DOI:
10.1039/C9RA08248D
(Paper)
RSC Adv., 2019,
9, 39904-39913
Retracted Article: MicroRNA-1271 modulates hepatitis B virus replication, cell proliferation and apoptosis in hepatitis B virus-related hepatocellular carcinoma by targeting SIRT1
Received
10th October 2019
, Accepted 25th November 2019
First published on 2nd December 2019
Abstract
Chronic hepatitis B virus (HBV) infection is a leading cause of hepatocellular carcinoma (HCC). Certain studies have revealed that microRNAs play crucial roles in HBV-related HCC. The aim of this study was to investigate the effects of microRNA-1271 (miR-1271) on HBV replication, cell proliferation and apoptosis in HBV-related HCC. The expression of HBV DNA and miR-1271 was detected by quantitative real time-polymerase chain reaction (qRT-PCR). The mRNA and protein levels of SIRT1 were detected by qRT-PCR and western blot analysis, respectively. HBV replication was assessed by the expression of HBV DNA and the levels of HBsAg and HBeAg. Cell proliferation was assessed by cell counting kit-8 (CCK-8) and 5-bromo-2-deoxyuidine (BrdU) assay, and apoptosis was evaluated by flow cytometry assay, enzyme-linked immunosorbent assay (ELISA) and the activity of caspase-3. The relationship between miR-1271 and SIRT1 was predicated by online software and confirmed by dual-luciferase reporter assay, RNA immunoprecipitation (RIP) and pull-down assay. We first found that the expression of miR-1271 was downregulated and SIRT1 was upregulated in both HBV-related HCC tissues and cells. Overexpression of miR-1271 inhibited HBV replication and cell proliferation whilst promoting apoptosis in HBV-related HCC cells. Subsequently, SIRT1 was identified as a target of miR-1271. Moreover, overexpression of SIRT1 reversed the effects of miR-1271 overexpression on HBV replication, cell proliferation and apoptosis in HBV-related HCC cells. In conclusion, our study demonstrated that miR-1271 inhibited HBV replication and proliferation and promoted apoptosis of HBV-related HCC cells via targeting SIRT1, which might contribute to the diagnosis and therapy of HBV-related HCC.
1. Introduction
Hepatocellular carcinoma (HCC) is a prevalent malignant tumor around the world, and about 700
000 new cases occur every year.1 Among them, at least 50% are infected by Hepatitis B virus (HBV).2 HBV is one of the members of hepadnaviridae family, and its genome is a double-stranded DNA.3 After HBV infects a host, its genome transforms into a covalently closed circular DNA (cccDNA), playing a vital function in HBV replication.4 The control of HBV replication is an effective therapeutic strategy for HBV-related HCC. Hence, to explore the mechanism of the host gene in regulating HBV replication and cell proliferation is of great significance for the therapy of HBV-related HCC.
MicroRNAs (miRNAs) are a kind of RNAs with approximative 20 nucleotides which cannot be translated into proteins. It is indicated that miRNAs involve in various biochemical processes via sponging mRNAs to regulate their expression.5,6 Recently studies have proved that miRNAs regulate the progression of HBV-related HCC.7,8 For instance, miR-125a-5p level is reduced in HBV-related HCC tissues, and overexpression of miR-125a-5p impedes HBV-related HCC cells growth.9 MiRNA-520a inhibits HBV replication in HBV-related HCC cells by passivating AKT.10 Besides, miRNA can be considered as an applicable diagnostic target for HBV-related HCC.11 MiR-1271 has been revealed to play a tumor-suppressor activity in HBV-related HCC.12 However, the molecular mechanism of miR-1271 on regulating HBV-related HCC development still remains largely unknown.
Sirtuin1 (SIRT1) is a histone deacetylase, which is related to many diseases, including cancers. It can deacetylate histone as well as other target proteins, such as p53, AP-1 and NF-κB, thereby playing a role in certain cellular processes.13,14 SIRT1 has been indicated to serve as a tumor promoter in HCC.15,16 SIRT1 facilitates tumorigenesis and predicts poor prognosis in HCC.17 Upregulation of SIRT1 facilitates epithelial–mesenchymal transition (EMT) in HCC cells.18 In addition, SIRT1 is upregulated in HBV-infected liver tissues, and it interacts with viral transactivator X protein and reinforces HBV gene expression.19
In this study, the levels of miR-1271 and SIRT1 in HBV-related HCC tissues and cells were measured. Subsequently, we researched the roles of miR-1271 in HBV replication, cell proliferation and apoptosis in HBV-related HCC cells. Finally, the relationship between miR-1271 and SIRT1 was confirmed and the regulatory mechanism was further investigated.
2. Materials and methods
2.1. Clinical sample and cell culture
After 12 patients with HBV-related HCC signed the informed consent, tumor and adjacent normal tissues were obtained by surgical dissection at The Third Central Clinical College of Tianjin Medical University (Tianjin Institute of Hepatobiliary Disease, Tianjin Key Laboratory of Artificial Cells). All patients had not undergone any other therapy. This study had acquired approval from Ethics Committee of The Third Central Clinical College of Tianjin Medical University (Tianjin Institute of Hepatobiliary Disease, Tianjin Key Laboratory of Artificial Cells) (Tianjin, China.), which was performed in strict accordance with the Declaration of Helsinki Principles for Care and Use of Laboratory Animals. Informed consents were obtained from all participants.
Huh7, HepAD38 and HepaRG were bought from Biopredic International (Rennes, France). The vector pcDNA 3.0–1.3 mer containing a 1.3 mer fragment of HBV genomic DNA was transfected into Huh7 cells, named Huh7-1.3 cells. Cells were cultured in Dulbecco's modified Eagle medium (DMEM; Invitrogen, Carlsbad, CA, USA) with 10% fetal bovine serum (FBS; Invitrogen), maintaining the conditions of 37 °C and 5% CO2.
2.2. Quantitative real time-polymerase chain reaction (qRT-PCR)
Total RNA was extracted by TRIzol reagent (Invitrogen). Then reverse-transcription was implemented using PrimeScript RT Kit (TaKaRa, Dalian, China). U6 and glyceraldehyde-3-phosphate dehydrogenase (GAPDH) were employed to normalize miR-1271 and SIRT1 mRNA. The qPCR was carried out with SYBR Green PCR Master Mix (Ambion, Carlsbad, CA, USA). The 2−ΔΔCt method was used to assess the relative expression. The primers were listed below.
miR-1271-F: CAGCACTTGGCACCTAGCA,
miR-1271-R: TATGGTTGTTCTCCTCTCTGTCTC;
U6-F: CGCTTCGGCAGCACATATACTAA,
U6-R: TATGGAACGCTTCACGAATTTGC;
SIRT1-F: TAGCCTTGTCAGATAAGGAAGGA,
SIRT1-R: ACAGCTTCACAGTCAACTTTGT;
GAPDH-F: CGGATTTGGTCGTATTGGG,
GAPDH-R: CTGGAAGATGGTGATGGGATT.
For the detection of HBV DNA, DNA extraction kits (Guangzhou Jianlun Biotechnology Co., Ltd., Guangzhou, China) was used to extract the DNA. The HBV DNA level was detected by qRT-PCR with the primers of 5′-GGTCTGCGCACCAGCACC-3′ (forward) and 5′-GAACTTTAGGCCCATATTAGTG-3′ (reverse). HBV DNA level was determined by the average threshold cycle values.
2.3. Western blot analysis
Whole proteins were extracted from HCC tissues and cells by the protein extraction kit (Beyotime, Shanghai, China). Then proteins were subjected to 10% sodium dodecyl sulfate-polyacrylamide gel electrophoresis (SDS-PAGE) and shifted onto a polyvinylidene difluoride (PVDF) membrane (Millipore, Billerica, MA, USA). After blocked with 5% skim milk dissolved with TBST buffer, the membrane was incubated with primary antibodies anti-SIRT1 (1
:
1000, Abcam, Cambridge, UK) or anti-β-actin (1
:
5000, Abcam) at 4 °C overnight. Subsequently, the secondary antibody (1
:
5000, Abcam) was used to incubate the membrane at room temperature for 1 h. An enhanced chemiluminescence reagent (Beyotime) was used to detect the protein levels.
2.4. Detection of HBV surface antigen (HBsAg) and e antigen (HBeAg)
The culture supernatant was converged, and the concentrations of HBsAg and HBeAg were detected by diagnostic kit for HBsAg and HBeAg (Kehua Bio-engineering, Shanghai, China) according to the instructions, respectively.
2.5. Cell transfection
MiR-1271 mimic (miR-1271) and the control fragment miR-NC were obtained from Sangon Biotech (Shanghai, China). SIRT1 overexpression vector (SIRT1) was constructed by cloning the full sequences of SIRT1 cDNA and inserting it to pcDNA3.1 vector. When the confluence of cells was ∼70%, transfection was carried out using Lipofectamine 2000 reagent (Invitrogen) under the instructions.
2.6. Cell viability assay
Cell Counting Kit-8 (CCK-8; Beyotime) was used for the detection of cell viability. Cells were placed into a 96-well plate at 2000 cells per well. At 24 h after transfection, the cells were added with Cell Counting Kit-8 reagent and incubated for 2 h. Finally, the absorbance at 450 nm was detected with a microplate reader (Thermo Fisher Scientific, Waltham, MA, USA) for the evaluation of cell viability.
2.7. Cell proliferation assay
5-Bromo-2-deoxyuidine (BrdU) assay was used to detect cell proliferation. Cells were placed in a 96-well plate. After transfection for 24 h, BrdU cell proliferation enzyme-linked immunosorbent assay (ELISA) kit (Abcam) was used to measure cell proliferation in conformity to the instructions.
2.8. Flow cytometry assay
Apoptosis was determined by annexin V-fluorescein isothiocyanate (V-FITC)/propidium iodide (PI) apoptosis kit (BD Bioscience, San Diego, CA, USA) under the manufacturers' manuals. Cells were collected at 24 h after transfection and resuspended with binding buffer. Annexin V-FITC and PI were added to stain the cells for 20 min in a dark condition. Apoptosis was measured by flow cytometry (BD Bioscience).
2.9. Detection of apoptosis
The cell death ELISA detection kit (Roche, Inc., Basel, Switzerland) was used for the detection of apoptosis as described.20 Cell apoptosis was detected with a spectrophotometer at 405 nm.
2.10. Detection of caspase-3 activity
The activity of caspase-3 was detected by caspase-3 fluorescent assay kit (Nanjing KeyGEN Biotech, Nanjing, China). After proteins were extracted from cells, synthetic fluorescent substrates were added to equal protein samples for a reaction for 1 h at 37 °C. The activity of caspase-3 was assessed through the absorbance at 405 nm.
2.11. Dual-luciferase reporter assay
The potential binding sites between miR-1271 and SIRT1 were predicted by DIANA tools-microT-CDS. The 3′-untranslated region (3′-UTR) of the SIRT1 gene containing the putative binding sequence of miR-1271 (SIRT 3′UTR WT) and the control (SIRT 3′UTR MUT) were cloned and inserted into the pGL3 (Promega, Madison, WI, USA), respectively. Reporter vector (SIRT 3′UTR WT or SIRT 3′UTR MUT) and miR-1271 mimic or miR-NC were co-transfected into Huh7-1.3 and HepAD38 cells. The luciferases activities were detected after transfection for 48 h with a Dual-Luciferase reporter system (Promega).
2.12. RNA immunoprecipitation (RIP) assay
RIP assay was carried out with an RNA-Binding Protein Immunoprecipitation Kit (Millipore). MiR-1271 mimic or miR-NC was transfected into Huh7-1.3 and HepAD38 cells, and cells were lysed with RIP lysis buffer after 48 h. Magnetic beads and human anti-Ago2 (Abcam) or negative control anti-IgG (Abcam) were used to incubate the cell lysates. Samples were digested with proteinase K, and then the co-precipitated RNAs were isolated and purified for qRT-PCR.
2.13. RNA pull-down assay
Pull-down assay was carried out as described.21 Biotinylated miRNA (Bio-NC, Bio-miR-1271 WT or Bio-miR-1271 MUT) was transfected into Huh7-1.3 and HepAD38 cells. Cells were collected after 48 h and then lysed with lysis buffer. Cells lysate was incubated with MyOne C1 Dynabeads (Invitrogen) and then washed by lysis buffer. After elution, the RNA level was assessed by qRT-PCR.
2.14. Statistical analysis
Each experiment was done with at least three biological replicates. Data were analyzed by SPSS 22.0 and showed as mean ± standard deviation. Comparison between two groups was assayed by Student's t-test. While one-way analysis of variance (ANOVA) with the Tukey HSD post hoc test was employed to compare the difference of multiple groups. P-value < 0.05 was considered statistically significant.
3. Results
3.1. The expression of miR-1271 was downregulated and the level of SIRT1 was upregulated in HBV-HCC tissues and cells
To explore whether miR-1271 and SIRT1 play roles in HBV-HCC, we first measured the expression of miR-1271 and SIRT1 in HBV-HCC tissues and cells. The qPCR result showed that the expression of miR-1271 was lower in HBV-HCC tissues than that in normal tissues (Fig. 1A). Moreover, miR-1271 expression was observably downregulated in Huh7-1.3 and HepAD38 cells compared with that in Huh7 and HepaRG cells (Fig. 1B). Inversely, SIRT1 mRNA level was upregulated in HBV-HCC tissues as well as Huh7-1.3 and HepAD38 cells (Fig. 1C and D). Also, the western blot result indicated that the protein level of SIRT1 was significantly increased in Huh7-1.3 and HepAD38 cells (Fig. 1E), which was in accordance with the qRT-PCR results. These data suggested that miR-1271 and SIRT1 might have functions in HBV-related HCC.
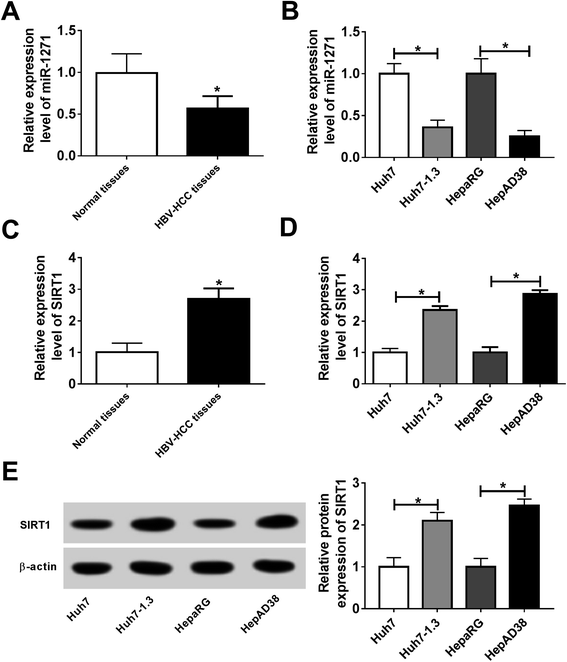 |
| Fig. 1 Expression of miR-1271 and SIRT1 in HCC tissues and cells. (A) The expression of miR-1271 in HBV-HCC tissues and normal tissues was detected by qRT-PCR. (B) The expression of miR-1271 in Huh7, Huh7-1.3, HepaRG and HepAD38 cells was detected by qRT-PCR. (C) The mRNA level of SIRT1 in HBV-HCC tissues and normal tissues was detected by qRT-PCR. (D and E) The mRNA and protein levels of SIRT1 in Huh7, Huh7-1.3, HepaRG and HepAD38 cells were detected by qRT-PCR and western blot. *P < 0.05. | |
3.2. Overexpression of miR-1271 inhibited the replication of HBV in HBV-HCC cells
The levels of HBV DNA and secretions were considered as evidences of HBV infection. In our study, we found that the HBV DNA level was increased in Huh7-1.3 and HepAD38 cells when compared with Huh7 and HepaRG cells (Fig. 2A). Besides, the concentrations of HBsAg and HBeAg were also elevated in Huh7-1.3 and HepAD38 cells (Fig. 2B and C), proving that Huh7-1.3 and HepAD38 cells were infected by HBV. Subsequently, we overexpressed miR-1271 in Huh7-1.3 and HepAD38 cells, and qRT-PCR results showed that the expression of miR-1271 was enhanced in Huh7-1.3 and HepAD38 cells transfected with miR-1271 (Fig. 2D). The level of HBV DNA was downregulated by miR-1271 overexpression (Fig. 2E), and HBsAg and HBeAg were also markedly reduced in Huh7-1.3 and HepAD38 cells transfected with miR-1271 (Fig. 2F and G). These results revealed that miR-1271 overexpression repressed HBV replication.
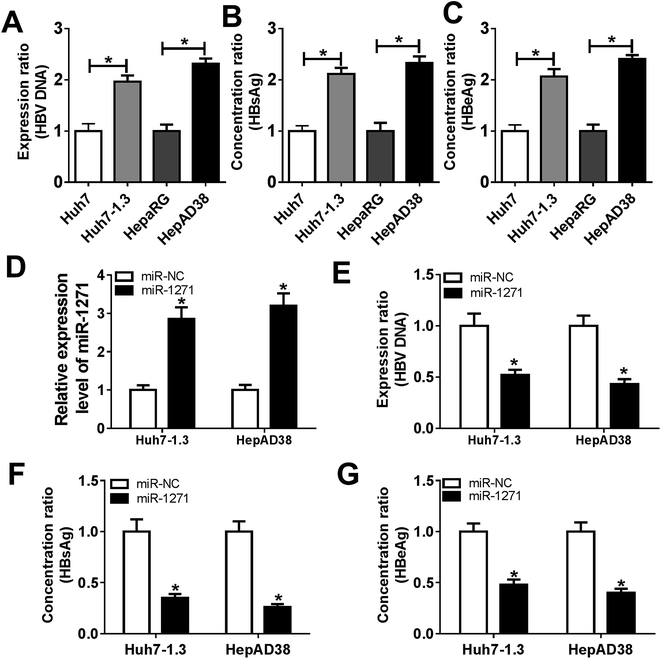 |
| Fig. 2 MiR-1271 inhibited HBV replication in HBV-related HCC cells. (A) The level of HBV DNA in Huh7, Huh7-1.3, HepaRG and HepAD38 cells was detected by qRT-PCR. (B and C) HBsAg and HBeAg levels were detected from Huh7, Huh7-1.3, HepaRG and HepAD38 cells culture. (D) The levels of miR-1271 in Huh7-1.3 and HepAD38 cells transfected with miR-1271 mimic was detected by qRT-PCR. (E) The level of HBV DNA in Huh7-1.3 and HepAD38 cells transfected with miR-1271 mimic was detected by qRT-PCR. (F and G) HBsAg and HBeAg levels were detected after miR-1271 was overexpressed in Huh7-1.3 and HepAD38 cells. *P < 0.05. | |
3.3. Overexpression of miR-1271 suppressed proliferation and induced apoptosis in HBV-HCC cells
To investigate the effects of miR-1271 on HBV-HCC cells, we detected cell viability, proliferation and apoptosis. CCK-8 assay and BrdU assays exhibited that overexpression of miR-1271 inhibited cell viability and proliferation (Fig. 3A and B). Then apoptosis was determined by flow cytometry and ELSIA. The results presented that apoptosis was promoted in Huh7-1.3 and HepAD38 cells transfected with miR-1271 (Fig. 3C and D). In addition, the activity of caspase-3 was also measured to assess apoptosis. After transfection with miR-1271, the activity of caspase-3 was remarkably elevated (Fig. 3E).
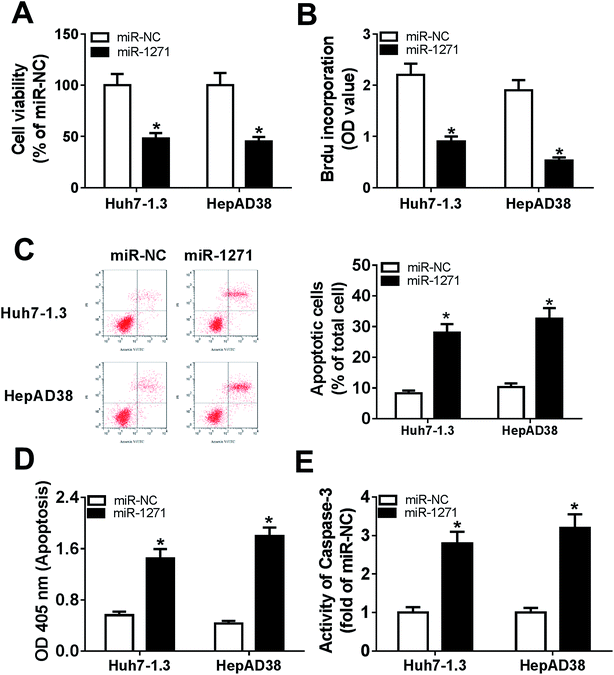 |
| Fig. 3 MiR-1271 suppressed proliferation and promoted apoptosis in HBV-HCC cells. Huh7-1.3 and HepAD38 cells were transfected with miR-1271 mimic or miR-NC for 24 h. (A) Cell viability was assessed by CCK-8 assay. (B) Cell proliferation was measured by BrdU-ELISA assay. (C and D) Apoptosis was assessed by flow cytometry and cell death ELISA detection kit. (E) The activity of caspase-3 was detected to assess apoptosis. *P < 0.05. | |
3.4. SIRT1 was a direct target of miR-1271
The relationship between miR-1271 and SIRT1 was predicted by DIANA tools-microT-CDS, and the predicted binding sites were presented (Fig. 4A). Dual-luciferase assay was performed to confirm the targeted relationship. The luciferase activity was significantly reduced by miR-1271 in both Huh7-1.3 and HepAD38 cells transfected with SIRT1 3′UTR WT rather than SIRT1 3′UTR MUT (Fig. 4B and C). The Ago proteins bind to the mature miRNA and generate RNA-induced silencing complex (RISC), thereby resulting gene silencing. We detected the enrichment of SIRT1 with anti-Ago2 by RIP, and the result suggested that miR-1271 and SIRT1 generated RISC in Huh7-1.3 and HepAD38 cells (Fig. 4D). We next carried out RNA pull-down assay, and the data indicated that SIRT1 was bonded by Bio-miR-1271 WT but not Bio-miR-1271 MUT in both Huh7-1.3 and HepAD38 cells (Fig. 4E and F). These results proved that SIRT1 was a direct target of miR-1271. In addition, the protein level of SIRT1 was downregulated in Huh7-1.3 and HepAD38 cells transfected with miR-1271 (Fig. 4G).
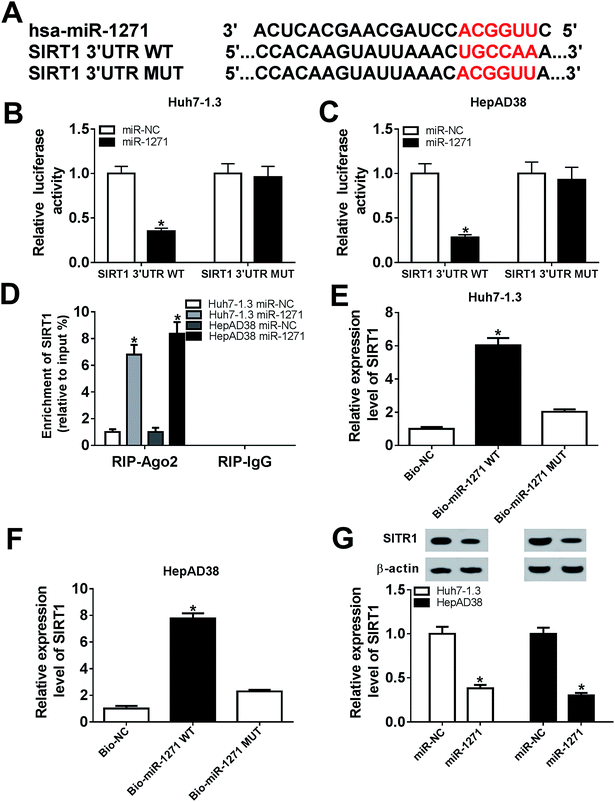 |
| Fig. 4 SIRT1 was a direct target of miR-1271. (A) The potential binding site between miR-1271 and SIRT1 was predicted by online software. (B and C) The relative luciferase activities of SIRT1 3′UTR WT and SIRT1 3′UTR MUT in Huh7-1.3 and HepAD38 cells were detected. (D) Enrichment of SIRT1 was detected by RIP assay after transfected with miR-1271 in Huh7-1.3 and HepAD38 cells. (E and F) Pull down assay was performed to confirm the relationship between miR-1271 and SIRT1 in Huh7-1.3 and HepAD38 cells. (G) The protein level of SIRT1 in Huh7-1.3 and HepAD38 cells transfected with miR-1271 was detected by western blot. *P < 0.05. | |
3.5. Overexpression of SIRT1 reversed effects of miR-1271 mimic on HBV replication in HBV-HCC cells
To further explore the relationship between miR-1271 and SIRT1, we first overexpressed SIRT1 in Huh7-1.3 and HepAD38 cells, and the western blot result verified the efficiency of SIRT1 overexpression (Fig. 5A). Then we detected the level of HBV DNA, and the result indicated that overexpression of SIRT1 promoted the expression of HBV DNA (Fig. 5B). Moreover, the concentrations of HBsAg and HBeAg were increased when Huh7-1.3 and HepAD38 cells were transfected with SIRT1 compared with the control (Fig. 5C and D). Subsequently, Huh7-1.3 and HepAD38 cells were transfected with miR-1271 or miR-1271 + SIRT1, and the protein level of SIRT1 was downregulated by miR-1271 mimic but reversed by SIRT1 overexpression (Fig. 5E). The HBV DNA level was decreased in Huh7-1.3 and HepAD38 cells transfected with miR-1271 and reverted when transfected with miR-1271 + SIRT1 (Fig. 5F). Similarly, the suppressed HBsAg and HBeAg levels by miR-1271 were reversed by the overexpression of SIRT1 in Huh7-1.3 and HepAD38 cells (Fig. 5G and H).
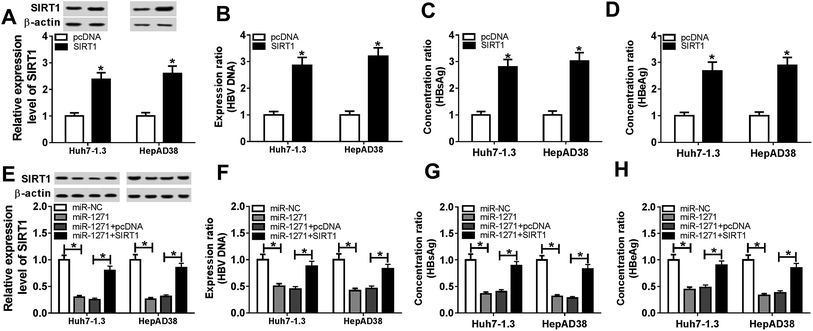 |
| Fig. 5 Overexpression of SIRT1 reversed effects of miR-1271 on HBV replication in HBV related HCC cells. (A) The protein level of SIRT1 was detected by western blot in Huh7-1.3 and HepAD38 cells transfected with SIRT1. (B) The level of HBV DNA was detected by western blot in Huh7-1.3 and HepAD38 cells transfected with SIRT1. (C and D) HBsAg and HBeAg levels were detected after SIRT1 was overexpressed in Huh7-1.3 and HepAD38 cells. (E) The protein level of SIRT1 was detected by western blot in Huh7-1.3 and HepAD38 cells transfected with miR-1271 or miR-1271 + SIRT1. (F) The level of HBV DNA was detected by western blot in Huh7-1.3 and HepAD38 cells transfected with miR-1271 or miR-1271 + SIRT1. (G and H) HBsAg and HBeAg levels were detected after Huh7-1.3 and HepAD38 cells were transfected with miR-1271 or miR-1271 + SIRT1. *P < 0.05. | |
3.6. Overexpression of SIRT1 reversed effects of miR-1271 mimic on proliferation and apoptosis in HBV-HCC cells
We further investigated the effects of SIRT1 overexpression on proliferation and apoptosis in Huh7-1.3 and HepAD38 cells. As shown in Fig. 6A and B, cell viability and proliferation of Huh7-1.3 and HepAD38 cells were enhanced by SIRT1 overexpression. On the contrary, overexpression of SIRT1 repressed apoptosis of Huh7-1.3 and HepAD38 cells (Fig. 6C and D). And the activity of caspase-3 was also inhibited by SIRT1 overexpression (Fig. 6E). Then, miR-1271 or miR-1271 + SIRT1 was transfected into Huh7-1.3 and HepAD38 cells. The inhibited cell viability and proliferation resulted by miR-1271 were rescued by overexpression of SIRT1 (Fig. 6F and G). Additionally, apoptosis was induced when transfected with miR-1271 and reversed when transfected with miR-1271 + SIRT1 in Huh7-1.3 and HepAD38 cells (Fig. 6H and I). Moreover, we detected the activity of caspase-3, and the result was consistent with apoptosis result (Fig. 6J).
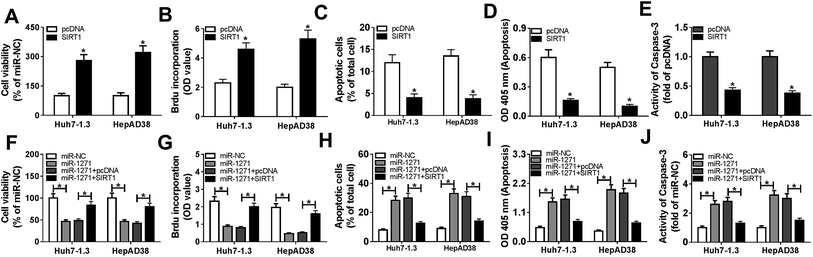 |
| Fig. 6 Overexpression of SIRT1 reversed effects of miR-1271 on proliferation and apoptosis of HBV related HCC cells. (A) Cell viability of Huh7-1.3 and HepAD38 cells transfected with SIRT1 was assessed by CCK-8 assay. (B) The proliferation of Huh7-1.3 and HepAD38 cells transfected with SIRT1 was measured by BrdU-ELISA assay. (C and D) Apoptosis of Huh7-1.3 and HepAD38 cells transfected with SIRT1 was assessed by flow cytometry and cell death ELISA detection kit. (E) The activity of caspase-3 was detected in Huh7-1.3 and HepAD38 cells transfected with SIRT1. (F) Cell viability was assessed by CCK-8 assay in Huh7-1.3 and HepAD38 cells transfected with miR-1271 or miR-1271 + SIRT1. (G) Cell proliferation was measured by BrdU-ELISA assay in Huh7-1.3 and HepAD38 cells transfected with miR-1271 or miR-1271 + SIRT1. (H and I) Apoptosis was assessed by flow cytometry and cell death ELISA detection kit in Huh7-1.3 and HepAD38 cells transfected with miR-1271 or miR-1271 + SIRT1. (J) The activity of caspase-3 was detected in Huh7-1.3 and HepAD38 cells transfected with miR-1271 or miR-1271 + SIRT1. *P < 0.05. | |
4. Discussion
HCC is the most primary type of liver cancer, accounting for more than 70% of liver cancer.22 Despite many efforts and progresses in HCC diagnosis and therapy have been made, the lack of early diagnostic method and poor prognosis are still severe problems.23 Therefore, it is significant to explore the regulatory mechanism of HCC development. HBV is well known to associate with HCC. The interaction between host and virus is crucial for the progress of HBV-related HCC.24 Increasing evidence indicated that miRNAs played vital roles in HBV-related HCC. Here, our data showed that miR-1271 was downregulated in HBV-related HCC tissues and cells. The reduced expression of miR-1271 implied that miR-1271 connected with HBV infection, and miR-1271 might be a promising diagnosis target for HBV-related HCC.
MiRNAs have been shown to impact on viral replication through direct and indirect mechanisms.25 MiR-1307 promoted the degradation of the foot-and-mouth disease virus structural protein VP3 through the proteasome pathway, leading to a decreased viral replication.26 Overexpression of miR-let-7c obviously inhibited HCV replication through activating haem oxygenase-1 connection (HO-1).27 We explored the function of miR-1271 on HBV replication and demonstrated overexpression of miR-1271 decreased HBV DNA level. At the meantime, HBsAg and HBeAg levels were also downregulated by miR-1271. These results exhibited that miR-1271 acted as an inhibitor on HBV replication.
High level of HBV replication contributes to HCC oncogenesis. The inhibiting effect of miR-1271 on HBV replication suggested that miR-1271 might serve as an anti-oncogene in HBV-related HCC. MiR-1271 has been indicated to involve in tumor progress and development in various cancers. A previous study revealed that miR-1271 suppressed EMT and cell viability in ovarian cancer.28 Overexpression of miR-1271 inhibited ERK/MAPK signaling pathway and restrained gastric cancer development.29 In addition, miR-1271 was also proved to play a tumor-suppressor role in HCC.30–32 Here, we manifested that overexpression of miR-1271 impeded proliferation and induced apoptosis in HBV-related HCC cells, which is in accord with the previous report.12
We next explored the regulatory mechanism of miR-1271 on HBV-related HCC, and SIRT1 was identified as the downstream target of miR-1271. Previous studies revealed that the role of SIRT in cancers seems to remain highly controversial. For example, SIRT1 was reported to inhibit metastasis by inhibiting miR-15b-5p expression in colorectal cancer.33 On the contrary, SIRT1 was proved to promote tumor development in breast cancer and endometrial cancer.34,35 Alves-Fernandes et al. suggested that the contradictory role of SIRT1 might due to the different cell type and SIRT1 localization.36 A previous study revealed that SIRT1 promoted HBV replication in hepatocytes.37 Consistent with this study, our study showed that SIRT1 was overexpressed in HBV-related HCC tissues and cells, and overexpression of SIRT1 expedited HBV replication and cell proliferation as well as suppressed apoptosis. In addition, SIRT1 overexpression reversed miR-1271-mediated effects on HBV replication, cell proliferation and apoptosis in HBV-HCC cells. These findings revealed miR-1271 inhibited HBV-related HCC development by sponging SIRT1. However, the impact of miR-1271 on migration and invasion in HBV-related HCC and the in vivo experiments still need further investigation.
5. Conclusion
In summary, our study detected that miR-1271 expression was decreased and SIRT1 level was elevated in HBV-related HCC tissues and cells. The targeted relationship between miR-1271 and SIRT1 was identified. Furthermore, we demonstrated that miR-1271 restrained HBV replication and cell proliferation and facilitated apoptosis in HBV-related HCC cells via targeting SIRT1. It might provide new light on the development of diagnosis and cure strategies in HBV-related HCC.
Abbreviations
HCC | Hepatocellular carcinoma |
HBV | Hepatitis B virus |
cccDNA | Covalently closed circular |
EMT | Epithelial–mesenchymal transition |
FBS | Fetal bovine serum |
GAPDH | Glyceraldehyde-3-phosphate dehydrogenase |
PVDF | Polyvinylidene fluoride |
RIP | RNA immunoprecipitation |
Funding
No funding was received.
Availability of data and materials
The analyzed data sets generated during the present study are available from the corresponding author on reasonable request.
Authors' contribution
Conceptualization and methodology: Fengmei Wang and Hongmin Lv; formal analysis and data curation: Huiling Xiang and Yi Liu; validation and investigation: Fei Tang and Ping Liu; writing – original draft preparation and writing – review and editing: Fei Tang, Fengmei Wang and Hongmin Lv; approval of final manuscript: all authors.
Ethics approval and consent to participate
The present study was approved by the ethical review committee of The Third Central Clinical College of Tianjin Medical University.
Patient consent for publication
Not applicable.
Conflicts of interest
The authors declare that they have no competing interests.
References
- L. A. Torre, F. Bray, R. L. Siegel, J. Ferlay, J. Lortet-Tieulent and A. Jemal, Ca-Cancer J. Clin., 2015, 65, 87–108 CrossRef PubMed.
- Y. Xie, Adv. Exp. Med. Biol., 2017, 1018, 11–21 CrossRef CAS PubMed.
- D. Cohen, Y. Adamovich, N. Reuven and Y. Shaul, Hepatology, 2010, 51, 1538–1546 CrossRef CAS PubMed.
- M. Levrero, T. Pollicino, J. Petersen, L. Belloni, G. Raimondo and M. Dandri, J. Hepatol., 2009, 51, 581–592 CrossRef CAS PubMed.
- C. M. Croce and G. A. Calin, Cell, 2005, 122, 6–7 CrossRef CAS PubMed.
- L. A. Macfarlane and P. R. Murphy, Curr. Genomics, 2010, 11, 537–561 CrossRef CAS PubMed.
- G. Wang, F. Dong, Z. Xu, S. Sharma, X. Hu, D. Chen, L. Zhang, J. Zhang and Q. Dong, BMC Cancer, 2017, 17, 805 CrossRef PubMed.
- W. Lou, J. Liu, B. Ding, D. Chen, L. Xu, J. Ding, D. Jiang, L. Zhou, S. Zheng and W. Fan, J. Transl. Med., 2019, 17, 7 CrossRef PubMed.
- G. Li, W. Zhang, L. Gong and X. Huang, Oncol. Res., 2019, 27, 449–458 CrossRef PubMed.
- W. Sun, J. Zhang and J. Chen, J. Int. Med. Res., 2018, 46, 4693–4704 CrossRef CAS PubMed.
- F. Yu, Z. Lu, B. Chen, P. Dong and J. Zheng, Diagn. Pathol., 2015, 10, 129 CrossRef PubMed.
- Y. Chen, Z. X. Zhao, F. Huang, X. W. Yuan, L. Deng and D. Tang, J. Cell. Physiol., 2019, 234, 3555–3569 CrossRef CAS PubMed.
- S. Michan and D. Sinclair, Biochem. J., 2007, 404, 1–13 CrossRef CAS PubMed.
- Y. Olmos, J. J. Brosens and E. W. Lam, Drug Resist. Updates, 2011, 14, 35–44 CrossRef CAS PubMed.
- H. N. Choi, J. S. Bae, U. Jamiyandorj, S. J. Noh, H. S. Park, K. Y. Jang, M. J. Chung, M. J. Kang, D. G. Lee and W. S. Moon, Oncol. Rep., 2011, 26, 503–510 CAS.
- J. Chen, B. Zhang, N. Wong, A. W. Lo, K. F. To, A. W. Chan, M. H. Ng, C. Y. Ho, S. H. Cheng, P. B. Lai, J. Yu, H. K. Ng, M. T. Ling, A. L. Huang and X. F. Cai, et al., Cancer Res., 2011, 71, 4138–4149 CrossRef CAS PubMed.
- H. C. Chen, Y. M. Jeng, R. H. Yuan, H. C. Hsu and Y. L. Chen, Ann. Surg. Oncol., 2012, 19, 2011–2019 CrossRef PubMed.
- C. Hao, P. X. Zhu, X. Yang, Z. P. Han, J. H. Jiang, C. Zong, X. G. Zhang, W. T. Liu, Q. D. Zhao, T. T. Fan, L. Zhang and L. X. Wei, BMC Cancer, 2014, 14, 978 CrossRef PubMed.
- J. J. Deng, K. E. Kong, W. W. Gao, H. V. Tang, V. Chaudhary, Y. Cheng, J. Zhou, C. P. Chan, D. K. Wong, M. F. Yuen and D. Y. Jin, Biochim. Biophys. Acta, Gene Regul. Mech., 2017, 1860, 491–501 CrossRef CAS PubMed.
- Z. Zhao, C. Li, H. Xi, Y. Gao and D. Xu, Mol. Med. Rep., 2015, 12, 5415–5422 CrossRef CAS PubMed.
- N. Cloonan, S. Wani, Q. Xu, J. Gu, K. Lea, S. Heater, C. Barbacioru, A. L. Steptoe, H. C. Martin, E. Nourbakhsh, K. Krishnan, B. Gardiner, X. Wang, K. Nones and J. A. Steen, et al., Genome Biol., 2011, 12, R126 CrossRef CAS PubMed.
- F. X. Bosch, J. Ribes, M. Diaz and R. Cleries, Gastroenterology, 2004, 127, S5–S16 CrossRef PubMed.
- J. M. Llovet, A. Burroughs and J. Bruix, Lancet, 2003, 362, 1907–1917 CrossRef.
- Y. Gao, J. Feng, G. Yang, S. Zhang, Y. Liu, Y. Bu, M. Sun, M. Zhao, F. Chen, W. Zhang, L. Ye and X. Zhang, Hepatology, 2017, 66, 1413–1429 CrossRef CAS PubMed.
- D. W. Trobaugh and W. B. Klimstra, Trends Mol. Med., 2017, 23, 80–93 CrossRef CAS PubMed.
- L. Qi, K. Wang, H. Chen, X. Liu, J. Lv, S. Hou, Y. Zhang and Y. Sun, Virology, 2019, 535, 162–170 CrossRef CAS PubMed.
- W. C. Chen, C. K. Wei and J. C. Lee, J. Viral Hepatitis, 2019, 26, 655–665 CrossRef CAS PubMed.
- Y. Chen, L. Wang and J. Zhou, J. Obstet. Gynaecol. Res., 2019, 45, 2243–2254 CrossRef CAS PubMed.
- B. Lim, H. J. Kim, H. Heo, N. Huh, S. J. Baek, J. H. Kim, D. H. Bae, E. H. Seo, S. I. Lee, K. S. Song, S. Y. Kim, Y. S. Kim and M. Kim, Cancer Med., 2018, 7, 3411–3424 CrossRef CAS PubMed.
- M. Maurel, S. Jalvy, Y. Ladeiro, C. Combe, L. Vachet, F. Sagliocco, P. Bioulac-Sage, V. Pitard, H. Jacquemin-Sablon, J. Zucman-Rossi, B. Laloo and C. F. Grosset, Hepatology, 2013, 57, 195–204 CrossRef CAS PubMed.
- A. Qin, J. Zhu, X. Liu, D. Zeng, M. Gu and C. Lv, Oncol. Lett., 2017, 14, 6783–6788 Search PubMed.
- C. Li, Y. Jiang, R. Miao, K. Qu, J. Zhang and C. Liu, Int. J. Oncol., 2018, 52, 536–546 CAS.
- L. N. Sun, Z. Zhi, L. Y. Chen, Q. Zhou, X. M. Li, W. J. Gan, S. Chen, M. Yang, Y. Liu, T. Shen, Y. Xu and J. M. Li, Cancer Lett., 2017, 409, 104–115 CrossRef CAS PubMed.
- S. Yarahmadi, Z. Abdolvahabi, Z. Hesari, M. Tavakoli-Yaraki, Z. Yousefi, P. Seiri, S. Hosseinkhani and M. Nourbakhsh, Gene, 2019, 711, 143939 CrossRef CAS PubMed.
- Y. Lv, S. Chen, J. Wu, R. Lin, L. Zhou, G. Chen, H. Chen and Y. Ke, Artif. Cells, Nanomed., Biotechnol., 2019, 47, 2083–2090 CrossRef CAS PubMed.
- D. K. Alves-Fernandes and M. G. Jasiulionis, Int. J. Mol. Sci., 2019, 20, 3153 CrossRef PubMed.
- J. H. Ren, Y. Tao, Z. Z. Zhang, W. X. Chen, X. F. Cai, K. Chen, B. C. Ko, C. L. Song, L. K. Ran, W. Y. Li, A. L. Huang and J. Chen, J. Virol., 2014, 88, 2442–2451 CrossRef PubMed.
|
This journal is © The Royal Society of Chemistry 2019 |
Click here to see how this site uses Cookies. View our privacy policy here.