Diallyl sulfide treatment protects against acetaminophen-/carbon tetrachloride-induced acute liver injury by inhibiting oxidative stress, inflammation and apoptosis in mice
Received
14th July 2018
, Accepted 25th October 2018
First published on 25th October 2018
Abstract
The purpose of the present study was to investigate the effects and underlying mechanisms of diallyl sulfide (DAS), an organosulfur compound extracted from garlic, on drug-induced or chemical-induced liver injury caused by acetaminophen (APAP) or carbon tetrachloride (CCl4) in mice. DAS (100, 200, or 400 μmol kg−1) was orally administered 1 hour before APAP or CCl4 intraperitoneal injection, and the serum and liver tissue were collected 24 hours after APAP or CCl4 exposure. The serum aminotransferase activities and liver histopathological examination showed that DAS exhibited obvious hepatoprotective effects against acute liver injury induced by APAP or CCl4. In addition, exposure to APAP or CCl4 resulted in an increased content of malonaldehyde as well as a decreased ratio of reduced to oxidized glutathione, and a decreased level of superoxide dismutase and catalase activity in the liver (p < 0.05); however, pretreatment with DAS restored the perturbations of the antioxidant system in the liver. Beyond that, DAS pretreatment reduced the APAP-/CCl4-induced increase in phosphorylation of inhibitor of kappa B alpha (IκBα) and p65 subunit of nuclear factor kappa B (NF-κB) expression in the cytoplasm and nucleus in the liver. DAS pretreatment also decreased the excessive level of TNF-α caused by APAP or CCl4 in serum (p < 0.05). Moreover, DAS pretreatment regulated the expression of cleaved caspase 3, Bax and Bcl-2 in the liver and suppressed APAP-/CCl4-induced hepatocyte apoptosis. In conclusion, DAS exhibits hepatoprotective effects against drug-induced and chemical-induced liver injuries induced by APAP or CCl4 in mice, probably due to its ability to reduce hepatic oxidative stress and inhibit inflammatory injury and hepatocyte apoptosis.
1. Introduction
The liver, playing important roles in metabolism and detoxification in the human body, is one of the most sensitive organs to a series of stimuli, including viral infection, autoimmune disease and various chemical toxins. Excessive stimulation often leads to acute liver injury, which may result in life-threatening clinical problems, such as serious blood coagulation disorders, and eventually develop into acute hepatic failure.1 Although the rate of hepatic failure survival has improved substantially in recent years due to critical care management and emergency liver transplantation, more challenges still exist in finding more efficient therapeutic treatments for acute liver injury and preventing the progression of acute liver injury into acute hepatic failure.2,3 Nowadays, large numbers of synthetic and phytochemicals exhibit protective effects against acute liver injury, however, the application of most of these agents is limited due to a lack of geographical distribution. It is necessary to find a widely accepted and abundant agent from our daily life to protect against acute liver injury. Garlic (Allium sativum L.), a global cultivated plant, can be found everywhere and is used as a spice or condiment in various raw or cooked dishes. It possesses various beneficial effects and protects against several diseases, such as microbial infections, hyperlipidemia, heart disease and acute or chronic liver injury.4,5 The various pharmacological activities exhibited by garlic are highly related to the various organosulfur compounds produced by metabolic transformation. When raw garlic is crushed or cut, the vacuolar enzyme allinase is released into the cytoplasm and converts alliin into diallyl thiosulfinate (allicin), which exerts a variety of pharmacological activities.6,7 However, allicin is unstable and readily decomposed into oil-soluble allyl sulfur compounds, including diallyl sulfide (DAS), diallyl disulfide (DADS) and diallyl trisulfide (DATS).5,8 These three diallyl organosulfur compounds (DAS, DADS and DATS) have similar chemical structures and also show similar biological activities,9,10 but DAS has the lowest toxicity (LD50 > 2000 mg per kg body weight, mouse, oral) and shows better stability than DADS and DATS in nature. Therefore, DAS may have broader application value than DADS and DATS in alleviating acute liver injury.
Acetaminophen (APAP) and carbon tetrachloride (CCl4) are two well-known hepatotoxins that can induce acute liver injury in animals.11,12 APAP is a widely used antipyretic and analgesic drug, which can induce serious drug-induced acute liver injury when taken as an overdose, while CCl4 was once a popular solvent in organic chemistry and can be used to establish a chemically induced acute liver injury model. Nowadays, the acute liver injury models induced by these two compounds have been widely used to screen hepatoprotective agents. We selected these two compounds to clarify the effects and mechanisms of DAS on drug-induced and chemical-induced acute liver injury. Previous studies only focused on the fact that DAS, as a competitive inhibitor of cytochrome P450 2E1 (CYP 2E1), exhibits protective effects against APAP-/CCl4-induced acute liver injury by reducing metabolic activation.13,14 In contrast, a growing number of studies have shown that DAS protects against various diseases due to its anti-inflammatory and antioxidative effects.15,16 In earlier reports, DAS was shown to attenuate fipronil or thallium-induced oxidative injury via enhancing tissue antioxidant defenses in rats.17,18 In another study, it was also found that DAS can inhibit gentamicin-induced nephrotoxicity by enhancing antioxidases and suppressing the release of inflammatory cytokines.19 Obviously, previous research studies have neglected the role of the anti-inflammatory and antioxidative capabilities of DAS in the process of inhibiting acute liver injury. Therefore, we hypothesized that DAS can exert protective effects against APAP-/CCl4-induced acute liver injury by inhibiting inflammation and oxidative stress in mice, and the current study was designed to investigate the protective effects and underlying mechanisms of DAS on drug-induced and chemical-induced acute liver injury induced by APAP and CCl4 in mice.
2. Materials and methods
2.1 Materials
Diallyl sulfide (DAS) (purity >97%) was purchased from Sigma-Aldrich Co. (St Louis, MO, USA). CCl4 and APAP were purchased from Aladdin® (Shanghai, China). Alanine aminotransferase (ALT) and aspartate aminotransferase (AST) assay kits were obtained from MedicalSystem Biotechnology (Ningbo, China). The malondialdehyde (MDA), reduced/oxidized glutathione (GSH/GSSG), superoxide dismutase (SOD) and catalase (CAT) kits were obtained from Beyotime Biotechnology (Zhejiang, China). The mouse tumor necrosis factor-alpha (TNF-α) enzyme-linked immunosorbent assay (ELISA) kit was purchased from R&D Systems (Minneapolis, MN, USA). The In situ Cell Death Detection Kit, POD (TUNEL) kit was purchased from Roche (Roche Molecular Biochemicals, Germany). The BCATM protein assay kit was purchased from Pierce Biotechnology (Rockford, IL, USA). Western blotting detecting reagent (ECL kits) was provided by Millipore (Bedford, MA, USA). All other chemicals were of the highest quality commercially available.
2.2 Animals
Specific pathogen-free male ICR mice (18–22 g) were provided by the Laboratory Animal Center of Shandong University. The animal room was maintained at approximately 22 °C and 50% humidity with a 12 h light/12 h dark cycle and the mice had free access to standard chow and tap water. All experiments were carried out in accordance with the NIH Guide for Care and Use of Laboratory Animals and the use of animals was in accordance with the guidelines established by the Animal Care Committee of Shandong University.
2.3 Experiment design
The model of acute liver injury induced by APAP was generated as previously described.20,21 In detail, after 5 days of acclimatization, the animals were randomly divided into six groups with 12 mice in each group: (1) control, (2) DAS, (3) APAP model, (4) APAP combined with 100 μmol kg−1 DAS, (5) APAP combined with 200 μmol kg−1 DAS, (6) APAP combined with 400 μmol kg−1 DAS. After fasting for 12 hours (overnight), the mice in the APAP group and APAP plus DAS groups were orally administered with corn oil or DAS (100, 200, or 400 μmol per kg body weight, dissolved in corn oil), and one hour later, the animals were intraperitoneally injected with a single dose of APAP (300 mg per kg body weight, dissolved in sterile saline, 20 ml per kg body weight). The animals in the control group were given an equal volume of corn oil and saline, while the mice in the DAS group were given an equal volume of DAS (400 μmol kg−1). The mice were anesthetized and sacrificed 24 hours after APAP injection, and serum and liver tissue were immediately collected. A small portion of the liver tissues was fixed in 4% paraformaldehyde solution, while the remaining tissues were frozen in liquid nitrogen and then stored at −80 °C until analysis.
In another experiment, a model of CCl4-induced acute liver injury was created as previously described.22,23 The animal grouping and experimental procedure were similar to the APAP experiments, except that the APAP was substituted by CCl4. A single dose of 0.5% CCl4 (0.5 ml CCl4 dissolved in 100 ml oil, 10 ml per kg body weight) was used to induce acute liver injury by intraperitoneal injection. The mice were anesthetized and sacrificed 24 hours after CCl4 injection, and serum and liver tissue were immediately collected. A small portion of the liver tissues was fixed in 4% paraformaldehyde solution, while the remaining tissues were frozen in liquid nitrogen and then stored at −80 °C until analysis.
2.4 Serum biochemical analyses
The activities of the serum ALT and AST were measured using an Automatic Biochemical Analyzer (AU480, Backman) according to the instructions provided by the commercial assay kits.
2.5 Histopathological examination
The liver tissues from the same lobes in each animal were fixed in 4% paraformaldehyde for 24 hours, dehydrated in alcohol and then embedded in paraffin. The liver sections (5 μm) were stained with hematoxylin and eosin (H&E) following a standard protocol. The histopathological changes of each mouse were examined with an optical microscope.
2.6 Determination of hepatic lipid peroxidation
Haptic lipid peroxidation (LPO) was determined by the thiobarbituric acid reactive substances method (TBARS) and was expressed as MDA concentration, which was assayed using an MDA assay kit (Beyotime) according to the manufacturer's instructions. The absorbance was measured at 532 nm using a Microplate Spectrophotometer (Infinite 200 PRO, TECAN Inc. Switzer) as previously reported,24 and the results were expressed as mmol per mg-protein.
2.7 Assay of the changes in the antioxidant system in the liver
The ratio of GSH/GSSG and the activity of SOD and CAT in the liver were measured by commercial assay kits (Beyotime) and using a Microplate Spectrophotometer to obtain optical density, and the results are expressed as 100%, U per mg-protein, and U per mg-protein, respectively. All procedures were performed strictly according to the instructions of the commercial assay kits.
2.8 Enzyme-linked immunosorbent assay (ELISA)
The level of TNF-α in the serum was measured using an ELISA kit according to the manufacturer's protocol (R&D Systems) and the results were expressed as pg ml−1. Briefly, standards or samples (100 μl) were added to antibody-coated 96-well plates and incubated for 2 hours at room temperature. The plate was then washed and the samples were incubated in detection antibody for 2 hours. Then the plate was washed and incubated in horseradish peroxidase-conjugated streptavidin for 20 min at room temperature. The substrate solution (tetramethylbenzidine) was added, and incubation was continued at room temperature in the dark for 30 min, until the reaction was stopped using 2 N sulfuric acid. The optical density was measured at 450 nm using a Microplate Spectrophotometer. TNF-α concentrations were calculated relative to the appropriate standard curve.
2.9 Terminal-deoxynucleotidyl transferase mediated nick end labeling (TUNEL) assay
Liver tissues were fixed for at least 24 hours in 4% paraformaldehyde, and then dehydrated in graded ethanol, and embedded in paraffin. Paraffin sections (5 μm) were incubated in TUNEL reaction mixture from a kit according to the manufacturer's instructions. Briefly, after 25 min of incubation at 37 °C with proteinase K solution, the sections were incubated with TUNEL solution for 60 min at 37 °C in a moist and dark environment. Subsequently, the sections were incubated with converter-POD solution in a humidified environment at 37 °C for 30 min. After that, TUNEL-positive cells were stained with diaminobenzidine tetrahydrochloride (DAB) and the sections were counterstained with hematoxylin. The sections were photographed using an optical microscope with a computer-aided imaging system.
2.10 Western blotting analysis
Western blotting analysis was performed as previously described.25 Primary antibodies against IKB-α, p-IKB-α, NF-κB p65, cleaved caspase 3, Bax and Bcl-2 were provided by Cell Signaling Technology (Beverly, MA, USA). Primary antibodies against Lamin B and β-actin were purchased from Proteintech (Wuhan, China). The membranes were exposed to X-ray film to get the protein bands. The immunoreactive bands of proteins were scanned using an Agfa Duoscan T1200 scanner, and the digitized data were quantified as integrated optical density (IOD) using the Kodak Imaging Program. To ensure equal loading, the results were normalized to β-actin or Lamin B.
2.11 Statistical analysis
Data were expressed as mean ± SEM. SPSS 20.0 statistical software was used for statistical analysis. Data were analyzed using one-way analysis of variance (ANOVA) followed by Tukey's multiple comparison tests. Differences between groups were considered statistically significant at p < 0.05.
3. Results
3.1 DAS treatment protected against APAP-/CCl4-induced acute liver injury
As shown in Fig. 1, APAP or CCl4 treatment significantly increased the activities of ALT and AST in serum compared with the control group (p < 0.05), while no significant difference in serum aminotransferase activity was found between the control group and the DAS group (p > 0.05). However, the increased activities of serum AST and ALT were significantly reversed by pretreatment with different dosages of DAS in a dose-dependent manner (p < 0.05). The histopathological examination showed that the liver injury model groups induced by APAP or CCl4 showed obvious pathological changes such as extensive hemorrhage, necrosis, and infiltration of inflammatory cells. Interestingly, these pathological changes were significantly ameliorated or even vanished following pretreatment with DAS (Fig. 1C).
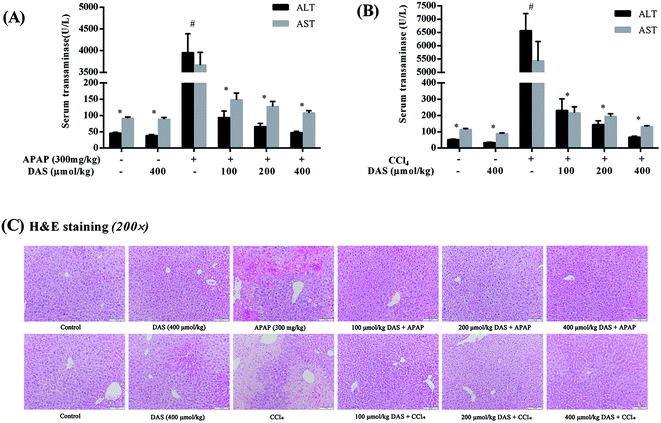 |
| Fig. 1 Effects of DAS on APAP-/CCl4-induced acute liver injury and histopathological alterations. Mice were pretreated with vehicle or various dosages of DAS (100, 200 or 400 μmol kg−1) at 1 h before APAP (300 mg per kg bw) or CCl4 (0.5%, dissolved in corn oil) exposure. The serum activities of ALT and AST were measured at 24 h after intraperitoneal injection of APAP (A) or CCl4 (B); all values are expressed as mean ± SEM (n = 12). *p < 0.05 compared with the APAP group; #p < 0.05 compared with the control group. (C) Liver samples were harvested at 24 h after APAP or CCl4 injection, and the same lobes of the liver in each animal were fixed in 4% paraformaldehyde, embedded in paraffin, sectioned (5 μm), and then stained with H&E for histological observation and representative liver sections of each group are shown (original magnification, 200× and scale bar is 100 μm). | |
3.2 DAS treatment protected against APAP-/CCl4-induced oxidative stress in the liver
As shown in Fig. 2, APAP or CCl4 exposure induced severe lipid peroxidation in the liver as expressed by the significantly increased content of MDA compared with the control group (p < 0.05). Different dosages of DAS, however, were able to efficiently inhibit APAP-/CCl4-induced lipid peroxidation and reduce the MDA content in a dose-dependent manner (p < 0.05). Additionally, the activities of SOD and CAT in the liver were significantly decreased after APAP or CCl4 exposure compared with the control group (p < 0.05), while DAS pretreatment significantly boosted the activities of SOD and CAT compared with the APAP or CCl4 group (p < 0.05). Beyond that, APAP or CCl4 exposure led to a significant decrease in the concentration of GSH and an increased concentration of GSSG in the liver, and the ratio of GSH/GSSG was correspondingly significantly reduced compared with the control group (p < 0.05). However, DAS pretreatment significantly restored the GSH concentration and increased the ratio of GSH/GSSH in the liver compared with the APAP or CCl4 group (p < 0.05).
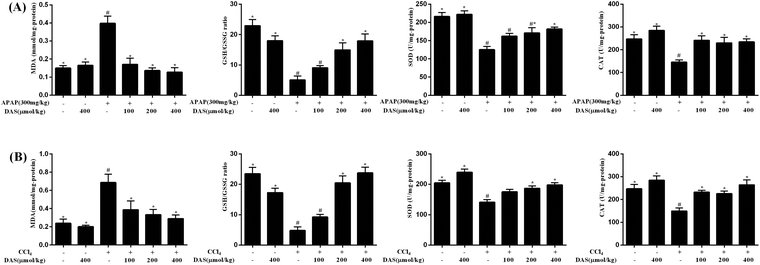 |
| Fig. 2 Effects of DAS on APAP-/CCl4-induced hepatic oxidative stress. Mice were pretreated with vehicle or various dosages of DAS (100, 200, 400 μmol kg−1) at 1 h before APAP (300 mg per kg bw) or CCl4 (0.5%, dissolved in corn oil) intraperitoneal injection, and the levels of MDA, GSH/GSSG, SOD and CAT in the liver were measured at 24 h after APAP (A) or CCl4 (B) exposure. All values are expressed as mean ± SEM of eight animals per group; *p < 0.05 compared with the APAP or CCl4 group; #p < 0.05 compared with the control group. | |
3.3 DAS treatment protected against APAP-/CCl4-induced inflammation in mice
As shown in Fig. 3A and B, no significant difference in the TNF-α level in the serum was found between the control group and DAS group (p > 0.05). However, APAP or CCl4 exposure significantly increased the level of TNF-α in the serum (vs. control, p < 0.05). Compared with the APAP or CCl4 group, pretreatment with DAS (100, 200, of 400 μmol kg−1) significantly decreased the level of TNF-α in a dose-dependent manner (p < 0.05). In addition, changes in protein expression in the nuclear factor kappa B (NF-κB) signaling pathway were detected using western blotting. As shown in Fig. 3C and D, the expression of inhibitor of kappa B alpha (IκBα) in the cytoplasm was markedly decreased after APAP or CCl4 exposure and different doses of DAS pretreatment prevented this degradation in a dose-dependent manner (p < 0.05). Inversely, the phosphorylation of IκBα and the ratio of p-IκBα/IκBα were dramatically increased after APAP or CCl4 exposure, which was inhibited by DAS in a dose-dependent manner (p < 0.05). APAP or CCl4 exposure markedly increased the amount of NF-κB p65 subunit in the cytoplasm and nucleus (p < 0.05); however, pretreatment with DAS normalized the level of NF-κB p65 in the cytoplasm and nucleus.
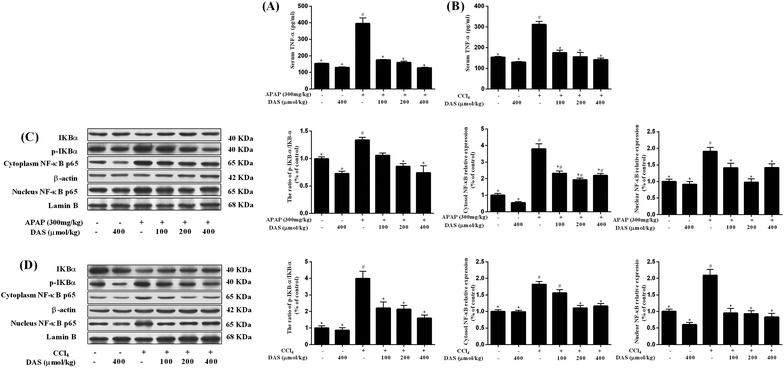 |
| Fig. 3 Effects of DAS on the APAP-/CCl4-induced inflammatory response. The level of TNF-α in serum was measured at 24 h after APAP (A) or CCl4 (B) exposure. All values are expressed as mean ± SEM of eight animals per group; *p < 0.05 compared with the APAP or CCl4 group; #p < 0.05 compared with the control group. Western blotting analysis of cytoplasm IKBα, p-IKBα, NF-κB p65 and nuclear NF-κB p65 after APAP (C) or CCl4 (D) treatment, followed by the integrity optical density analyses of p-IKBα/IKBα, cytoplasm NF-κB p65 and nuclear NF-κB p65. β-Actin and lamin b were used as an invariant control to verify equal protein loading. All values are expressed as mean ± SEM from at least 3 independent experiments and expressed as a percentage of the control; *p < 0.05 compared with the APAP or CCl4 group; #p < 0.05 compared with the control group. | |
3.4 DAS treatment protected against APAP-/CCl4-induced hepatocyte apoptosis
As shown in Fig. 4A, no TUNEL-positive hepatocytes were observed in the control or DAS group, but APAP or CCl4 exposure significantly increased hepatocyte apoptosis compared with the control group. In contrast, different dosages of DAS pretreatment markedly inhibited APAP-/CCl4-induced hepatocyte apoptosis. In addition, the apoptosis-related protein expressions of cleaved caspase 3, Bax and Bcl-2 in liver tissue were also detected using western blotting. As shown in Fig. 4B, compared with the control group, APAP treatment significantly increased the protein expression of cleaved caspase 3 and Bax by 82.3% and 61.2%, and decreased the protein expression of Bcl-2 by 40.5% in the liver (p < 0.05). Although the Bax expression was not altered after pretreatment with DAS, DAS pretreatment significantly decreased cleaved caspase 3 expression and increased Bcl-2 expression compared with the APAP group (p < 0.05). Beyond that, as shown in Fig. 4C, compared with the control group, CCl4 exposure significantly increased the protein expression of cleaved caspase 3 and Bax by 137.7% and 88.9%, and decreased the protein expression of Bcl-2 by 45.6% in the liver (p < 0.05). However, pretreatment with DAS significantly reversed the increased expression of cleaved caspase 3 and Bax and the decreased expression of Bcl-2 caused by CCl4 exposure compared with the CCl4 group (p < 0.05).
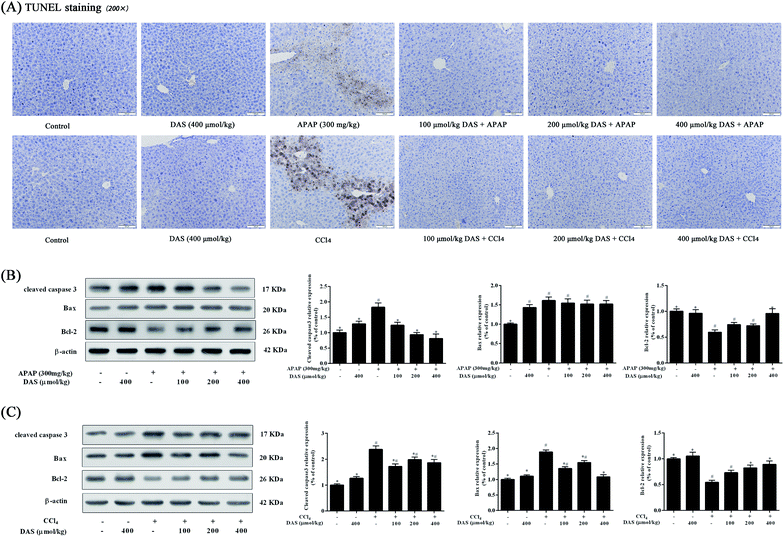 |
| Fig. 4 Mice were pretreated with vehicle or various dosages of DAS (100, 200 or 400 μmol kg−1) at 1 h before APAP (300 mg kg per bw) or CCl4 (0.5%, dissolved in corn oil) injection, and the liver tissue was harvested at 24 hours after APAP or CCl4 intraperitoneal injection for TNUEL staining and western blotting. (A) The TUNEL staining was used to observe hepatocyte apoptosis and representative liver sections of each group are shown (original magnification, 200× and scale bar is 100 μm). The protein expression of cleaved caspase 3, Bax and Bcl-2 in liver tissue from the APAP (B) or CCl4 (C) groups were detected by western blotting, and β-actin was used as an invariant control for equal loading, followed by the integrity optical density analyses of cleaved caspase 3, Bax, Bcl-2. All values are expressed as mean ± SEM from at least 3 independent experiments, and expressed as a percentage of the control; *p < 0.05 compared with the APAP group, #p < 0.05 compared with the control group. | |
4. Discussion
In the present study, two different types of animal model were used to explore the effects and mechanisms of DAS on acute liver injury. APAP-induced liver injury is widely used to explore novel liver-protective agents for drug-induced liver injury.12 APAP is bio-activated to its toxic intermediate N-acetyl-p-aminophenol (NAPQI) by cytochrome P450 enzymes, including CYP2E1 and CYP1A2, and excess NAPQI causes significant depletion of glutathione and triggers mitochondrial oxidative stress, which ultimately initiates hepatocellular apoptosis and/or necrosis.26 Beyond that, the necrosis of hepatocytes releases a variety of cellular contents, some of which can function as damage-associated molecular patterns (DAMPs), which can initiate an inflammatory response that aggravates liver injury.27 In contrast, CCl4, as an exogenous chemical, is widely used to induce chemical-induced liver injury in animals because of its hepatotoxicity.28 CCl4 is also initially converted into trichloromethyl radicals (CCl3˙) and trichloromethyl peroxyl radicals (OOCCl3˙) by cytochrome P450 enzymes, especially CYP 2E1. The produced radicals can lead to enzyme activity disruption, membrane dysfunction and lipid peroxidation, and then generate reactive oxygen species (ROS), ultimately causing hepatocyte degeneration, necrosis and liver dysfunction.29,30 Necrosis of hepatocytes further releases inflammatory mediators such as cellular contents and protein degradation products, which can activate Kupffer cells and then generate large amounts of cytokines, such as TNF-α and IL-β, which can recruit circulating neutrophils and monocytes into the liver, resulting in inflammation-mediated hepatocyte injury.31 Thus, metabolic activation, oxidative stress and inflammatory damage are involved in the injury mechanisms of these two different types of acute liver injury. Previous studies have only focused on the protective effects of DAS against APAP-/CCl4-induced acute liver injury by inhibiting CYP 2E1-mediated metabolic activation, and the role of other protective biological activities of DAS in preventing acute liver injury induced by APAP or CCl4 has received little attention.13,14 Therefore, the present study was undertaken to investigate the protective effects of DAS on APAP-/CCl4-induced acute liver injury and elucidate whether the antioxidant and anti-inflammatory effects of DAS are involved in this protective process in mice.
The serum activities of ALT and AST, acting as sensitive indicators, are widely used to reflect hepatic damage.32 Previous research has shown that APAP or CCl4 exposure can significantly increase the activity of ALT and AST in serum.33,34 In our study, APAP or CCl4 treatment induced a dramatic increase in ALT and AST activity in the serum; however, DAS pretreatment prevented the elevation of serum ALT and AST in a dose-dependent manner and showed a prominent protective effect against acute liver injury. Even with a lower dose of DAS (100 μmol kg−1 equal to 11.4 mg kg−1), it was still able to exhibit remarkable hepatoprotective effects. Meanwhile, the severe pathological lesions were significantly ameliorated or even vanished following DAS pretreatment.
Oxidative stress is closely correlated with APAP-/CCl4-induced acute liver injury.23,35 However, there is a lack of information about the changes in the antioxidant system in the process of DAS inhibition of APAP-/CCl4-induced acute liver injury. MDA, a product of lipid peroxidation, was detected to reflect the liver oxidative damage. Concordant with previous observations,34,36 our results revealed that the administration of APAP or CCl4 induced a significant increase in the MDA content in the liver, indicating that the liver suffered severe oxidative damage. However, DAS pretreatment significantly attenuated liver oxidative damage induced by APAP or CCl4 as evidenced by the reduced content of MDA in the liver. GSH can protect cells from oxidative damage by scavenging free radicals and other oxygen species through non-enzymatic and enzymatic processes,37 and the ratio of reduced to oxidized glutathione (GSH/GSSG) is also widely used to reflect cellular toxicity and oxidative stress.38 Our finding of a markedly lower ratio of GSH/GSSG in the APAP or CCl4 group indicated a potential redox imbalance and higher vulnerability to oxidative stress; however, DAS pretreatment significantly increased the content of GSH and reversed the low GSH/GSSG ratio in the liver, indicating the alleviation of oxidative stress. In addition to GSH, antioxidases also play key roles in the antioxidant systems, and SOD and CAT are involved in the antioxidant system by scavenging oxygen radical species and promoting the decomposition of hydrogen peroxide. Previous studies have found that APAP-/CCl4-induced liver oxidative damage is often accompanied by a decrease in liver antioxidase activity.39 Significantly decreased activities of SOD and CAT were observed after APAP or CCl4 treatment in our results, but all of the found perturbations of antioxidases could be restored by pretreatment with different dosages of DAS. Thus, our results suggest that DAS was able to alleviate APAP-/CCl4-induced liver oxidative damage by regulating the antioxidant system.
The cellular contents released from dead cells due to oxidative damage in the liver can activate the NF-κB pathway in Kupffer cells, which secrete large amounts of proinflammatory cytokines, such as TNF-α and IL-1β, leading to inflammatory damage.40,41 Previous studies have shown that the acute liver injury induced by APAP or CCl4 is significantly alleviated by suppressing the level of TNF-α in the serum.39,42 In our study, APAP or CCl4 treatment induced a significant increase in the TNF-α level in the serum. Nevertheless, pretreatment with different dosages of DAS significantly decreased the serum content of TNF-α in a dose-dependent manner. The secretion of TNF-α is regulated by the NF-κB pathway. It has been shown that the NF-κB pathway is markedly activated in APAP-/CCl4-induced acute liver injury, and the inhibition of the NF-kB pathway can effectively reduce APAP-/CCl4-induced inflammatory damage.43,44 Our results also showed that DAS treatment significantly suppressed the phosphorylation of IκBα, reducing its ubiquitination and proteasomal degradation. Meanwhile, the excessive NF-κB p65 transcriptional expression caused by APAP or CCl4 in the cytoplasm and nucleus was significantly inhibited by DAS treatment. Thus, we believe that DAS is able to mitigate inflammatory injury in these two different types of acute liver injury by inhibiting the NF-κB pathway. Beyond that, previous studies have shown that the inflammatory cytokine TNF-α can further induce hepatocyte apoptosis via a TNF-receptor mediated pathway.45 Therefore, we inferred that DAS may also exert a protective effect against acute liver injury by reducing hepatocyte apoptosis.
Hepatocyte apoptosis, a pivotal pathological manifestation of acute liver injury, is an important target for preventing liver injury.46,47 Previous studies have shown that a significant increase of hepatocyte apoptosis is observed in acute liver injury induced by APAP or CCl4, and accumulating evidence has demonstrated that these different types of acute liver injury can be alleviated by inhibiting hepatocyte apoptosis.48–51 Consistent with previous research, our results showed that APAP or CCl4 exposure obviously increased the number of apoptotic hepatocytes, as evidenced by a large number of TUNEL-positive cells in liver sections. However, TUNEL-positive cells were significantly reduced or disappeared after pretreatment with different doses of DAS. These effects were consistent with the improvement of serum biomarkers and liver antioxidant enzyme activity, which further demonstrated that DAS pretreatment significantly alleviated APAP-/CCL4-induced acute liver injury by suppressing hepatocyte apoptosis. Consistent with the TUNEL staining results, western blotting analysis revealed that the expression of cleaved caspase 3 in the APAP or CCl4 group was notably increased, while the ratio of Bcl-2/Bax was reduced. In contrast, after pretreatment with DAS at different dosages, the expression of cleaved caspase 3 was down-regulated, and the ratio of Bcl-2/Bax was significantly increased in the liver tissue compared with the APAP or CCl4 group. These results indicate that DAS inhibited APAP-/CCl4-induced hepatocyte apoptosis by regulating the expression of cleaved caspase 3, Bcl-2 and Bax in the liver, which in turn contributed to the protective effects of different types of acute liver injury induced by APAP or CCl4.
In conclusion, we used APAP and CCl4 to mimic drug-induced and chemical-induced acute liver injury. The results clearly indicate that DAS exhibits remarkable hepatoprotective effects against acute liver injury induced by APAP or CCl4 in mice, and the hepatoprotective mechanisms of DAS against these two different types of acute liver injury may be related to its ability to alleviate oxidative stress, and to suppress inflammatory injury and hepatocyte apoptosis. These results also suggest that DAS may exhibit protective effects against other types of acute liver injury, such as those induced by lipopolysaccharide or concanavalin A, through the same mechanisms, which is what we will verify in the future. In addition, compared with other antioxidants, DAS is one of the most common agents with a simple chemical structure in our daily life, so it may have broad application prospects, and our research provides a reasonable basis for the application of DAS in acute liver injury and for the further development of DAS or garlic in medicine.
Conflicts of interest
There are no conflicts of interest to declare.
Acknowledgements
This work was supported by grants from the National Natural Science Foundation of China (No. 81373044).
References
- W. Bernal and J. Wendon, Acute liver failure, N. Engl. J. Med., 2013, 369, 2525–2534 CrossRef CAS PubMed.
- W. Bernal, A. Hyyrylainen, A. Gera, V. K. Audimoolam, M. J. McPhail, G. Auzinger, M. Rela, N. Heaton, J. G. O'Grady, J. Wendon and R. Williams, Lessons from look-back in acute liver failure? A single centre experience of 3300 patients, J. Hepatol., 2013, 59, 74–80 CrossRef PubMed.
- W. Bernal, G. Auzinger, A. Dhawan and J. Wendon, Acute liver failure, Lancet, 2010, 376, 190–201 CrossRef.
- M. S. Butt, M. T. Sultan, M. S. Butt and J. Iqbal, Garlic: nature's protection against physiological threats, Crit. Rev. Food Sci. Nutr., 2009, 49, 538–551 CrossRef CAS PubMed.
- S. V. Rana, R. Pal, K. Vaiphei, S. K. Sharma and R. P. Ola, Garlic in health and disease, Nutr. Res. Rev., 2011, 24, 60–71 CrossRef CAS PubMed.
- M. M. Abdel-Daim, N. K. Abdelkhalek and A. M. Hassan, Antagonistic activity of dietary allicin against deltamethrin-induced oxidative damage in freshwater Nile tilapia; Oreochromis niloticus, Ecotoxicol. Environ. Saf., 2015, 111, 146–152 CrossRef CAS PubMed.
- M. M. Abdel-Daim, O. E. Kilany, H. A. Khalifa and A. A. M. Ahmed, Allicin ameliorates doxorubicin-induced cardiotoxicity in rats via suppression of oxidative stress, inflammation and apoptosis, Cancer Chemother. Pharmacol., 2017, 80, 745–753 CrossRef CAS PubMed.
- M. Iciek, I. Kwiecien and L. Wlodek, Biological properties of garlic and garlic-derived organosulfur compounds, Environ. Mol. Mutagen., 2009, 50, 247–265 CrossRef CAS PubMed.
- H. M. Yun, J. O. Ban, K. R. Park, C. K. Lee, H. S. Jeong, S. B. Han and J. T. Hong, Potential therapeutic effects of functionally active compounds isolated from garlic, Pharmacol. Ther., 2014, 142, 183–195 CrossRef CAS PubMed.
- M. J. Guan, N. Zhao, K. Q. Xie and T. Zeng, Hepatoprotective effects of garlic against ethanol-induced liver injury: A mini-review, Food Chem. Toxicol., 2018, 111, 467–473 CrossRef CAS PubMed.
- D. Scholten, J. Trebicka, C. Liedtke and R. Weiskirchen, The carbon tetrachloride model in mice, Lab. Anim., 2015, 49, 4–11 CrossRef CAS PubMed.
- J. C. Mossanen and F. Tacke, Acetaminophen-induced acute liver injury in mice, Lab. Anim., 2015, 49, 30–36 CrossRef CAS PubMed.
- J. J. Hu, J. S. Yoo, M. Lin, E. J. Wang and C. S. Yang, Protective effects of diallyl sulfide on acetaminophen-induced toxicities, Food Chem. Toxicol., 1996, 34, 963–969 CrossRef CAS PubMed.
- J. F. Brady, M. H. Wang, J. Y. Hong, F. Xiao, Y. Li, J. S. Yoo, S. M. Ning, M. J. Lee, J. M. Fukuto and J. M. Gapac,
et al., Modulation of rat hepatic microsomal monooxygenase enzymes and cytotoxicity by diallyl sulfide, Toxicol. Appl. Pharmacol., 1991, 108, 342–354 CrossRef CAS PubMed.
- S. Kalayarasan, N. Sriram and G. Sudhandiran, Diallyl sulfide attenuates bleomycin-induced pulmonary fibrosis: critical role of iNOS, NF-kappaB, TNF-alpha and IL-1beta, Life Sci., 2008, 82, 1142–1153 CrossRef CAS PubMed.
- A. Hall, A. Troupin, B. Londono-Renteria and T. M. Colpitts, Garlic Organosulfur Compounds Reduce Inflammation and Oxidative Stress during Dengue Virus Infection, Viruses, 2017, 9, 159 CrossRef PubMed.
- M. M. Abdel-Daim, H. M. Shaheen, A. I. Abushouk, E. A. Toraih, M. S. Fawzy, W. S. Alansari, L. Aleya and S. Bungau, Thymoquinone and diallyl sulfide protect against fipronil-induced oxidative injury in rats, Environ. Sci. Pollut. Res. Int., 2018, 25, 23909–23916 CrossRef CAS PubMed.
- M. M. Abdel-Daim and R. H. Abdou, Protective Effects of Diallyl Sulfide and Curcumin Separately against Thallium-Induced Toxicity in Rats, Cell J., 2015, 17, 379–388 Search PubMed.
- S. Kalayarasan, P. N. Prabhu, N. Sriram, R. Manikandan, M. Arumugam and G. Sudhandiran, Diallyl sulfide enhances antioxidants and inhibits inflammation through the activation of Nrf2 against gentamicin-induced nephrotoxicity in Wistar rats, Eur. J. Pharmacol., 2009, 606, 162–171 CrossRef CAS PubMed.
- A. K. Kopec, N. Joshi, H. Cline-Fedewa, A. V. Wojcicki, J. L. Ray, B. P. Sullivan, J. E. Froehlich, B. F. Johnson, M. J. Flick and J. P. Luyendyk, Fibrin(ogen) drives repair after acetaminophen-induced liver injury via leukocyte alphaMbeta2 integrin-dependent upregulation of Mmp12, J. Hepatol., 2017, 66, 787–797 CrossRef CAS PubMed.
- S. J. Kim, K. M. Kim, J. H. Yang, S. S. Cho, J. Y. Kim, S. J. Park, S. K. Lee, S. K. Ku, I. J. Cho and S. H. Ki, Sestrin2 protects against Acetaminophen-induced liver injury, Chem.-Biol. Interact., 2017, 269, 50–58 CrossRef CAS PubMed.
- Y. H. Wang, X. J. Xu and H. L. Li, Hepatoprotective effects of Mimic of Manganese superoxide dismutase against carbon tetrachloride-induced hepatic injury, Int. Immunopharmacol., 2014, 22, 126–132 CrossRef CAS PubMed.
- Y. Lu, D. Hu, S. Ma, X. Zhao, S. Wang, G. Wei, X. Wang, A. Wen and J. Wang, Protective effect of wedelolactone against CCl4-induced acute liver injury in mice, Int. Immunopharmacol., 2016, 34, 44–52 CrossRef CAS PubMed.
- T. Zeng, C. L. Zhang, N. Zhao, M. J. Guan, M. Xiao, R. Yang, X. L. Zhao, L. H. Yu, Z. P. Zhu and K. Q. Xie, Impairment of Akt activity by CYP2E1 mediated oxidative stress is involved in chronic ethanol-induced fatty liver, Redox Biol., 2018, 14, 295–304 CrossRef CAS PubMed.
- S. Wang, M. Li, X. Wang, X. Li, H. Yin, L. Jiang, W. Han, G. Irving, T. Zeng and K. Xie, Diallyl trisulfide attenuated n-hexane induced neurotoxicity in rats by modulating P450 enzymes, Chem.-Biol. Interact., 2017, 265, 1–7 CrossRef CAS PubMed.
- M. Abdel-Daim, A. I. Abushouk, R. Reggi, N. S. Yarla, M. Palmery and I. Peluso, Association of antioxidant nutraceuticals and acetaminophen (paracetamol): Friend or foe?, J. Food Drug Anal., 2018, 26, S78–S87 CrossRef CAS PubMed.
- B. L. Woolbright and H. Jaeschke, Role of the inflammasome in acetaminophen-induced liver injury and acute liver failure, J. Hepatol., 2017, 66, 836–848 CrossRef CAS PubMed.
- R. O. Recknagel, E. A. Glende Jr., J. A. Dolak and R. L. Waller, Mechanisms of carbon tetrachloride toxicity, Pharmacol. Ther., 1989, 43, 139–154 CrossRef CAS PubMed.
- N. M. Fahmy, E. Al-Sayed, M. M. Abdel-Daim, M. Karonen and A. N. Singab, Protective effect of Terminalia muelleri against carbon tetrachloride-induced hepato and nephro-toxicity in mice and characterization of its bioactive constituents, Pharm. Biol., 2016, 54, 303–313 CrossRef CAS PubMed.
- E. Al-Sayed, M. M. Abdel-Daim, O. E. Kilany, M. Karonen and J. Sinkkonen, Protective role of polyphenols from Bauhinia hookeri against carbon tetrachloride-induced hepato- and nephrotoxicity in mice, Renal Failure, 2015, 37, 1198–1207 CrossRef CAS PubMed.
- H. A. Khan, M. Z. Ahmad, J. A. Khan and M. I. Arshad, Crosstalk of liver immune cells and cell death mechanisms in different murine models of liver injury and its clinical relevance, Hepatobiliary Pancreatic Dis. Int., 2017, 16, 245–256 CrossRef.
- M. G. Sturgill and G. H. Lambert, Xenobiotic-induced hepatotoxicity: mechanisms of liver injury and methods of monitoring hepatic function, Clin. Chem., 1997, 43, 1512–1526 CAS.
- W. P. Jiang, S. S. Huang, Y. Matsuda, H. Saito, N. Uramaru, H. Y. Ho, J. B. Wu and G. J. Huang, Protective Effects of Tormentic Acid, a Major Component of Suspension Cultures of Eriobotrya japonica Cells, on Acetaminophen-Induced Hepatotoxicity in Mice, Molecules, 2017, 22, 830 CrossRef PubMed.
- W. Zhang, X. Zhang, K. Zou, J. Xie, S. Zhao, J. Liu, H. Liu, J. Wang and Y. Wang, Seabuckthorn berry polysaccharide protects against carbon tetrachloride-induced hepatotoxicity in mice via anti-oxidative and anti-inflammatory activities, Food Funct., 2017, 8, 3130–3138 RSC.
- C. Pang, Z. Zheng, L. Shi, Y. Sheng, H. Wei, Z. Wang and L. Ji, Caffeic acid prevents acetaminophen-induced liver injury by activating the Keap1-Nrf2 antioxidative defense system, Free Radical Biol. Med., 2016, 91, 236–246 CrossRef CAS PubMed.
- J. W. Ko, S. H. Park, N. R. Shin, J. Y. Shin, J. W. Kim, I. S. Shin, C. Moon, J. D. Heo, J. C. Kim and I. C. Lee, Protective effect and mechanism of action of diallyl disulfide against acetaminophen-induced acute hepatotoxicity, Food Chem. Toxicol., 2017, 109, 28–37 CrossRef CAS PubMed.
- T. Zeng, C. L. Zhang, Z. P. Zhu, L. H. Yu, X. L. Zhao and K. Q. Xie, Diallyl trisulfide (DATS) effectively attenuated oxidative stress-mediated liver injury and hepatic mitochondrial dysfunction in acute ethanol-exposed mice, Toxicology, 2008, 252, 86–91 CrossRef CAS PubMed.
- Y. Lv, B. Zhang, G. Xing, F. Wang and Z. Hu, Protective effect of naringenin against acetaminophen-induced acute liver injury in metallothionein (MT)-null mice, Food Funct., 2013, 4, 297–302 RSC.
- W. Xie, M. Wang, C. Chen, X. Zhang and M. F. Melzig, Hepatoprotective effect of isoquercitrin against acetaminophen-induced liver injury, Life Sci., 2016, 152, 180–189 CrossRef CAS PubMed.
- B. Zeng, M. Su, Q. Chen, Q. Chang, W. Wang and H. Li, Protective effect of a polysaccharide from Anoectochilus roxburghii against carbon tetrachloride-induced acute liver injury in mice, J. Evidence-Based Complementary Altern. Med., 2017, 200, 124–135 CAS.
- K. Kiso, S. Ueno, M. Fukuda, I. Ichi, K. Kobayashi, T. Sakai, K. Fukui and S. Kojo, The role of Kupffer cells in carbon tetrachloride intoxication in mice, Biol. Pharm. Bull., 2012, 35, 980–983 CrossRef CAS.
- J. Liu, Q. Y. Zhang, L. M. Yu, B. Liu, M. Y. Li and R. Z. Zhu, Phycocyanobilin accelerates liver regeneration and reduces mortality rate in carbon tetrachloride-induced liver injury mice, World J. Gastroenterol., 2015, 21, 5465–5472 CrossRef CAS PubMed.
- R. Feng, Y. Wang, C. Liu, C. Yan, H. Zhang, H. Su, J. X. Kang, C. Z. Shang and J. B. Wan, Acetaminophen-induced liver injury is attenuated in transgenic fat-1 mice endogenously synthesizing long-chain n-3 fatty acids, Biochem. Pharmacol., 2018, 154, 75–88 CrossRef CAS PubMed.
- J. Liu, Y. Fu, H. Zhang, J. Wang, J. Zhu, Y. Wang, Y. Guo, G. Wang, T. Xu, M. Chu and F. Wang, The hepatoprotective effect of the probiotic Clostridium butyricum against carbon tetrachloride-induced acute liver damage in mice, Food Funct., 2017, 8, 4042–4052 RSC.
- F. Van Herreweghe, N. Festjens, W. Declercq and P. Vandenabeele, Tumor necrosis factor-mediated cell death: to break or to burst, that's the question, Cell. Mol. Life Sci., 2010, 67, 1567–1579 CrossRef PubMed.
- K. Wang, Autophagy and apoptosis in liver injury, Cell Cycle, 2015, 14, 1631–1642 CrossRef CAS PubMed.
- W. B. Chen, S. S. Lai, D. C. Yu, J. Liu, S. Jiang, D. D. Zhao, Y. T. Ding, C. J. Li and B. Xue, GGPPS deficiency aggravates CCl4-induced liver injury by inducing hepatocyte apoptosis, FEBS Lett., 2015, 589, 1119–1126 CrossRef CAS PubMed.
- H. Yu, L. Zheng, L. Yin, L. Xu, Y. Qi, X. Han, Y. Xu, K. Liu and J. Peng, Protective effects of the total saponins from Dioscorea nipponica Makino against carbon tetrachloride-induced liver injury in mice through suppression of apoptosis and inflammation, Int. Immunopharmacol., 2014, 19, 233–244 CrossRef CAS PubMed.
- E. Song, J. Fu, X. Xia, C. Su and Y. Song, Bazhen decoction protects against acetaminophen induced acute liver injury by inhibiting oxidative stress, inflammation and apoptosis in mice, PLoS One, 2014, 9, e107405 CrossRef PubMed.
- T. Yan, H. Wang, M. Zhao, T. Yagai, Y. Chai, K. W. Krausz, C. Xie, X. Cheng, J. Zhang, Y. Che, F. Li, Y. Wu, C. N. Brocker, F. J. Gonzalez, G. Wang and H. Hao, Glycyrrhizin Protects against Acetaminophen-Induced Acute Liver Injury via Alleviating Tumor Necrosis Factor alpha-Mediated Apoptosis, Drug Metab. Dispos., 2016, 44, 720–731 CrossRef CAS PubMed.
- H. Wu, Y. Qiu, Z. Shu, X. Zhang, R. Li, S. Liu, L. Chen, H. Liu and N. Chen, Protective effect of Trillium tschonoskii saponin on CCl4-induced acute liver injury of rats through apoptosis inhibition, Can. J. Physiol. Pharmacol., 2016, 94, 1291–1297 CrossRef CAS PubMed.
|
This journal is © The Royal Society of Chemistry 2019 |