Effects of indoor coal fine particulate matter on the expression levels of inflammatory factors in ovalbumin-induced mice†
Received
17th August 2018
, Accepted 18th October 2018
First published on 1st November 2018
Abstract
Objective: Cooking and heating with coal is the main source of household air pollution in acid rain-plagued areas of China and is a leading contributor to disease burden. In this study, we investigated the adverse effects of exposure to indoor fine particulate matter emission from coal combustion on the expression levels of inflammatory factors in ovalbumin (OVA)-induced mice. Methods: Forty BALB/c male mice were randomly divided into four groups (control group, PM2.5 group, OVA group, and OVA + PM2.5 group; n = 10) and treated with ovalbumin (OVA) or PM2.5, alone or together. Interleukin-4 (IL-4), interleukin-7 (IL-7), interleukin-8 (IL-8), interleukin-17 (IL-17), transforming growth factor β1 (TGF-β1), and interferon γ (IFN-γ) protein expression levels were measured in bronchoalveolar lavage fluid (BALF). Additionally, serum immunoglobulin (Ig) IgE and IgG1 levels were measured. The mRNA expression levels of IL-7 and IFN-γ in pulmonary tissue were also analyzed. Bronchoalveolar lavage (BAL), inflammatory cell counts, and histopathological examinations were also performed. Results: Exposure to PM2.5 + OVA induced abnormal pathological changes and inflammatory responses in lungs compared to the control. The levels of IL-4, IL-7, IL-8 and IL-17 in BALF from the OVA + PM2.5 group were higher than those in BALF from the control group, OVA group, and PM2.5 group (P < 0.05). PM2.5 plus OVA significantly raised the serum IgE and IgG1 levels compared with the control group. An increasing IL-7 mRNA trend was found among the treatment groups (P < 0.05). The expression level of IFN-γ mRNA was significantly higher in the control group than in the other 3 groups (P < 0.05). Conclusion: Indoor coal PM2.5 was sufficient by itself to cause inflammatory cellular infiltration of pulmonary tissue, leading to organelle injury and physiological structure change. Additionally, it promoted the occurrence and development of asthma by influencing the expression levels of IL-7 and various relevant inflammatory factors (such as IL-4 and IL-8) and changing the equilibrium between Treg and Th17 cells.
1. Introduction
The incidence and mortality rate of bronchial asthma are increasing continuously worldwide. The incidence of bronchial asthma has doubled during the past decade in Europe, and in developed countries the increase has been over 60% since the 1980s.1 There are about 30
000
000 asthma patients in China every year, and the clinical incidence is up to 2.1%.2 For about 70% of people with asthma, symptoms are triggered (at least partly) by allergens, such as pollen, house dust mites, or pet dander.3 In a previous study, we found that the incidence of asthma among 1207 adult residents in winter (from November to early February the next year) in urban Zunyi was 1.8%, and the total incidence of adult asthma and asthma-related symptoms was up to 13.1%.4 Zunyi is an old industrial base in Western China and its atmospheric pollution is a typical pollution mixture containing coal smoke and motor vehicle exhaust. Coal resources in Zunyi are abundant. Zunyi is a city seriously polluted by acid rain, and it has recorded the lowest pH value of acid precipitation ever reached: 3.2.4 Due to the wet and cold environment in winter and the low cost of coal, some families use coal as their main energy source in daily life.5 Thus, the health hazard posed by indoor coal pollution cannot be ignored. Currently, there are very few studies on the toxicological effects associated with indoor coal PM2.5 pollution. The available pertinent investigations mainly focus on the cytotoxicity or genetic toxicity caused by PM2.5 present in indoor cooking oil fumes, as well as the correlation of pollution caused by biofuel (such as wood) with resultant pulmonary function change.6 In the coal-mining regions of China, studies evaluating exposure to indoor PM2.5 emission from coal combustion and its impact on bronchial function are scarce. Therefore, it is necessary to explore the correlation between levels of exposure to PM2.5 pollution and bronchial asthma onset in coal-mining regions.
Hizume et al. reported that the indoor smoke from cigarettes aggravated the inflammatory anaphylactic reaction in OVA-sensitized and challenged mice and increased the expression levels of inflammatory response-related cytokines (such as IL-4, Il5, and chemokine (C–C motif) ligand 11).7 After human bronchial epithelial cells were exposed to particles collected from ambient air in Beijing, the expression of many immune factors (such as TNF, IL1A, IL1B, IL6, IL8, and IL24) was increased. Due to the accuracy of the high expression of IL6 in predicting respiratory diseases in children, IL-6 has been suggested for use as a biomarker for childhood respiratory diseases.8 Various inflammatory factors have been proven to participate in the etiological process of asthma induced by pollution. However, there has been no report on the effects of indoor PM2.5 emission from coal combustion on the levels of IL-17 in bronchial airway inflammation in OVA-induced mice, or on the relevant mechanism.
In this study, using an asthma mouse model, pathological changes in the respiratory tract were examined, the expression levels of various cytokines closely related with innate immunity were measured, and the effects and mechanism of coal-derived PM2.5-induced airway inflammation in OVA-induced mice were investigated.
2. Materials and methods
2.1 Methods
2.1.1 Sampling and collection of PM2.5 from coal combustion.
In a lab with an area of 60 m3, an air sampler (TH150-A, Wuhan Tianhong INST Group) equipped with a PM2.5 filter membrane was used for sampling. Zunyi coal was processed into lumps with sizes of 3–5 cm. When a stable coal fire had been generated, coal briquettes were weighed and placed into the stove. The air sampler flow rate was set at 100 L min−1, and the height was 1.5 m. A PM2.5 quartz fiber filter membrane was used for sampling 3 times daily, for 4 h each time, for 7 consecutive days. After collection, samples were covered with aluminum-foil paper, placed in a drying vessel, and stored at −20 °C, avoiding light.
2.1.2 Coal PM2.5 extraction and pollution solution preparation.
The sampling surface of the filter membrane was placed in a 250 mL conical flask with 100 mL of distilled water, and sonicated 3 times, for 20 min each time, at 500 W. The extraction solution was filtered with 12-layer sterile gauze. These extraction steps were repeated three times. The filter membrane fragments were removed, and the filtration solution was transferred into sterilized glass bottles, with 5 mL in each bottle, lyophilized overnight at −80 °C under vacuum into powder, and stored in a refrigerator at −80 °C. Powder samples were prepared in solution at a concentration of 5 mg mL−1 with saline using ultrasonication; then they were sterilized and stored at 4 °C. Before use, the solution was shaken to resuspend the particles. Detailed extraction techniques can be found in Bein and Wexler.9
2.1.3 Animal model.
Forty 6–8 weeks old BALB/c male mice with body weights of 30–40 g were used in experiments. The mice were randomly divided into 4 groups (control group, PM2.5 group, OVA group and OVA + PM2.5 group; n = 10). The PM2.5 samples were suspended in phosphate-buffered saline (PBS) at a concentration of 4 mg mL−1. OVA (Sigma Chemicals Co., St Louis, MO, USA) was dissolved in PBS at a concentration of 10 μg mL−1. The aerosolized OVA concentration was 10 mg mL−1.10 The mice were acclimated for 1 week, and then were sensitized. The mice in the OVA group and PM2.5 + OVA group were intraperitoneally injected with 10 μg of OVA and 1 mg of Al(OH)3 suspension11 on days 0, 7 and 14 using a modified protocol previously described in ref. 12. Those in the control group and PM2.5 group were intraperitoneally injected with an equal volume of PBS (once weekly for a total of 3 times). Seven days after the final i.p. injection, during the trigger (challenge) phase, the mice in the OVA group and PM2.5 + OVA group were given 10 mg mL−1 OVA through aerosol inhalation13 for 30 min daily from day 21 to day 51. Those in the control group and PM2.5 group were given PBS (30 min each time, once every 3 days for a total of 10 times). Mice were placed in a plexiglas chamber (35 × 25 × 25 cm; patent no. ZL2016211116330, China) and exposed to an aerosol generated using an ultrasonic nebulizer (402B, Yuwell, Jiangsu, China) with an airflow of ≥3 ml min−1. The mice in the PM2.5 group and PM2.5 + OVA group were given 5 μL of PM2.5 suspension at a dose of 4 μg μL−1via an anterior nasal cavity drop (in both the left and right cavity each time).14 Within 24 h of the last aerosolized exposure, the mice were sacrificed using intraperitoneal chloral hydrate (100 g L−1).
2.1.4 Blood and bronchoalveolar lavage fluid (BALF) collection.
All mice fasted overnight and were anesthetized through administering 10% chloral hydrate via intraperitoneal injection (3.5 mL kg−1). Blood was collected from the abdominal aorta, allowed to coagulate, and centrifuged at 3000 rpm for 10 min. Serum was collected and stored at −80 °C. The right lung was ligated, and a T incision was made in the neck. The trachea was separated to perform tracheal intubation. The left lung was lavaged 3 times with 1 ml of PBS. The recovery rate of lavage fluid was higher than 80%. The cells in the collected BALF were counted using a Neubauer hemocytometer. Subsequently, the BALF was centrifuged at 1500 rpm for 10 min at low temperature to separate the supernatant and precipitate. The precipitate was collected and used to perform smears and cell counting after staining. The supernatant was stored at −80 °C for subsequent analysis.
2.1.5 Sample detection.
(1) Cell detection in BALF
a. Cell counting in BALF: after sacrificing the mouse, BALF was collected and centrifuged, and the cells in the supernatant were counted using a Neubauer hemocytometer.
b. Liu's staining: the BALF precipitate was used to perform cell smears. After the smear was dried and fixed, 1 ml of LiuA reagent containing Eosin Y (for cytoplasm staining) was added to the slides at room temperature. After standing for 30 s, 1 ml of LiuB reagent containing Azur I and methylene azure (for nuclei staining) was added for 60 s at room temperature, which was followed by the gentle mixing of the reagents by blowing on the slides. The slides were washed with running water for 2 min and then air-dried (Liu's stain solution, Zhuhai Baso Biological Technology Co., Ltd, Zhuhai, China). The monocytes (Mon), neutrophil granulocytes (Neu), lymphocytes (Lym) and eosinophils (Eos) were counted.
(2) Pathological hematoxylin and eosin (HE) staining
Tissue slices with a size of 1 × 1 cm were collected from the upper right lung, washed with physiological saline, and fixed in 10% paraformaldehyde for 24 h. The fixed samples were washed in running tap water for 24 h and placed in 75% ethanol for long storage.
(3) Sampling for scanning electron microscopy (SEM)
All tools were pre-cooled at 4 °C. Samples from the upper right lung with a size of 1 mm3 were cut out and fixed in 2.5% glutaraldehyde. The tissue samples were completely immersed after vibration and fixed for 2 h at 4 °C. Subsequently, samples were examined with a scanning electron microscope.
(4) BALF cytokine analysis
A sensitive indirect enzyme linked immunosorbent assay (ELISA) was established to measure cytokine protein levels, as previously described.15 Assays for IL-4, IL-7, IL-8, IL-17, TGF-β1 and IFN-γ were conducted using matched antibody pairs obtained from Solarbio (Beijing, China) according to the manufacturer's instructions. Optical density readings of samples were converted to picograms per milliliter using values obtained from standard curves generated with varying concentrations of mouse recombinant IL-4, IL-7, IL-8, IL-17, TGF-β1 and IFN-γ. The limit of detection of each assay was 2 pg ml−1. Absorbance was read at 450 nm.
(5) OVA-specific serum IgE and IgG1 analysis
OVA-specific IgE and IgG1 antibodies were measured with a mouse OVA-IgE ELISA kit and a mouse OVA-IgG1 ELISA kit (Beijing Suolaibao Technology, Beijing, China). According to the manufacturer's protocol, 1 U of anti-OVA IgE was defined as 1.3 ng of antibody, and 1 U of anti-OVA IgG1 as 160 ng of antibody. The absorption at 450 nm (sub-wave length, 620 nm) for OVA-specific IgE and IgG1 antibodies was measured using an iMark microplate reader (BioRad, Hercules, California, USA).
(6) Detection of IL-7 and IFN-γ mRNA in lung tissue
A real-time reverse transcriptase–polymerase chain reaction (RT-PCR) was performed with a c1000 thermal cycler (BioRad, Hercules, California, USA) to detect the IL-7 and IFN-γ mRNA levels in pulmonary tissue samples. In brief, lung tissue was dissected on ice, weighed and homogenized in 1 mL of TRIzol using a homogenizer (FlukoÒ Equipment Shanghai Co., Ltd, China). The homogenate was mixed vigorously with 0.2 mL of chloroform and centrifuged at 12
000g for 15 min at 4 °C. The supernatant was collected and 0.5 mL of isopropanol was added. The mixture was centrifuged at 12
000g for 10 min at 4 °C, and then the RNA precipitate was washed once with 1 mL of 75% ethanol and dissolved in 0.1 mL of DEPC water. The concentration and quality of total RNA were assessed via a spectrometric method with NanoPhotometer apparatus (IMPLEN, München, Germany). The sequences of the primers used for this analysis are listed in Table 1.
Table 1 Primer sequences of ACTB, IL-7 and IFN-γ
Gene |
Primer sequence |
ACTB |
Forward primer – 5′-CACCCGCGAGTACAACCTTC-3′ |
Reverse primer – 5′-CCCATACCCACCATCACACC-3′ |
IL-7 |
Forward primer – 5′-TTCCTCCACTGATCCTTGTTCT-3′ |
Reverse primer – 5′-AGCAGCTTCCTTTGTATCATCAC-3′ |
IFN-γ |
Forward primer – 5′-GCCACGGCACAGTCATTGA-3′ |
Reverse primer – 5′-TGCTGATGGCCTGATTGTCTT-3′ |
2.2 Statistical analysis
Statistical analyses were performed with SPSS software, version 20.0 for Windows (SPSS Inc., Chicago, IL, USA). Values for all variables are presented as means with standard deviations. One-way analysis of variance (ANOVA) was used to determine the effects of different treatments, and the least-significant difference (LSD) test was used for paired comparisons among multiple groups. A level of p < 0.05 is considered statistically significant.
2.3 Ethics statement
All experimental procedures were conducted at Zunyi Medical University and adhered to the guidelines and regulations set out by the Zunyi Medical University Ethics Committee with full approval from its Animal Care and Use Committee.
3. Results
3.1 Behavioral observations
The mice in the control group showed normal behavior without any significant behavioral changes. Those in the OVA group caught their ears and washed their faces, and their breath showed dramatic fluctuations. In the PM2.5 group, the mice occasionally caught their ears, showing dysphoria. In the OVA + PM2.5 group, the mice breath deeply and quickly, and the frequency of ear catching significantly increased, with dysphoria and irritability shown.
3.2 Naked-eye observation of lung tissue
There were no abnormal findings for the majority of mice in the control group, whose lung profile was clear, smooth and pink. The occasional occurrence of local edema and obtuse edges could be seen on the rough surfaces of lungs in the OVA group. Other than a dark color, the findings relating to lung changes in the PM2.5 group were similar to the OVA group. Dark color, obtuse edges, obvious edema and partly destructed lung tissue structures were observed in the OVA + PM2.5 group (Fig. 1).
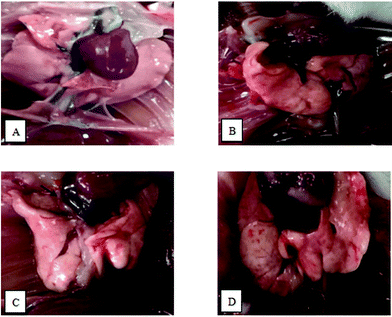 |
| Fig. 1 Images showing pathological changes in mouse pulmonary tissue from each group: (A) control group; (B) OVA group; (C) PM2.5 group; and (D) OVA + PM2.5 group. | |
3.3 Cell distribution in BALF
The inflammatory cell distributions in BALF from each mouse group are shown in Fig. 2. In the OVA group mice, the numbers of Mon, Lym, Neu and Eos and the total number of cells were significantly higher than in the control group (p < 0.05). Additionally, their numbers in the PM2.5 group were also significantly higher than in the control group (p < 0.05), but they were not significantly different compared with the OVA group (p > 0.05). In addition, the numbers of these various cell types were significantly higher in the OVA + PM2.5 group than in the other three groups (p < 0.05).
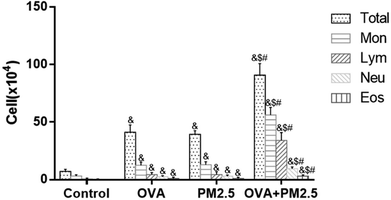 |
| Fig. 2 The distribution of inflammatory cells in BALF of mice from the different groups. Statistical significances are indicated as follows: & vs. control: p < 0.05; $ vs. OVA: p < 0.05; # vs. PM2.5: p < 0.05. | |
3.4 Pathological sections of pulmonary tissue
As shown in Fig. 3, in lung tissue from the control group, the cells did not slough off, and exudation and aggregation of inflammatory cells were not observed. The morphologies of the alveolar and bronchial mucosal epithelia were normal. In lung tissue from the OVA group, massive Eos aggregation occurred, as indicated by the upward arrow symbol (↑), and Mon and Lym can be observed in the bronchial walls and surroundings. Mucosal epithelial cells were located as indicated by the asterisk symbol (*). In lung tissue from the PM2.5 group, Eos aggregated in the alveoli, and congested and inflamed alveoli were observed. In lung tissue from the OVA + PM2.5 group, there were massive inflammatory cell aggregates in pulmonary and bronchial tissues. Additionally, interstitial infiltrates were evident, and bronchial epithelial cells fell off and were exuded in bronchi, as indicated by the cross symbol (×) in Fig. 3.
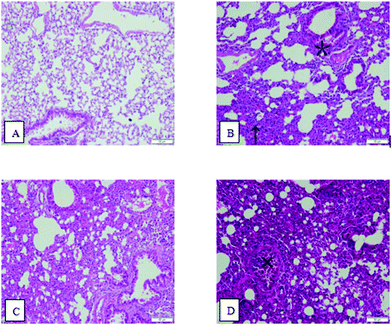 |
| Fig. 3 Images of pulmonary hematoxylin and eosin (HE) stained sections: (A) control group; (B) OVA group; (C) PM2.5 group; and (D) OVA + PM2.5 group. | |
3.5 Scanning electron microscopy (SEM) analysis
As shown in Fig. 4, normal architecture was observed in the lungs of the control group. Osmiophilic lamellar bodies are rich in the cytoplasm of type II epithelial plasma cells. In lung tissue from OVA group mice, Neu can be observed in some capillary lumina. Additionally, alveolar type I epithelial cells did not show any abnormalities, and a few osmiophilic lamellar bodies and the evacuation of lamellar bodies can be observed in the Type II epithelial plasma cells. Moreover, no significant abnormalities were observed in mitochondria. Also, microvilli-like protrusions on the cell surface decreased and disappeared. In addition, macrophage infiltration was observed. In lung tissue from the PM2.5 group mice, some alveolar Type I epithelial cells showed significant swelling. Meanwhile, the conditions in the Type II epithelial plasma cells were the same as in the OVA group, but swelling was observed in the mitochondria. Moreover, the microvilli-like protrusions on the cell surface disappeared without hyperplasia. In lung tissue from the OVA + PM2.5 group mice, some alveolar type I epithelial cells showed significant swelling. Also, a few osmiophilic lamellar bodies and the evacuation of lamellar bodies could be observed in the type II epithelial plasma cells. In addition, the mitochondria showed swelling, and macrophages were occasionally observed. Additionally, there was increased collagen deposition in the alveolar septa.
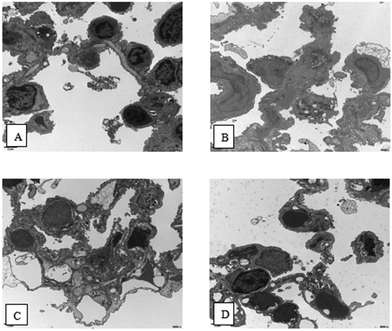 |
| Fig. 4 Scanning electron microscopy (SEM) images of lung tissue from different mouse groups. (A) control group; (B) OVA group; (C) PM2.5 group; and (D) OVA + PM2.5 group. | |
3.6 Measurement of IL-4 and IL-7 levels in BALF
The results shown in Fig. 5 reveal that the expression level of IL-4 in the control group was significantly lower than in the other three groups (p < 0.05). However, there was no statistically significant difference between the OVA group and PM2.5 group (p > 0.05). Also, the IL-4 expression level in the OVA + PM2.5 group was significantly higher than in the other three groups (p < 0.05; F = 26.884). For the IL-7 expression level, there was no significant difference between the control group and the PM2.5 group (p = 0.776). Also, the expression level of IL-7 in the OVA + PM2.5 group was the highest, followed by the OVA group and then the PM2.5 group (p < 0.05; F = 15.327) (Fig. 5).
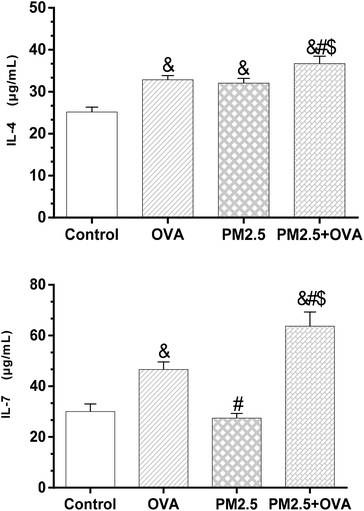 |
| Fig. 5 IL-4 and IL-7 expression levels in BALF. Statistical significances are indicated as follows: & vs. control: p < 0.05; # vs. OVA: p < 0.05; $ vs. PM2.5: p < 0.05. | |
3.7 Analysis of IL-8 and IL-17 expression levels in BALF
The data presented in Fig. 6 reveals that the expression level of IL-8 in the OVA + PM2.5 group was significantly higher than in the other three groups (p < 0.05), but there was no statistically significant difference between the OVA group and the PM2.5 group (p = 0.062). Also, the expression level of IL-8 in the control group was significantly lower than in the OVA group and the PM2.5 group (p < 0.05; F = 45.324). Regarding the expression of IL-17, the OVA + PM2.5 group had the highest expression level, followed by the OVA group, the PM2.5 group and the control group (p < 0.05; F = 26.744).
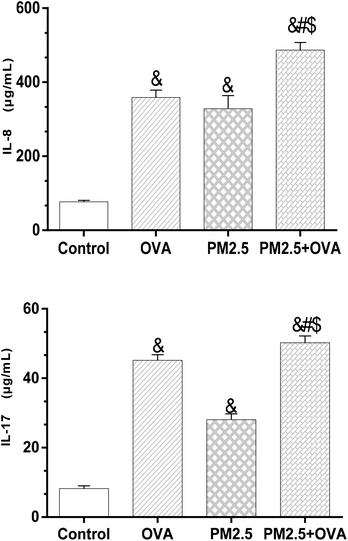 |
| Fig. 6 IL-8 and IL-17 levels in BALF. Statistical significances are indicated as follows: & vs. control: p < 0.05; # vs. OVA: p < 0.05; $ vs. PM2.5: p < 0.05. | |
3.8 Measurement of IFN-γ and TGF-β1 expression levels in BALF
The results presented in Fig. 7 reveal that there was no significant difference in IFN-γ expression levels between the OVA group and PM2.5 group (p = 0.071), but there was between the rest of the groups (p < 0.05; F = 17.526). Similarly, a comparison of the expression of TGF-β1 between these groups showed the same trends (Fig. 7).
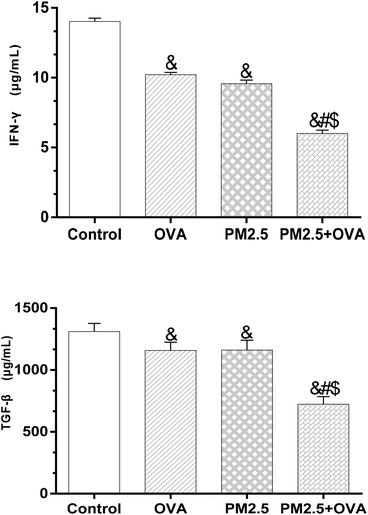 |
| Fig. 7 IFN-γ and TGF-β1 levels in BALF. Statistical significances are indicated as follows: & vs. control: p < 0.05; # vs. OVA: p < 0.05; $ vs. PM2.5: p < 0.05. | |
3.9 Measurement of serum IgE and IgG1 levels
As shown in Fig. 8, the expression levels of IgE in the different mouse groups were arranged as follows (from low to high): control < OVA < PM2.5 < OVA + PM2.5 (p < 0.05; F = 19.374). Regarding the IgG1 expression, the level in the control group was significantly lower than in the other groups (p < 0.05). Also, the expression of IgG1 in the OVA group was significantly higher than in the PM2.5 group (p < 0.05), but there was no statistical difference compared with the OVA + PM2.5 group (p = 0.088; F = 33.611).
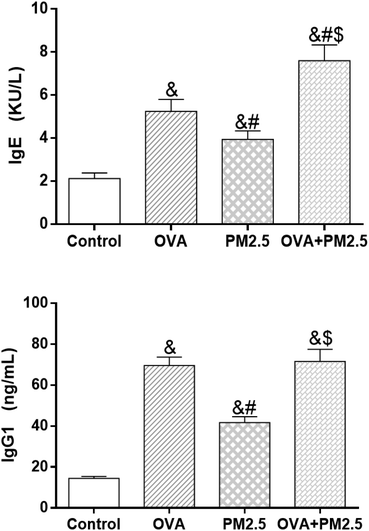 |
| Fig. 8 Expression levels of serum IgE and IgG1. Statistical significances are indicated as follows: & vs. control: p < 0.05; # vs. OVA: p < 0.05; $ vs. PM2.5: p < 0.05. | |
3.10 Analysis of IL-7 and IFN-γ mRNA expression levels in lung tissue
The data shown in Fig. 9 reveals that the IL-7 mRNA expression levels in the treatment groups were higher than in the control group; however, there was no statistical significance (p > 0.05; F = 0.028). In addition, the data also shows that the expression level of IFN-γ mRNA was significantly higher in the control group compared with the other three groups (p < 0.05), but there was no statistically significant difference between the other three groups (F = 63.651).
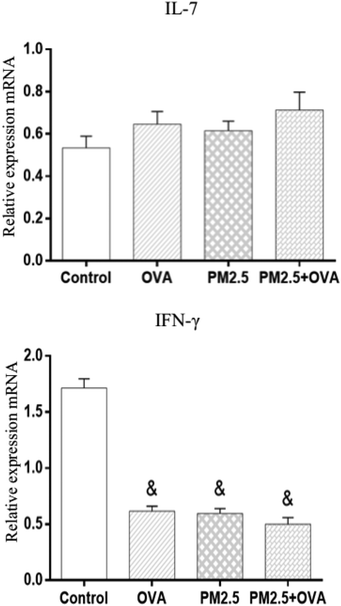 |
| Fig. 9 Relative expressions of IL-7 and IFN-γ mRNA in pulmonary tissue. Statistical significances are indicated as follows: & vs. control: p < 0.05. | |
4. Discussion
Indoor PM2.5 air pollution is one of the most highly concerning pollution issues worldwide.16 In poverty-stricken areas of China, most families use coal or other biofuels as energy sources for cooking or heating. However, since coal and other fuels cannot be completely combusted and their partial combustion results in the production of harmful gases (such as CO) and particle emission and aggregation, a lack of safety awareness leads families who live in such environments for a very long time to be exposed to such toxicants.17 It has been reported that the levels of indoor PM2.5 generated from coal combustion are higher than from biofuels, and much higher than from clean energy sources.18 PM2.5 enters into the human body more easily than inhalable particulate matter, and the carried toxic matter has higher toxicity. A higher PM2.5 concentration in the environment will cause more severe damage to human health.19
As asthma gradually aggravates, pulmonary tissue undergoes pathological changes. Makoto et al. (2013)20 provided a comprehensive perspective of the histopathological features of asthma. The lungs are usually hyperinflated as a consequence of extensive mucous plugging in segmental and subsegmental bronchi and peripheral airways.20 The common pathological changes include tissue looseness, reduced elasticity, and thickening bronchial walls, accompanied by mucosal swelling.21 Here, after exposure to indoor coal PM2.5, mice in the OVA + PM2.5 group showed obvious naked-eye observational changes compared with mice in the control group. The pulmonary tissue showed dark color, obtuse edges, obvious edema and partly destructed lung tissue structures. Additionally, the OVA + PM2.5 group also had the most severe inflammatory response. There were numerous inflammatory cell aggregates in the pulmonary and bronchial tissues. Interstitial infiltrates were evident, and bronchial epithelial cells fell and were exuded in bronchi. SEM analysis revealed some alveolar type I epithelial cells with significant swelling. A few osmiophilic lamellar bodies and the evacuation of lamellar bodies were observed in the type II epithelial plasma cells. Additionally, mitochondria showed swelling, and macrophages were occasionally observed. Also, some alveolar septa showed obvious bunchy collagen fiber. Overall, the comparison suggested that PM2.5 promoted and improved the inflammatory response and pathological tissue changes in OVA-induced mice.
Allergic asthma usually starts during childhood and is characterized by allergen-dependent, often seasonal, symptoms with positive skin tests to allergens and elevated total and allergen-specific serum. In contrast, non-allergic asthma usually begins in adulthood, is perennial and more severe, has no elevation in serum IgE, and is often associated with sinusitis and nasal polyposis.22 T lymphocytes play a vital role in the regulation and coordination of immune responses and the activation of T cells with the secretion of lymphokines may be involved in the pathogenesis of different types of asthma.23
Helper T lymphocytes (HTL) perform most of their effects or functions via the activity of secreted lymphokines. Cloned CD4+ HTL lines can be segregated into at least two mature subsets, termed T-Helper one (Th1) and T-Helper two (Th2), on the basis of the array of secreted lymphokines. Th1 cells secrete IL-2, IFN-g, and TNF-b, whereas Th2 cells secrete IL-4, IL-5, IL-6, IL-10, and IL-13. This distinction, based on secreted lymphokines, corresponds to a functional phenotype. Th1 cells appear to be responsible for delayed-type hypersensitivity reactions and the activation of CD8+ cytolytic T lymphocytes, whereas Th2 cells are optimal at providing help for B cell proliferation and Ab production, particularly of the IgE and IgG1 isotypes.24 The Th1/Th2 balance within an immune response is regulated by positive and negative feedback within and between, respectively, both types of cell.
Several previous studies have suggested that the Th1/Th2 equilibrium theory can be used to interpret most of the occurrences and developments of asthma.24,25 However, with advances in research, it is now known that pathophysiological conditions cannot be fully explained by this theory alone, or some characteristics could not be explained by such a theory. Accordingly, the Th1/Th2 equilibrium theory may simplify the pathogenesis of asthma, and more and more cell subsets and cytokines have been found and proposed to be involved in asthma pathogenesis and pathophysiology, such as Th17 cells, etc.26 More recent studies have reported that all these cell subsets and cytokines may participate in the development of asthma. The classic Th1/Th2 equilibrium theory has been expanded to the more comprehensive Th1/Th2/Th17/Treg system, with a more in-depth understanding of the occurrence and development of asthma.
In previous studies, IFN-γ and IL-4 were found to promote the differentiation of Th1 and Th2, respectively. Additionally, they interacted with each other to regulate the equilibrium between Th1 and Th2.27 In known asthma immune mechanisms, an increase in IL-4 causes a shift to Th2, which drives IgE antibody production, and accelerating airway pathological changes and remodeling. Meanwhile, the number of Th1 cells decreases with increased IFN-γ.28 Research on asthma has always been a hot topic, and there have been more and more advances in this area. For asthma mechanisms that could not be explained by a Th1/Th2 imbalance, the Th17/Treg imbalance (regulatory T cells) represents a major explanation for such mechanisms.29 Under the effects of TGF-β1, IL-17 participates in and induces the transformation of airway epithelial cells into interstitial cells. Nie et al. found that TGF-β1 was not only a kind of pro-fibrosis growth factor, but was also a key factor in Th17 differentiation and proliferation.30 It acts by reducing the production of IFN-γ and IL-4, as well as by directly exerting an effect on primitive T-cell precursors. However, their results were not completely consistent with ours (increased IL-4 and IL-17 expression and decreased TGF-β1 and IFN-γ), suggesting that TGF-β1 does not participate in the induction of IL-17 production.
As a minor fraction of CD4+ T cells, Tregs expressing the forkhead/winged helix transcription factor (Foxp3) plays an important role in preventing autoimmunity and maintaining self-tolerance. The effect of indoor coal PM2.5 on the expression level of Foxp3 mRNA in OVA-induced rats, which were intratracheally instilled with PM2.5 and OVA, was conducted in another study.
Jiang reported that a high expression of IL-7 provided the microenvironment for extending the survival of IL17-producing cells,31 which is in line with our results. In our study, the expression of IL-7 was same as that of IL-17. Also, although the expression of IL-7 mRNA in the pulmonary tissue showed no significant difference, it exhibited a general trend, which might be due to the small sample size. Meanwhile, IL-8 was increased in the groups producing IL-17. As a factor that could promote asthma, IL-8 has been reported to effectively decrease the amount of Neu and Eos infiltration and exudation in asthmatic Guinea pigs.32 Moreover, Song et al. reported that an increase in IL-8 was often accompanied by asthma aggravation. The signaling pathway in which the IL-17 receptor participates could promote the increase in the IL-8 level in fibroblasts and bronchial epithelial cells. In this study, there was no significant difference in the levels of IL-7 between the PM2.5 group and control group. However, the IL-17 level in the PM2.5 + OVA group was significantly higher than in the OVA group, which preliminarily indicated that the inflammatory changes caused by coal PM2.5 alone did not occur through the IL-7 pathway in normal mice. The IL-17 level was increased by promoting IL-7 gene and protein expression, which further enhanced IL-8-induced inflammation and participated in the occurrence and development of asthma.
After allergens invade the bronchial tract, plasma cells in the mucosal lamina propria produce IgE, which binds with target cells (i.e., mast cells and/or basophilic granulocyte), putting the body into the sensitization state. When the allergens enter the body again, IgE will bind with them to initiate a specific reaction.33 Many studies have reported that PM2.5 can cause increase in IgE levels and the number of lymphocytes in asthma patients. PM2.5 from various sources has different effects on different species. Coal PM2.5 alone can cause an increase in IgE and IgG1, but the inducing effect was lower than that of OVA. The IgE level in the OVA + PM2.5 group was significantly higher compared with the OVA group, but the IgG1 level was not significantly increased. This suggested that coal PM2.5 could not only induce an inflammatory response in normal mice but could also promote an increase in IgE levels in OVA-induced mice. Additionally, in this study, the effects of indoor PM2.5 from coal combustion on respiratory tract inflammation, pathophysiology and related-cytokine changes were observed. Coal PM2.5 was sufficient by itself to cause injury in the respiratory system and promoted pathological damage and related inflammatory factor changes in OVA-induced mice. Therefore, this investigation into the effects of indoor PM2.5 from the combustion of coal on the occurrence and development of asthma as well as the pathway involved could provide a theoretical basis and reference for the prevention and treatment of asthma.
5. Conclusions
Indoor PM2.5 from the combustion of coal PM2.5 was sufficient by itself to cause inflammatory cellular infiltration in pulmonary tissue, leading to organelle injury and pathophysiological structure change. Additionally, it promoted the occurrence and development of asthma through influencing IL-7 and various relevant inflammatory factor levels (such as IL-4 and IL-8) and also by changing the equilibrium between Treg and Th17 cells. During this process, as an important helper T lymphocyte, Th17 warrants further investigation.
Author contributions
Yu Jie and Xu Jie designed the study. Tang Yin, Yu Jie and Xu Jie analyzed and interpreted the data. Tang Yin conducted the laboratory work. Tang Yin and Yu Jie participated in the sample collection process. Yu Jie wrote the manuscript, and Xu Jie revised the manuscript. All authors read and approved this manuscript.
Availability of data and materials
The datasets used and/or analysed during the current study are available from the corresponding author upon request.
Conflicts of interest
The authors declare that they have no competing interests.
Acknowledgements
This work was supported by the foundation of the National Natural Science Foundation of China (81560527, 81760580); Advanced Programs of Overseas Students Science and Technology Activities, Ministry of Human Resources and Social Security of the People's Republic of China [2016](08); Key Program of the Department of Science and Technology of Guizhou Province, China (2018-142); Scientific and Technological Talent Support Program of the Educational Commission of Guizhou Province of China (KY[2018]054); Fund for Key Discipline Construction in Zunyi Medical University (2015); Scientific and Technological Fund of Department of Health of Guizhou Province, China (Fund No. gzwjkj2016-1-045, gzwjkj2017-1-053); and the Excellent Youth Science and Technique Talents of Guizhou Province [2017]5612.
References
- M. F. Jeebhay, D. Ngajilo and N. le Moual, Risk factors for nonwork-related adult-onset asthma and occupational asthma: a comparative review, Curr. Opin. Allergy Clin. Immunol., 2014, 14(2), 84–94 CrossRef CAS PubMed.
-
Y. Pei, The clinical evaluation of severity and treatment for 12 weeks of newly diagnosed patients with asthma, M.D. thesis, Dalian Medical University, Dalian, 2016 Search PubMed.
- P. J. Busse, R. D. Cohn and P. M. Salo,
et al., Characteristics of allergic sensitization among asthmatic adults older than 55 years: results from the National Health and Nutrition Examination Survey, 2005-2006, Ann. Allergy, Asthma, Immunol., 2013, 110, 247–252 CrossRef PubMed.
- J. Yu, M. I. Zaleha and J. Xu,
et al., Asthma and asthma-related symptoms among adults of an acid rain-plagued city in Southwest China: Prevalence and risk factors, Pol. J. Environ. Stud., 2013, 22(3), 717–726 Search PubMed.
- J. Yu, L. Zhang and Y. Luo,
et al., Indoor Environmental Factors Related to the Prevalence of Asthma and Asthma-Related Symptoms Among Adults During Summer in Zunyi, Guizhou Province, China, J. Environ. Health, 2016, 79(3), E1–E8 Search PubMed.
- K. Ni, E. Carter and J. J. Schauer,
et al., Seasonal variation in outdoor, indoor, and personal air pollution exposures of women using wood stoves in the Tibetan Plateau: Baseline assessment for an energy intervention study, Environ. Int., 2016, 94, 449–457 CrossRef CAS PubMed.
- D. C. Hizume, A. C. Toledo and H. T. Moriya,
et al., Cigarette smoke dissociates inflammation and lung remodeling in OVA-sensitized and challenged mice, Respir. Physiol. Neurobiol., 2012, 181(2), 167–176 CrossRef CAS PubMed.
- Z. Zhou, Y. Liu and F. Duan,
et al., Transcriptomic Analyses of the Biological Effects of Airborne PM2.5 Exposure on Human Bronchial Epithelial Cells, PLoS One, 2015, 10(9), e0138267 CrossRef PubMed.
- K. Bein and A. S. Wexler, Compositional variance in extracted particulate matter using different filter extraction techniques, Atmos. Environ., 2015, 107, 24–34 CrossRef CAS.
- E. Daley, C. Emson, C. Guignabert, R. de Waal Malefyt, J. Louten, V. P. Kurup, C. Hogaboam, L. Taraseviciene-Stewart, N. F. Voelkel, M. Rabinovitch, E. Grunig and G. Grunig, Pulmonary arterial remodeling induced by a Th2 immune response, J. Exp. Med., 2008, 205(2), 361–372 CrossRef CAS PubMed.
- B. Khor, J. D. Gagnon, G. Goel, M. I. Roche, K. L. Conway, K. Tran, L. N. Aldrich, T. B. Sundberg, A. M. Paterson, S. Mordecai, D. Dombkowski, M. Schirmer, P. H. Tan, A. K. Bhan, R. Roychoudhuri, N. P. Restifo, J. J. O'Shea, B. D. Medoff, A. F. Shamji, S. L. Schreiber, A. H. Sharpe, S. Y. Shaw and R. J. Xavier, The kinase DYRK1A reciprocally regulates the differentiation of Th17 and regulatory T cells, eLife, 2015, 4, e05920 CrossRef PubMed.
- K. Sakai, A. Yokoyama, N. Kohno and K. Hiwada, Effect of different sensitising doses of antigen in a murine model of atopic asthma, Clin. Exp. Immunol., 1999, 118, 9–15 CrossRef CAS PubMed.
- M. Das, A. Ram and B. Ghosh, Luteolin alleviates bronchoconstriction and airway hyperreactivity in ovalbumin sensitized mice, Inflammation Res., 2003, 52(3), 101–106 CrossRef CAS PubMed.
- M. Gualtieri, J. Øvrevik, J. A. Holme, M. G. Perrone, E. Bolzacchini, P. E. Schwarze and M. Camatini, Differences in cytotoxicity versus pro-inflammatory potency of different PM fractions in human epithelial lung cells, Toxicol. in Vitro, 2010, 24(1), 29–39 CrossRef CAS PubMed.
- E. A. Kelly, R. R. Rodriguez, W. W. Busse and N. N. Jarjour, The effect of segmental bronchoprovocation with allergen on airway lymphocyte function, Am. J. Respir. Crit. Care Med., 1997, 156, 1421–1428 CrossRef CAS PubMed.
- Y. Lin, J. Zou, W. Yang and C.-Q. Li, A Review of Recent Advances in Research on PM2.5 in China, Int. J. Environ. Res. Public Health, 2018, 15(3), 438 CrossRef PubMed.
- S. Semple, C. Garden and M. Coggins,
et al., Contribution of solid fuel, gas combustion or tobacco smoke to indoor air pollutant concentrations in Irish and Scottish homes, Indoor Air, 2012, 22(3), 212–223 CrossRef CAS PubMed.
- Y. Jie, H. Houjin and M. Xun,
et al., Relationship between pulmonary function and indoor air pollution from coal combustion among adult residents in an inner-city area of southwest China, Braz. J. Med. Biol. Res., 2014, 47(11), 982–989 CrossRef CAS PubMed.
- Z. A. Chafe, M. Brauer and Z. Klimont,
et al., Household Cooking with Solid Fuels Contributes to Ambient PM2.5 Air Pollution and the Burden of Disease, Environ. Health Perspect., 2014, 122(12), 1314–1320 CrossRef CAS PubMed.
- M. Kudo, Y. Ishigatsubo and I. Aoki, Pathology of asthma, Front. Microbiol., 2013, 4, 263 Search PubMed.
- S. M. Burburan, D. G. Xisto and H. C. Ferreira,
et al., Lung mechanics and histology during sevoflurane anesthesia in a model of chronic allergic asthma, Anesth. Analg., 2007, 104(3), 631–637 CrossRef CAS PubMed.
- M. Kämpe, M. Vosough, A. Malinovschi, M. Alimohammadi, K. Alving, B. Forsberg, J. Lötvall, R. Middelveld, B. Dahlén and C. Janson, Upper airway and skin symptoms in allergic and non-allergic asthma: Results from the Swedish GA2LEN study, J. Asthma, 2017, 55(3), 275–283 CrossRef PubMed.
- C. Apert, P. Romagnoli and J. P. M. van Meerwijk, IL-2 and IL-15 dependent thymic development of Foxp3-expressing regulatory T lymphocytes, Protein Cell, 2018, 9(4), 322–332 CAS.
- O. Kaminuma, M. Saeki, T. Nishimura, N. Kitamura, N. Watanabe, T. Hiroi and A. Mori, Differential Contribution of Adhesion Molecules to Th1 and Th2 Cell-Mediated Lung and Bowel Inflammation, Biol. Pharm. Bull., 2017, 40(10), 1801–1805 CrossRef CAS PubMed.
- N. F. Ji, Y. C. Xie and M. S. Zhang,
et al., Ligustrazine corrects Th1/Th2 and Treg/Th17 imbalance in a mouse asthma model, Int. Immunopharmacol., 2014, 21(1), 76–81 CrossRef CAS PubMed.
- J. F. Alcorn, C. R. Crowe and J. K. Kolls, TH17 cells in asthma and COPD, Annu. Rev. Physiol., 2010, 72(72), 495–516 CrossRef CAS PubMed.
- O. Abehsira-Amar, M. Gibert and M. Joliy,
et al., IL-4 plays a dominant role in the differential development of Tho into Th1 and Th2 cells, J. Immunol., 1992, 148(12), 3820–3829 CAS.
- Y. C. Lee and S. H. Kim, Immunomodulatory effect of Juglans sinensis, Psoralea corylifolia, Cheong-a-hwan extract and cyclosporine A on Th1(IFN-γ)/Th2(IL-4) cytokine balance, eosinophil accumulation in a murine model of asthma, Phytochem. Lett., 2008, 1(1), 6–10 CrossRef CAS.
- L. Cosmi, F. Liotta and E. Maggi,
et al., Th17 cells: new players in asthma pathogenesis, Allergy, 2011, 66(8), 989–998 CrossRef CAS PubMed.
- Q. R. Nie, X. J. An, X. M. Liu and W. Y. Zhang, Study between the expression of IL-17 on the airway and the airway remolding of bronchial asthma patient, Chin. J. Asthma, 2010, 4(3), 129–132 CAS.
-
M. Jiang, Potential contribution of Thelper type 17 survival to neutrophilic airway inflamation in asthma, Ph.D thesis, Guangxi Medical University, Nanning, 2013 Search PubMed.
- K. Lv, H. Qi and Y. Wang,
et al., The Relationship between Interleukin 8 and Airway Hyperresponsiveness, Chin. J. Tuberc. Respir. Dis., 1997, 20(5), 280–282 Search PubMed.
- D. Dunea, S. Iordache and H.-Y. Liu,
et al., Quantifying the impact of PM2.5 and associated heavy metals on respiratory health of children near metallurgical facilities, Environ. Sci. Pollut. Res., 2016, 23, 15395–15406 CrossRef CAS PubMed.
Footnote |
† Electronic supplementary information (ESI) available. See DOI: 10.1039/c8tx00221e |
|
This journal is © The Royal Society of Chemistry 2019 |