DOI:
10.1039/C9FO00627C
(Paper)
Food Funct., 2020,
11, 585-595
The anti-inflammatory effects of low- and high-molecular-weight beta-glucans from Agrobacterium sp. ZX09 in LPS-induced weaned piglets
Received
26th March 2019
, Accepted 5th December 2019
First published on 10th December 2019
Abstract
The physicochemical characteristics of beta-glucans determine the immune responses of the intestines and whole body. It is hypothesized that glucans with different molecular weights have diverse modes of action on LPS-mediated immune activity. This study aimed to verify the immune-modulatory effects of two types of beta-glucans in LPS-induced weaned piglets. The results indicated that dietary beta-glucan supplementation could prevent losses in body weight gain caused by LPS challenge. Supplementation with different molecular weights of beta-glucans decreased the production of IL-1β and TNF-α and increased IL-10 production, which is likely associated with key factors such as TLR4 and NF-κB. High-molecular-weight beta-glucans seemed to have a strong functional capacity to modulate the innate immune response through the Dectin-1 receptor. Therefore, the results indicate that supplementing piglets with Agrobacterium sp. ZX09 beta-glucans inhibits LPS-mediated depression in the growth performance and plays a protective role during LPS challenge possibly via the Dectin-1 receptor and the TLR4/NF-κB pathway. The results reveal the potential therapeutic activity of purified Agrobacterium sp. ZX09 beta-glucan following experimental LPS infusion.
Introduction
Carbohydrates have key roles in a broad range of biological processes, including transduction and immune responses. Thus, studies of the relationship between their structures and functions are of particular importance to clarify the mystery of the roles of polysaccharides in life-associated events.1 As a component of potent biological response modifiers (BRMs), beta-glucans are derived from yeast, fungi, grains and seaweed. They have shown therapeutic benefits in a variety of animal disease models such as diabetes, cardiovascular disease, hypertension and other prevailing diseases.2 Dietary beta-glucan supplementation has been proven to modulate the immune system, influence growth characteristics and thus enhance disease resistance in farmed animals, particularly in weaned piglets, which are at a vulnerable stage for pig survival.3 The work published so far has shown no consistent effects of dietary glucans on the immune response of pigs.4 The divergent results might be caused by the quality of the relatively unknown glucans used in different studies. Additionally, no further information on glucans has been provided other than the fact that they are of Saccharomyces cerevisae origin.5–7
The physical characteristics and biological activities of beta-glucans are largely dependent on their primary structure, solubility, degree of branching, purity, and molecular weight.8,9 Compared with soluble beta-glucans, particulate beta-glucans enhance stronger immune responses.10 The alteration of the molecular structure or decrease in the molecular weight enhances the antioxidant properties of the molecule.11,12 However, few studies have compared the immune responses of beta-glucans having distinct molecular weights.
The immune effects of beta-glucans on pattern recognition receptors (PRRs) including Dectin-1, complement receptor 3 and Toll-like receptors (TLRs) have been confirmed and documented.13,14 Dectin-1 is considered as the key receptor for beta-glucans.15 TLRs (TLR2, 4 and 6) respond to beta-glucans by co-binding with Dectin-1.16 The binding of beta-glucans to Dectin1-TLRs induces the release of pro-inflammatory cytokines.17 The physicochemical characteristics of beta-glucans determine the mode of action on modulating immune responses through the interplay between Dectin-1 and TLRs.18 Compared with soluble beta-glucans, particulate beta-glucans lead to the strong activation of Dectin-1 and subsequently stimulate the release of pro-inflammatory cytokines such as TNF-α.19 However, despite these observations, there is a lack of knowledge on whether beta-glucans with different molecular weights have divergent immunological effects on binding to Dectin-1 and co-acting with TLRs.
Lipopolysaccharide (LPS) infusion can reduce the growth performance in farm animals, particularly in post-weaned pigs, mainly due to its negative effects of activating oxidative stress and increasing inflammatory cytokine secretion.20 Moreover, LPS leads to activated NF-κB signaling via TLR4, which regulates a number of pro-inflammatory genes and increases the secretion of pro-inflammatory cytokines.21 As the main absorbing region in the gastrointestinal tract, the jejunum is the main target tissue for LPS infection-induced inflammation.22 Taking this into account, the focus of this study is to determine the specific receptor-mediated immunomodulation function elicited by preparations of Agrobacterium ZX09 beta-glucan with different molecular weights (2000 kDa versus 300 kDa), including the effects on growth performance and anti-inflammatory parameters related to the TLRs/NF-κB signal pathway in the jejunal mucosa of weaned piglets within an LPS-induced model.
Materials and methods
All animal procedures were performed in accordance with the Guidelines for Care and Use of Laboratory Animals of Sichuan Agricultural University and approved by the Animal Ethical and Welfare Committee (AEWC) of China (Approval No. 18-2086).
Animals and diets
A total of 32 crossbred (Duroc × large white × landrace) barrows weaned at 21 d of age were housed individually in a metabolic cage (1.5 m × 0.7 m × 1.0 m) for a period of 4 weeks with water and diets available ad libitum. The room temperature was kept at approximately 30 °C in the first week and was gradually reduced until it reached 24 °C. Body weights and feed consumption were recorded at days 0, 21 and 28 to calculate the growth performance, including ADG, ADFI and F
:
G.
Agrobacterium sp. ZX09 (Salecan®) used in this study was isolated from a soil sample from the ocean coast, and kindly provided by Synlight Bio Co. Ltd of Sichuan, China. The beta-glucan was further purified according to previously described methods.23 The total sugar contents of the fraction were determined by the phenol-sulfuric acid method, using glucose for the standard curve,24 and the purity of beta-glucan was determined using a test kit (Megazyme, Ireland). The chemical composition of Agrobacterium sp. ZX09 beta-glucan is presented in Table 1. The average molecular weights of HG and LG were 2000 kDa and 300 kDa, respectively. The diets were formulated according to NRC requirements for pigs and supplemented with 50 mg kg−1 high (HG) or low (LG) molecular weight beta-glucans, respectively. The basal dietary composition is shown in Table 2.
Table 1 Chemical content of beta-glcuan preparations
|
Protein (%) |
Starch (%) |
Crude fat (%) |
Beta-glucan content (%) |
Moisture (%) |
ZX09-HG: High molecular weight of Agrobacterium sp. ZX09 beta-glucan. ZX09-LG: Low molecular weight of Agrobacterium sp. ZX09 beta-glucan. |
ZX09-HG |
1.9 |
0.1 |
0.2 |
90.1 |
5.0 |
ZX09-LG |
1.5 |
0.1 |
0.1 |
92.4 |
4.7 |
Table 2 The composition and nutrient content of the basal diet
Ingredients |
Composition, % |
Provided the following per kg of diet: vitamin A, 9000 IU; vitamin D3, 3000 IU; vitamin E, 20 IU; vitamin K3, 3.0 mg; vitamin B1, 1.5 mg; vitamin B2, 4.0 mg; vitamin B12, 0.2 mg; nicotonic, 30 mg; pantothenic, 15 mg; folic acid, 0.75 mg; biotin, 0.1 mg.
Provided the following per kg of diet: Fe (FeSO4·7H2O),100 mg; Cu (CuSO4·5H2O), 6 mg; Zn (ZnSO4·7H2O), 100 mg; Mn (MnSO4·H2O), 4 mg; Se (Na2SeO3·5H2O), 0.3 mg; I (KI), 0.14 mg.
Calculated values.
Measured values.
|
Corn |
29.02 |
Extruded corn |
29.02 |
Fish meal |
5.00 |
Whey powder |
3.00 |
Soybean meal |
13.00 |
Extruded full-fat soybean |
12.00 |
Soy protein concentrate |
4.50 |
D-Glucose |
2.00 |
L-Lysine·HCL (78%) |
0.19 |
L-Threonine (98.5%) |
0.05 |
DL-Methionine (99%) |
0.15 |
Choline chloride |
0.15 |
Sodium chloride |
0.30 |
Calcium carbonate |
0.54 |
Dicalcium phosphate |
0.75 |
Vitamin premixa |
0.03 |
Mineral premixb |
0.30 |
Nutrient composition, g kg−1 |
|
Digestible energyc, MJ kg−1 |
14.32 |
Crude proteind |
20.54 |
Total lysined |
1.56 |
Total methionine and cystinec |
0.74 |
Total tryptophanc |
0.21 |
Total threonined |
0.90 |
Calciumc |
0.81 |
Phosphorus availablec |
0.43 |
Experimental design
Based on the initial body weight (6.71 ± 0.03 kg), thirty-two weaned piglets were randomly assigned to one of three dietary groups supplemented with 0 mg kg−1 beta-glucan (n = 16), 50 mg kg−1 HG (n = 8) and 50 mg kg−1 LG (n = 8) for 21 days. On day 22, half of the piglets (n = 8) on the basal diet received an intramuscular injection (150 μg per kg BW) of LPS from Escherichia coli (Sigma-Aldrich, O55: B5, St Louis, MO, USA), while the other half (n = 8) on the basal diet were injected with sterile saline solution. All piglets in the HG (n = 8) and LG (n = 8) groups were infused with the same LPS as in the negative control group. No medical treatments or antibiotics were used throughout the course of the entire experiment.
On day 28, following weighing, blood samples of 20 mL were obtained via the jugular vein. The cell counts of erythrocytes, leucocytes, and lymphocytes were assayed by an automatic blood analyzer (HITACH 3100, Japan). Following the blood sampling, the piglets were euthanized by an intracardial injection of Na pentobarbital (50 mg per kg body weight) and jugular exsanguinations. The small intestine was then removed and the jejunum (proximal half of the small intestine) was quickly isolated and flushed with ice-cold saline. The tissues of jejunal mucosa were collected by scraping the intestinal wall with a glass microscope slide, frozen in liquid nitrogen and stored at −80 °C until ELISA, RT-PCR and Western Blot analysis.
Lymphocyte subpopulation assay
Whole blood with EDTA-K2 treatment was collected in 1.5 mL Eppendorf tubes and centrifuged at 200g for 5 min. The supernatant was discarded and lymphocytes were collected. The cell concentration was diluted to 1.0 × 106 cells per mL with phosphate-buffered saline (PBS), then 100 μL cell suspensions were transferred into another centrifuge tube and respectively stained with 5 μL anti-porcine CD4 (SouthernBiotech, Birmingham, AL, USA) and anti-porcine CD8 (SouthernBiotech, Birmingham, AL, USA) for 20 min at room temperature. Next, 1× RBC lysis buffer was added and centrifugal elutriation was performed once. The cells were resuspended in 0.5 mL PBS and the percentages of CD4 and CD8 cell counts were determined using a Beckman Cytoflex flow cytometer.
Cytokine concentration in serum
Concentrations of interleukin (IL)-1β, IL-10 and TNF (tumor necrosis factor)-α in serum were measured using commercially available ELISA kits (R&D Systems Inc., Minneapolis, MN) for pigs, with a within-assay CV of less than 10% for all 3 assays. The assays were analyzed colorimetrically using a BioTek Synergy HT microplate reader (BioTek Instruments, Winooski, VT).
mRNA expression of Dectin-1, TLRs and cytokines in the jejunal mucosa
Total RNA was extracted from snap-frozen jejunal mucosa samples using TRIzol reagent (Invitrogen, Carlsbad, CA) and quantified by measuring the absorption at 260 nm. The RT-PCR procedure was based on a previously established method.25 The RNA was reverse-transcribed using a high-capacity cDNA reverse transcription kit (Applied Biosystems, Foster City, CA) with a 3 μg RNA sample according to the manufacturer's instructions and then amplified by PCR using gene-specific primers (Table 3). Amplification was performed using SYBR green (Applied Biosystems Inc.) by an Option DNA Engine (Bio-Rad), with the following PCR amplification conditions: 95 °C for 10 s, 40 cycles at 95 °C for 5 s, 60 °C for 25 s, followed by a final single extension step of 72 °C for 5 min. A melting curve analysis was generated after each quantitative real-time PCR assay to check and verify the specificity and purity of all PCR products, which were further checked for size and specificity by agarose gel electrophoresis. The relative standard curve method was used for quantification. β-Actin was used as the internal standard reporter gene.
Table 3 Primers used for quantitative RT-PCR
Gene product |
Forward primer(5′ to 3′) |
Reverse primer(5′ to 3′) |
GenBank accession no. |
IL-1β |
AACGTGCAGTCTATGGAGT |
GAACACCACTTCTCTCTTCA |
NM214055.1 |
IL-6 |
CTGGCAGAAAACAACCTGAACC |
TGATTCTCATCAAGCAGGTCTCC |
AB194100.1 |
IL-10 |
CAGATGGGCGACTTGTTG |
ACAGGGCAGAATTGATGAC |
L20001 |
TNF-α |
AACCTCAGATAAGCCCGTCG |
ACCACCAGCTGGTTGTCTTT |
EU682384.1 |
TLR4 |
TCTACATCAAGTGCCCCTAC |
TAAATTCTCCCAAAACCAAC |
NC_010443 |
Dectin-1 |
CTCTCACAACCTCACCAGGAGAT |
CAGTAATGGGTCGCCAATAAGG |
FJ386384.1 |
β-Actin |
CACGCCATCCTGCGTCTGGA |
AGCACCGTGTTGGCGTAGAG |
DQ845171.1 |
Protein immunoblot analysis for NF-κB
Protein levels for the NF-κB and β-actin in the jejunal mucosa were determined by Western blot analysis as described previously.26 Briefly, the proteins were separated by electrophoresis and transferred to polyvinyl difluoride membranes. The membranes were blocked with Tris-buffered saline (pH 7.0) containing 5% bovine serum albumin and 0.05% (v/v) Tween-20. The membranes were probed with anti-phospho-NF-κB or total-NF-κB (1
:
1000) antibodies (Santa Cruz, CA). After the primary antibody incubation, the membranes were washed and then incubated with horseradish peroxidase-conjugated anti-rabbit immunoglobulin G (1
:
1000). Immunoreactive protein bands were determined using enhanced chemiluminescence reagents and the membranes were exposed on Kodak X-Omat film (Rochester, NY, USA).
DNA extraction and real-time PCR analysis of colonic bacteria
The genomic DNAs were extracted from 0.1 g of digesta samples using a commercially available rapid bacterial genomic DNA isolation kit (Sangon Biotech, Shanghai, China) for pigs. Analysis of colonic bacteria was conducted according to the method previously described.27 Based on the genetic sequence of the 16S rRNA of bacteria, the fluorescent quantitative specific primers and probe designed for Bifidobacterium, Lactobacillus, Bacillus, and Escherichia coli are presented in Table 4. The copy numbers of total bacteria, Bifidobacterium, Lactobacillus, Bacillus, and Escherichia coli in the colonic samples were quantified by real-time PCR on a Bio-Rad CFX96 real-time system (Bio-Rad, Hercules, CA, USA) with optical-grade 96-well plates. The program of reaction was as follows: 95 °C for 10 s, 40 cycles at 95 °C for 5 s, 50–60 °C for 25 s, followed by 95 °C for 10 s. A melting curve analysis was generated after each quantitative real-time PCR assay to check and verify the specificity and purity of all PCR products: 40 cycles at 95 °C for 39 s, 55 °C for 1 min, and 95 °C for 1 min. Results are presented as l g copy numbers per gram of dry digesta.
Table 4 Primers/probes for real-time PCR of bacteria
Item |
Primers/probes and sequence (5′–3′) |
Product length |
Total bacteria |
Eub338F: ACTCCTACGGGAGGCAGCAG |
200 bp |
Eub518R: ATTACCGCGGCTGCTGG |
Lactobacillus
|
F: GAGGCAGCAGTAGGGAATCTTC |
126 bp |
R: CAACAGTTACTCTGACACCCGTTCTTC |
P: (FMA)AAGAAGGGTTTCGGCTCGTAAAACTCTGTT(BHQ-1) |
Bifidobacterium
|
F: CGCGTCCGGTGTGAAAG |
121 bp |
R: CTTCCCGATATCTACACATTCCA |
P: (FMA) ATTCCACCGTTACACCGGGAA(BHQ-1) |
Bacillus
|
F: GCAACGAGCGCAACCCTTGA |
92 bp |
R: TCATCCCCACCTTCCTCCGGT |
P: (FMA)CGGTTTGTCACCGGCAGTCACCT(BHQ-1) |
Escherichia coli
|
F: CATGCCGCGTGTATGAAGAA |
96 bp |
R: CGGGTAACGTCAATGAGCAAA |
P: (FMA)AGGTATTAACTTTACTCCCTTCCTC(BHQ-1) |
Statistical analysis
The data were analyzed by one-way analysis of variance (ANOVA) using the GLM procedure of SAS (release 9.0; SAS Institute). If a significant treatment effect was observed, the significance between the treatment differences was identified by Duncan's multiple comparisons test. Normal distribution was confirmed. Results were expressed as treatment means with their pooled SEM. A probability value of P < 0.05 was considered statistically significant.
Results
Effects of beta-glucans on the growth performance of the weaned piglets
Beta-glucans improved the growth performance of the weaned piglets in LPS-challenged and non-challenged status (Table 5). During days 1–21 of the trial, the average daily gain (ADG) of the piglets was markedly improved in the LG group as compared with the control group (P < 0.05). Average daily feed intake (ADFI) was higher in the LG and HG groups when compared to the control group (P < 0.05). The F
:
G ratio was similar among dietary groups (p > 0.05). During days 22–28, there were no differences in ADFI in the LG or HG groups as compared to the control group (p > 0.05). However, LPS infusion reduced the ADG of piglets (P < 0.05), while LG and HG treatment attenuated the LPS-induced ADG decrease. Similarly, the F
:
G ratio was improved while treated with LG and HG in the LPS-challenged piglets (P < 0.05).
Table 5 Growth performance of piglets fed diets with different molecular weight beta-glucan
Item |
Control |
LPS |
LGa |
HGb |
SEM |
P-Value |
LG, experimental group fed with low molecular weight beta-glucan.
HG, experimental group fed with high molecular weight beta-glucan.
ADG: Average daily gain.
ADFI: Average daily feed intake.
F/G: Feed intake/daily gain.
a,bMean values within a row with unlike superscript letters were significantly different (P < 0.05). |
Initial BW (kg) |
6.71 |
6.71 |
6.71 |
6.71 |
0.03 |
0.970 |
Final BW (kg) |
18.38a |
15.87b |
17.68a |
18.25a |
0.18 |
0.041 |
1–21 d |
ADGc (g) |
305.56a |
— |
371.56b |
348.89ab |
29.12 |
0.048 |
ADFId (g) |
538.73a |
— |
597.04b |
582.93b |
24.73 |
0.039 |
F/Ge |
1.78 |
— |
1.63 |
1.67 |
0.12 |
0.338 |
22–28 d |
ADG (g) |
415.18a |
325.71b |
390.63a |
411.52a |
27.47 |
0.035 |
ADFI (g) |
656.86 |
602.56 |
621.10 |
637.86 |
29.35 |
0.550 |
F/G |
1.60a |
1.88b |
1.58a |
1.56a |
0.09 |
0.027 |
Effects of beta-glucans on blood cell counts and blood leukocyte population in the weaned piglets
LPS challenge and beta-glucan ingestion changed the cellular immunity of the weaned piglets as indicated by blood cell counts (Table 6). LPS challenge had no influence on erythrocytes, but increased leucocytes in the blood cells (P < 0.05). Piglets fed LG or HG-containing diets had higher levels of leucocytes after LPS infusion than piglets fed the control diet (P < 0.05). Furthermore, lymphocytes were promoted after LPS injection and maintained at high levels when the piglets were fed the LG-containing diet (P < 0.05).
Table 6 Blood cell counts of piglets fed diets with different molecular weight beta-glucan
Item |
Control |
LPS |
LPS + LGa |
LPS + HGb |
SEM |
P-Value |
LPS + LG, LPS injection group fed with low molecular weight beta-glucan.
LPS + HG, LPS injection group fed with high molecular weight beta-glucan.
Proportion of total white blood cell.
a,bMean values within a row with unlike superscript letters were significantly different (P < 0.05). |
Erythrocytes (×106 ml−1) |
7.20 |
7.14 |
7.12 |
7.16 |
0.11 |
0.476 |
Leucocytes (×103 ml−1) |
13.62a |
33.36b |
31.78b |
27.54b |
2.32 |
0.012 |
Lymphocytesc (%) |
40.26a |
59.05b |
55.05b |
49.43ab |
2.65 |
0.023 |
The lymphocyte populations are shown in Table 7. Blood leukocyte populations were positively modified by beta-glucans after LPS infusion. Counts of CD4 and CD8 positive cells were increased following the LPS challenge (P < 0.05). Piglets fed LG had a higher frequency of CD4 and CD8 positive cells than the control pigs (P < 0.05). Furthermore, the LPS challenge caused a significant decrease in the ratio of CD4:CD8 (P < 0.05), which was effectively prevented with dietary HG treatment.
Table 7 Lymphocyte subpopulation in piglets fed diets with different molecular weight beta-glucan
Item |
Control |
LPS |
LPS + LGa |
LPS + HGb |
SEM |
P-Value |
LPS + LG, LPS injection group fed with low molecular weight beta-glucan.
LPS + HG, LPS injection group fed with high molecular weight beta-glucan.
a,bMean values within a row with unlike superscript letters were significantly different (P < 0.05). |
CD4+ (%) |
15.75a |
27.66b |
36.76b |
32.25b |
0.98 |
0.014 |
CD8+ (%) |
19.20a |
58.51b |
64.18b |
40.82b |
0.61 |
0.009 |
CD4+ : CD8+ |
0.82a |
0.47b |
0.57b |
0.79a |
0.06 |
0.047 |
Effects of beta-glucans on cytokine production in the jejunal mucosa and serum of weaned piglets
In the present study, increased concentrations of IL-1β and TNF-α in the serum of piglets were observed with the LPS challenge (Table 8). Beta-glucan ingestion attenuated the enhanced concentrations of pro-inflammatory cytokines and further increased anti-inflammatory cytokine IL-10 after LPS stimulation (Table 8). Cytokine transcripts in the jejunum were also determined (Fig. 1). The LPS challenge increased the transcript levels of IL-1 (Fig. 1A), IL-6 (Fig. 1B), IL-10 (Fig. 1C), and TNF-α (Fig. 1D) in the jejunal mucosa of weaned piglets (P < 0.05). LG and HG-supplemented diets could down-regulate transcripts of IL-1β (Fig. 1A) and TNF-α (Fig. 1D) and stimulated anti-inflammatory cytokine IL-10 expression (Fig. 1C) in the piglets after LPS infusion. Interestingly, the HG-supplemented diet caused a further decrease in IL-6 expression (P < 0.05) (Fig. 1B). No difference in cytokine expression was detected when comparing the LG and HG supplemented groups.
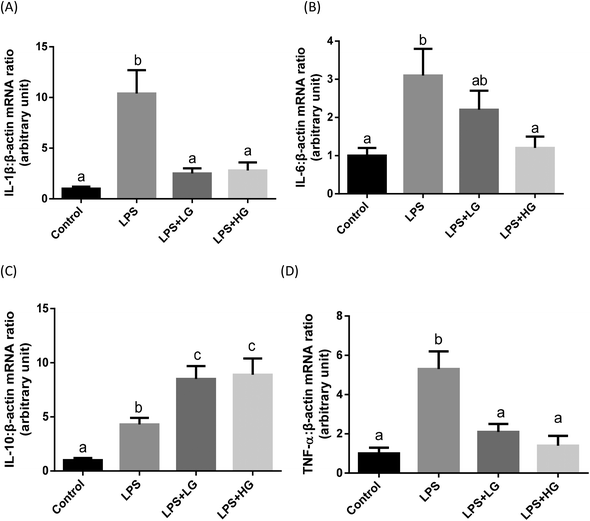 |
| Fig. 1 mRNA expression levels of cytokines in the jejunal mucosa of piglets fed diets with different molecular weight beta-glucans. Data are shown as means ± SE. (A) Interleukin-1β (IL-1β); (B) Interleukin-6 (IL-6); (C) Interleukin-10 (IL-10); (D) tumour necrosis factor α (TNF-α). a,b Bars with different superscript letters are significantly different (P < 0.05). LPS + LG: LPS injection group fed with low molecular weight beta-glucan; LPS + HG: LPS injection group fed with high molecular weight beta-glucan. | |
Table 8 Cytokine concentrations in serum of piglets fed diets with different molecular weight beta-glucan
Item |
Control |
LPS |
LPS + LGa |
LPS + HGb |
SEM |
P-Value |
LPS + LG, LPS injection group fed with low molecular weight beta-glucan.
LPS + HG, LPS injection group fed with high molecular weight beta-glucan.
a,bMean values within a row with unlike superscript letters were significantly different (P < 0.05). |
TNF-α (pg ml−1) |
126.25b |
165.59a |
118.71b |
125.25b |
6.78 |
0.027 |
IL-1β (ng L−1) |
11.20b |
13.60a |
10.65b |
11.29b |
0.31 |
0.018 |
IL-10 (ng L−1) |
14.66b |
17.50ab |
21.15a |
20.79a |
0.36 |
0.047 |
Effects of beta-glucans on Dectin-1 and TLRs/NF-κB expression in the jejunal mucosa of weaned piglets
Dectin-1 expression was determined in both LG and HG-treated groups. Its mRNA level was promoted by the addition of HG to the diet but not in the LG treated samples when piglets were challenged with LPS (P < 0.05) (Fig. 2A). As shown in this study, LPS could improve TLR4 (Fig. 2B), phosphorylated NF-κB p65 (Fig. 3A) and the ratio of phosphorylated NF-κB p65 to total NF-κB p65 (Fig. 3C) (P < 0.05). Treatment with LG and HG down-regulated the mRNA level of TLR4 to normal levels as compared to the untreated control (Fig. 2B). Moreover, the addition of beta-glucans effectively inhibited the LPS treatment-enhanced phosphorylated NF-κB p65 level (Fig. 3A) as well as the ratio of phosphorylated NF-κB p65 to total NF-κB p65 level in the jejunal mucosa (P < 0.05) (Fig. 3C).
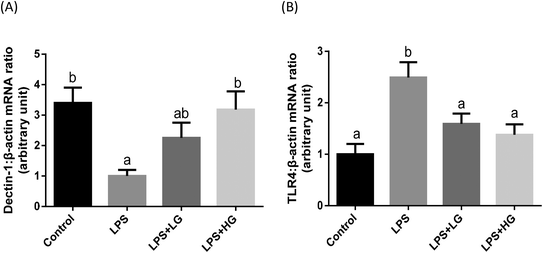 |
| Fig. 2 mRNA expression level of Dectin-1 and TLR4 in the jejunal mucosa of piglets fed diets with different molecular weight beta-glucans. Data are shown as means ± SE. (A) Dectin-1; (B) toll-like receptor 4 (TLR4). a,b Bars with different superscript letters are significantly different (P < 0.05). LPS + LG: LPS injection group fed with low molecular weight beta-glucan; LPS + HG: LPS injection group fed with high molecular weight beta-glucan. | |
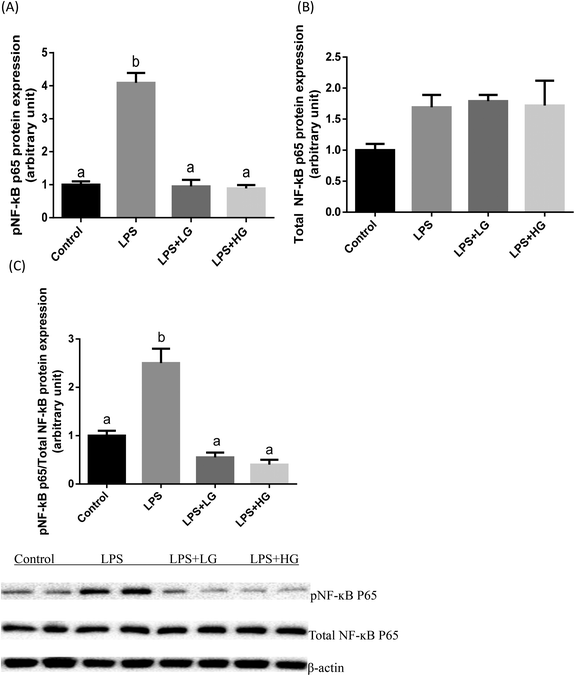 |
| Fig. 3 Protein levels of pNF-κB and total NF-κB P65 in the jejunal mucosa of piglets fed diets with different molecular weight beta-glucans. Data are shown as means ± SE. (A) Phosphorylated NF-κB P65. (B) Total NF-κB P65. (C) Ratio of phosphorylated NF-κB P65 to total NF-κB P65. a,b Bars with different superscript letters are significantly different (P < 0.05). LPS + LG: LPS injection group fed with low molecular weight beta-glucan; LPS + HG: LPS injection group fed with high molecular weight beta-glucan. | |
Quantitative difference in bacterial groups
Quantitative PCR revealed that piglets challenged by LPS had decreased numbers of Lactobacillus, Bifidobacterium, Bacillus, and total bacteria as compared to the control group (P < 0.05 or P < 0.01), but it showed increased numbers of Escherichia coli (P < 0.05) (Table 9). In addition, dietary β-glucan supplementation attenuated the impact of LPS infusion on the copy numbers of the assessed bacterial groups, particularly following HG ingestion. Compared with the control group, HG treatment increased the number of Bifidobacterium and Bacillus in colonic digesta samples of LPS-challenged piglets (P < 0.05), whereas dietary supplementation of LG did not show this kind of positive efficacy.
Table 9 Colonic microflora of piglets fed different molecular weight β-glucan (unit: Lg copies per gram of dry digesta)
Item |
Control |
LPSa |
LPS + LGb |
LPS + HG |
SEM |
p-Value |
LPS + LG, LPS injection group fed with low molecular weight beta-glucan.
LPS + HG, LPS injection group fed with high molecular weight beta-glucan.
a,bMean values within a row with unlike superscript letters were significantly different (P < 0.05). |
Lactobacillus
|
8.27a |
7.73 |
8.05b |
8.25a |
0.12 |
0.012 |
Bifidobacterium
|
5.66b |
5.02d |
5.33 |
6.03a |
0.28 |
<0.01 |
Bacillus
|
8.79b |
8.45 |
8.70b |
9.47a |
0.08 |
<0.01 |
Escherichia coli
|
7.47b |
8.08a |
7.99a |
7.42b |
0.15 |
0.011 |
Total bacteria |
11.16a |
9.61 |
10.75b |
11.18a |
0.20 |
<0.01 |
Discussion
Novel high purity, water-soluble extracellular beta-glucans of low and high molecular weights were produced in the present study. Similarly, in previous research, beta-glucans with two different molecular weights were obtained from oats.28,29 Beta-glucan from Agrobacterium sp. ZX09 had a higher purity as compared to the fractions obtained from oats. To our knowledge, this represents the first study investigating whether beta-glucan isolated from Agrobacterium sp. ZX09 could improve growth performance in weaned piglets, and comparing the immune-modulatory properties of beta-glucans with two different molecular weights.
Growth performance parameters are considered to be indicators of the severity of infection, and the negative effects of LPS injection on piglet growth performance are well established.30 Before LPS infusion, beta-glucans of low molecular weight effectively improved the growth performance of weaned piglets. After LPS injection, our results showed an impairment of growth performance parameters as a result of strong inflammatory responses. It is likely that nutrients are used for immune modulation in the challenged piglets instead of on growth performance. In the present study, beta-glucans attenuated the LPS-induced growth inhibition in weaned piglets regardless of the molecular weight. The results are inconsistent with previous studies that indicated that beta-glucan had no effects on non-immunochallenged piglets.31 The distinction could be partly related to the treatment concentration and the conformation and purity of beta-glucans used in the diet formulations. Our earlier reports strongly demonstrated that the optimal dosage of beta-glucan derived from Agrobacterium sp. ZX09 was 50 mg kg−1 of diet for weaned piglets (data not shown). In other studies the tested beta-glucans were variant and their sources were mainly obtained from the cell wall of Saccharomyces cerevisiae32 and purified from oats.33 In addition, the beta-glucan content in Agrobacterium sp. ZX09 was higher (>90%) than that in Saccharomyces cerevisiae and in oats (70–80%).
Changes in the CD4
:
CD8 ratio represent an initiation of the immune responses. CD8 T cells are recognized as Tc cells once they become activated and are generally classified as having a pre-defined cytotoxic role within the immune system.34 Upregulated CD4 and CD8 in the blood demonstrated that LPS may damage the balance of the immune systems in the challenged weaned piglets. Nevertheless, HG treatment resulted in the normalization of the CD4
:
CD8 ratio, possibly through the mechanism of activating cytotoxic T cells within a class-I-restricted antigen.34 The results were in line with the previous finding of increased lymphocyte proliferation activity after 14 days of beta-1,3/1,6-glucan administration.30 Interestingly, beta-glucans originating from oats and S. cerevisiae were not able to show immunomodulatory action in unchallenged animals.35 The differences seen in that study may be due to the different sources of beta-glucans, thus making it difficult to draw overall conclusions. Treg cells constitute an essential part in regulating innate and adaptive immune responses. Within the CD4+ T cell compartment, IL-10-producing type 1 regulatory (Tr1) cells are one of the best-understood subsets of Tregs.36 We hypothesize that Treg cells in beta-glucan-fed piglets may have the ability to control the innate immune response during jejunum injury.
Pro-inflammatory cytokines and anti-inflammatory cytokines work together to mutually affect the innate immune system. It is generally recognized that IL-1β, IL-6 and TNF-α are pro-inflammatory cytokines, which modulate immunity, regulate nutrient utilization, and depress the growth performance of postnatal animals.37 The cardinal anti-inflammatory cytokine IL-10 can inhibit T-cell proliferation, development and function, as well as inhibit the secretion of Th1- and Th2-type cytokines.38 IL-10 can also suppress the activity of the signal transduction of nuclear transcription factor κB, which is a major transcription factor of pro-inflammatory cytokines.39 In this study, beta-glucans, specifically those with high molecular weight, reduced pro-inflammatory cytokines and induced anti-inflammatory cytokine production by blocking LPS-induced inflammation. If feeding with beta-glucan decreases the secretion of pro-inflammatory cytokines and promotes the secretion of anti-inflammatory cytokines, then less activation of the immune system would be achieved. This hypothesis is also partially supported by previous research on yeast-isolated beta-glucan.40,41 However, this effect was non-significant, suggesting that the supplementation had marginal benefits with regards to cytokine production.35 With the exception of reducing the transforming growth factor β (TGF-β) mRNA in the lung, whole yeast beta-glucan particles have no influence on lung-associated adaptive immunity in piglets.42 Furthermore, yeast product supplementation in the diets of weaned piglets appears to increase the incidence of diarrhea and has adverse effects on intestinal immune function.35 Thus, we concluded that depending on the strain, species and preparation process, pure beta-glucans from Agrobacterium sp. ZX09 appear to be strong modulators of intestinal inflammation in the LPS model. Conversely, it appears that some beta-glucan fractions isolated from yeasts are not, and high molecular weight beta-glucan seems to display the most potent anti-inflammatory effect in comparison to low molecular weight beta-glucan.
Improved microbiota balance may explain one of the mechanisms behind the beneficial immune effects of beta-glucans. Special carbohydrates have dominant roles in shaping gut microbiota compositions and profiles of short-chain fatty acids (SCFA) in the intestine, which may account for lower mortality and a less frequency of several inflammatory diseases including inflammatory bowel disease and allergies. Manipulation of immune responses by improved microflora population and enhanced SCFA production have been demonstrated.43 Consistent with previous reports, our microbiological data showed that beta-glucans positively modified the profile of microbiota in the colon. Importantly, this study on beta-glucans with different molecular weights revealed that HG had more positive effects on Bifidobacterium and Bacillus than LG, supporting the concept of the improvement of bacterial population by glucans of high molecular weight. The direct relationship between microbiota analyses and immune responses is worth focusing on.
Acting as an immune regulatory ligand for TLR4, beta-glucan induced Dectin-1-TLR4 in human dendritic cells10 and THP-1 macrophages.17 In this study, beta-glucans exerted stronger immune responses by the clustering of Dectin-1 receptors and blocking of TLR4, resulting in the decreased production of pro-inflammatory cytokines such as IL-1β, IL-6 and TNF-αand increased production of anti-inflammatory cytokine IL-10. The data illustrate that TLR4 binding by beta-glucan leads to the manipulation of intracellular pathways and downstream cytokine production. Beta-glucans with high molecular weights are stronger Dectin-1 activators than the low molecular weight molecules. Moreover, Dectin-1 enhances an immune regulatory pathway depending on TLR4. However, other TLRs such as TLR2, 5 and 6 cannot, therefore, be excluded in response to the regulation of cytokine production. Thus, we conclude that the existence of an anti-inflammatory role of TLR4 after beta-glucan stimulation and the activation of Dectin-1 can be synergistically modulated by the high molecular weight of beta-glucans. Our results indicate that beta-glucans can down-regulate the TLR4/NF-κB signal-related factors in LPS-induced inflammation, thereby alleviating the LPS-mediated activation of innate host immune responses in jejunal tissue. These results concur with the previous finding that beta-glucan attenuated the LPS induction of TLR4/NF-κB and inhibited the LPS-induced inflammation factor in mammary epithelial cells and THP cells, respectively.44,45 The mechanisms may be associated with the SYK kinase or MyD88 signaling cascade during beta-glucan-mediated Dectin-1 activation.46 Collectively, our study demonstrates structure-effector relationships between beta-glucans and PRRs, which may have important implications in the design of more effective food products containing high molecular weight beta-glucans.
Conclusions
Both investigated Agrobacterium sp. ZX09 beta-glucan fractions, with low and high molecular weights, could prevent the loss in body weight gain of weaned piglets caused by LPS. Beta-glucans have strong immune-modulatory properties via reducing the pro-inflammatory cytokines and inducing the anti-inflammatory cytokines caused by LPS-induced inflammation. The underlying mechanisms may be related to key factors such as TLR4 and NF-κB, which are involved in the manipulation of the immune response of beta-glucans. The difference in efficacy between the two molecules is attributed to the clustering of Dectin-1. With a high affinity for Dectin-1, high molecular weight beta-glucans seem to show stronger anti-inflammatory activity in comparison to low molecular weight beta-glucans. This insight might assist and facilitate the development of effective beta-glucan preparations to guide immune responses and create opportunities for therapeutic or protective usage in functional food formulations.
Conflicts of interest
There are no conflicts to declare.
Acknowledgements
This research was supported by a grant from the International Science & Technology Cooperation Foundation, Sichuan, China (2016HH0004) and 111 project (D17015). All authors read and approved the final manuscript.
References
- H. Li, J. Yan, S. Qin, Y. Chen and H. La, Extraction and antioxidant function of polysaccharides from seaweed, Agric. Biotechnol., 2015, 4, 73–77 CAS.
- V. Vetvicka, L. Vannucci and P. Sima, The effects of β-glucan on pig growth and immunity, Open Biochem. J., 2014, 8, 89–93 CrossRef PubMed.
- V. Vetvicka and C. Oliveira, β(1-3)(1-6)-D-glucans modulate immune status in pigs: potential importance for efficiency of commercial farming, Ann. Transl. Med., 2014, 2, 16 Search PubMed.
- S. Hiss and H. Sauerwein, Influence of dietary ss-glucan on growth performance, lymphocyte proliferation, specific immune response and haptoglobin plasma concentrations in pigs, J. Anim. Physiol. Anim. Nutr., 2003, 87, 2–11 CrossRef CAS PubMed.
- F. Molist, E. Eerden, H. K. Parmentier and J. Vuorenmaa, Effects of inclusion of hydrolyzed yeast on the immune response and performance of piglets after weaning, Anim. Feed Sci. Technol., 2014, 195, 136–141 CrossRef CAS.
- M. A. D. Saleh, A. B. Amorim, H. A. T. Grecco, D. A. Berto, C. R. Padovani, R. O. Orsi and M. L. P. Tse, Effects of β-(1→3,1→6)-D-glucan and density of diets on the blood profiles of immunologically challenged weaned piglets, Int. J. Biol. Macromol., 2015, 80, 659–667 CrossRef CAS PubMed.
- S. H. Moon, I. Lee, X. Feng, H. Y. Lee, J. Kim and D. U. Ahn, Effect of dietary beta-glucan on the performance of broilers and the quality of broiler breast meat, Asian-Australas. J. Anim. Sci., 2016, 29, 384–389 CrossRef CAS PubMed.
- X. Zheng, S. Zou, H. Xu, Q. Liu, J. Song, M. Xu, X. Xu and L. Zhang, The linear structure of β-glucan from baker's yeasr and its activation of macrophage-like RAW264.7 cells, Carbohydr. Polym., 2016, 148, 61–68 CrossRef CAS PubMed.
- Y. Ishimoto, K. Ishibashi, D. Yamanaka, Y. Adachi, K. Kanzaki, K. Okita, Y. Iwakura and N. Ohno, Modulation of an innate immune response by soluble yeast β-glucan prepared by a heat degradation method, Int. J. Biol. Macromol., 2017, 104, 367–376 CrossRef CAS PubMed.
- N. M. Sahasrabudhe, J. Dokter-Fokkens and P. de Vos, Particulate β-glucans synergistically activate TLR4 and Dectin-1 in human dendritic cells, Mol. Nutr. Food Res., 2016, 60(11), 2514–2522 CrossRef CAS PubMed.
- K. Błaszczyk, J. Wilczak, J. Harasym, S. Gudej, D. Suchecka, T. Krolikowski, E. Lange and J. Gromadzka-Ostrowska, Impact of low and high molecular weight oat beta-glucan on oxidative stress andantioxidant defense in spleen of rats with LPS induced enteritis, Food Hydrocolloids, 2015, 51, 272–280 CrossRef.
- A. A. Khan, A. Gani, F. A. Masoodi, F. Amin, I. A. Wani, F. A. Khanday and A. Gani, Structural thermal, functional, antioxidant & antimicrobial properties of β-d-glucan extracted from baker's yeast (Saccharomyces cereviseae)—Effect of γ-irradiation, Carbohydr. Polym., 2016, 140, 442–450 CrossRef CAS PubMed.
- J. Fang, Y. Wang, X. Lv, X. Shen, X. Ni and K. Ding, Structure of a beta-glucan from Grifola frondosa and its antitumor effect by activating Dectin-1/Syk/NF-kappaB signaling, Glycoconjugate J., 2012, 29, 365–377 CrossRef CAS PubMed.
- N. Dalonso, G. H. Goldman and R. M. Gern, Beta-(1−>3),(1−>6)-Glucans: medicinalactivities, characterization, biosynthesis and new horizons, Appl. Microbiol. Biotechnol., 2015, 99, 7893–7906 CrossRef CAS PubMed.
- K. Baert, E. Sonck, B. M. Goddeeris, B. Devriendt and E. Cox, Cell type-specific differences in β-glucan recognition and signaling in porcine innate immune cells, Dev. Comp. Immunol., 2015, 48, 192–203 CrossRef CAS PubMed.
- Y. Guo, T. Fukuda, K. Donai, K. Kuroda, M. Masuda, S. Nakamura, H. Yoneyama and E. Isogai, Leptospiral lipopolysaccharide stimulates the expression of toll-like receptor 2 and cytokines in pig fibroblasts, Anim. Sci. J., 2015, 86, 238–244 CrossRef CAS PubMed.
- P. Kanjan, N. M. Sahasrabudhe, B. J. de Haan and P. de Vos, Immune effects of beta-glucan are determined by combined effects on Dectin-1, TLR2, 4 and 5, J. Funct. Foods, 2017, 37, 433–440 CrossRef CAS.
- A. Rieder, S. Grimmer, S. O. Kolset, T. E. Michaelsen and S. H. Knutsen, Cereal beta-glucan preparations of different weight average molecular weights induce variable cytokine secretion in human intestinal epithelial cell lines, Food Chem., 2011, 128, 1037–1043 CrossRef CAS.
- A. Rieder, S. Grimmer, F. L. Aachmann, B. Westereng, S. O. Kolset and S. H. Knutsen, Generic tools to assess genuine carbohydrate specific effects on in vitro immune modulation exemplified by β-glucans, Carbohydr. Polym., 2013, 92, 2075–2083 CrossRef CAS PubMed.
- S. Danicke, H. Valenta, M. Ganter, B. Brosig, S. Kersten, A. Diesing, S. Kahlert, P. Panther, J. Kluess and H. Rothkotter, Lipopolysaccharides (LPS) modulate the metabolism of deoxynivalenol (DON) in the pig, Mycotoxin Res., 2014, 30, 161–170 CrossRef PubMed.
- S. Gessi, P. A. Borea, S. Bencivenni, D. Fazzi, K. Varani and S. Merighi, The activation of u-opioid receptor potentiates LPS-induced NF-kB promoting an inflammatory phenotype in microglia, FEBS Lett., 2016, 590, 2813–2816 CrossRef CAS PubMed.
- B. Abad, J. E. Mesonero, M. T. Salvador, J. Garcia-Herrera and M. J. Rodriquez-Yoldi, Cellular mechanism underlying LPS-induced inhibition of invitro L-leucine transport across rabbit jejunum, Innate Immun., 2002, 8, 127–133 CAS.
- Y. Zhang, L. Xia, W. Pang, T. Wang, P. Chen, B. Zhu and J. Zhang, A novel soluble β-1,3-D-glucan salecan reduces adiposity and improves glucose tolerance in high-fat diet-fed mice, Br. J. Nutr., 2013, 109, 254–262 CrossRef CAS PubMed.
- M. DuBios, K. A. Gilles, J. K. Hamilton, P. A. Rebers and F. Smith, Colorimetric Method for Determination of Sugars and Related Substances, Anal. Chem., 1956, 28, 350–356 CrossRef.
- B. U. Metzler-Zebeli, M. G. Ganzle, R. Mosenthin and R. T. Zijlstra, Oat β-glucan and dietary Calcium and phosphorus differentially modify intestinal expression of proinflammatory cytokines and monocarboxylate transporter 1 and cecal morphology in weaned pigs, J. Nutr., 2012, 142, 668–674 CrossRef CAS PubMed.
- E. B. Byun, S. H. Park, B. S. Jang, N. Y. Sung and E. H. Byun, Gamma-irradiated β-glucan induces immunomodulation and anticancer activity through MAPK and NF-kB pathways, J. Sci. Food Agric., 2016, 96, 695–702 CrossRef PubMed.
- G. Q. Han, Z. T. Xiang, B. Yu, D. W. Chen, H. W. Qi, X. B. Mao, H. Chen, Q. Mao and Z. Q. Huang, Effects of different starch sources on Bacillus spp. in intestinal tract and expression of intestinal development related genes of weanling piglets, Mol. Biol. Rep., 2012, 39, 1869–1876 CrossRef CAS PubMed.
- D. Suchecka, J. P. Harasym, J. Wilczak, M. Gajewska, M. Oczkowski, S. Gudej, K. Blaszczk, D. Kamola, R. Filip and J. Gromadzka-Ostrowska, Antioxidative and anti-inflammatory effects of high beta-glucan concentration purified aqueous extract from oat in experimental model of LPS-induced chronic enteritis, J. Funct. Foods, 2015, 14, 244–254 CrossRef CAS.
- D. Suchecka, K. Blaszczyk, J. Harasym, S. Gudej, J. Wilczak and J. Gromadzka-Ostrowska, Impact of purified oat 1-3,1-4-β-d-glucan of different molecular weight on alleviation of inflammation parameters during gastritis, J. Funct. Foods, 2017, 28, 11–18 CrossRef CAS.
- T. X. Zhou, J. H. Jung, Z. F. Zhang and I. H. Kim, Effect of dietary
β-glucan on growth performance, fecal microbial shedding and immunological responses after lipopolysaccharide challenge in weaned pigs, Anim. Feed Sci. Technol., 2013, 179, 85–92 CrossRef CAS.
- S. N. Hester, S. S. Comstock, S. C. Thorum, M. H. Monaco, B. D. Pence, J. A. Woods and S. M. Donovan, Intestinal and systemic immune development and response to vaccination are unaffected by dietary (1,3/1,6)-β-D-glucan supplementation in neonatal piglets, Clin. Vaccine Immunol., 2012, 19, 1499–1508 CrossRef CAS PubMed.
- Y. Shao, Z. Wang, X. Tian, Y. Guo and H. Zhang, Yeast β-D-glucans induced antimicrobial peptide expressions against Salmonella infection in broiler chickens, Int. J. Biol. Macromol., 2016, 85, 573–584 CrossRef CAS PubMed.
- D. Suchecka, J. Harasym, J. Wilczak and J. Gromadzka-Ostrowska, Hepato- and gastro- protective activity of purified oat 1-3,1-4-β-D-glucans of different molecular weight, Int. J. Biol. Macromol., 2016, 91, 1177–1185 CrossRef CAS PubMed.
- A. S. Rathore, S. Kumar, R. Konwar, A. Makker, M. P. Negi and M. M. Goel, CD3+, CD4+& CD8+ tumour infiltrating lymphocytes (TILs) are predictors of favourable survival outcome in infiltrating ductal carcinoma of breast, Indian J. Med. Res., 2014, 140, 361–269 Search PubMed.
- H. Yang, F. Wu, L. Long, T. Li and X. Xiong, Effects of yeast products on the intestinal morphology, barrier function, cytokine expression, and antioxidant system of weaned piglets, J. Zhejiang Univ., Sci., B, 2016, 17, 752–762 CrossRef CAS PubMed.
- Y. Yao, J. Vent-Schmidt, M. D. McGeough, M. Wong, H. M. Hoffman, T. S. Steiner and M. K. Levings, Tr1 cells, but not Foxp3+ regulatory T cells, suppress NLRP3 inflammasome activation via an IL-10-dependent mechanism, J. Immunol., 2015, 195, 488–497 CrossRef CAS PubMed.
- U. Lodemann, S. Amasheh, J. Radloff, M. Kern, A. Bethe, L. H. Wieler, R. Pieper, J. Zentek and J. R. Aschenbach, Effects of ex vivo infection with ETEC on jejunal barrier properties and cytokine expression in probiotic-supplemented pigs, Dig. Dis Sci., 2017, 62, 922–933 CrossRef CAS PubMed.
- W. Ouyang, S. Rutz, N. K. Crellin, P. A. Valdez and S. G. Hymowitz, Regulation and functions of the IL-10 family of cytokines in inflammation and disease, Annu. Rev. Immunol., 2011, 29, 71–109 CrossRef CAS PubMed.
- H. Toker, E. P. Gorgun and E. M. Korkmaz, Analysis of IL-6, IL-10 and NF-kB gene polymorphisms in aggressive and chronic periodontitis, Cent. Eur. J. Public Health, 2017, 25, 157–162 CrossRef CAS PubMed.
- Z. Wang, Y. Guo, J. Yuan and B. Zhang, Effect of β-1,3/1,6-glucan supplementation on growth performance, immune response and plasma prostaglandin E2, growth hormone and ghrelin in weanling piglets, Asian-Australas. J. Anim. Sci., 2008, 21, 707–714 CrossRef CAS.
- J. Li, D. F. Li, J. J. Xing, Z. B. Cheng and C. H. Lai, Effects of β-glucan extracted from Saccharomyces cerevisiae on growth performance, and immunological and somatotropic responses of pigs challenged with Escherichia coli lippolysaccharide, J. Anim. Sci., 2006, 84, 2374–2381 CrossRef CAS PubMed.
- S. C. Thorum, S. N. Hester, S. S. Comstock, M. H. Monaco, B. D. Pence, J. A. Woods and S. M. Donovan, Dietary (1,3/1,6)-β-glucan decreases transforming growth factor β expression in the lung of the neonatal piglet, Nutr. Res., 2013, 33, 322–331 CrossRef CAS PubMed.
- Y. Luo, H. Chen, B. Yu, J. He, P. Zheng, X. Mao, J. Yu, J. Luo, Z. Huang and D. Chen, Dietary pea fibre alters the microbial community and fermentation with increase in fibre degradation-associated bacterial groups in the colon of pigs, J. Anim. Physiol. Anim. Nutr., 2018, 102, e254–e261 CrossRef CAS PubMed.
- W. Zhu, H. Ma, J. Miao, G. Huang, M. Tong and S. Zou, β-Glucan modulates the lipopolysaccharide-induced innate immune response in rat mammary epithelial cells, Int. Immunopharmacol., 2013, 15, 457–465 CrossRef CAS PubMed.
- F. Liu, X. Zhang, Y. Li, Q. Chen, F. Liu, X. Zhu, L. Mei, X. Song, X. Liu, Z. Song, J. Zhang, W. Zhang, P. Ling and F. Wang, Anti-inflammatory effects of a mytilus coruscus α-D-glucan (MP-A) in activated macrophage cells via TLR4/NF-κB/MAPK pathway inhibition, Mar. Drugs, 2017, 15, 294 CrossRef PubMed.
- B. Li, D. Cramer, S. Wagner, R. Hansen, C. King, S. Kakar, C. Ding and J. Yan, Yeast glucan particles activate murine resident macrophages to secrete proinflammatory cytokines via MyD-88- and Syk kinase-dependent pathways, Clin. Immunol., 2007, 124, 170–181 CrossRef CAS PubMed.
|
This journal is © The Royal Society of Chemistry 2020 |
Click here to see how this site uses Cookies. View our privacy policy here.