAS.
- Y. T. Cheng, S. L. Wu, C. Y. Ho, S. M. Huang, C. L. Cheng and G. C. Yen, Beneficial effects of Camellia oil (Camellia oleifera Abel.) on ketoprofen-induced gastrointestinal mucosal damage through upregulation of HO-1 and VEGF, J. Agric. Food Chem., 2014, 62, 642–650 CrossRef CAS PubMed.
- H. B. He, X. M. Li, H. L. Yu, S. Zhu, Y. M. He, K. Komatsu, D. Y. Guo, X. Q. Li, J. Z. Wang, H. J. Luo, D. X. Xu and K. Zou, Gastroprotective effect of araloside A on ethanol and aspirin-induced gastric ulcer in mice: involvement of H+/K+-ATPase and mitochondrial-mediated signaling pathway, J. Nat. Med., 2019, 73, 339–352 CrossRef CAS PubMed.
- Y. Niv and M. Banić, Gastric barrier function and toxic damage, Dig. Dis., 2014, 32, 235–242 CrossRef PubMed.
- J. M. Li, K. L. Li, J. L. Gao, X. F. Guo, M. Q. Lu, Z. H. Li and D. Li, Maternal exposure to an n-3 polyunsaturated fatty acid diet decreases mammary cancer risk of female offspring in adulthood, Food Funct., 2018, 9, 5768–5777 RSC.
- S. W. Hu, J. H. Wang, J. F. Wang, H. C. Yang, S. J. Li, W. Jiang, Y. Liu and J. L. Li, Long-chain bases from sea cucumber inhibits renal fibrosis and apoptosis in type 2 diabetic mice, J. Funct. Foods, 2018, 40, 760–768 CrossRef CAS.
- H. Yandrapu and J. Sarosiek, Protective factors of the gastric and duodenal mucosa: an overview, Curr. Gastroenterol. Rep., 2015, 17, 1–8 CrossRef PubMed.
- J. Ge, J. Y. Zhu, B. Xia, H. G. Cao, Y. Peng, X. Li, T. Y. Yu, G. Y. Chu, G. S. Yang and X. Shi, miR-423-5p inhibits myoblast proliferation and differentiation by targeting Sufu, J. Cell. Biochem., 2018, 119, 7610–7620 CrossRef CAS PubMed.
- X. M. Li, X. Q. Li, H. B. He, Y. F. Zhang, H. Y. Xu, J. H. Zhang, J. Z. Wang and T. Luo, Research progress of miR-423-5p, TFFs and NAG-1 in anti-NASIDs induced gastric mucosal injury, Chin. J. Clin. Pharmacol. Ther., 2018, 24, 1068–1074 Search PubMed.
- C. Palileo and J. D. Kaunitz, Gastrointestinal defense mechanisms, Curr. Opin. Gastroenterol., 2011, 27, 543–548 CrossRef CAS PubMed.
- L. C. Bi and J. D. Kaunitz, Gastroduodenal mucosal defense: an integrated protective response, Curr. Opin. Gastroenterol., 2013, 19, 526–532 CrossRef PubMed.
- X. Yio, M. Diamond, J. Y. Zhang, H. Weinstein, L. H. Wang, L. Werther and S. Itzkowitz, Trefoil factor family-1 mutations enhance gastric cancer cell invasion through distinct signaling pathways, Gastroenterology, 2006, 130, 1696–1706 CrossRef CAS PubMed.
- M. W. Babyatsky, M. DeBeaumont, L. Thim and D. K. Podolsky, Oral trefoil peptides protect against ethanol- and indomethacin-induced gastric injury in rats, Gastroenterology, 1996, 110, 489–497 CrossRef CAS PubMed.
- Y. Moon, NSAID-activated gene 1 and its implications for mucosal integrity and intervention beyond NSAIDs, Pharmacol. Res., 2017, 121, 122–128 CrossRef CAS.
- K. S. Kim, S. J. Baek, G. P. Flake, C. D. Loftin, B. F. Calvo and T. E. Eling, Expression and regulation of nonsteroidal anti-inflammatory drug-activated gene (NAG-1) in human and mouse tissue, Gastroenterology, 2002, 122, 1388–1398 CrossRef CAS.
- X. B. Zhang, Y. Kang, T. X. Huo, R. Tao, X. P. Wang, Z. Y. Li, Q. L. Guo and L. Zhao, GL-V9 induced upregulation and mitochondrial localization of NAG-1 associates with ROS generation and cell death in hepatocellular carcinoma cells, Free Radicals Biol. Med., 2017, 112, 49–59 CrossRef CAS PubMed.
- H. F. Tsai and P. N. Hsu, Modulation of tumor necrosis factor-related apoptosis-inducing ligand (TRAIL)-mediated apoptosis by Helicobacter pylori in immune pathogenesis of gastric mucosal damage, J. Microbiol., Immunol. Infect., 2017, 50, 4–9 CrossRef CAS PubMed.
- Q. Qin, Y. W. Lin, X. Y. Zheng, H. Chen, Q. Q. Mao, K. Yang, S. J. Huang and Z. Y. Zhao, RNAa-mediated overexpression of WT1 induces apoptosis in HepG2 cells, World J. Surg. Oncol., 2012, 10, 1–8 CrossRef PubMed.
- H. S. Xu, Y. F. Li, B. Han, Z. X. Li, B. Wang, P. Jiang, J. Zhang, W. Y. Ma, D. Q. Zhou, X. G. Li and X. L. Ye, Anti-breast-cancer activity exerted by β-sitosterol-D-glucoside from sweet potato via upregulation of microRNA-10a and via the PI3K-Akt signaling pathway, J. Agric. Food Chem., 2018, 66, 9704–9718 CrossRef CAS PubMed.
- B. Han, P. Jiang, Z. X. Li, Y. Yu, T. Huang, X. L. Ye and X. G. Li, Coptisine-induced apoptosis in human colon cancer cells (HCT-116) is mediated by PI3K/Akt and mitochondrial-associated apoptotic pathway, Phytomedicine, 2018, 48, 152–160 CrossRef CAS PubMed.
- J. H. Wang, S. W. Hu, J. F. Wang, S. J. Li and W. Jiang, Fucoidan from Acaudina molpadioides protects pancreatic islet against cell apoptosis via inhibition of inflammation in type 2 diabetic mice, Food Sci. Biotechnol., 2016, 25, 293–300 CrossRef CAS PubMed.
- R. J. Youle and A. Strasser, The BCL-2 protein family: opposing activities that mediate cell death, Nat. Rev. Mol. Cell Biol., 2008, 9, 47–59 CrossRef CAS PubMed.
- J. Z. Wang, A. L. Wang, H. B. He, X. X. She, Y. M. He, S. Li, L. Q. Liu, T. Luo, N. Y. Huang, H. J. Luo and K. Zou, Trametenolic acid B protects against cerebral ischemia and reperfusion injury through modulation of microRNA-10a and PI3K/Akt/mTOR signaling pathways, Biomed. Pharmacother., 2019, 112, 1–15 Search PubMed.
|
This journal is © The Royal Society of Chemistry 2020 |