DOI:
10.1039/C9FO02296A
(Paper)
Food Funct., 2020,
11, 680-688
Enhancement of the anti-inflammatory properties of grape pomace treated by Trametes versicolor
Received
1st October 2019
, Accepted 18th December 2019
First published on 19th December 2019
Abstract
The application of solid-state fermentation for the production of value-added products from the agro- and food-industry residues has been recently investigated greatly. The white-rot basidiomycete Trametes versicolor is a widely used fungi for the degradation lignocellulosic material in solid-state conditions. Grape pomace constitutes the major by-product of Vitis vinifera L. and is a source of compounds with recognized health benefits. In this study, a process for treating grape pomace with Trametes versicolor for 15 days under solid-state conditions was developed, and the phenolic profile and anti-inflammatory potential of the grape pomace extracts before and after treatment was studied. The anti-inflammatory potential of the grape pomace extracts was studied via tests based on the inhibition of 5-lipoxygenase and hyaluronidase, two key enzymes in inflammatory processes. A total of 24 phenolic compounds were identified and quantified by HPLC methods. With the exception of anthocyanins, an increase in phenolic acids, flavan-3-ols and the flavonol rutin was observed after a treatment period of 1–4 days with T. versicolor. Moreover, the increase in the phenolic content was accompanied by an enhancement in the anti-inflammatory activity of the grape pomace extracts, which was confirmed by the strong correlation between them. This is the first study providing evidence of the benefits of the application of fungal-based solid-state fermentation as an environmentally friendly process for the enhancement of the phenolic composition and anti-inflammatory potential of grape pomace, increasing the possibility of profiting from the great waste produced by the grape-processing industry.
Introduction
In the last two decades, sustainable food production and valorisation of by-products have become imperative in the modern agriculture and food industry.1 Simultaneously, the awareness about the relation between nutrition enriched with bioactive compounds and chronic disease prevention has increased.2 Nowadays, it is broadly accepted that inflammation plays a pivotal role in the development of different degenerative processes (neurodegenerative and cardiovascular diseases, diabetes, cancer, asthma, rheumatoid arthritis, etc.) in the human body.3 Thus, the anti-inflammatory activity of natural compounds can be manifested by their capacity to inhibit enzymes involved in inflammatory processes such as hyaluronidase and lipoxygenases.3 Grape pomace (GP) is a source of bioactive phenolic compounds including flavonoids (flavan-3-ols, anthocyanins and flavonols) and non-flavonoids (phenolic acids) among the compounds known to have great benefits for health, which are related to anti-inflammatory, antimicrobial, antiallergenic, antithrombotic, cardioprotective and vasodilatory effects.4 However, the recovery and utilization of phenolic compounds strongly depend on the isolation procedures, material properties, and pretreatment process of GP. Also, there is no standardized method considering the great structural diversity of polyphenolic compounds.5 GP, as a predominantly lignocellulosic material (lignin: 28.7–42.2%, cellulose: 9.2–14.5%; hemicellulose: 4.0–10.3%),2,6,7 contains a significant content of low-soluble polyphenols (e.g. highly polymerized condensed tannins bonded in complexes) incorporated in its complex lignin structure. The extraction of low-soluble polyphenolic compounds requires additional degradation processes, such as the use of acid hydrolysis or commercial enzymes. These processes increase production costs and/or are not environmentally friendly.8,9
Thus, the development of bioprocesses for the production/extraction of bioactive substances, which enable the production of high quality extracts in an environmentally friendly way, has been recently investigated greatly. Solid-state fermentation (SSF) is a bioprocess in which filamentous fungi are used for the production of numerous high-value products, including bioactive polyphenolic compounds, using agro-food waste as substrates.10 This process is based on the cultivation of an appropriate microorganism in moist solid materials in the absence of free water, which may be carriers and substrates for microorganism growth.11,12 During their growth, these microorganisms synthesize enzymes that catalyse the degradation of the complex polymeric substrate structure, leading to the “release” of phenolic compounds trapped in the lignin fraction, thus they become more available for extraction.
Several studies regarding the production of phenolic compounds from different agro- and food-industry wastes using SSF have been reported this far. For instance, Buenrostro-Figueroa et al.13 reported the treatment of fig (Ficus carica L.) by-products with Rhizopus oryzae, Trichoderma sp. and Aspergillus niger. Cultivation of chokeberry with Rhizopus oligosporus and Aspergillus niger was investigated by Dulf et al.14 However, the only work reporting the cultivation of microorganisms on grape waste was published by Martínez-Ávila et al.15 In their work, they reported the application of Aspergillus and Penicillium strains for grape waste treatment with the aim to improve the production of phenolic antioxidant compounds. Furthermore, it has been proven that the biological treatment of materials in SSF conditions increases the bioaccessibility of polyphenols in the digestive system.16
Trametes versicolor (known as Coriolus versicolor, Polyporus versicolor, “Yun-Zhi”, “Kawaratake” and “Turkey tail”) is a white-rot fungus, which belongs to the Basidiomycota division. In nature it inhabits different types of trees but mostly grows on rotten trees and plant material. T. versicolor is a widely studied fungus due to the high interest in the use of its enzymatic complex systems and its production of polysaccharopeptides with important biological activities (e.g. anticancer activity). Because of its health benefits, it has been used in traditional Chinese medicine for centuries. T. versicolor produces extracellular lignocellulolytic enzymes such as laccases, manganese and lignin peroxidases. Complex enzyme systems have a wide range of applications, and the use of laccase was reported for the wine and beer industries, pulp and paper and textile industries and for bioremediation.17–19
In recent years, our group has shown that T. versicolor can to biotransform agro-food substrates into high-value compounds such as enzymes and phenolic acids8,20 and improve biogas production.21 Based on these findings, it is supposed that grape pomace, due to its chemical composition, may be a good substrate for the growth of T. versicolor. The main purpose of this work was to enhance the production of phenolic compounds and to improve the anti-inflammatory potential of grape pomace after solid-state treatment with T. versicolor.
Materials and methods
Standards and reagents
Ethanol (analytical grade) and hydrochloric acid were acquired from Fisher Scientific (Waltham, USA). Formic acid was acquired from LabKem (Barcelona, Spain), and methanol LiChrosolv® and acetonitrile LiChrosolv® were from Merck (Darmstadt, Germany). Quercetin (Q), p-hydroxybenzoic acid (p-HBA), p-coumaric acid (p-CuA), trans-resveratrol (t-RAS), linoleic acid, hyaluronic acid (HA) sodium salt, hyaluronidase (HAase), lipoxygenase from Glycine max (L.) Merr. (type V-S; EC 1.13.11.12), 1-butanol, sodium hydroxide, bovine serum albumin (BSA), and p-dimethylaminobenzaldehyde (DMAB) were obtained from Sigma-Aldrich (St Louis, MO, USA). Syringic acid (SA), ferulic acid (FA), gallic acid (GA), vanillic acid (VA), ellagic acid (EA), ((+) catechin (CT), (−)-epicatechin (ECT), (−)-epicatechin gallate (ECG), (−) gallocatechin gallate (GCG), rutin (Rut), delphinidin-3-O-glucoside, cyanidin-3-O-glucoside, petunidin-3-O-glucoside; peonidin 3-O-glucoside; malvidin-3-O-glucoside; peonidin, peonidin-3-O-p-coumaroylglucoside, malvidin-3-O-p-coumaroylglucoside), procyanidin B1 (B1) and procyanidin B2 (B2) were obtained from Extrasynthese (Genay, France). Deionised water was obtained from a Milli-Q water purification system (Millipore, Bedford, MA, USA).
Grape pomace samples and microorganism
The GP sample represents the residue from red grape (mixture of three varieties: Cabernet Sauvingnon, Cabernet Frank and Merlot) processing. GP was obtained from a local winery from East Croatia (harvest October, 2016). After collection, GP was stored at −20 °C prior to use for the biological pretreatment of T. versicolor. T. versicolor TV-6 (MZKI, Ljubljana, Slovenia) was cultivated on PDA medium for 7 days at 27 °C.
Biological pretreatment of GP by T. versicolor
50 g of defreezed and coarse ground grape pomace was mixed with 30 mL of distilled water in 720 mL laboratory jars, autoclaved (121 °C per 20 min) and cooled overnight. A total of sixty laboratory jars were prepared, as described above, and fifty four of them were inoculated with 5 mycelial plugs (diameter 1 cm) and suspended in 10 mL of sterile distilled water and six jars were used for the biologically untreated samples. After inoculation, the biological treatments were performed at 27 °C for 1–6, 9, 12 and 15 days in an incubator with the air fan set at 10% (KB 115, BINDER GmbH, Germany). Biological treatment was stopped in six jars after the first day by sterilization (121 °C per 20 min), in a further six jars after the second day and so on. After the treatment, all the samples were dried at ambient temperature for 48 h, then milled using an Ultra-Centrifugal Mill (Retsch ZM200, Germany) to 1 mm particle size and stored at +4 °C until extraction. The biologically untreated sample was prepared using the same procedure but without inoculation by microorganism and classified as sample at 0 day.
Preparation of GP extracts for phenolic compound determination
Liquid GP extracts preparation.
Milled grape pomace (1 g) was extracted with 40 mL of ethanol
:
water (1
:
1) in sealed flasks at 80 °C. Extractions were performed in a water bath (Julabo, SW-23, Germany) by shaking (200 rpm) for 120 min, in duplicate. After extraction, the extraction suspension was centrifuged (Z 326 K, Hermle Labortechnik GmbH) at 10
000g for 10 min. The supernatant (liquid extract) was decanted and used for the determination of phenolic acids, flavan-3-ols, flavonols and trans-resveratrol content.
Dry GP extract preparation.
The liquid GP extracts prepared were evaporated at reduced pressure (Rotavapor Buchi B-215) and freeze-dried in a Virtis SP Scientific Sentry 2.0 apparatus (Gardiner, NY, USA). The lyophilized extracts were kept in a desiccator, in the dark, until analysis of the anthocyanins content and anti-inflammatory activities.
Phenolic compound determination
Analysis of phenolic acids, flavanols, flavonols and stilbenes.
The identification and quantification of phenolic acids, flavan-3-ols, flavonols and trans-resveratrol (stilbene) in the GP liquid extracts was performed via ultra-high liquid performance chromatography (UHPLC) according to a previously published method.8 Separations were carried out using a reverse-phase Kinetex® C18 core–shell column (100 × 4.6 mm, 2.6 μm; Phenomenex). Spectral data from all peaks were collected in the range of 190–370 nm and chromatograms were recorded at different wavelengths (254–370 nm) according to the absorption maxima of the analysed compounds.
The data were processed using the LabSolutions 5.87 software. Phenolic compounds were identified by comparing their retention times and UV-Vis spectra with that of authentic standards analysed under the same chromatographic conditions. Quantification was performed using the external standard method. Flavan-3-ols were determined at 273–279 nm, hydroxybenzoic acids at 254–280 nm, hydroxycinnamic acids at 307–320 nm, trans-resveratrol at 305 nm and flavonols at 355–370 nm. All compounds were determined individually.
Anthocyanins analysis.
An appropriate amount of each dry GP extract (75 mg) was dissolved in 1 mL of acidified ethanol
:
water (1
:
1) (pH 3), membrane-filtered (0.45 μm) and analyzed by HPLC-DAD. Anthocyanins were analyzed on an analytical HPLC unit (Gilson) using a Spherisorb ODS2 column (25.0 cm × 0.46 cm; 5 μm particle size Waters, Milford, MA, USA). The injection volume was 20 μL. The mobile phase consisted of water/formic acid/acetonitrile (87
:
10
:
3, v/v/v; eluent A) and water/formic acid/acetonitrile (40
:
10
:
50, v/v/v; eluent B) using a gradient program as follows: from 10% to 25% B (10 min), from 25% to 31% B (5 min), from 31 to 40% (5 min), from 40% to 50% B (10 min), from 50% to 100% B (10 min), and from 100% to 10% B (5 min). The flow rate was 0.8 mL min−1. Detection was performed at 500 nm. Compounds were identified by comparing their chromatographic behaviour and UV spectra with that of authentic standards and the literature.22,23 Quantification was performed using the external standard method.
Petunidin-3-O-glucoside and petunidin-3-O-p-coumaroylglucoside were quantified as petunidin; and peonidin-3-O-p-coumaroylglucoside and malvidin-3-O-p-coumaroylglucoside were determined as peonidin-3-O-glucoside and malvidin-3-O-glucoside, respectively. The other compounds were determined as themselves.
Anti-inflammatory potential of grape pomace extracts
The anti-inflammatory potential of the GP extracts was estimated based on the ability of the extract to inhibit two enzymes involved in the inflammatory process, 5-lipoxygenase (5-LOX) and hyaluronidase (HAase).
5-LOX inhibition assay.
The inhibitory effect on 5-LOX was assessed in 96-well plates at room temperature following the oxidation of linoleic acid to 13-hydroperoxy linoleic acid at 234 nm according to the procedure described by Pereira et al.3 20 μL of each dissolved dry GP extract was mixed with 200 μL of phosphate buffer (pH 9) and 20 μL of soybean lipoxygenase 100 U (blank). Then, 20 μL linoleic acid (4.18 mM in ethanol) was added and the reaction was followed for 3 min on a Multiscan plate reader (Multiscan Go, ThermoScientific). 5-LOX inhibitory activity (%) = 100 × (1 − [mean V of sample/mean V of control]), where mean V corresponds to the mean velocity of the kinetic well analysis. The percentage of inhibitory activity was plotted against the sample concentration to obtain the half maximal inhibitory concentration of grape pomace extracts (IC50) (plots not shown). Four independent experiments were performed in triplicate. Quercetin was used as the positive control.
HAase inhibition assay.
The inhibitory effect on HAase was evaluated according the procedure described by Muckenschnabela et al.24 with some modifications. Briefly, a stock solution of 5 mg mL−1 HA was prepared in water and stored at 4 °C. The HA stock solution (25 μL) and 50 μL of buffer (0.2 M sodium formate, 0.1 M NaCl and 0.2 mg mL−1 BSA, pH adjusted to 3.68 with formic acid) were added to 100 μL of water. Sample serial dilutions were prepared in water and 25 μL of each dilution was added to each reaction tube. The reaction was started by the addition of 25 μL of HAase (900 U mL−1) prepared in 0.9% NaCl. The reaction mixture was incubated at 37 °C for 30 min. The enzymatic reaction was stopped by adding 25 μL of an alkaline solution consisting of di-sodium tetraborate (0.8 M in water) and subsequent heating for 3 min in a boiling water bath. The test tubes were cooled at room temperature and 375 μL of DMAB solution was added (2 g of DMAB dissolved in a mixture of 2.5 mL of 10 N HCl and 17.5 mL of glacial acetic acid and further diluted 1
:
2 with glacial acetic acid immediately before use). The tubes were incubated at 37 °C for 20 min. The absorbance of the colored product was measured at 560 nm on a Multiscan plate reader (Multiscan Go, ThermoScientific). HAase inhibitory activity (%) = 100 × (1 − [absorbance of sample/absorbance of control]) was plotted against the sample concentration to obtain the IC50 (plots not shown). Three independent assays were performed in triplicate.
Data analysis and statistics
The content of phenolic compounds was calculated from three determinations, and the results are shown as mean (±SD).
The anti-inflammatory potential results are expressed as the mean of at least three independent experiments, performed in triplicate. IC50 values were calculated using the GraphPad Prism 6 software (San Diego, CA, USA) and expressed as μg of dry extract per mL. All the results are presented as mean value ± standard error of mean (SEM). The correlation coefficient (R) to determine the relationship between anti-inflammatory activity and content of phenolic compounds was calculated at the 95% confidence level (p < 0.05) using the Statistica 13 software (Stat Soft Inc., Tulsa, USA).
Results and discussion
GP phenolic composition before and after biological treatment with T. versicolor
GP is considered as a potential value-added waste by-product due to the numerous functional actions of its phytochemical constituents. SSF with T. versicolor was investigated with the purpose to enhance the extractability of phenolic compounds trapped in the complex lignocellulosic matrix of GP and to enhance the anti-inflammatory efficiency of the obtained extracts. The content of phenolic compounds of the extracts from biologically treated GP was compared with that determined in the extracts prepared from GP prior to SSF (initial sample or day 0). One of drawbacks of the SSF process is the co-extraction of a variety of different compounds, not only desirable phenolic compounds. These compounds can interfere with the commonly reported colorimetric methods for the determination of total phenolic compounds and can cause overestimation of the real phenolic content. Therefore, analysis of the profile of individual phenolic compounds as performed herein can more clearly demonstrate the influence of SSF on the liberation of phenolic compounds. Among the analyzed phenolic acids, flavanols, flavonols and stilbene phenolic compounds (Fig. 1–3) in the biologically untreated GP extracts (day 0), the flavan-3-ols CT (3.5 × 103 mg kgdb−1), ECT (1.7 × 103 mg kgdb−1), GCG (0.9 × 103 mg kgdb−1), ECG (0.7 × 103 mg kgdb−1) and procyanidins B1 (1.1 × 103 mg kgdb−1) and B2 (0.5 × 103 mg kgdb−1) were the compounds presented in the greatest amount.
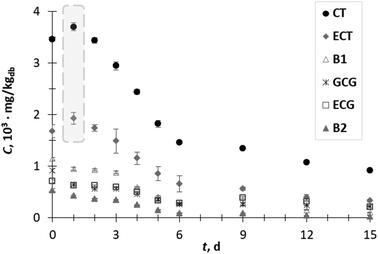 |
| Fig. 1 Content (C) of catechin (CT), epicatechin (ECT), procyanidin B1 (B1), gallocatechin gallate (GCG), epicatechin gallate (ECG) and procyanidin B2 (B2) of grape pomace extracts obtained during 15 days (t) of biological treatment with T. versicolor. | |
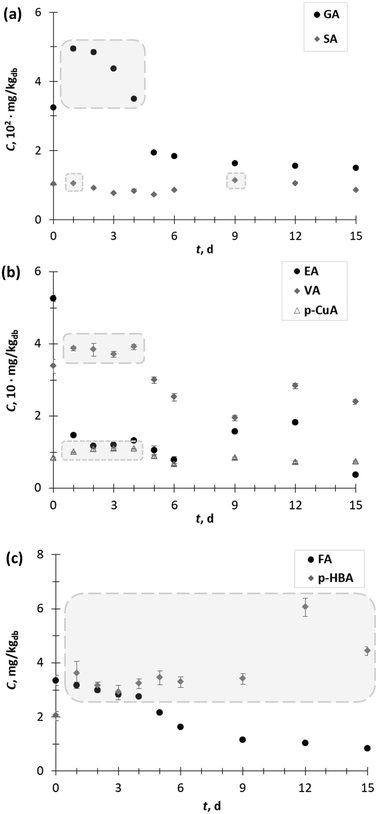 |
| Fig. 2 Content (C) of (a) gallic acid (GA) and syringic acid (SA), (b) ellagic acid (EA), vanillic acid (VA) and p-coumaric acid (p-CuA), and (c) ferulic acid (FA) and p-hydroxybenzoic acid (p-HBA) of grape pomace extracts obtained during 15 days (t) of biological treatment with T. versicolor. | |
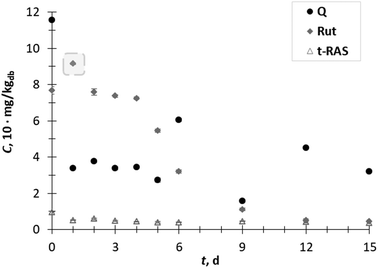 |
| Fig. 3 Content (C) of the flavonols quercetin (Q) and rutin (Rut), and of the stilbene trans-resveratrol (t-RAS) in the GP extracts obtained during 15 days (t) of biological treatment with T. versicolor. | |
Fig. 1 illustrates the changes in the flavan-3-ols content during 15 days of SSF process. The content of CT and ECT in the GP extract after day 1 of fermentation increased by 1.1-fold to 3.7 × 103 mg kgdb−1 and 1.9 × 103 mg kgdb−1, respectively. Their concentration started to decrease from day 2 up to the end of the process. The increment in the content of phenolic compounds during the SSF process can be explained by their release due to the structural breakdown of the cell wall associated with fungal growth or due to the action of various hydrolytic and ligninolytic enzymes.1,9,13,14 On the other hand, the decrease in the phenolic contents (from day 2 onwards) can be explained by phenolic degradation and/or phenolic polymerization catalyzed by the fungal enzymatic system.1,25 The content of other flavan-3-ols such as B1, GCG, ECG and B2 decreased during fermentation. The short-term increment in the CT and ECT content may be the result of the degradation procyanidins by fungus since procyanidins B1 and B2 consist of two flavanol units (catechin or epicatechin) linked by a C4–C8 linkage.26 Kendari et al.27 noticed that the increase in the catechin content in cocoa powder could be the result of the degradation of procyanidin during the vacuum roasting of cocoa.
Furthermore, among the hydroxycinnamic (p-CuA, FA) and hydroxybenzoic acids (GA, SA, p HBA, and EA), GA (325.07 mg kgdb−1) was the major phenolic acid in the GP extract obtained prior to SSF, followed by SA (104.25 mg kgdb−1), EA (52.6 mg kgdb−1), VA (33.8 mg kgdb−1), p-CuA (8.4 mg kgdb−1), FA (3.4 mg kgdb−1) and p-HBA (2.0 mg kgdb−1) (Fig. 2a and c).
The content of GA (Fig. 2a) increased during the first 4 days of SSF from 1.5-fold on the 1st day (494.6 mg kgdb−1) to 1.1-fold on the 4th day (349.5 mg kgdb−1). On the other hand, SSF did not have a significant effect on changing the SA content in the extracts. However, exceptions occurred on the 1st day (105.6 mg kgdb−1) and 9th day (114.1 mg kgdb−1) when a 1.1-fold increase in SA content was recorded (Fig. 2a). An increase in the VA (up to 1.2-fold) and p-CuA extraction yield (up to 1.3-fold) was observed during the first 4 days of SSF (Fig. 2b).
The content of p-HBA (Fig. 2c) in all the extracts of GP after SSF was higher than before SSF (day 0). The lowest increase in p-HBA was observed after 3 days of SFF (1.4-fold), while the highest increase occurred after the 9th day (3.0-fold). The SSF with T. versicolor during 15 days did not have a positive effect on the recovery of EA (Fig. 2b) and FA (Fig. 2c) from GP. Since most of the phenolic acids from agro-food waste occur in conjugated forms with sugar moieties linked to hydroxyl groups, it can be supposed that in the exponential phase of the growth of the microorganism, the release of free phenolic acids is the result of the activities of some carbohydrate-metabolizing enzymes, most likely β-glucosidase.1 In the later stages of fungal growth, generally, a decrease in the phenolic acid content occurred, with the exception of p-HBA, VA and SA verified on day 12 and p-CuA on day 9. These “peaks” in the phenolic acid content profile, especially for p-HBA and SA, with contents higher than at the beginning of the process (day 0), are probably the result of the action of extracellular ligninolytic enzymes (e.g. laccase), which break down the complex lignin structure and release the phenolic acids trapped in its structure. Shortly after liberation, the phenolic acids are polymerized by the further action of the ligninolytic enzymes, which is reflected in the decline in the phenolic acid content. Therefore, it is very important to conduct the SSF process under controlled process conditions and stop it when the desired product is obtained.
The content of the flavonol rutin increased 1.2-fold on day 1, after which it decreased with some fluctuation between 6–15 day of SSF. The content of flavonol quercetin and stilbene trans-resveratrol during all days of SSF decreased (Fig. 3).
Anthocyanins are an important subgroup of phenolic compounds responsible for red, purple, and blue colors, and are produced during ripening of fruits and vegetables.4 Recently, GP has been considered as a potential source of natural pigments with functional properties.28 It is well known that anthocyanins exert multiple protective effects against many health disorders involving simultaneous biological effects in the body, such as the prevention of generation of free radicals, inhibition of key enzymes involved in the onset of the inflammatory response; decrease in lipid peroxidation, decrease in blood sugar and concentration.28,29 In this study, eight anthocyanins were identified and quantified (Table 1). In the extracts obtained prior to the biological treatment (day 0), the results show that the dominant compound was malvidin-3-O-glucoside (83.9 mg kgdb−1) followed by peonidin-3-O-glucoside (28.0 mg kgdb−1), malvidin 3-O-p-coumaroylglucoside (12.4 g kgdb−1), delphinidin-3-O-glucoside (12.3 mg kgdb−1), cyaniding-3-O-glucoside (3.6 mg kgdb−1), petunidin-3-O-glucoside (3.5 mg kgdb−1), peonidin-3-O-p-coumaroylglucoside (2.6 mg kgdb−1) and peonidin (1.5 mg kgdb−1). The total content of anthocyanins was 147.7 mg kgdb−1. The obtained results are consistent with the study by Corrales et al.,30 where grape skin anthocyanins were extracted by high hydrostatic pressure. They reported that the recovery of anthocyanins increased when the glucoside moiety was linked to the flavylium nucleus or when the a higher number of methoxyl and hydroxyl groups was present. Ramirez-Lopez and DeWitt31 reported 2.80–38.29% lower values for associated detected anthocyanins in acetone: methanol (50
:
50) extracts of commercial dried GP in comparison with the results of this study. Otherwise, SSF did not have a positive effect on the recovery of anthocyanins from GP. Some compounds such as delphinidin-3-O-glucoside, cyaniding-3-O-glucoside, and petunidin 3-O-glucoside were present only in trace amounts in the extracts obtained from the biologically treated GP at days 1 and 2.
Table 1 Anthocyanin content of GP ethanol
:
water (1
:
1) extracts (mg kgdb−1)a obtained before (0 day) and during SSF (1–15 days) with T. versicolor
t, days |
Delph-3-O-glu |
Cyan-3-O-glu |
Petun-3-O-glu |
Peon-3-O-glu |
Malv-3-O-glu |
Peon |
Peon-3-O-p-coumglu |
Malv-3-O-p-coumglu |
Total |
Results are expressed as mean ± standard deviation of three determinations; “Total” sum of the determined anthocyanins; “—” not detected; “nq” not quantified; GP (grape pomace); SSF (solid-state fermentation); Delph-3-O-glu (delphinidin-3-O-glucoside); Cyan-3-O-glu (cyanidin-3-O-glucoside); Petun-3-O-glu (petunidin-3-O-glucoside); Peon-3-O-glu (peonidin-3-O-glucoside); Malv-3-O-glu (malvidin-3-O-glucoside); Peon (peonidin); Peon-3-O-p-coumglu (peonidin-3-O-p-coumaroylglucoside); Malv-3-O-p-coumglu (malvidin-3-O-p-coumaroylglucoside).
|
0 |
12.25 ± 2.30 |
3.64 ± 0.36 |
3.53 ± 0.83 |
28.04 ± 4.98 |
83.86 ± 9.70 |
1.51 ± 0.20 |
2.55 ± 0.31 |
12.36 ± 0.77 |
147.73 ± 19.46 |
1 |
nq |
nq |
nq |
2.06 ± 0.17 |
6.75 ± 0.15 |
0.87 ± 0.00 |
1.32 ± 0.03 |
6.93 ± 0.24 |
17.92 ± 0.59 |
2 |
nq |
nq |
nq |
2.12 ± 0.08 |
7.18 ± 0.39 |
0.87 ± 0.08 |
1.35 ± 0.01 |
7.36 ± 0.14 |
18.88 ± 0.69 |
3 |
— |
— |
— |
1.57 ± 0.01 |
5.14 ± 0.30 |
0.69 ± 0.06 |
1.29 ± 0.10 |
5.88 ± 0.14 |
14.57 ± 0.60 |
4 |
— |
— |
— |
1.45 ± 0.10 |
5.01 ± 0.55 |
0.64 ± 0.04 |
1.21 ± 0.09 |
5.49 ± 0.31 |
13.80 ± 1.08 |
5 |
— |
— |
— |
0.85 ± 0.06 |
2.47 ± 0.16 |
0.28 ± 0.00 |
0.72 ± 0.07 |
4.17 ± 0.34 |
8.48 ± 0.63 |
6 |
— |
— |
— |
0.87 ± 0.03 |
2.70 ± 0.16 |
0.27 ± 0.01 |
0.69 ± 0.01 |
4.04 ± 0.20 |
5.58 ± 0.41 |
9 |
— |
— |
— |
0.62 ± 0.08 |
1.60 ± 0.06 |
nq |
0.147 ± 0.08 |
3.35 ± 0.10 |
6.03 ± 0.32 |
12 |
— |
— |
— |
nq |
1.14 ± 0.12 |
nq |
nq |
1.12 ± 0.02 |
2.25 ± 0.14 |
15 |
— |
— |
— |
— |
nq |
— |
— |
0.54 ± 0.03 |
0.54 ± 0.03 |
There are several possible reasons that led to a reduction in the content of anthocyanin during the fermentation process. Firstly, anthocyanins are highly sensitive to temperature, light, oxygen, pH, etc. A fluctuation in pH, temperature, or oxygen concentration can occur during fermentation or in the further steps of extraction, purification and identification.28 Some studies suggest that the protective role of anthocyanins in different health disorders is better when they act in synergy with other phytochemicals from whole food instead when they are used in isolated forms such as the case in dietary supplements.29 Secondly, anthocyanins usually appear in plants in the glycosylated form, where the sugar moiety can be easily metabolized and consumed as a carbohydrate source for the growth of T. versicolor during the SSF process. Recently, it has been published that during the SSF process of pomegranate juice by Lactobacillus strains32 and during SSF of chokeberry pomace by the Aspergillus and Rhizopus strains,14 a reduction in the content of conjugated anthocyanins and increase in aglycone content simultaneously occurred. Thirdly, it may be assumed that the degradation of polyphenolic compounds presented in grape pomace occurs due to the reactions catalysed by laccase.1 Laccases are known to catalyse the oxidation of phenolic lignin units and a wide number of phenolic compounds and aromatic amines to radicals.33
Anti-inflammatory activities
Extracts from natural sources are rich in bioactive compounds, and therefore have the capacity to inhibit enzymes involved in inflammation processes, which may serve as templates for the development of potent anti-inflammatory drugs or used as potential alternatives to non-steroid drugs (NSAIDs), which are commonly used in inflammatory processes.34,35
Although mechanism of the anti-inflammatory activities of phenolic compounds is not fully understood, it has been hypothesized that these compounds exert anti-inflammatory activity by the inhibition of pro-inflammatory mediators and/or gene expression. The structure of polyphenolic compounds (a planar ring system, unsaturation in the C ring, number and position of hydroxyl groups in the B ring, methylation of hydroxyl groups, number and position of glucoside moiety, etc.) significantly influences the mechanism of their anti-inflammatory activity. For instance, C2–C3 double bond flavonoids exhibit higher anti-inflammatory potential than flavonoids lacking double bonds since they induce coplanarity between rings A and C and stimulate interaction of the double bond flavonoids with the active site of enzymes. Considering their structural diversity, polyphenols can act in multiple ways with minimal or null adverse side effects (e.g. gastrointestinal disorders) as opposite to drugs.35,36
The anti-inflammatory potential of lyophilized grape pomace extracts obtained prior to and after the biological treatment was determined via two tests based on the inhibition of key enzymes (5-LOX and HAase) in inflammatory processes.
Lipoxygenases (LOXs) are enzymes responsible for generating different leukotrienes, which are lipid mediators that play a key role in inflammation. Leukotrienes are synthesized in leucocytes from the arachidonic acid released from damaged membrane phospholipids via the action of 5-LOX.37,38
Phenolic compounds have the ability to block the cascade process of arachidonic acid metabolism by inhibiting lipoxygenase activity, and may serve as a scavenger of reactive free radicals, which are produced during arachidonic acid metabolism.39
In this study, it was observed that all the extracts presented promising 5-LOX inhibitory activity (IC50 ranging from 37 to 240 μg mL−1) compared with quercetin (IC50 = 3 μg mL−1), which was used as a positive control. The results suggest that certain phenolic components determined in the GP extracts (GA, VA, p-CuA, and p-HBA (Fig. 2), and Rut (Fig. 3)), as well as the possible synergistic effect of all extract components may contribute to the inhibition of 5-LOX. Additionally, SSF of GP with T. versicolor positively influenced the increase in 5-LOX inhibition, resulting in the lowest IC50 value (IC50 = 37 μg mL−1) on the 3rd day of fermentation, after which the 5-LOX inhibition capacity decreased.
HA is a mucopolysaccharide glycosaminoglycan occurring naturally throughout the human body including connective, epithelial, and neural tissues. HA is responsible for tissue integrity, which can be impaired under oxidative stress due to the depolymerisation induced by the enzyme hyaluronidase. Thus, the level of HA in serum can be used as a reliable biomarker of arthritis progression.40 For the HAase test, the highest anti-inflammatory activity was observed on the second day of SSF (IC50 = 444 μg mL−1). Bralley et al.41 reported that the seed and skin of the muscadine grape contain phenolic compounds that inhibit hyaluronidase (IC50 from 300 to 1000 μg mL−1) in vitro.
It can be noticed that a most effective inhibition for 5-LOX and HAase in the first 2–3 days of fermentation corresponds to the aforementioned increase in phenolic compounds over the same period, indicating that SSF contributes to the strengthening of the anti-inflammatory potential of GP extracts (Fig. 4). Additionally, a moderate to strong negative correlation between anti-inflammatory properties and individual phenolic compounds determined during 0–15 days of SSF was calculated. A correlation was determined for phenolic compounds, which were positively affected by SSF.
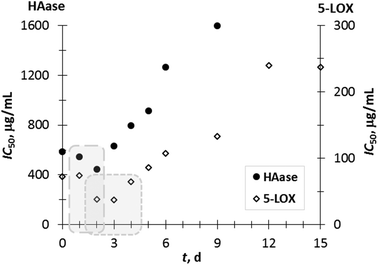 |
| Fig. 4 Inhibitory capacity (IC50) of the GP extracts towards 5-LOX and HAase. | |
The correlation coefficients (R) between the IC50 values for 5-LOX and content of VA, p-CuA, GA, CT, ECT, and Rut were −0.68, −0.74, −0.76, −0.80, −0.81 and −0.88, respectively. Similarly, the correlation coefficients (R) between HAase-IC50 and content of p-CuA, GA, CT, ECT, VA, and Rut were −0.66, −0.87, −0.92, −0.92, −0.93 and −0.97, respectively. These relations indicate that these compounds considerably contribute (p < 0.05) to the anti-inflammatory potential of grape pomace extracts.
Additionally, considering the fact that T. versicolor possess anti-inflammatory properties, some of the increased anti-inflammatory effects of the extract of the grape pomace treated by T. versicolor can be attributed to the bioactive compounds produced by the fungus. Recently, 27 different chemical constituents, such as triterpenoids, sterols, ribonucleotides, phenols, glycosides and furan were isolated from T. versicolor, and it was proven that sterols and triterpenoids significantly inhibit the production of cytokines NO, TNF-α and IL-6.42
Conclusions
The plain SSF process of GP by T. versicolor was studied. This study suggests that SSF is a potential biotechnological process, which can improve the anti-inflammatory properties of GP extracts. It was proven that the SSF process for a duration of up to four days can result in an increase in certain phenolic compounds and anti-inflammatory properties in GP extracts. For a better understanding of the mechanism of the SSF process, future research should focus on monitoring the fungal growth parameters and key enzyme kinetics, as well as possibilities of the addition of inducers for fungal growth and enzyme production, selection of specific microorganisms and optimization of the SSF process for GP. The results of this extensive study indicate the compounds in the GP extract responsible for its anti-inflammatory activity.
Conflicts of interest
There are no conflicts to declare.
Acknowledgements
This work was supported by the Croatian Science Foundation under the project (IP-2018-01-1227) “Development of a sustainable integrated process for the production of bioactive isolates from food industry residues” (POPI-WinCEco) and by UID/QUI/50006/2019 with funding from Fundação para a Ciência e a Tecnologia/Ministério da Ciência, Tecnologia e Ensino Superior (FCT/MCTES) through Portuguese funds, and by Programa de Cooperación Interreg V-A España – Portugal (POCTEP) 2014–2020 (project 0377_IBERPHENOL_6_E).
Fátima Fernandes thanks to REQUIMTE for the research contract (DL57/2016/CP1346/CT0018).
References
- C. M. Ajila, F. Gassara, S. K. Brar, M. Verma, R. D. Tyagi and J. R. Valéro, Polyphenolic antioxidant mobilization in apple pomace by different methods of solid-state fermentation and evaluation of its antioxidant activity, Food Bioprocess Technol., 2012, 5, 2697–2707 CrossRef CAS.
- A. Teixeira, N. Baenas, R. Dominguez-Perles, A. Barros, E. Rosa, D. A. Moreno and C. Garcia-Viguera, Natural Bioactive Compounds from Winery By-Products as Health Promoters: A Review, Int. J. Mol. Sci., 2014, 15, 15638–15678 CrossRef CAS PubMed.
- R. B. Pereira, M. Taveira, P. Valentão, C. Sousa and P. B. Andrade, Fatty acids from edible sea hares: anti-inflammatory capacity in LPS-stimulated RAW 264.7 cells involves iNOS modulation, RSC Adv., 2015, 5, 8981–8987 RSC.
- C. Beres, G. N. S. Costa, I. Cabezudo, N. K. da Silva-James, A. S. C. Teles, A. P. G. Cruz, C. Mellinger-Silva, R. V. Tonon, L. M. C. Cabral and S. P. Freitas, Towards integral utilization of grape pomace from winemaking process: A review, Waste Manage., 2017, 68, 581–594 CrossRef PubMed.
- M. Naczk and F. Shahidi, Extraction and analysis of phenolics in food, J. Chromatogr. A, 2004, 1054, 95–111 CrossRef CAS PubMed.
- P. Manara, A. Zabaniotu, C. Vanderghem and A. Richel, Lignin extraction from Mediterranean agro-waste: Impact of pretreatment conditions of lignin chemical structure and thermal degradation behaviour, Catal. Today, 2014, 22, 25–34 CrossRef.
- E. C. Sousa, A. M. A. Uchôa-Thomaz, J. O. B. Carioca, S. M. de Morais, A. de Lima, C. G. Martinis, C. D. Alexandrino, P. A. T. Ferreira, A. L. M. Rodrigues, S. P. Rodrigues, J. N. Silva and L. L. Rodrigues, Chemical composition and bioactive compounds of grape pomace (Vitis vinifera L.), Benitaka variety, grown in the semiarid region of Northeast Brazil, Food Sci. Technol., 2014, 31, 135–142 CrossRef.
- A. Bucić-Kojić, G. Šelo, B. Zelić, M. Planinić and M. Tišma, Recovery of phenolic acids and enzymes production from corn silage biologically treated by Trametes versicolor, Appl. Biochem. Biotechnol., 2017, 181, 948–960 CrossRef PubMed.
- T. B. Dey, S. Chakraborty, K. K. Jain, A. Sharma and R. C. Kuhad, Antioxidant phenolics and their microbial production by submerged and solid state fermentation process: A review, Trends Food Sci. Technol., 2016, 53, 60–74 CrossRef.
- S. Martins, S. I. Mussatto, G. Martínez-Avila, J. Montañez-Saenz, C. N. Aguilar and J. A. Teixeira, Bioactive phenolic compounds: Production and extraction by solid-state fermentation. A review, Biotechnol. Adv., 2011, 29, 365–373 CrossRef CAS PubMed.
- D. A. Mitchell, N. Krieger, D. M. Stuart and A. Pandey, New developments in solid-state fermentation: II. Rational approaches to the design, operation and scale-up of bioreactors, Process Biochem., 2006, 35, 1211–1225 CrossRef.
- R. R. Singhania, A. K. Patel, C. R. Soccol and A. Pandey, Recent advances in solid-state fermentation, Biochem. Eng. J., 2009, 44, 13–18 CrossRef CAS.
- J. J. Buenrostro-Figueroa, M. Velázquez, O. Flores-Ortega, J. A. Ascacio-Valdés, S. Huerta-Ochoa, C. N. Aguilar and L. A. Prado-Barragán, Solid state fermentation of fig (Ficus carica L.) by-products using fungi to obtain phenolic compounds with antioxidant activity and qualitative evaluation of phenolics obtained, Process Biochem., 2017, 62, 16–23 CrossRef CAS.
- F. V. Dulf, D. C. Vodnar, E.-H. Dulf, Z. Diaconeasa and C. Socaciu, Liberation and recovery of phenolic antioxidants and lipids in chokeberry (Aronia melanocarpa) pomace by solid state bioprocessing using Aspergillus niger and Rhizopus oligosporus strains, LWT–Food Sci. Technol., 2018, 87, 241–249 CrossRef CAS.
- G. C. Martínez-Ávila, A. F. Aguilera-Carbó, R. Rodríguez-Herrera and C. N. Aguilar, Fungal enhancement of the antioxidant properties of grape waste, Ann. Microbiol., 2012, 62, 923–930 CrossRef.
- S. Huang, Y. Ma, C. Zhang, S. Cai and M. Pang, Bioaccessibility and antioxidant activity of phenolics in native and fermented Prinsepia utilis Royle seed during a simulated gastrointestinal digestion in vitro, J. Funct. Foods, 2017, 37, 354–362 CrossRef CAS.
- M. Planinić, B. Zelić, I. Čubel, A. Bucić-Kojić and M. Tišma, Corn forage biological pretreatment by Trametes versicolor in a tray bioreactor, Waste Manage. Res., 2016, 34, 802–809 CrossRef PubMed.
- A. Blagodatski, M. Yatsunskaya, V. Mikhailova, V. Tiasto, A. Kagansky and V. L. Katanaev, Medicinal mushrooms as an attractive new source of natural compounds for future cancer therapy, Oncotarget, 2018, 9, 29259–29274 CrossRef PubMed.
- V. E. Pinheiro, M. Michelin, A. C. Vici, P. Zaghetto de Almeida and M. de Lourdes Teixeira de Moraes Polizeli,
Trametes versicolor laccase production using agricultural wastes: a comparative study in Erlenmeyer flasks, bioreactor and tray, Bioprocess Biosyst. Eng., 2019, 1–8 Search PubMed.
- A. P. M. Tavares, S. R. Pereira and A. M. R. B. Xavier, Biotechnological Applications of Trametes versicolor and their Enzymes, Curr. Biotechnol., 2017, 6, 78–88 CrossRef CAS.
- M. Tišma, M. Planinić, A. Bucić-Kojić, M. Panjičko, D. G. Zupančič and B. Zelić, Corn silage fungal-based solid-state pretreatment for enhanced biogas production in anaerobic co-digestion with cow manure, Bioresour. Technol., 2018, 253, 220–226 CrossRef PubMed.
- M. S. Dopico-García, A. Fique, L. Guerra, J. M. Afonso, O. Pereira, P. Valentão, P. B. Andrade and R. M. Seabra, Principal components of phenolics to characterize red Vinho Verde grapes: Anthocyanins or non-coloured compounds?, Talanta, 2008, 75, 1190–1120 CrossRef PubMed.
- V. Ferreira, F. Fernandes, O. Pinto-Carnide, P. Valentão, V. Falco, J. P. Martín, J. M. Ortiz, R. Arroyo-García, P. B. Andrade and I. Castro, Identification of Vitis vinifera L. grape berry skin color mutants and polyphenolic profile, Food Chem., 2016, 194, 94117–94127 CrossRef PubMed.
- I. Muckenschnabela, G. Bernhardta, T. Sprussa, B. Dietlb and A. Buschauera, Quantitation of hyaluronidases by the Morgan-Elson reaction: Comparison of the enzyme activities in the plasma of tumor patients and healthy volunteers, Cancer Lett., 1998, 131, 13–20 CrossRef.
- C. Zambrano, A. Kotogán, O. Bencsik, T. Papp, C. Vágvölgyi, K. C. Mondal, J. Krisch and M. Takó, Mobilization of phenolic antioxidants from grape, apple and pitahaya residues via solid-state fungal fermentation and carbohydrase treatment, LWT–Food Sci. Technol., 2018, 89, 457–465 CrossRef CAS.
- S. De Pascual-Teresa, D. A. Moreno and C. García-Viguera, Flavanols and Anthocyanins in Cardiovascular Health: A Review of Current Evidence, Int. J. Mol. Sci., 2010, 11, 1679–1703 CrossRef CAS PubMed.
- T. Kendari, Harijono, S. S. Yuwono, T. Estiasih and U. Santoso, The change of catechin antioxidant during vacuum roasting of Cocoa powder, J. Nutr. Food Sci., 2012, 2, 174 Search PubMed.
- V. B. De Souza, A. Fujita, M. Thomazini, E. R. da Silva, J. F. Lucon, M. I. Genovese and C. S. Favaro-Trindade, Functional properties and stability of spray-dried pigments from Bordo grape (Vitis labrusca) winemaking pomace, Food Chem., 2014, 164, 380–386 CrossRef PubMed.
- M. A. Lila, Anthocyanins and human health: An in vitro investigative approach, J. Biomed. Biotechnol., 2004, 5, 306–313 CrossRef PubMed.
- M. Corrales, M. C. García, P. Butz and B. Tauscher, Extraction of anthocyanins from grape skins assisted by high hydrostatic pressure, J. Food Eng., 2009, 90, 415–421 CrossRef CAS.
- L. M. Ramirez-Lopez and C. A. M. DeWitt, Analysis of phenolic compounds in commercial dried grape pomace by high-performance liquid chromatography electrospray ionization mass spectrometry, Food Sci. Nutr., 2014, 2, 470–477 CrossRef CAS PubMed.
- Z. E. Mousavi, S. M. Mousavi, S. H. Razavi, M. Hadinejad, Z. Emam-Djomeh and M. Mirzapour, Effect of fermentation of pomegranate juice by Lactobacillus plantarum and Lactobacillus acidophilus on the antioxidant activity and metabolism of sugars, organic acids and phenolic compounds, Food Biotechnol., 2013, 27, 1–13 CrossRef CAS.
- R. Sindhu, P. Binod and A. Pandey, Biological pretreatment of lignocellulosic biomass - An overview, Bioresour. Technol., 2016, 199, 76–82 CrossRef CAS PubMed.
- S. A. Adebayo, J. P. Dzoyem, L. J. Shai and J. N. Elof, The anti- inflammatory and antioxidant activity of 25 plant species used traditionally to treat pain in southern African, BMC Complementary Altern. Med., 2015, 15, 1–10 CrossRef CAS PubMed.
- D. L. Ambriz-Pérez, N. Leyva-López, E. P. Gutierrez-Grijalva and J. B. Heredia, Phenolic compounds: Natural alternative inflammation treatment, A Review, Cogent Food Agric., 2016, 2, 1–14 Search PubMed.
- U. R. Kuppusamy, H. E. Khoo and N. P. Das, Structure-activity studies of flavonoids as inhibitors of hyaluronidase, Biochem. Pharmacol., 1990, 40, 397–401 CrossRef CAS PubMed.
- J. N. Sharma and L. A. Mohammed, The role of leukotrienes in the pathophysiology of inflammatory disorders: Is there a case for revisiting leukotrienes as therapeutic targets? Review, Inflammopharmacology, 2006, 14, 10–16 CrossRef CAS PubMed.
- M. Figueiredo-González, P. Valentão and P. B. Andrade, Tomato plant leaves: From by-products to the management of enzymes in chronic diseases, Ind. Crops Prod., 2016, 94, 621–629 CrossRef.
- U. S. Akula and B. Odhav,
In vitro 5-Lipoxygenase inhibition of polyphenolic antioxidants from undomesticated plants of South Africa, J. Med. Plants Res., 2008, 2, 207–2012 Search PubMed.
- V. N. Sumantran, A. A. Kulkarni, A. Harsulkar, A. Wele, S. J. Koppikar, R. Chandwaskar, V. Gaire, M. Dalvi and U. V. Wagh, Hyaluronidase and collagenase inhibitory activities of the herbal formulation Triphala guggulu, J. Biosci., 2007, 32, 755–761 CrossRef CAS PubMed.
- E. Bralley, P. Greenspan, J. L. Hargrove and D. K. Hartle, Inhibition of hyaluronidase activity by Vitis rotundifolia (Muscadine) berry seeds and skins, Pharm. Biol., 2007, 45, 667–673 CrossRef CAS.
- M. Jin, W. Zhou, C. Jin, Z. Jiang, S. Diao, Z. Jin and G. Li, Anti-inflammatory, activities of the chemical constituents isolated from Trametes versicolor, Nat. Prod. Res., 2019, 33, 2422–2425 CrossRef CAS PubMed.
|
This journal is © The Royal Society of Chemistry 2020 |