DOI:
10.1039/C9RA08323E
(Paper)
RSC Adv., 2019,
9, 38807-38813
Removal of phthalic acid dieters with dissolved organic matter by an anaerobic/anoxic/oxic leachate treatment process
Received
12th October 2019
, Accepted 21st November 2019
First published on 27th November 2019
Abstract
The removal of di-n-butyl phthalate (DBP) and di(2-ethylhexyl) phthalate (DEHP) with dissolved organic matter (DOM) was studied in a laboratory scale anaerobic/anoxic/oxic reactor for landfill leachate treatment. The removal rate was up to 98.0% for DBP and 78.2% for DEHP, which was related to humification of DOM (i.e., the aromaticity and molecular weight (MW) of humic substances in landfill leachate). The dissolved organic carbon (DOC) was mostly humic acid and fulvic acid in the fraction of 1–100 kDa MW, indicating strong aromaticity and a high DBP/DEHP concentration. With complete removal of the fraction, the removal rate of DBP/DEHP was also high. The positive correlation of the DOC and DBP/DEHP concentration in raw leachate and the effluent from each reactor showed that the interaction between DOM and DBP/DEHP facilitated the removal of organic pollutants.
1. Introduction
Landfill leachate is a typical wastewater with a high concentration of organic matter and difficult biodegradable compounds because of its complex composition that poses a great threat to the environment.1,2 Environmental endocrine disrupting compounds (EDCs) are exogenous substances that may lead to endocrine disorders in organisms.3 Phthalic acid diesters (PAEs) are a type of EDCs that are widely used as plasticizers in plastics.4 PAEs have estrogenic effects and can destroy the hormonal balance of human beings, animals and their offspring.5,6 Among PAEs, di-n-butyl phthalate (DBP) and di(2-ethylhexyl) phthalate (DEHP) are the most widely used and are considered to be the main substance that produces estrogen in leachate.7,8
Dissolved organic matter (DOM) in landfill leachate is mainly distributed in humic acid (HA), fulvic acid (FA) and hydrophilic organic components (HyI). Research shows that hydrophobic DOM with a molecular weight of 3–14 kDa and less than 600 Da is the primary source of estrogen production in leachate.9,10 The aromaticity and humification degree of DOM will gradually increase with the age of leachate.11 The molecular weight of refractory organic matter in moderately aged and old leachate is mainly distributed in the components of 10–100 kDa and less than 3.5 kDa. These mainly consist of HA and FA humus, with the HA content being higher than the FA.12 These organic components can improve the apparent solubility of hydrophobic PAEs and play an important role in their removal from leachate.11–13 HA fragments contain many PAEs with concentration distributions that increase exponentially with the molecular weight of DOM fragments, demonstrating there is a strong interaction between DOM and PAEs. This interaction may be related to the hydrogen bonds of phenolic, hydroxyl or amide groups in DOM. Peptide bonds present in HA can explain the strong attraction of HA to PAEs.14–16
In recent years, studies of DOM in landfill leachate have focused on the structural characteristics of DOM and its variation with different landfill treatment systems and landfill time.17 However, few studies have investigated changes in DOM in the landfill leachate treatment process. In addition, few studies have investigated the relationship between PAEs removal and DOM composition in landfill leachate treatment processes. Moreover, the main processes studied are advanced treatment technologies such as physicochemistry and combined processes.18 In this paper, the typical biological process anaerobic/anoxic/oxic (A/A/O) was used to treat landfill leachate to investigate the relationship between the removal rates of DBP and DEHP and the formation and properties of DOM in leachate before and after treatment.
2. Experimental
2.1. Reagents
The dichloromethane and methanol used in this study were of high-performance liquid chromatography grade, while all of the other reagents were of analytical grade. The water used in the experiment was generated by a Milli-Q system. DBP and DEHP (≥99%) and all of the other reagents were obtained from Tianjin Siyou Co. (Tianjin, China).
2.2. Sample collection
Leachate and sludge samples were collected from a MSW landfill leachate treatment facility in the Yuhang district of Hangzhou, China that has been operating since 1996. Leachate samples were taken from the adjusting pool, and sludge samples were collected from the anaerobic and aerobic pools. The pH of the raw leachate was 7.69, while the TOC, COD, BOD, TN, NH4+–N, NO3−–N, and TP values were 398, 1095, 221, 306, 236, 43, and 0.4 mg L−1, respectively. These findings indicate that the leachate is old. The B/C ratio of the leachate was only 0.20 and the leachate biodegradability was very low. The original DBP and DEHP concentrations in this leachate were 39.5 and 51.7 μg L−1, respectively, confirming the presence of PAEs.19 The anaerobic sludge was black with a particle size of 0.26 mm, density of 1.05 g cm−1, SVI of 47.71 mL g−1 and SV30 of 15.5%. The aerobic sludge was brown with a particle size of 4.82 mm, density of 1.01 g cm−1, SVI of 51.85 mL g−1, and SV30 of 16.0%.
2.3. Experimental set-up and operation conditions
A schematic diagram of the experimental set-up is shown in Fig. 1. The laboratory-scale A/A/O system consisted of a 6 L anaerobic reactor (A1), a 6 L anoxic reactor (A2), a 24 L oxic reactor (O1, O2, O3, and O4), and a 25 L sedimentation reactor, which were all made of Plexiglas. Motor-driven mixers were used in the anaerobic and anoxic reactors. Three air pumps and a set of diffusion aerators were used to supply air to the oxic reactor. A peristaltic pump was used to automatically fill the system from the feed tank. The sludge was returned from the bottom of the settling reactor to the anaerobic reactor via a peristaltic pump. The mixed liquor was recycled from the oxic reactor to the anoxic reactor by a peristaltic pump. The circulation ratios for the sludge and mixed liquor were one and two, respectively.
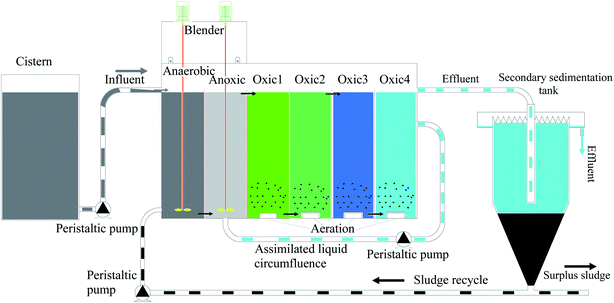 |
| Fig. 1 Schematic diagram of the A/A/O system. | |
The entire system was placed in a temperature-controlled room at 25 °C. The dissolved oxygen contents in the anaerobic, anoxic, and oxic reactors were measured using a dissolved oxygen meter (HQ30d, HACH) and found to be in the ranges of 0.11–0.31, 0.16–0.48, and 6.60–7.53 mg L−1, respectively. The mixed liquor suspended solid concentration in the system was 2500 mg L−1. The HRT was 3.6 d and the SRT was 30 d. The system was operated continuously for 6 months.
2.4. Analytical methods
The sludge indices, such as the SVI and MLSS, were determined using the standard methods.20 The indices, including the pH, TOC, COD, BOD5, TN, NH4+–N, NO3−–N, TP, Pb, Cr, Cd, Hg and As concentrations were monitored in the samples from each unit of the leachate treatment system. The Pb, Cr, Cd, Hg and As concentrations were determined using the standard methods.20 The leachate pH was determined using a pH meter (FE20, Mettler), the TOC concentration was determined with a TOC analyzer (Shimadzu), the COD concentration was determined with a COD analyzer (DBR200, DR1010, HACH), the BOD concentration was determined with a BOD analyzer (Trak II, HACH) and TN, NH4+–N, NO3−–N, and TP concentrations were determined with a continuous flow analyzer (AA3, Seal).
The samples for organic component analysis were processed as follows: the leachate was filtered through 0.45 μm millipore filters (Menbrana, Germany), then was ultrafiltered through different sized MW ultrafiltration membranes (100 kDa, 10 kDa, and 1 kDa, Millipore, USA). The dissolved organic carbon (DOC) was subsequently measured using a total organic carbon analyzer (Multi N/C3100; Analytik Jena AG, Germany).
Leachate from low to high concentrations was used to start the reactor. After the reactor reached stable operation, the target DBP and DEHP were added to give an initial PAE concentration of 300.0 μg L−1. The leachate sample extraction was conducted as follows. The HC–C18 cartridges (500 mg/3 mL, CNWBOND) were conditioned with 6 mL of methanol and 6 mL of pure water. Then the leachate sample (8 mL) was passed through the activated cartridge at a flow rate of 1.0 mL min−1. The cartridges were subsequently washed using 5 mL methanol
:
water (4
:
6 v
:
v), vacuumed for 30 s, and dried under nitrogen flow for 5 min. The extracted compounds were then eluted with 5 mL of methanol, after which the eluate was concentrated to 1.0 mL under a gentle stream of nitrogen. Next, the target DBP and DEHP were analyzed by high-performance liquid chromatography (e2695, Waters, USA). To accomplish this, the extracted sample was injected onto a sunfire-C18 column (4.6 × 150 mm, 5 μm, column temperature 25 °C) using a methanol
:
water (90
:
10 v
:
v) mixture as the mobile phase at a flow rate of 1.0 mL min−1. The concentration was determined by an ultraviolet detector set to 225 nm, and the injection volume was 40 μL. The recoveries of DBP in the leachate and sludge were 66.1–74.4% and 73.6–97.6%, respectively, while those of DEHP in the leachate and sludge were 84.2–95.9% and 66.2–107.2%. The detection limits of DBP and DEHP were 0.1 and 0.2 μg L−1, respectively.
3. Results and discussion
3.1. Removal of COD and other leachate contaminants
The efficiencies of COD, BOD5, TN, NH4+–N, NO3−–N, TP, Pb, Cr, Cd, Hg and As removal from the leachate by the treatment process are given in Table 1. The removal efficiencies were not high. Specifically, the removal efficiencies of COD and BOD5 were 46.8% and 50.5% respectively, the process had a very small effect on NH4+–N and no NO3−–N was removed. After the leachate was processed by all of the treatments, the removal efficiencies of TN and TP were 41.4% and 62.7%, respectively. Among metals, Pb, Cr, Cd, Hg and As were detected in all of the leachate samples. The treatment system removed all of the metals but, the removal efficiencies differed. Specifically, the removal efficiencies of Pb, Cd, Hg and As were 20.0%, 50.0%, 33.3% and 17.6%, respectively, while it is only 5.1% for Cr. With the exception of Hg and As, none of the other parameters of the leachate met the standards for pollution control of the MSW landfill site.21 These findings indicate that the effluent from the bioreactor must be treated again by other methods, such as membranes.22
Table 1 Removal efficiencies of leachate contaminants
Parameter |
COD |
BOD5 |
TN |
NH4+–N |
NO3−–N |
TP |
Pb |
Cr |
Cd |
Hg |
As |
Influent (mg L−1) |
1095 |
279 |
181 |
173 |
6.8 |
10.2 |
0.15 |
0.29 |
0.08 |
0.09 μg L−1 |
9.1 μg L−1 |
Effluent (mg L−1) |
582 |
138 |
106 |
89 |
6.7 |
3.8 |
0.12 |
0.26 |
0.04 |
0.06 μg L−1 |
7.5 μg L−1 |
Removal (%) |
46.8 |
50.5 |
41.4 |
48.6 |
1.5 |
62.7 |
20.0 |
5.1 |
50.0 |
33.3 |
17.6 |
3.2. Removal of TOC
TOC represents the level of organic matter in wastewater based on the carbon content. The TOC value was better than the COD at reflecting removal of organic matter in leachate in this biological treatment process. The TOC concentration was 393.0 mg L−1 in influent, while it was 26.0 mg L−1 in effluent, giving a removal rate of 93.4%. These findings indicate the biological treatment process had a good removal effect on organic matter. Landfill leachate mainly contains three kinds of organic compounds with molecular weight.23 Small molecular organic compounds were primarily composed of volatile fatty acids (VFA) and amino acids, which are easily biodegradable. The molecular weight of middle molecular weight organic matter is generally between 500 and 10
000 Da, mainly is fulvic acid. These materials contain carboxyl groups and phenolic hydroxyl groups. These substances are not only difficult to biodegrade, but also pass through microfiltration membranes, which is the cause of high COD levels in effluent. High molecular weight organic compounds are primarily composed of polysaccharides, proteins and humic acids, which are difficult to biodegrade under normal conditions.
Based on the classification of the above organic components, the removal efficiency of TOC in the four molecular weight interception regions after treatment was determined (Fig. 2). As shown in Fig. 2, the removal rate of TOC was high in each interception molecular weight range. When the molecular weight of components was less than 1 kDa, the influent concentration of TOC was 62.0 mg L−1 and the TOC removal rate was 95.8%. The highest concentration of influent TOC (182.1 mg L−1) was found for organic components that weighed between 1 and 10 kDa, which resulted in an effluent TOC concentration of 21.5 mg L−1 and a removal rate of 88.2%. Among the components weighing between 10 and 100 kDa, the removal rate of the influent TOC was highest, with a removal of 97.6% being observed when the influent concentration of TOC was 95.4 mg L−1. Among the components weighing more than 100 kDa, the TOC concentration was lowest (46.0 mg L−1) and the removal rate was 85.6%. These findings indicate that the main components of the landfill leachate were with molecular weights of 1–100 kDa. A large number of organic components were removed by this biological treatment process.
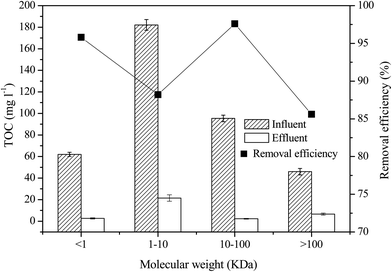 |
| Fig. 2 Distribution and removal of TOC in different MW fractions before and after treatment. | |
3.3. Removal of PAEs
The mean concentrations of DBP and DEHP in the leachate decreased from 300.0 to 6.1 μg L−1 and from 300.0 to 65.5 μg L−1, respectively, following leachate treatment, which included the A1, A2, O1, O2, O3 and O4 units (Fig. 3). These findings indicate that this treatment process had high simultaneous removal efficiencies for the two pollutants. The final DBP removal efficiency was 98.0%, and there were only trace levels of DBP in the effluent. In addition, DBP had a high removal efficiency of 95.5% after the anaerobic treatment, after which it was further degraded by each step. The DBP residual concentration in the oxic reactor was less than in the anaerobic and anoxic reactors, which is in agreement with the results of previous studies.24 The removal efficiency of DEHP was not as high as that of DBP in the treatment process, with the overall removal only reaching 78.2%. The removal rate of DEHP in the initial anaerobic reactor was only 43.2%. Although DEHP has stronger hydrophobicity and stronger affinity to sludge adsorption, its removal rate was lower than that of DBP. These findings indicated that the removal effect of sludge adsorption is limited and that degradation by anaerobic microorganisms was dominant in this process, which is similar to the results of a study reported by Asakura.25 The DEHP removal efficiency in the oxic reactor was clearly higher than those in the anaerobic and anoxic reactors. Overall, the results of this study show that the degradation rate of DEHP was much lower than that of DBP, although DBP and DEHP were degraded to a certain extent during landfill leachate treatment.
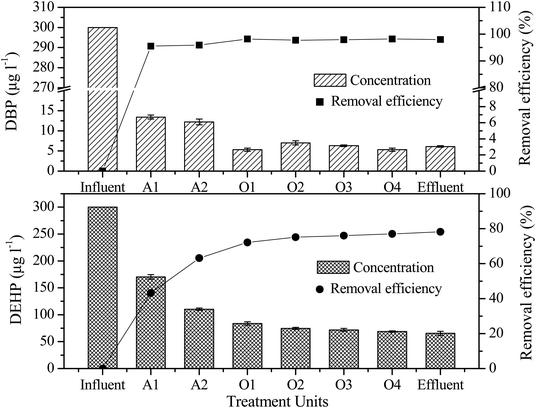 |
| Fig. 3 Effluent concentration and removal efficiency of DBP and DEHP in each unit. | |
3.4. Correlation between dissolved organic carbon and PAEs removal
DOM, which comprises the main fraction of leachate organics and is the primary source of residual COD, is usually expressed as DOC concentration (mg L−1) and has a significant effect on the transformation, degradation, and bioavailability of organic matter in leachate. DOM adsorption of organic pollutants is by hydrogen bond, van der Waals force and hydrophobic interaction. DOM is mainly composed of humic acid and has a high adsorption capacity for PAEs.13 The distribution of DBP and DEHP in different MW fractions of DOC in raw leachate is shown in Fig. 4. The results showed that DOC occurred in the highest concentration of the 1–10 kDa MW fraction, and that the DBP and DEHP concentrations were also higher in this fraction than in other MW fractions. The distribution of DOC and PAE concentrations between different MW ranges was the same and was positively correlated to some extent.
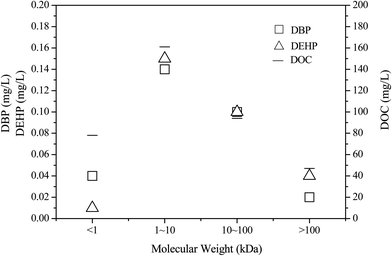 |
| Fig. 4 Distribution of DBP and DEHP in different MW fractions of DOC in raw leachate. | |
As shown in Fig. 5, the distribution of DOC and PAEs between the leachate treatment units was also similar. With movement from one treatment process to the next, DBP, DEHP, and DOC concentrations all decreased and showed some positive correlations with one another. These findings indicate that there may be some interaction between DOM and PAEs, which had some effect on the removal of PAEs in leachate.26
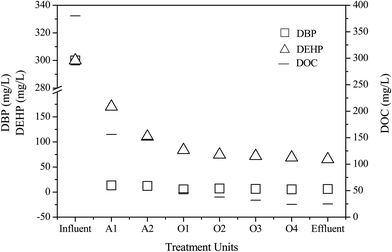 |
| Fig. 5 Concentration of DOC and PAEs of each unit during the leachate treatment process. | |
3.5. Relationship between dissolved organic matter humification and PAEs removal rate
The content of humic acid in leachate increases with increased landfill age. Humic acid is an important component of DOM in aged landfill leachate that is difficult to biodegrade and causes problems during leachate treatment. Many studies have confirmed the adsorption of humus on PAEs.27 The binding strength primarily depends on the hydrophobicity of PAEs and the structure and composition of humus. The molecular weight and aromaticity of DOM in humus are important characteristics determining the interaction between DOM and PAEs, and a higher the aromaticity of humus is associated with a higher molecular weight and a stronger association reaction with PAEs.28–32 As a result, the humification of DOM is enhanced, the adsorption of PAEs is enhanced, and the removal of PAEs is affected.
The DOC value and PAE concentration distribution of water samples in different molecular weight components before and after treatment are shown in Fig. 6. The DOC value of the influent was high in organic fractions with different molecular weights, with the maximum value of 178 mg L−1 appearing in components of 1–10 kDa. When combined with the distribution of organic components of TOC in Fig. 2, it can be seen that both TOC and DOC values showed the highest concentration of organic components in the molecular weight range of 1–10 kDa, indicating that organic compounds in this weight range have high aromaticity. After treatment, the DOC of each molecular weight interval decreased accordingly.
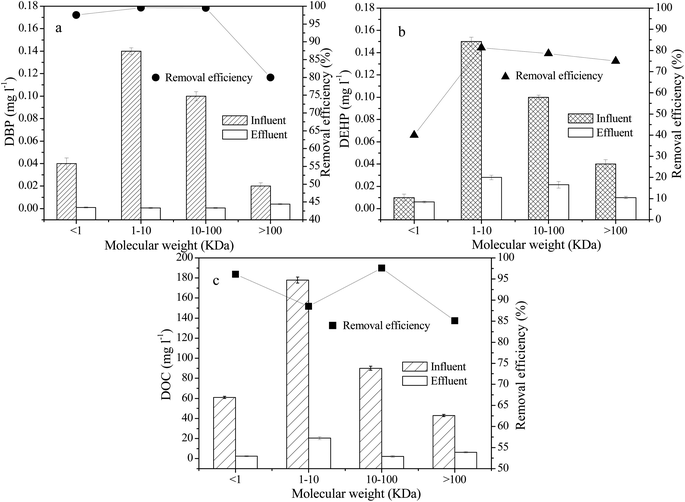 |
| Fig. 6 Change of DOC and PAEs in different size fractions of DOM before and after treatment (a) DBP, (b) DEHP, (c) DOC. | |
The DBP and DEHP in raw leachate was mainly observed in the 1–10 kDa MW fraction, and the DOC value was also relatively high in this fraction. These findings indicate that the degree of humification of DOM in leachate was high, and its adsorption effect on DBP and DEHP was significant. Following treatment, the removal rate of DOC was high in components with molecular weights ranging from 1 to 100 kDa, and was greater than that observed for other molecular intervals. Additionally, the removal rate of DBP and DEHP was over 90% at this time point, which was greater than that of other molecular regions. These findings indicate that the adsorption effect between DOM and PAEs indirectly influences the removal efficiency of PAEs. The main function of DOM is to provide a growth substrate for bacteria. PAEs are used effectively by microorganisms when they are absorbed onto the DOM in high quantities. The octanol–water partitioning coefficient (Kow) of DEHP is higher than that of DBP, and DEHP has a greater ability to adsorb organic molecules. In the present study, the DEHP concentrations were all higher than those of DBP when the MW of the organic fraction exceeded 1 kDa. Correspondingly, after process treatment, the removal rate of DBP was higher than that of DEHP for components with molecular weights less than 1 kDa, while the removal rates were similar for components with molecular weights greater than 100 kDa.
Overall, the results indicated the interaction between DOM and PAEs influenced PAE removal. Humus is a major component of DOM in aged landfill leachate that has an aromaticity and molecular weight that determine the interaction with PAEs, which influences the removal rate of PAEs. Some similar results were obtained from the study which evaluated the removal of PAEs in leachate by a coagulation and flocculation process. The removal of PAEs in the leachate was related to the hydrophobicity of PAEs and the MW of the leachate DOM. The decrease of the PAEs concentrations had a close relationship with the high aromaticity and large MW of the DOM present in the raw leachate.16 Hydrophobic contaminants with high Kow and DOM in leachate can be removed simultaneously through the complexation–flocculation process.17 In this study, both DOC and PAE concentrations were relatively high in raw leachate in the 1–100 kDa MW fraction, and the PAE removal efficiencies were higher when the DOC concentrations were lower in this fraction. The positive correlation between DOC and PAE concentration in raw leachate and in each treatment unit indicates that a high abundance of humus in leachate facilitates the removal of PAEs during the leachate treatment process.
4. Conclusions
For aged landfill leachate, the A/A/O process has a good removal effect on DBP and DEHP, with removal efficiencies reaching 98.0% and 78.2%, respectively. In addition, there was a positive correlation between DOC and PAEs concentration in landfill raw leachate and process treatment. The results also showed that the adsorption of PAEs by DOM in leachate is beneficial to the effective removal of DBP and DEHP in the leachate treatment process, and that DOM humification was closely related to PAEs removal. Finally, DOC was mainly distributed in the 1–100 kDa region, as were the aromatic components of humus and PAEs. Overall, the effective reduction of DOC and aromaticity of PAEs resulted in a significant improvement in PAEs removal efficiency.
Conflicts of interest
There are no conflicts of interest to declare.
Acknowledgements
This work was funded by the National Natural Science Foundation of China (Grant No. 51678531, 51878617, 51778579, and 21876165). We thank Jeremy Kamen, MSc, from Liwen Bianji, Edanz Group China (http://www.liwenbianji.cn/ac), for editing the English text of a draft of this manuscript.
References
- Q. Q. Zhang, B. H. Tian, X. Zhang, A. Ghulam, C. R. Fang and R. He, Waste Manag., 2013, 33, 2277–2286 CrossRef CAS PubMed.
- C. R. Fang, Y. X. Chu, L. H. Jiang, H. Wang, Y. Y. Long and D. S. Shen, J. Mater. Cycles Waste Manage., 2018, 20, 585–591 CrossRef CAS.
- H. Asakura, T. Matsuto and N. Tanaka, Waste Manag., 2004, 24, 613–622 CrossRef CAS PubMed.
- C. R. Fang, Y. Y. Long and D. S. Shen, Bioresour. Technol., 2009, 100, 5664–5670 CrossRef CAS PubMed.
- G. Lottrup, A. M. Andersson, H. Leffers, G. K. Mortensen, J. Toppari, N. E. Skakkebaeek and K. M. Main, Int. J. Androl., 2006, 29, 172–180 CrossRef CAS PubMed.
- D. W. Liang, T. Zhang and H. H. Fang, Appl. Microbiol. Biotechnol., 2008, 80, 183–198 CrossRef CAS PubMed.
- J. Ejlertsson, M. Alnervik, S. Jonsson and B. H. Svensson, Environ. Sci. Technol., 1997, 3, 2761–2764 CrossRef.
- J. Boonnorat, C. Chiemchaisri, W. Chiemchaisri and K. Yamamoto, J. Hazard. Mater., 2014, 277, 93–101 CrossRef CAS PubMed.
- F. Lu, C. H. Chang, D. J. Lee, P. J. He, L. M. Shao and A. Su, Chemosphere, 2009, 74, 575–582 CrossRef CAS PubMed.
- H. Zhang, C. H. Chang, F. Lu, D. J. Lee, P. J. He, L. M. Shao and A. Su, Sci. Total Environ., 2009, 407, 879–886 CrossRef CAS PubMed.
- Z. Zheng, P. J. He, L. M. Shao and D. J. Lee, Water Res., 2007, 41, 4696–4702 CrossRef CAS PubMed.
- Y. D. Xu, D. B. Yue, Y. Zhu and Y. F. Nie, Chemosphere, 2006, 64, 903–911 CrossRef CAS PubMed.
- M. J. Bauer and R. Herrmann, Waste Manage. Res., 1998, 16, 446–454 CrossRef CAS.
- S. K. Marttinen, R. H. Kettunen and J. A. Rintala, Sci. Total Environ., 2003, 301, 1–12 CrossRef CAS PubMed.
- F. Lu, H. Zhang, C. H. Chang, D. J. Lee, P. J. He, L. M. Shao and A. Su, Chemosphere, 2008, 72, 1381–1386 CrossRef CAS PubMed.
- Z. Zheng, H. Zhang, P. J. He, L. M. Shao, Y. Chen and L. Pang, Chemosphere, 2009, 75, 180–186 CrossRef CAS PubMed.
- C. X. Zhang and Y. X. Wang, Waste Manag., 2009, 29, 110–116 CrossRef PubMed.
- P. J. He, Z. Zheng, H. Zhang, L. M. Shao and Q. Y. Tang, Sci. Total Environ., 2009, 407, 4928–4933 CrossRef CAS PubMed.
- C. R. Fang, Y. Y. Long and D. S. Shen, Environ. Sci. Pollut. Res., 2014, 21, 7641–7649 CrossRef CAS PubMed.
- EPA of China, Standard for waste water analysis, China Environmental Science Press, Beijing, China, 1989 Search PubMed.
- China’s Environmental Protection Agency, Standard for pollution control on the landfill site of municipal solid waste (GB 16889-2008), China Environmental Science Press, Beijing, China, 2008 Search PubMed.
- H. Y. Liu, Y. X. Chu and C. R. Fang, Environ. Eng. Manage. J., 2016, 15, 2127–2133 CrossRef CAS.
- B. Wichitsathian, S. Sindhuja, C. Visvanathan and K. H. Ahn, J. Environ. Sci. Health, Part A: Toxic/Hazard. Subst. Environ. Eng., 2004, 39, 2391–2404 CrossRef CAS PubMed.
- J. L. Wang, P. Liu and Y. Qian, Process Biochem., 1999, 34, 745–749 CrossRef CAS.
- H. Asakura and T. Matsuto, Waste Manag., 2009, 29, 1852–1859 CrossRef CAS PubMed.
- X. L. Chai, R. Ji, J. Wu, H. H. Tong and Y. C. Zhao, Chemosphere, 2009, 78, 1362–1367 Search PubMed.
- S. Renou, S. Poulain, J. G. Givaudan and P. Moulin, J. Membr. Sci., 2008, 313, 9–22 CrossRef CAS.
- H. Yamamoto, H. M. Liljestrand, Y. Shimizu and M. Morita, Environ. Sci. Technol., 2003, 37, 2646–2657 CrossRef CAS PubMed.
- P. J. He, J. F. Xue, L. M. Shao, G. J. Li and D. J. Lee, Water Res., 2006, 40, 1465–1473 CrossRef CAS PubMed.
- C. Niederer, R. P. Schwarzenbach and K. U. Goss, Environ. Sci. Technol., 2007, 41, 6711–6717 CrossRef CAS PubMed.
- I. V. Perminova, N. Y. Grechishcheva and V. S. Petrosyan, Environ. Sci. Technol., 1999, 33, 3781–3787 CrossRef CAS.
- L. Persson, T. Alsberg, A. Ledin and G. Odham, Chemosphere, 2006, 64, 1093–1099 CrossRef CAS PubMed.
|
This journal is © The Royal Society of Chemistry 2019 |
Click here to see how this site uses Cookies. View our privacy policy here.