DOI:
10.1039/C9FO02089F
(Paper)
Food Funct., 2020,
11, 779-786
Mild heat combined with lactic acid fermentation: a novel approach for enhancing sulforaphane yield in broccoli puree
Received
9th September 2019
, Accepted 23rd December 2019
First published on 26th December 2019
Abstract
This study evaluated for the first time the feasibility of mild preheating treatment of broccoli florets combined with lactic acid bacteria fermentation for enhancing sulforaphane yield in broccoli puree. The optimum preheating condition for in-pack processing of broccoli florets was 3 min treatment at 65 °C increasing sulforaphane yield in broccoli puree by ∼5 times compared to untreated broccoli. Preheating of broccoli florets in-pack (65 °C per 3 min) combined with lactic acid bacteria fermentation further enhanced the sulforaphane content by ∼16 times compared to untreated broccoli. The sulforaphane content of the preheated-fermented puree remained stable (∼94% retention) for two weeks at 4 °C. The results indicate that a combination of judicious heat treatment of broccoli florets with lactic acid bacteria fermentation enables production of safe and high sulforaphane content broccoli products with potential health benefits.
Introduction
Epidemiological studies indicate that a diet rich in vegetables may play a significant role in preventing many chronic diseases, such as cancer, immune dysfunction, cardiovascular diseases, and type II diabetes.1,2 Cruciferous vegetables, such as broccoli are rich sources of glucosinolates, which contribute to their beneficial effects on human health.3–5 The predominant glucosinolate in broccoli is glucoraphanin, which is not bioactive. However, when plant tissue containing glucoraphanin is shredded, chewed or crushed, glucoraphanin is hydrolysed into different breakdown products by the endogenous enzyme ‘myrosinase’ (thioglucoside glucohydrolase EC 3:2:3:1).6 Sulforaphane, one of the products of glucoraphanin hydrolysis, is the main biologically active compound that contributes to human health.7 Sulforaphane exerts a multitude of health benefits, including anti-inflammatory, immunomodulatory, anti-thrombotic, anti-diabetic and anti-cancer effects.7–10
The bioavailability of sulforaphane and the health benefits that are derived from it are dependent on its mode of delivery. The bioavailability of sulforaphane from administration of glucoraphanin without active myrosinase, as in cooked broccoli, is on average 11.5%.11 On the other hand, the average bioavailability from glucoraphanin administered with active myrosinase is ∼40% (ref. 12) whereas the bioavailability from administering pre-formed sulforaphane is between 70 to 90%.11 The challenge in the delivery of pre-formed sulforaphane include (1) the low yield of sulforaphane in traditionally cooked or frozen broccoli due to thermal inactivation of myrosinase,13,14 (2) the low yield of sulforaphane in raw broccoli due to the presence of a protein co-factor epithiospecifier protein (ESP) which directs conversion of glucoraphanin away from sulforaphane to the non-bioactive sulforaphane nitrile15 and (3) sulforaphane's characteristic instability16 and its sensitivity to degradation during thermal processing required for ensuring microbial safety.17
The extent of glucoraphanin hydrolysis during tissue disruption and the yield of different metabolites depend on factors such as the presence of active ESP, ferrous ions, pH and temperature. Myrosinase cleaves the β-D-glucose of glucoraphanin to form the unstable intermediate aglucone, which spontaneously rearranges into sulforaphane nitrile and sulforaphane at different proportions depending on the reaction condition (Fig. 1). The presence of active ESP, ferrous ion, low temperature and low pH favour the formation of sulforaphane nitrile.18 In raw broccoli, sulforaphane nitrile is the main product of glucoraphanin hydrolysis.14,19 Sulforaphane nitrile has limited biological activity with 1000 times concentration of sulforaphane nitrile compared to sulforaphane required to have the same degree of induction of phase II detoxification enzymes20 and cytotoxic effects against cancer cells.21
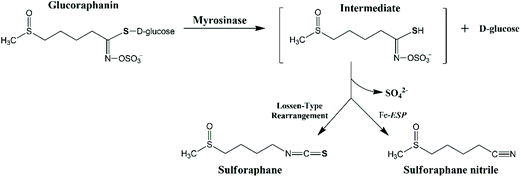 |
| Fig. 1 The proposed mechanism of the hydrolysis of glucoraphanin into the main metabolites i.e. sulforaphane and sulforaphane nitrile. | |
Because of the various health benefits of sulforaphane, there is a great deal of interest in approaches for maximising the yield of sulforaphane and improve its stability. Matusheski et al.22 studied the possibility of enhancing sulforaphane yield in broccoli through selective inactivation of ESP while maintaining myrosinase activity through mild heat treatment. They observed that heat treatment of broccoli floret for 5 min at 60 °C enables selective inactivation of ESP and maximises sulforaphane yield.20 Guo et al.23 investigated enzymatic hydrolysis conditions for optimising the yield of isothiocyanates including sulforaphane in broccoli sprouts. They varied pH (4.0 to 6.0), ascorbic acid (0.1 to 0.3 g) and EDTA (0.02 to 0.06 mmol) concentration in 0.2 g broccoli sprouts homogenized in 4 mL phosphate-citrate buffer according to a response surface experimental design, while maintaining the temperature at 40 °C. Among the conditions examined, the optimum isothiocyanate yield of 699 mg per 100 g fresh weight was obtained at pH 4.0, with 0.02 mmol EDTA and 0.16 mg ascorbic acid added into the reaction mixture.23 As mentioned earlier, the challenge with the delivery of pre-formed sulforaphane in a broccoli matrix is not only low sulforaphane yield but also its susceptibility to chemical and thermal degradation.16,24 Therefore, other studies investigated alternative approaches for improving sulforaphane yield in broccoli products while minimizing sulforaphane degradation. Processes examined to date include the use of physical emerging processes such as high pressure processing6 and lactic acid bacteria fermentation. Van Eylen et al.6 evaluated high pressure combined with heat treatment to increase the yield of sulforaphane. High pressure treatment for 15 min at 20 °C and pressures ranging from 200 to 500 MPa resulted in equivalent sulforaphane yield of about 50% on glucoraphanin basis as treatment of the same duration at 60 °C. The highest sulforaphane yield of about 70% was obtained after 35 min processing at 300 MPa and 40 °C.6 We investigated the feasibility of using lactic acid bacteria (LAB) fermentation for enhancing the yield and stability of sulforaphane in broccoli matrix. Lactic acid bacteria fermentation of broccoli puree using autochthonous lactic acid bacteria doubled sulforaphane yield compared to non-fermented puree and maintained high stability of sulforaphane during storage for about three months at 4 °C.25
Based on our previous work on the impact of lactic acid bacteria fermentation on sulforaphane yield25 and preceding research that showed that mild heat treatment selectively inactivates ESP and enhance the formation of sulforaphane,22 we hypothesized that mild heat treatment of broccoli combined with fermentation may further increase the yield of sulforaphane while enabling the production of a microbially safe and stable product. Our earlier study25 showed that lactic acid bacteria fermentation enables control of undesirable spoilage and pathogenic microorganisms following processing and during storage for up to three months at 4 and 25 °C. In this study, we evaluated for the first time the combined effect of mild heat pre-treatment and lactic acid bacteria fermentation of broccoli puree on sulforaphane yield and stability. The study included investigation of mild heat treatment conditions for optimal yield of sulforaphane and the impact of mild pre-heating under optimized condition combined with LAB fermentation on the yield of sulforaphane. The stability of sulforaphane in the fermented product for 14 days storage at 4 and 25 °C was also evaluated.
Experimental
Materials
Broccoli (cv. ‘Viper’) was purchased from a local supermarket (Coles, Werribee South, VIC, Australia). DeMan–Rogosa–Sharp (MRS) broth (1823477, CM0359, Oxoid) was purchased from Thermo Fisher Scientific (Australia). DL-Sulforaphane was purchased from Sigma-Aldrich (St Louis, Missouri, USA) and glucoraphanin was purchased from Chromadex (USA). Glucono-delta-lactone, ethyl acetate, acetonitrile, formic acid, ammonium formate were all analytical grade and were purchased from Merck (Macquarie Park, NSW, Australia).
Experiments to optimize pre-heating conditions for maximizing sulforaphane yield
Broccoli florets were cut at approximately 2 cm below the head, and each 30 g of randomly mixed broccoli florets were used in the pre-heating experiments. Two types of pre-heating experiments were conducted; in pack processing and direct water blanching. In the case of the in-pack experiments, broccoli florets were packed in retort pouches (Caspak Australia, Melbourne), sealed and pre-heated for various times in a thermostated water bath maintained at 60 °C, 65 °C and 80 °C. The temperature of the broccoli samples at the slowest heating point was measured by using a thermometer. The pointed head of a thermometer was inserted into the core of a broccoli floret in the centre of a sealed dummy sample that was heated together with an actual sample. Time 0 was defined as the time for the core temperature to reach the designated experimental temperature. The treatment times were 0, 1, 3, and 5 min for 60 °C and 65 °C and 0, 1, 2, 3 min for 80 °C. With the direct water-blanching experiments, the broccoli florets were immersed in Milli-Q water (10 mL per gram of broccoli) heated to the experimental temperature in a glass beaker that was kept in a thermostated water-bath maintained at the experimental temperature. The direct water blanching experiments were conducted at 60 °C and 65 °C, and the treatment times were 0, 1, 3, and 5 min. The experimental temperatures were selected based on the results of the in-pack heating experiments. The temperature of the broccoli samples was continuously measured using a thermometer and timing started once the temperature at the slowest heating point attained the designated experimental temperature as described above. All thermal treatment experiments were carried out in triplicate. Unheated broccoli florets were used as controls. Immediately following the heat treatment, the samples were cooled in ice water and were homogenized with Milli-Q water in a ratio of 3 parts of broccoli to 2 parts of water for 1 min using a kitchen scale magic bullet blender (Nutribullet pro 900 series, LLC, USA). The homogenized samples were incubated in dark for 4 h at 25 °C to allow the enzymatic hydrolysis of glucoraphanin.14 After incubation, all the samples were kept frozen at −20 °C until sulforaphane analysis. All experiments were conducted in triplicates.
Preparation of starter cultures
Pooled cultures of Leuconostoc mesenteroides (BF1, BF2) and Lactobacillus plantarum (B1, B2, B3, B4) isolated from broccoli as described in Cai et al.25 were used in the fermentation experiments. The lactic acid bacteria stock cultures, which were stored at −80 °C, were activated by inoculation into 10 mL MRS broth (Oxoid, Victoria, Australia) and incubation at 30 °C for 24 hours to get the primary inoculum. 2 mL of the primary cultures were inoculated into 200 mL of MRS broth to obtain the secondary cultures. After 24 h incubation, the 7 secondary cultures were centrifuged, washed twice with sterile phosphate buffer saline (PBS) and each of the culture was resuspended in Milli-Q water at a concentration of 10 log colony-forming units per millilitre (CFU mL−1) to obtain an initial biomass of 8 log CFU mL−1 in 100 g broccoli puree samples. The L. Plantarum cultures were mixed with the L. mesenteroides cultures at 1
:
1 proportion prior to inoculation into the broccoli puree samples.
Sample preparation for fermentation
Broccoli florets were cut at approximately 2 cm below the crown and were separated into two lots; heat treated and non-treated. After heat treatment at the optimal condition selected based on the results of the optimal pre-heating experiments, the samples were cooled in ice-water, shredded and homogenized with Milli-Q water in ratio of 3
:
2 for 1 min using a kitchen scale magic bullet blender (Nutribullet pro 900 series, LLC, USA). Non-treated broccoli was also pureed in a similar way. The broccoli puree, after mixing well, was aliquoted into sterile plastic containers (100 mL) with screw lids (Technoplast Australia) for further experiments.
Fermentation
Broccoli puree samples (pre-heated and untreated) were inoculated with the LAB culture prepared as described in ‘preparation of starter cultures’ section. In order to evaluate the impact of acidification without fermentation on conversion of glucoraphanin into sulforaphane, acidification experiments were conducted on pre-heated and untreated broccoli puree using glucono-delta-lactone (GDL) to attain the pH of the fermented broccoli puree. Preheated broccoli puree and untreated broccoli puree without further treatment were used as controls. The experimental plan is schematically shown in Fig. 2.
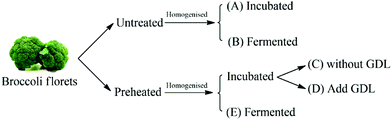 |
| Fig. 2 The schematic representation of the experimental design. GDL stands for glucono-delta-lactone. | |
For the fermentation experiment, each broccoli puree sample was inoculated with the prepared starter culture at a dose of 8 log CFU g−1. Samples were then incubated at 30 °C for ∼15 h for the pre-heated samples and ∼24 h for the control samples until the pH reached ∼4.0. Once the fermentation was completed, 3 samples from each group were taken and stored at −20 °C until analysis as day ‘0’ samples. The rest of the ferments were randomly separated into two lots for the storage trials: one lot was stored under refrigerated condition (4 °C) and the second lot was stored at 25 °C for assessment of the stability of sulforaphane in the samples after 14 days storage. Similarly, the untreated broccoli puree, the preheated broccoli puree and the preheated-GDL treated broccoli puree were incubated at 30 °C for equivalent period as the respective fermented samples. At the end of the incubation period (time ‘0’), samples randomly selected for analysis as time zero samples were frozen and stored at −20 °C until analysis. The rest of the samples were divided into two lots for the 14 days storage trial at 25 and 4 °C. After 14 days storage, all the samples were frozen and kept at −20 °C until sulforaphane analyses. All the experiments were conducted in triplicate.
Sulforaphane and glucoraphanin analysis
Sulforaphane analysis was performed according to the method of Li et al.26 with some modifications.25 Accordingly, sulforaphane was extracted by adding 2 mL Milli-Q water into 2 g broccoli, to which 20 mL ethyl acetate was added after vortexing followed by sonication for 5 min and shaking for 20 min at 4 °C. After the first extraction, the supernatant was collected, and 15 mL ethyl acetate was added to the precipitate for second extraction. The supernatants from the two extractions were pooled together and evaporated to dryness with a vacuum spin dryer (SC250EXP, Thermo Fisher Scientific, CA, USA) at 25 °C. The dried extracts were stored at −20 °C until analysis. For sulforaphane analysis, the dried samples were suspended in 30% acetonitrile and the suspension was filtered using a 0.22 μm membrane filter (Merk Millipore, Billerica, MA, USA) before injection into the HPLC column.
Chromatographic analysis of the samples were carried out using an Acquity™ Ultra Performance LC system managed by Acquity console software (Waters Corporation, Milford, MA, USA). Separation was achieved on an Acquity BEH C18 chromatography column (2.1 × 50 mm). The column oven temperature was maintained at 30 °C and the autosampler at 4 °C. The mobile phase consisted of 0.1% formic acid in Milli-Q water (mobile phase A) and 0.1% formic acid in acetonitrile (mobile phase B) at a flow rate of 0.350 mL min−1 with an injection volume of 5 μL. The elution gradient conditions were: 0–2 min, 10% (mobile phase B); 2–5 min, 20% B; 5–10 min, 10% B, with a total run time of 10 min.
The identification of each peak was based on retention time and spectral data acquired through the chromatography of an authentic standard. The concentration of sulforaphane was calculated based on a standard curve and the result was expressed as micromoles per kilogram dry weight (DW) (μmol per kg DW) of broccoli. The DW of each sample was determined from the moisture content measured using HR73 Halogen Moisture Analyzer (Mettler Toledo Ltd, Victoria, Australia).
The glucoraphanin content of the broccoli samples was analysed as described in Cai et al.25 In short, for fresh broccoli samples, 2 g of broccoli floret samples were mixed with 20 mL boiling Milli-Q water and were heated for 10 min at 100 °C to inactivate endogenous myrosinase. The samples were cooled and then homogenised using Ultraturax for 3 min. This was followed by centrifugation for 15 min at 15
000g at 4 °C. The supernatant was collected, and the precipitate was redissolved in 8 mL boiling Milli-Q water for a second extraction. The supernatants from the two extractions were pooled together and were evaporated to dryness with a vacuum spin dryer (SC250EXP, Thermo Fisher Scientific, CA, USA) at 35 °C, and stored at −20 °C until analysis. The dried samples were resuspended in 1 mL of 30 mM Ammonium formate solution just before HPLC analysis, and filtered using a 0.22 μm membrane filter (Merk Millipore, Billerica, MA, USA). The analysis was conducted using an Alliance HPLC system (Waters Corporation, Milford, MA, USA) equipped with Photo Diode Array Detector 2998 using Luna® 3 μM Hydrophilic Interaction Liquid Chromatography (HILIC) 200° A (100 × 4.6 mm; Phenomenex, Torrance, CA, USA) column. The identification of the glucoraphanin peak was based on the retention time and the chromatography of an authentic glucoraphanin standard. The quantification of glucoraphanin was based on a standard curved developed with a series of known concentration of glucoraphanin standards and the result was expressed as micromoles glucoraphanin per kilogram DW (μmol per kg DW) of broccoli.
Statistical analysis
All the experiments were conducted in triplicates and the results were expressed as mean ± SD. Statistical analysis of data was performed using the statistical software, SPSS 16.0 for Windows (SPSS Inc., Chicago, IL, USA). Data was analysed by one-way ANOVA to evaluate the significance of the differences among the mean values, at 0.05 significance level (p < 0.05).
Results and discussion
Optimization of heat treatment conditions for improving sulforaphane yield
The average glucoraphanin content of the broccoli floret samples was 3141.2 ± 133.5 μmol per kg DW. The influence of heat treatment conditions on the yield of sulforaphane from glucoraphanin hydrolysis in in-pack heated broccoli florets are shown in Fig. 3. In-pack heat treatment at all the studied conditions increased sulforaphane yield compared to raw broccoli puree in line with previous reports.22,27 Mild thermal treatments of broccoli florets results in a relatively higher level of inactivation of epithiospecifier protein (ESP) compared to myrosinase, which reduces the formation of sulforaphane nitrile while enhancing sulforaphane production.22 For treatments at 60 °C, increasing treatment time from 0 to 5 min resulted in an increase in sulforaphane yield. The concentration of sulforaphane in these samples were 2343.5 ± 124.1, 2661.5 ± 10.9, 2780.9 ± 270.8, and 3147.7 ± 148.0 μmol per kg DW, in samples treated for 0 (samples pre-heated to 60 °C followed by immediate cooling), 1, 3 and 5 min respectively. On the other hand, when broccoli was processed at 65 °C, the sulforaphane yield initially increased with processing time from 3585.9 ± 119.2 (at 0 min) to the highest value of 3983.4 ± 30.5 μmol per kg DW at 3 min. Further increase in treatment time resulted in lower yield with the lowest value of 3620.1 ± 240.7 μmol per kg DW after 5 min treatment time. In contrast to treatments at 60 and 65 °C, a steady decrease in sulforaphane yield was observed with longer treatment times for samples that were processed at 80 °C with sulforaphane content of 1451.5 ± 43.5, 1446.8 ± 17.5, 1043.1 ± 94.2, and 981.2 ± 35.1 μmol per kg DW corresponding to 0, 1, 2 and 3 min treatments respectively. The highest yield of sulforaphane (3983.4 ± 30.5 μmol per kg DW) for in-pack treatment of broccoli was obtained for samples pre-heated at 65 °C for 3 min, which was ∼5 fold higher than raw broccoli (817.5 ± 9.3 μmol per kg DW).
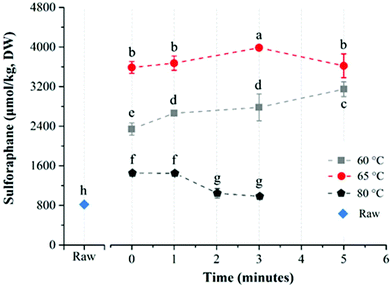 |
| Fig. 3 Sulforaphane yield (μmol per kg DW) in broccoli puree during in-pack heat treatment at different conditions. Different superscripts on the data points indicate significant difference (p < 0.05). Raw designates untreated broccoli puree. Time 0 designates samples pre-heated to the experimental temperature and immediately cooled. Reported values are means of three replicate samples and error bars represent standard deviation values. | |
The effect of direct water blanching on sulforaphane yield was dependent on temperature (Fig. 4). Overall, heating broccoli directly in water resulted in a lower yield of sulforaphane compared to in-pack processing. For direct water blanching at 60 °C, the sulforaphane yield increased with treatment time from 1698.0 ± 121.9 μmol per kg DW (0 min) to 2833.3 ± 118.6 μmol per kg DW (1 min) and then steadily decreased to the lowest value of 2345.8 ± 57.7 μmol per kg DW for 5 min treatment at 60 °C (Fig. 4). A sharp drop in sulforaphane yield compared to 60 °C was observed when samples were blanched at 65 °C. The sulforaphane yield was 503.7 ± 23.8 μmol per kg DW of broccoli after 5 min thermal treatment at 65 °C, which was even lower than the value obtained for raw broccoli. The reason could be the leaching of glucoraphanin into the blanching water28 coupled with partial inactivation of myrosinase resulting in low yield of sulforaphane. For direct water blanching, the best treatment temperature for maximizing sulforaphane yield was 60 °C, which is similar to the best treatment temperature for maximizing sulforaphane yield reported previously.22 This is most likely due to the similarity in the heat treatment procedure since direct water blanching was used in that study.
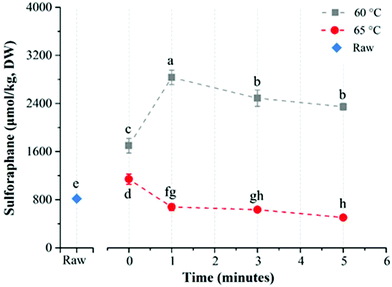 |
| Fig. 4 Sulforaphane (μmol per kg DW) in broccoli puree during direct water blanching at different conditions. Different superscripts on the data points indicate significant difference (p < 0.05). Raw designates untreated broccoli puree. Time 0 designates samples pre-heated to the experimental temperature and immediately cooled. Reported values are means of three replicate samples and error bars represent standard deviation values. | |
The heat treatment conditions that are required to enhance sulforaphane content could vary due to the differences in the initial level and thermal stability of ESP and myrosinase in different broccoli cultivars.14 The thermostability of myrosinase, the enzyme responsible for the conversion of glucoraphanin into sulforaphane, varies depending on factors such as source and matrix composition.29 Ludikhuyze et al.30 observed that broccoli myrosinase in buffer (pH 6.55) is stable up to 35 °C and is almost completely inactivated after 2 min treatment at 60 °C. In broccoli juice on the other hand, Van Eylen et al.17 reported that the enzyme was stable up to 40 °C, with ∼40% inactivation after 1 min treatment at 60 °C, ∼60% inactivation after 2 min at 60 °C and 90% inactivation after 10 min treatment at 60 °C. Similarly, a study by the same group30 showed that myrosinase in broccoli floret was stable up to 45 °C, with ∼30% inactivation after 1 min treatment at 60 °C, ∼63% inactivation after 2 min treatment at 60 °C and ∼90% inactivation after 10 min treatment at 60 °C. Another study reported a higher thermal stability for broccoli myrosinase with ∼80% of myrosinase activity remaining after 4 min treatment at 60 °C, 60% remaining after 8 min treatment at 60 °C and ∼50% remaining after 4 min treatment at 70 °C during in-pack processing of broccoli florets,28 which could be due to varietal differences and differences in growing conditions. ESP has been shown to be relatively more thermolabile compared to myrosinase with complete inactivation after 5 min treatment at 65 °C or 10 min treatment at 60 °C.16
Sulforaphane yield depends on the relative activity of myrosinase and ESP in the broccoli matrix and 3 min treatment at 65 °C during in-pack processing in this study was found to be the best condition that favours conversion into sulforaphane instead of sulforaphane nitrile. This indicates that the condition favours the inactivation of ESP to a larger extent while maintaining sufficient myrosinase activity resulting in optimal conversion into sulforaphane. Under this condition, it seems that all of the extractable glucoraphanin is converted to sulforaphane assuming 1 to 1 conversion, since the glucoraphanin content of the broccoli samples were determined to be 3141.2 μmol per kg DW whereas the sulforaphane yield was 3983 μmol per kg DW. The slightly higher sulforaphane yield than would be predicted from the level of glucoraphanin in raw broccoli requires further investigation. It is possible that the heat-treatment may have resulted in the release of bound glucoraphanin or enhanced tissue decompartmentalization and enzyme–substrate interaction resulting in a higher sulforaphane yield than would be expected based on the quantifiable amount of glucoraphanin present in the untreated broccoli.14
Direct water blanching of the broccoli florets reduced sulforaphane yield compared to in-pack processing, most probably due to leaching into the blanching water,28 since glucoraphanin is water soluble. It is also interesting to note that when broccoli florets were heated directly in water, the maximum amount of sulforaphane was obtained by heating at 60 °C for 1 min compared to 65 °C for 3 min during in-pack processing. This may be due to the higher leaching rate into the blanching water at 65 °C which counteracted the effects of higher level of inactivation of ESP at 65 °C. The process may have also resulted in a higher degree of myrosinase inactivation compared to in-pack processing. The rate of heat transfer into the broccoli tissue depends on the mode of heat treatment. The stated processing temperatures are temperatures at the slowest heating point in the tissue after temperatures equilibration. The spatial temperature distribution in the broccoli tissue during heating varies depending on the mode of heating and as such the cumulative effect of the heating process on the relative activity of ESP and myrosinase at different temperatures also depends on the mode of heating. Due to direct exposure to hot water, direct blanching at 65 °C may have resulted in a higher cumulative heat load and higher level of myrosinase inactivation compared to the same treatment during in-pack processing, which may have contributed to the observed difference in the best treatment condition between in-pack processing and direct blanching.
The effect of LAB fermentation and chemical acidification on sulforaphane yield
Broccoli florets were pre-heated in-pack at the best treatment condition selected above (65 °C, 3 min). Samples were then subjected to either fermentation by lactic acid bacteria or acidified using an acidulant (GDL). Consistent with the pre-treatment experiments, the sulforaphane yield of broccoli significantly increased (p < 0.05) after the heat treatment; with 806.2 ± 7.0 μmol per kg DW and 3536.0 ± 136.9 μmol per kg DW of sulforaphane yield for raw and pre-heated broccoli, respectively.
As can be seen in Table 1, after fermentation, the sulforaphane content of broccoli samples varied depending on the treatment of the broccoli prior to fermentation. The sulforaphane content of raw broccoli puree after fermentation (1617.4 ± 10.2 μmol per kg DW) was approximately twice the sulforaphane content of raw broccoli puree. Pre-heating of broccoli prior to pureeing resulted in a much higher increase in sulforaphane content after fermentation. The sulforaphane content of preheated-fermented broccoli (13
121.3 ± 440.8 μmol per kg DW) was about 8 times of the raw-fermented broccoli puree. The observed sulforaphane yield after the combined preheating-fermentation treatment is much higher than what would be expected based on the average quantifiable amount of glucoraphanin (3141.2 ± 113.5 μmol per kg DW) in the raw broccoli samples. It seems that the combined preheating and fermentation process enhances the release and accessibility of glucoraphanin for conversion over and above the inactivation of ESP by the pre-heating process. The pre-heating process coupled with microbial cell wall degrading enzymes may have enhanced the disruption of the cell compartment and release of bound glucosinolates in the matrix, that were not extractable or accessible in the raw broccoli. Some lactic acid strains produce polysaccharide degrading enzymes such as cellulases and pectinases capable of degrading the cell wall structure and enhance the release of wall bound components.31 In an earlier investigation on retorted (121 °C, 3 min) broccoli puree with no active myrosinase, fermentation with the lactic acid bacteria strains used in this study increased the glucoraphanin concentration from trace amount to 66–540 μmol per kg DW depending on the strain, indicating that fermentation results in the release of bound glucoraphanin.32
Table 1 Sulforaphane yield (μmol per kg DW) of broccoli after processing at different conditions
|
Sulforaphane (μmol per kg, DW) |
Raw |
Raw-fermented |
Preheat |
Preheat GDL |
Preheat-fermented |
Different letters within a group indicate a significant difference (p < 0.05). DW: dry weight. |
Day 0 |
806.2 ± 7.0e |
1617.4 ± 10.2d |
3536.0 ± 136.9b |
2169.4 ± 176.0c |
13 121.3 ± 440.8a |
In contrast, chemical acidification of preheated broccoli puree by GDL resulted in a significantly lower (p < 0.05) content of sulforaphane compared to pre-heated and preheated-fermented samples (Table 1). The sulforaphane content of the GDL acidified samples were 2169.4 ± 176.0 μmol per kg DW, which is 40% lower than the preheated broccoli sample (3536.0 ± 136.9 μmol per kg DW) (P < 0.05). It appears that the fast reduction to pH 4.04 during acidification may have reduced the conversion of glucoraphanin into sulforaphane in the GDL samples. It is well known that the conversion of glucosinolates is highly dependent on pH and acidic pH favours conversion into nitriles.19 In the case of the pre-heated fermented samples, the acidification occurs gradually over a period of 15 hours enabling the conversion of glucoraphanin mainly to sulforaphane since the activity of ESP is expected to be significantly reduced after preheating at 65 °C for 3 min.
Changes of sulforaphane content during storage
The concentration of sulforaphane of all the fermented samples declined after 14 days storage at 25 °C (Fig. 5a). Interestingly, increase in sulforaphane content was observed in all samples except the fermented samples after 14 days storage at 4 °C. Some increase in sulforaphane content was also observed in the control and the GDL acidified preheated samples during storage at 25 °C. The sulforaphane content of the raw puree almost doubled during storage at 4 °C. Similarly, the sulforaphane content of the pre-heated samples increased by ∼2.6 times whereas the sulforaphane content of the preheated GDL samples increased by ∼2.3 times, which suggests continuous release of glucoraphanin from the matrix during storage allowing further conversion to sulforaphane and increase in concentration counteracting the consequence of sulforaphane degradation during storage. With respect to the preheated-fermented samples, a significant reduction in sulforaphane content was observed specially after storage at 25 °C. Perhaps, all the accessible glucoraphanin is converted to sulforaphane during fermentation so much so that no further conversion occurred during storage but rather degradation albeit to a different extent depending on the temperature. As such, only a slight decline (∼6%) was observed after storage at 4 °C whereas the decline during storage at 25 °C was ∼70%. The rate of sulforaphane degradation during storage is higher at higher temperature.33
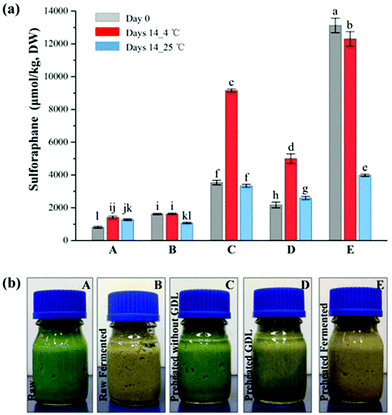 |
| Fig. 5 (a) Sulforaphane (μmol per kg DW) yield in broccoli puree processed at different conditions and its stability during storage at 4 °C and 25 °C. Different superscripts on the data points indicate significant difference (p < 0.05), (b) photographs of the samples processed at different conditions. A refers to raw broccoli puree, B refers to fermented raw broccoli puree, C refers to preheated broccoli puree without glucono-delta-lactone, D refers to preheated broccoli puree with glucono-delta-lactone, E refers to preheated fermented broccoli puree. Time 0 designates samples just after processing. Reported values are means of three replicate samples and error bars represent standard deviation values. | |
Conclusion
The results of this study for the first time showed that pre-heating coupled with lactic acid bacteria fermentation substantially enhance the sulforaphane content of broccoli-based products. In-pack pre-heating treatment of broccoli florets at 65 °C for 3 min followed by maceration and fermentation resulted in as much as ∼16 times increase in the yield of sulforaphane compared to raw broccoli puree. The sulforaphane content in the pre-heated fermented puree remained stable (93% retention) during storage at 4 °C for two weeks. On the other hand, chemical acidification of broccoli puree using glucono-delta-lactone did not improve sulforaphane yield nor its stability indicating that the positive impact of fermentation is perhaps due to the enhanced release of glucoraphanin from the broccoli matrix by the combined action of mild heat and microbial cell wall degrading enzymes. Further studies are required to confirm this hypothesis. The combined preheating-fermentation process enables the production of safe and functional broccoli products enriched with sulforaphane with potential health benefits.
Funding
The first author of this work was supported by National Key R&D Program of China (No. 2016YFD0400203) and Institute of Science and Technology Innovation, DGUT (No. KCYCXPT2017007).
Conflicts of interest
There are no conflicts of interest to declare.
References
- C. Manach, D. Milenkovic, T. Van de Wiele, A. Rodriguez-Mateos, B. de Roos, M. Teresa Garcia-Conesa, R. Landberg, E. R. Gibney, M. Heinonen, F. Tomas-Barberan and C. Morand, Addressing the inter-individual variation in response to consumption of plant food bioactives: Towards a better understanding of their role in healthy aging and cardiometabolic risk reduction, Mol. Nutr. Food Res., 2017, 61(6), 1600557 CrossRef PubMed.
- J. Vanamala, Food systems approach to cancer prevention, Crit. Rev. Food Sci. Nutr., 2017, 57, 2573–2588 CrossRef CAS PubMed.
- D. Lippmann, C. Lehmann, S. Florian, G. Barknowitz, M. Haack, I. Mewis, M. Wiesner, M. Schreiner, H. Glatt, R. Brigelius-Flohe and A. P. Kipp, Glucosinolates from pak choi and broccoli induce enzymes and inhibit inflammation and colon cancer differently, Food Funct., 2014, 5, 1073–1081 RSC.
- D. A. Moreno, M. Carvajal, C. Lopez-Berenguer and C. Garcia-Viguera, Chemical and biological characterisation of nutraceutical compounds of broccoli, J. Pharm. Biomed. Anal., 2006, 41, 1508–1522 CrossRef CAS PubMed.
- A. Raiola, A. Errico, G. Petruk, D. M. Monti, A. Barone and M. M. Rigano, Bioactive Compounds in Brassicaceae Vegetables with a Role in the Prevention of Chronic Diseases, Molecules, 2018, 23(1), 15 CrossRef PubMed.
- D. Van Eylen, N. Bellostas, B. W. Strobel, I. Oey, M. Hendrickx, A. Van Loey, H. Sorensen and J. C. Sorensen, Influence of pressure/temperature treatments on glucosinolate conversion in broccoli (Brassica oleraceae L. cv Italica) heads, Food Chem., 2009, 112, 646–653 CrossRef CAS.
- H. Bessler and M. Djaldetti, Broccoli and human health: immunomodulatory effect of sulforaphane in a model of colon cancer, Int. J. Food Sci. Nutr., 2018, 69, 946–953 CrossRef CAS PubMed.
- K. A. Jhang, J.-S. Park, H.-S. Kim and Y. H. Chong, Sulforaphane rescues amyloid-beta peptide-mediated decrease in MerTK expression through its anti-inflammatory effect in human THP-1 macrophages, J. Neuroinflammation, 2018, 15(1), 75 CrossRef PubMed.
- S.-K. Ku and J.-S. Bae, Antithrombotic activities of sulforaphane via inhibiting platelet aggregation and FIIa/FXa, Arch. Pharmacal Res., 2014, 37, 1454–1463 CrossRef CAS PubMed.
- B. Patel, G. E. Mann and S. J. Chapple, Concerted redox modulation by sulforaphane alleviates diabetes and cardiometabolic syndrome, Free Radicals Biol. Med., 2018, 122, 150–160 CrossRef CAS PubMed.
- J. W. Fahey, S. L. Wehage, W. D. Holtzclaw, T. W. Kensler, P. A. Egner, T. A. Shapiro and P. Talalay, Protection of Humans by Plant Glucosinolates: Efficiency of Conversion of Glucosinolates to Isothiocyanates by the Gastrointestinal Microflora, Cancer Prev. Res., 2012, 5, 603–611 CrossRef CAS PubMed.
- J. W. Fahey, W. D. Holtzclaw, S. L. Wehage, K. L. Wade, K. K. Stephenson and P. Talalay, Sulforaphane Bioavailability from Glucoraphanin-Rich Broccoli: Control by Active Endogenous Myrosinase, PLoS One, 2015, 10, e0140963 CrossRef PubMed.
- E. B. Dosz and E. H. Jeffery, Commercially produced frozen broccoli lacks the ability to form sulforaphane, J. Funct. Foods, 2013, 5, 987–990 CrossRef CAS.
- G. C. Wang, M. Farnham and E. H. Jeffery, Impact of Thermal Processing on Sulforaphane Yield from Broccoli (Brassica oleracea L. ssp italica), J. Agric. Food Chem., 2012, 60, 6743–6748 CrossRef CAS PubMed.
- N. V. Matusheski, R. Swarup, J. A. Juvik, R. Mithen, M. Bennett and E. H. Jeffery, Epithiospecifier protein from broccoli (Brassica oleracea L. ssp italica) inhibits formation of the anticancer agent sulforaphane, J. Agric. Food Chem., 2006, 54, 2069–2076 CrossRef CAS PubMed.
- F. S. Hanschen, E. Lamy, M. Schreiner and S. Rohn, Reactivity and Stability of Glucosinolates and Their Breakdown Products in Foods, Angew. Chem., Int. Ed., 2014, 53, 11430–11450 CrossRef CAS PubMed.
- D. Van Eylen, I. Oey, M. Hendrickx and A. Van Loey, Kinetics of the stability of broccoli (Brassica oleracea cv. Italica) myrosinase and isothiocyanates in broccoli juice during pressure/temperature treatments, J. Agric. Food Chem., 2007, 55, 2163–2170 CrossRef CAS PubMed.
- Z. X. Gu, Q. H. Guo and Y. J. Gu, Factors Influencing Glucoraphanin and Sulforaphane Formation in Brassica Plants: A Review, J. Integr. Agric., 2012, 11, 1804–1816 CrossRef CAS.
- K. P. Latte, K. E. Appel and A. Lampen, Health benefits and possible risks of broccoli - an overview, Food Chem. Toxicol., 2011, 49, 3287–3309 CrossRef CAS PubMed.
- N. V. Matusheski and E. H. Jeffery, Comparison of the bioactivity of two glucoraphanin hydrolysis products found in broccoli, sulforaphane and sulforaphane nitrile, J. Agric. Food Chem., 2001, 49, 5743–5749 CrossRef CAS PubMed.
- F. Kupke, C. Herz, F. S. Hanschen, S. Platz, G. A. Odongo, S. Helmig, M. M. Bartolomé Rodríguez, M. Schreiner, S. Rohn and E. Lamy, Cytotoxic and genotoxic potential of food-borne nitriles in a liver in vitro model, Sci. Rep., 2016, 6, 37631 CrossRef CAS PubMed.
- N. V. Matusheski, J. A. Juvik and E. H. Jeffery, Heating decreases epithiospecifier protein activity and increases sulforaphane formation in broccoli, Phytochemistry, 2004, 65, 1273–1281 CrossRef CAS PubMed.
- Q. H. Guo, L. P. Guo, Z. Y. Wang, Y. Zhuang and Z. X. Gu, Response surface optimization and identification of isothiocyanates produced from broccoli sprouts, Food Chem., 2013, 141, 1580–1586 CrossRef CAS PubMed.
- F. S. Hanschen, A. Bauer, I. Mewis, C. Keil, M. Schreiner, S. Rohn and L. W. Kroh, Thermally Induced Degradation of Aliphatic Glucosinolates: Identification of Intermediary Breakdown Products and Proposed Degradation Pathways, J. Agric. Food Chem., 2012, 60, 9890–9899 CrossRef CAS PubMed.
- Y. X. Cai, J. H. Wang, C. McAuley, M. A. Augustin and N. S. Terefe, Fermentation for enhancing the bioconversion of glucoraphanin into sulforaphane and improve the functional attributes of broccoli puree, J. Funct. Foods, 2019, 61, 103461 CrossRef CAS.
- Z. Li, Y. Liu, Z. Fang, L. Yang, M. Zhuang, Y. Zhang and P. Sun, Development and verification of sulforaphane extraction method in cabbage (Brassica oleracea L. var. capitata) and broccoli (Brassica oleracea L. var. italica Planch.), J. Med. Plants Res., 2012, 6, 4796–4803 CAS.
- C. Perez, H. Barrientos, J. Roman and A. Mahn, Optimization of a blanching step to maximize sulforaphane synthesis in broccoli florets, Food Chem., 2014, 145, 264–271 CrossRef CAS PubMed.
- S. K. Ghawi, L. Methven and K. Niranjan, The potential to intensify sulforaphane formation in cooked broccoli (Brassica oleracea var. italica) using mustard seeds (Sinapis alba), Food Chem., 2013, 138, 1734–1741 CrossRef CAS PubMed.
- N. S. Terefe, R. Buckow and C. Versteeg, Quality-Related Enzymes in Fruit and Vegetable Products: Effects of Novel Food Processing Technologies, Part 1: High-Pressure Processing, Crit. Rev. Food Sci. Nutr., 2014, 54, 24–63 CrossRef CAS PubMed.
- L. Ludikhuyze, L. Rodrigo and M. Hendrickx, The activity of myrosinase from broccoli (Brassica oleracea L. cv. Italica): Influence of intrinsic and extrinsic factors, J. Food Prot., 2000, 63, 400–403 CrossRef CAS PubMed.
-
H. Claus, Extra cellular enzymes and peptides of lactic acid bacteria: Significance for vinification, 2007 Search PubMed.
- J.-H. Ye, L.-Y. Huang, N. S. Terefe and M. A. Augustin, Fermentation-based biotransformation of glucosinolates, phenolics and sugars in retorted broccoli puree by lactic acid bacteria, Food Chem., 2019, 286, 616–623 CrossRef CAS PubMed.
- S. J. Franklin, S. E. Dickinson, K. L. Karlage, G. T. Bowden and P. B. Myrdal, Stability of sulforaphane for topical formulation, Drug Dev. Ind. Pharm., 2014, 40, 494–502 CrossRef CAS PubMed.
|
This journal is © The Royal Society of Chemistry 2020 |