DOI:
10.1039/C8SC04601H
(Edge Article)
Chem. Sci., 2019,
10, 2501-2506
A tandem dearomatization/rearomatization strategy: enantioselective N-heterocyclic carbene-catalyzed α-arylation†
Received
16th October 2018
, Accepted 21st December 2018
First published on 27th December 2018
Abstract
In this study, the first example of the carbene-catalyzed tandem dearomatization/rearomatization reaction of azonaphthalenes with α-chloroaldehydes is described. This protocol enables the efficient assembly of chiral dihydrocinnolinone derivatives in good yields with excellent enantioselectivities (up to 99% ee). Moreover, this strategy enables not only the highly enantioselective NHC-catalyzed nucleophilic aromatic substitution, but also a formal Csp2–Csp3 bond formation.
Advances in reaction development for the stereoselective construction of medicinally important scaffolds or high value-added chemicals depend on utilizing innovative and long-standing synthesis strategies.1 Over the past decade, a large number of organic transformations driven by N-heterocyclic carbene (NHC) catalysis have been rapidly developed that enabled certain types of reaction manifolds and asymmetric versions of important compounds.2 Since the seminar report of the Breslow intermediate in 1958, there has been an ever-increasing demand in recruiting novel NHC-bound intermediates for their applications in organic synthesis.3 Among these achievements, the exploration of NHC-bound enolates for new bond formations has attracted considerable attention from the synthetic community due to its high chemical reactivity and remarkable stereo-control. As shown in Fig. 1a, several readily available starting materials (e.g., enals, aldehydes, ketenes, carboxylic acids, esters) have proven to be effective reactants for the in situ generation of azolium enolates, thus resulting in a large number of distinct reactions and diversified core skeletons. In 2006, Bode et al. first reported an elegant example of the protonation of electron-deficient enals to produce azolium enolates and the subsequent trapping by N-sulfonyl, α,β-unsaturated imines to yield dihydropyridinones.4 Rovis et al. have pioneered in using α-chloroaldehydes as reactants to prepare azolium enolates for further protonation5a or internal redox reaction.5b Meanwhile, Smith et al. also confirmed that α-aroyloxyaldehydes as precursors could convert to azolium enolates in NHC-catalyzed redox esterification, amination, and cycloaddition reactions.6 Moreover, the Smith et al.7a and Ye et al.7b,c have documented that azolium enolates could be readily prepared from ketenes for further annulation. Rovis et al., Chi et al., Scheidt et al., and Ye et al. have utilized aliphatic aldehydes,8a activated esters,8b carboxylic acids8c,d and anhydrides8e as suitable reactants to successfully achieve azolium enolates, respectively. In brief, the synthetic utilities of azolium enolates have widely extended to various [2 + 2],7a–c,9 [2 + 2 + 2],9f,10 [3 + 2]11 and [4 + 2]4,6,7c,8a–c,12 cycloadditions. These broad diversities may be attributed to the special property of absence of polarity reversal of azolium enolates. Nonetheless, NHC-catalyzed α-arylation still remains a formidable challenge for synthetic chemists.
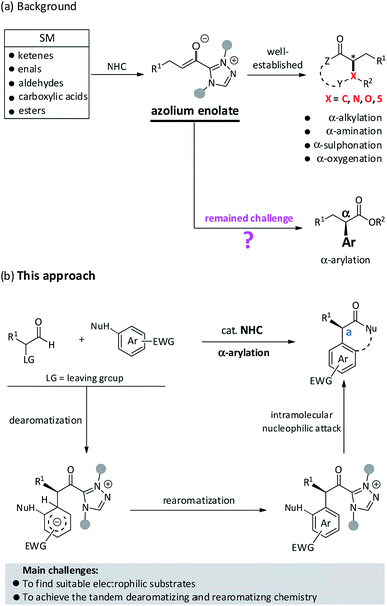 |
| Fig. 1 Representative transformations of azolium enolates catalyzed by NHCs. | |
Given the success of in situ generated azolium enolates, we anticipated that the desirable NHC-catalyzed α-arylation reaction might partially be attributed to the recruiting of suitable substrates. In 2015, Glorius et al. found that the NHC-bound enolates could react with aromatic azomethine imines to generate pyrazolo[5,1-a]isoquinolines via a direct dearomatization process.13 Inspired by this prominent result, we speculated that a matching electrophile (e.g., electron-deficient aromatic compound) may facilitate the initial dearomatization step via a nucleophilic attack and C–C bond formation, followed by a rearomatization process, if successfully, to offer the desired α-arylated molecules (Fig. 1b). We also envisioned that the thermodynamics of this intramolecular cycloaddition process probably helps to drive the nucleophilic dearomatization step.
In the process of recruiting suitable substrates, we particularly focused on the azobenzene scaffolds because it has the basic required characteristics of being the matching substrate. First, the azobenzene-type structures can be used as electrophiles in the critical addition step. Second, the azo group can assist in accomplishing the tandem dearomatization–rearomatization process via intermolecular tautomerization. In fact, azobenzene derivatives as synthons have been broadly utilized in metal-catalyzed transformations, as illustrated by rhodium-catalyzed [3 + 2] cyclization of electron-deficient alkenes with azobenzenes14 (Fig. 2a). Until recently, the Tan et al.15 reported an elegant example of chiral phosphoric acid-catalyzed [3 + 2] cyclization of azonaphthalenes with 2,3-disubstituted indoles (Fig. 2b). In these successful examples, the azo motif within the azonaphthalenes not only works as an electron-withdrawing group to activate the aromatic ring, but also plays a critical role in triggering the rearomatization process. Meanwhile, the NHC-bounded homoenolates, azolium enolates or acyl anions have proven to be effective nucleophiles in catalytic nucleophilic dearomatization.13,16 Inspired by these achievements,15,17 we then set out to explore the feasibility of NHC-catalyzed nucleophilic dearomatization of azonaphthalenes with α-chloroaldehyde. Unambiguously, the following challenges need to be overcome in this design: (1) controlling the regioselectivity as the nucleophilic addition of azobenzene derivatives can probably occur at either the N
N double bond7c,12h,i,18 or the aromatic ring. (2) Check the compatibility of reactive azonaphthalenes with the nucleophilic NHC-bounded enolate intermediates in the dearomatization reaction. (3) Identify the efficient catalytic system for achieving high enantioselectivity. Herein, we report an unprecedented example of NHC-catalyzed α-arylation of azonaphthalenes with α-chloroaldehydes. It should be mentioned that this process contains a formal Csp2–Csp3 bond formation and starts from an organocatalytic nucleophilic aromatic substitution (Fig. 2c).
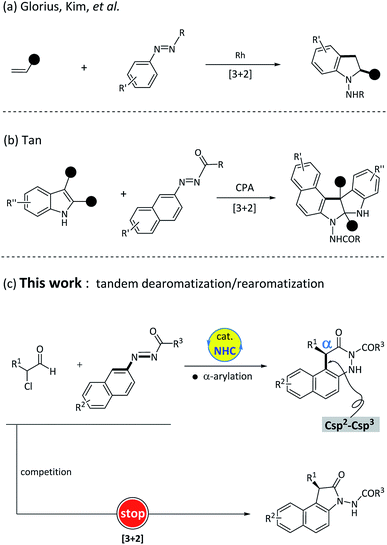 |
| Fig. 2 Anticipated cyclization reactions of azobenzene molecules. | |
To validate the feasibility of the hypothesis, our initial reaction development was conducted with α-chloroaldehyde (1a) and azonaphthalene (2a) in the presence of 20 mol% NHC precatalysts and DIPEA in THF at room temperature (Table 1). Only a trace amount or a moderate yield of 3a was achieved using NHC precatalyst A or B (entries 1 and 2). Gratifyingly, the N-Ph-substituted chiral triazolium catalyst (C2) afforded 3a in 73% yield with 90% ee (Table 1, entry 4). Further investigation indicated that the N-2,4,6-(Me)3C6H2- or N-2,6-(Et)2C6H3-substituted chiral triazolium catalyst C1 or C3 gave excellent enantioselectivities, but tolerated moderate yields (entries 3 and 5). Further fine-tuning of other parameters (e.g., base, solvent, and catalyst loading, see ESI† for more details) revealed that the optimal condition is a combination of room temperature, 10 mol% C1, 200 mol% DIPEA, and 1.0 mL of tBuOMe (Table 1, entry 19).
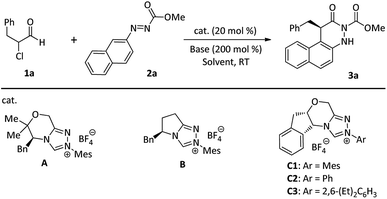
|
Entry |
Cat. |
Solvent |
Base |
Yieldb (%) |
eec (%) |
Reaction conditions: α-chloroaldehyde 1a (0.20 mmol, 2.0 equiv.), azonaphthalene 2a (0.10 mmol, 1.0 equiv.), cat. (0.02 mmol), base (0.20 mmol), solvent (1.0 mL), 10 h, room temperature.
Yields of isolated products after column chromatography.
The ee values were determined by HPLC using a chiral stationary phase.
C1 (10 mol%), 16 h.
C1 (5 mol%), 24 h.
|
1 |
A
|
THF |
DIPEA |
8 |
— |
2 |
B
|
THF |
DIPEA |
58 |
88 |
3 |
C1
|
THF |
DIPEA |
76 |
99 |
4 |
C2
|
THF |
DIPEA |
73 |
90 |
5 |
C3
|
THF |
DIPEA |
71 |
99 |
6 |
C1
|
THF |
DBU |
Trace |
— |
7 |
C1
|
THF |
Cs2CO3 |
22 |
24 |
8 |
C1
|
DCM |
DIPEA |
77 |
97 |
9 |
C1
|
t
BuOMe |
DIPEA |
90 |
99 |
10 |
C1
|
CH3CN |
DIPEA |
71 |
82 |
11d |
C1
|
t
BuOMe |
DIPEA |
89 |
99 |
12e |
C1
|
t
BuOMe |
DIPEA |
61 |
99 |
After establishing the optimal reaction conditions, we examined the substrate scope with respect to various α-chloroaldehydes 1 (Fig. 3). The reaction was applicable to α-chloroaldehyde derivatives with either aromatic rings or alkyl chains. The electronic natures or substituted patterns on the aromatic ring appeared to have limited effects on reaction results, affording the corresponding dihydrocinnolines in yields of 73–88% and ee values between 94 and 99% (3a–3i). When the aldehydes containing alkyl R-substituents located at the α-carbon were used, the corresponding dihydrocinnoline derivatives were also afforded with good yields and excellent enantio-controls (3j–3l). Notably, when α-chloroaldehydes bore functional alkyl subunits (e.g., alkene, chlorine, ether) at the side chain, good yields with excellent enantioselectivities were also regularly observed (3m–3r). Pleasingly, 2-chloro-2-phenylacetaldehyde also delivered the corresponding product (3s) in a moderate yield, albeit with a relatively low ee value.
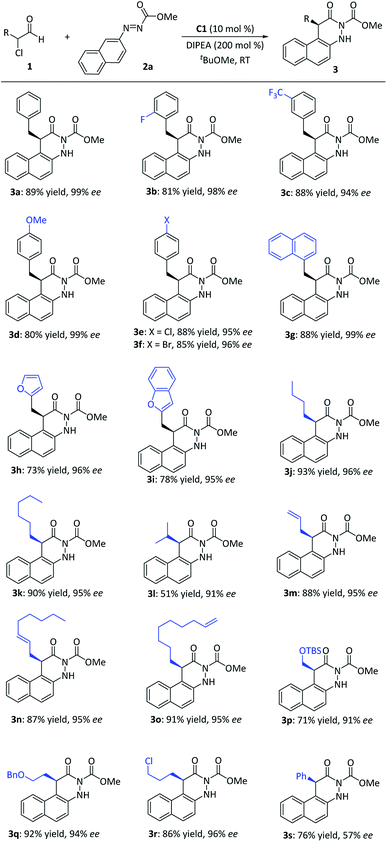 |
| Fig. 3 Scope of α-chloroaldehydes. aReaction conditions: 1 (0.20 mmol), 2a (0.10 mmol), cat. C1 (10 mol%), DIPEA (0.20 mmol), tBuOMe (1.0 mL), 12–16 h, room temperature. | |
Further investigation on the synthetic feasibility of azonaphthalenes (2) was performed with α-chloroaldehyde (1a), as shown in Fig. 4. The results indicated that this reaction tolerated a diverse array of azonaphthalenes, and afforded the dihydrocinnolines (4) in good to high yields (76–93%) and with high levels of enantio-controls (93–99% ee). When R′ substituents were alkyl oxide groups (4a–4d), amino group (4e) or sulfonyl group (4f), the products were obtained in high yields with excellent enantioselectivities. Specifically, the electronic nature or the substitution pattern of the aromatic rings showed limited effects on the reactivity (4g–4l). When an anthracene moiety replaced the naphthalene ring in azonaphthalenes, 76% yield and 95% ee were still achieved (4m). The absolute configuration of 3r was determined by single-crystal X-ray diffraction analysis,19 and those of other products were assigned by analogy.
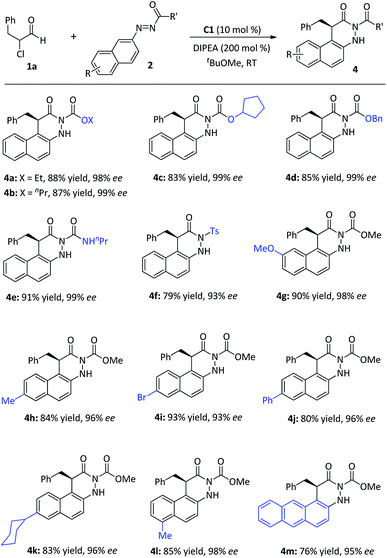 |
| Fig. 4 Scope of azonaphthalenes. aReaction conditions: 1a (0.20 mmol), 2 (0.10 mmol), cat. C1 (10 mol%), DIPEA (0.20 mmol), tBuOMe (1.0 mL), 16 h, room temperature. | |
Furthermore, to demonstrate the practicality of this catalytic transformation, a gram-scale synthesis of 3a was conducted under the optimal condition. There was almost no change in the reaction yield and enantioselectivity (Fig. 5, eqn (1), 90%, 97% ee), implying that the catalytic tandem reaction of azonaphthalenes with α-chloroaldehydes can be scaled up. Moreover, the mediated cleavage of 3a mediated by RANEY®-Ni led to the formation of α-substituted chiral amide (5) in 65% yield with the same ee value (Fig. 5, eqn (2)).
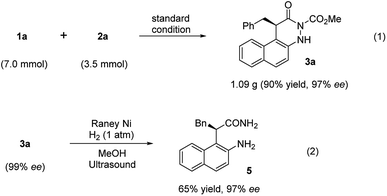 |
| Fig. 5 Scale-up synthesis and further transformation. | |
As illustrated in Scheme 1, a postulated mechanism is described. Initial addition of catalyst C1 to α-chloroaldehyde 1 followed by 1,2-H migration generates NHC-bounded enolate I. Nucleophilic addition of enolate I to azonaphthalene (2) results in a formal tandem reaction of dearomatization13,16 and rearomatization along with C–H cleavage and N–H formation to give the thermodynamically stable intermediate III. Finally, intramolecular N-acylation of III leads to the final product 3 or 4 and the NHC catalyst C1 is released for the next catalytic cycle. Surely, a concerted [4 + 2] annulation mechanism cannot be completely ruled out in this case.
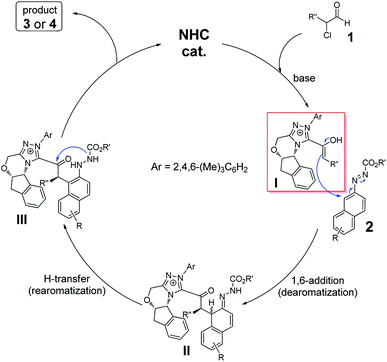 |
| Scheme 1 Proposed mechanism. | |
In summary, a novel NHC-catalyzed tandem dearomatization/rearomatization reaction of azonaphthalenes with α-chloroaldehydes has been developed.20 This protocol allows for the rapid assembly of the dihydrocinnolinone scaffolds in good to high yields with high to excellent enantioselectivities. Further investigations on the construction of other relevant frameworks as well as a detailed mechanistic study are currently underway in our laboratory.
Conflicts of interest
There are no conflicts to declare.
Acknowledgements
Generous financial support for this study was provided by the National Natural Science Foundation of China (No. 21672121, 21871160), the “Thousand Plan” Youth Program of China, the Tsinghua University, the Bayer Investigator fellow, the Fellowship of Tsinghua-Peking Centre for Life Sciences (CLS).
Notes and references
-
(a)
E. N. Jacobsen, A. Pfaltz and H. Yamamoto, Comprehensive asymmetric catalysis, Springer, New York, 1999 CrossRef
;
(b)
G. Shang, W. Li, X. Zhang and I. Ojima, Catalytic asymmetric synthesis, John Wiley & Sons, New York, 2010 Search PubMed
.
- For selected recent reviews on NHC catalysis:
(a) D. Enders, O. Niemeier and A. Henseler, Chem. Rev., 2007, 107, 5606–5655 CrossRef CAS PubMed
;
(b) A. T. Biju, N. Kuhl and F. Glorius, Acc. Chem. Res., 2011, 44, 1182–1195 CrossRef CAS PubMed
;
(c) D. T. Cohen and K. A. Scheidt, Chem. Sci., 2012, 3, 53–57 RSC
;
(d) X. Bugaut and F. Glorius, Chem. Soc. Rev., 2012, 41, 3511–3522 RSC
;
(e) A. Grossmann and D. Enders, Angew. Chem., Int. Ed., 2012, 51, 314–325 CrossRef CAS PubMed
;
(f) S. J. Ryan, L. Candish and D. W. Lupton, Chem. Soc. Rev., 2013, 42, 4906–4917 RSC
;
(g) S. J. Connon, Angew. Chem., Int. Ed., 2014, 53, 1203–1205 CrossRef CAS PubMed
;
(h) M. N. Hopkinson, C. Richter, M. Schedler and F. Glorius, Nature, 2014, 510, 485–496 CrossRef CAS PubMed
;
(i) J. Mahatthananchai and J. W. Bode, Acc. Chem. Res., 2014, 47, 696–707 CrossRef CAS PubMed
;
(j) R. S. Menon, A. T. Biju and V. Nair, Chem. Soc. Rev., 2015, 44, 5040–5052 RSC
;
(k) D. M. Flanigan, F. Romanov-Michailidis, N. A. White and T. Rovis, Chem. Rev., 2015, 15, 9307–9387 CrossRef PubMed
;
(l) R. S. Menon, A. T. Biju and V. Nair, Beilstein J. Org. Chem., 2016, 12, 444–461 CrossRef CAS PubMed
;
(m) M. H. Wang and K. A. Scheidt, Angew. Chem., Int. Ed., 2016, 55, 14912–14922 CrossRef CAS PubMed
;
(n) C. Zhang, J. F. Hooper and D. W. Lupton, ACS Catal., 2017, 7, 2583–2596 CrossRef CAS
.
-
(a) R. Breslow, J. Am. Chem. Soc., 1958, 80, 3719–3726 CrossRef CAS
; experimental evidence for Breslow intermediate, see:
(b) V. Nair, S. Bindu, V. Sreekumar and N. P. Rath, Org. Lett., 2003, 5, 665 CrossRef CAS PubMed
; for isolation and characterization of Breslow intermediate, see:
(c) A. Berkessel, V. R. Yatham, S. Elfert and J.-M. Neudöfl, Angew. Chem., Int. Ed., 2013, 52, 11158 CrossRef CAS PubMed
.
- M. He, J. R. Struble and J. W. Bode, Highly enantioselective azadiene Diels–Alder reactions catalyzed by chiral N-heterocyclic carbenes, J. Am. Chem. Soc., 2006, 128, 8418–8420 CrossRef CAS PubMed
.
-
(a) N. T. Reynolds, J. Read de Alaniz and T. Rovis, J. Am. Chem. Soc., 2004, 126, 9518–9519 CrossRef CAS PubMed
;
(b) N. T. Reynolds and T. Rovis, J. Am. Chem. Soc., 2005, 127, 16406–16407 CrossRef CAS PubMed
.
-
(a) A. Chan and D. Smith, Chem. Commun., 2011, 47, 373–375 RSC
; for reviews on α-functionalized aldehydes, see:
(b)
P.-C. Chiang and J. W. Bode, N-Heterocyclic carbine catalysed reactions of α-functionalized aldehydes, ed. B. List and K. Maruoka, Science of Synthesis, Asymmetric Organocatalysis, Thieme, Germany, 2012 Search PubMed
.
-
(a) N. Duguet, C. D. Campbell, A. M. Z. Slavin and A. D. Smith, Org. Biomol. Chem., 2008, 6, 1108–1113 RSC
;
(b) Y. R. Zhang, L. He, X. Wu, P. L. Shao and S. Ye, Org. Lett., 2008, 10, 277–280 CrossRef CAS PubMed
;
(c) X.-L. Huang, L. He, P.-L. Shao and S. Ye, Angew. Chem., Int. Ed., 2009, 48, 192–195 CrossRef CAS PubMed
.
- For pioneering work on aliphatic aldehydes, see:
(a) X. Zhao, K. E. Ruhl and T. Rovis, Angew. Chem., Int. Ed., 2012, 51, 12330–12333 CrossRef CAS PubMed
; for pioneering work on activated esters, see:
(b) L. Hao, Y. Du, H. Lv, X. Chen, H. Jiang, Y. Shao and Y. R. Chi, Org. Lett., 2012, 14, 2154–2157 CrossRef CAS PubMed
; for pioneering work on carboxylic acids, see:
(c) A. Lee, A. Younai, C. K. Price, J. Izquierdo, R. K. Mishra and K. A. Scheidt, J. Am. Chem. Soc., 2014, 136, 10589–10592 CrossRef CAS PubMed
;
(d) X.-Y. Chen, Z.-H. Gao, C.-Y. Song, C.-L. Zhang, Z.-X. Wang and S. Ye, Angew. Chem., Int. Ed., 2014, 53, 11611–11615 CrossRef CAS PubMed
; for pioneering work on anhydrides, see:
(e) Z. Jin, S. Chen, Y. Wang, P. Zheng, S. Yang and Y. R. Chi, Angew. Chem., Int. Ed., 2014, 53, 13506–13509 CrossRef CAS PubMed
.
- For [2 + 2] cycloadditions with azolium enolates, see:
(a) L. He, H. Lv, Y.-R. Zhang and S. Ye, J. Org. Chem., 2008, 73, 8101–8103 CrossRef CAS PubMed
;
(b) X.-L. Huang, X.-Y. Chen and S. Ye, J. Org. Chem., 2009, 74, 7585–7587 CrossRef CAS PubMed
;
(c) X.-N. Wang, P.-L. Shao, H. Lv and S. Ye, Org. Lett., 2009, 11, 4029–4031 CrossRef CAS PubMed
;
(d) X.-N. Wang, Y.-Y. Zhang and S. Ye, Adv. Synth. Catal., 2010, 352, 1892–1895 CrossRef CAS
;
(e) T. Wang, X.-L. Huang and S. Ye, Org. Biomol. Chem., 2010, 8, 5007–5011 RSC
;
(f) X.-N. Wang, L.-T. Shen and S. Ye, Org. Lett., 2011, 13, 6382–6385 CrossRef CAS PubMed
;
(g) T.-Y. Jian, L. He, C. Tang and S. Ye, Angew. Chem., Int. Ed., 2011, 50, 9104–9107 CrossRef CAS PubMed
.
- X.-N. Wang, L.-T. Shen and S. Ye, Chem. Commun., 2011, 47, 8388–8390 RSC
.
- For [3 + 2] cycloadditions with azolium enolates, see:
(a) P.-L. Shao, X.-Y. Chen and S. Ye, Angew. Chem., Int. Ed., 2010, 49, 8412–8416 CrossRef CAS PubMed
;
(b) L. Li, D. Du, J. Ren and Z. Wang, Eur. J. Org. Chem., 2011, 614–618 CrossRef CAS
;
(c) Q. Ni, H. Zhang, A. Grossmann, C. C. J. Loh, C. Merkens and D. Enders, Angew. Chem., Int. Ed., 2013, 52, 13562–13566 CrossRef CAS PubMed
;
(d) Z.-F. Zhang, C.-L. Zhang, Z.-Y. Song, Z.-H. Gao and S. Ye, Chem.–Eur. J., 2018, 24, 8302–8305 CrossRef CAS PubMed
.
- For selective recent work on [4 + 2] cycloadditions with azolium enolates, see:
(a) M. He, G. J. Uc and J. W. Bode, J. Am. Chem. Soc., 2006, 128, 15088–15089 CrossRef CAS PubMed
;
(b) T.-Y. Jian, P.-L. Shao and S. Ye, Chem. Commun., 2011, 47, 2381–2383 RSC
;
(c) J. Kaeobamrung, M. C. Kozlowski and J. W. Bode, Proc. Natl. Acad. Sci. U. S. A., 2010, 107, 20661–20665 CrossRef CAS PubMed
;
(d) X. Fang, X. Chen and Y. R. Chi, Org. Lett., 2011, 13, 4708–4711 CrossRef CAS PubMed
;
(e) Z. Wu, X. Wang, F. Li and J. Wang, Org. Lett., 2015, 17, 3588–3591 CrossRef CAS PubMed
;
(f) T.-Y. Jian, L.-H. Sun and S. Ye, Chem. Commun., 2012, 48, 10907–10909 RSC
;
(g) L. Yang, F. Wang, P. J. Chua, Y. Lv, L.-J. Zhong and G. Zhong, Org. Lett., 2012, 14, 2894–2897 CrossRef CAS PubMed
;
(h) J. E. Taylor, D. S. B. Daniels and A. D. Smith, Org. Lett., 2013, 15, 6058–6061 CrossRef CAS PubMed
;
(i) L. Yang, F. Wang, R. Lee, Y. Lv, K.-W. Huang and G. Zhong, Org. Lett., 2014, 16, 3872–3875 CrossRef CAS PubMed
;
(j) A. Lee and K. A. Scheidt, Chem. Commun., 2015, 51, 3407–3410 RSC
;
(k) X. Chen, R. Song, Y. Liu, C. Y. Ooi, Z. Jin, T. Zhu, H. Wang, L. Hao and Y. R. Chi, Org. Lett., 2018, 19, 5892–5895 CrossRef PubMed
;
(l) S. Lu, J.-Y. Ong, S. B. Poh, T. Tsang and Y. Zhao, Angew. Chem., Int. Ed., 2018, 57, 5714–5719 CrossRef CAS PubMed
.
- C. Guo, M. Fleige, D. Janssen-Müller, C. G. Daniliuc and F. Glorius, Nat. Chem., 2015, 7, 842–847 CrossRef CAS PubMed
.
-
(a) U. R. Aulwurm, J. U. Melchinger and H. Kisch, Organometallics, 1995, 14, 3385–3395 CrossRef CAS
;
(b) D. Zhao, S. Vasquez-Cespedes and F. Glorius, Angew. Chem., Int. Ed., 2015, 54, 1657–1661 CrossRef CAS PubMed
;
(c) S. H. Han, N. K. Mishra, H. Jo, Y. Oh, M. Jeon, S. Kim, W. J. Kim, J. S. Lee, H. S. Kim and I. S. Kim, Adv. Synth. Catal., 2017, 359, 2396–2401 CrossRef CAS
.
- L.-W. Qi, J.-H. Mao, J. Zhang and B. Tan, Nat. Chem., 2018, 10, 58–64 CrossRef CAS PubMed
.
- For NHC-catalyzed dearomatization, see
(a) V. Nair, M. Poonoth, S. Vellalath, E. Suresh and R. Thirumalai, J. Org. Chem., 2006, 71, 8964–8965 CrossRef CAS PubMed
;
(b) D. Janssen-Müller, M. Fleige, D. Schlüns, M. Wollenburg, C. G. Daniliuc, J. Neugebauer and F. Glorius, ACS Catal., 2016, 6, 5735–5739 CrossRef
;
(c) J.-H. Xu, S.-C. Zheng, J.-W. Zhang, X.-Y. Liu and B. Tan, Angew. Chem., Int. Ed., 2016, 55, 11834–11839 CrossRef CAS PubMed
;
(d) D. M. Flanigan and T. Rovis, Chem. Sci., 2017, 8, 6566–6569 RSC
;
(e) S. Bera, C. G. Daniliuc and A. Studer, Angew. Chem., Int. Ed., 2017, 56, 7402–7406 CrossRef CAS PubMed
;
(f) G. D. Carmine, D. Ragno, O. Bortolini, P. P. Giovannini, A. Mazzanti, A. Massi and M. Fogagnolo, J. Org. Chem., 2018, 83, 2050–2057 CrossRef PubMed
.
-
(a) C. Ma, J.-Y. Zhou, Y.-Z. Zhang, G.-J. Mei and F. Shi, Angew. Chem., Int. Ed., 2018, 57, 5398–5402 CrossRef CAS PubMed
;
(b) C. Li, D.-N. Xu, C. Ma, G.-J. Mei and F. Shi, J. Org. Chem., 2018, 83, 9190–9200 CrossRef CAS PubMed
.
- A. Chan and K. A. Scheidt, J. Am. Chem. Soc., 2008, 130, 2740–2741 CrossRef CAS PubMed
.
- CCDC 1858695 (3r) contains the supplementary crystallographic data for this paper.†.
-
(a) J. Kaeobamrung, J. Mahatthananchai, P. Zheng and J. W. Bode, J. Am. Chem. Soc., 2010, 132, 8810–8812 CrossRef CAS PubMed
;
(b) J. Kaeobamrung, J. Mahatthananchai and J. W. Bode, ACS Catal., 2012, 2, 494–503 CrossRef PubMed
.
Footnote |
† Electronic supplementary information (ESI) available. CCDC 1858695. For ESI and crystallographic data in CIF or other electronic format see DOI: 10.1039/c8sc04601h |
|
This journal is © The Royal Society of Chemistry 2019 |