DOI:
10.1039/C9RA07199G
(Paper)
RSC Adv., 2019,
9, 37630-37641
Construction of a phosphate-rich polyacrylonitrile fiber surface microenvironment for efficient purification of crystal violet wastewater†
Received
8th September 2019
, Accepted 11th November 2019
First published on 19th November 2019
Abstract
Wastewater purification using fibrous adsorbents has received much attention due to their high efficiency, low cost, and recyclability. In this work, phosphate modified polyacrylonitrile fiber (B-PANEAPF) was prepared and used to remove cationic dyes. The B-PANEAPF showed the best adsorption capacity for crystal violet (CV) when compared with rhodamine B, methyl green, Victoria blue B, methylene blue, and neutral red. The adsorption tests revealed that the fiber possessed high adsorption efficiency and achieved semi-saturated adsorption within 15 min. The maximum adsorption capacity of 354.46 mg g−1 as calculated by the Langmuir adsorption model was higher than many other adsorbents. Furthermore, the B-PANEAPF was used to remove 210 mL of CV in a continuous-flow process with a high removal efficiency over 90%. Besides, the phosphate functionalized fiber could easily decrease the concentration of CV to below 0.5 mg L−1 which is below the maximum effluent discharge standard of 15 mg L−1 prescribed in China. It could also be fully recovered and easily separated from the solution to achieve re-use 10 cycles. Moreover, the adsorption mechanism indicated that the adsorption process of the fiber for CV was mainly attributed to electrostatic interaction and hydrogen bonding. In conclusion, the results suggested that the B-PANEAPF characterized by its simplicity, efficiency, eco-friendliness, and reusability, could be a promising candidate for CV removal.
1. Introduction
Crystal violet (CV) is widely used as a coloring agent, chromotropic indicator, bacteriostatic agent as well as in food and cosmetics industries.1 To date, increasing industrial demand and unreasonable consumption of dyes have become a serious environmental problem threating both human health and ecosystems.2 For example, CV contains triphenylmethane with high chemical resistance that can result in kidney failure and vision injuries.3 Wastewater containing dyes is also a kind of visual pollution.4 Furthermore, cationic dyes are more toxic than anionic ones because they can easily interact with cell membrane surfaces and concentrate in the cytoplasm.5 Besides, it is known that about 12% of the dyes are lost during production and processing, and 20% of this enters the water environment,6 resulting in the waste of resources and environmental pollution. Hence, it is necessary to develop effective methods for the enrichment, purification, and recovery of CV in wastewater.
To purify the dyes contaminated water, many techniques such as chemical precipitation, reverse osmosis, membrane filtration, solvent extraction, photocatalytic degradation and adsorption method have been well attempted.7–11 Among which, adsorption method becomes one of the most effective methods.12 Especially, the introduction of functional groups on the surface of solid supports to prepare novel supported adsorbents has attracted widespread attention for its compelling advantages including low-cost, good stability, simple operation process, and high efficiency.13 Recently, various solid supports such as minerals like zeolite,8 silica,14 carbon materials,15 magnetic nanocomposites,16 biomass carriers,17 and polymers18 have been used to remove CV together with fruitful achievements. On the basis of practicality, however, the degree of modification for some supports like minerals is low affecting the removal efficiency. The cost of some nanomaterials is high, and the nanomaterials may also cause secondary pollution in water and soil environments with complex removal operation. These defects inevitably limit their practical applications. Therefore, new supports to fabricate functionalized materials with more excellent absorption ability have received major attention but challenges still remain.
Organic textile fibers are widely used in people's daily life. Nowadays, the preparation of new functionalized fibers through surface modification is becoming a hot topic in current research. Different functionalized polyacrylonitrile fiber (PANF), polypropylene fiber, cotton fiber etc. are used as emerging class of water pollutant scavenger with many advantages of being easily modifiable and separable, and excellent reusability and environmental friendly properties.19–22 Among which, PANF is one of the most promising fibers due to its low-cost, high strength and appropriate hydrophilicity etc. Chemical modification of PANF is simple, which can be modified by conversion of surface functional groups or photochemical grafting.23,24 Moreover, PANF is known for its merits for heavy metals' removal.25 Until recently, the application of functionalized PANFs to remove organic pollutants in water has gradually drawn people's attention.26–28 Our previous work also showed that PANF is an ideal support to prepare novel fiber catalysts29–32 and heavy ion colorimetric sensors.33 It is found that the modification of the fiber is a deep-seated with high-density modification to construct the specific fiber surface microenvironment constituted by fiber polymer block and modified functional molecules.29 More distinctively, the special microenvironment is proved to enrich organic compound impressively in aqueous solution.31,34 Inspired by these findings, the functionalized fiber may show better removal capacity for dye in water than traditional adsorption materials. It is worth mentioning that the use of PANF in organic dye removal has been very rare35,36 especially no functionalized PANF has been reported for the removal of CV. Hence, it is necessary to use new functionalized PANFs for the removal of CV.
Anionic groups such as carboxylic ion, sulfonate anion, and phenoxy anion have been proved to be effective ways for removing cations dyes.37–39 However, the purification of cationic dyes by phosphate has been rarely reported.40 Phosphorus compounds containing Brønsted acidic (P–OH) and Lewis basic (P
O) sites are powerful groups characterized by their stability, high efficiency, and eco-friendliness. Various phosphorus compounds functionalized SBA-15, carbon fibers, and nano diamond have been reported and made important progresses in catalytic, adsorption of metal ions, and electrochemistry.41–43 In this work, phosphate functionalized polyacrylonitrile fiber (B-PANEAPF) is prepared by chemical grafting via stable covalent bond. The anionic surface micro-environment is formed on the surface of the fibers which would interact well with cationic dyes. The B-PANEAPF was first used to remove cationic dyes. It is easy to modify the fiber with high density, which is beneficial to improve its adsorption performance. In addition, the simple preparation method, economical raw materials and excellent reusability and recyclability of the functionalized fiber make it have high potential in dye purification. The present study tested the adsorption performance of phosphate functionalized fiber for cationic dyes a (Fig. 1). And the adsorption behaviors such as temperature effect, time behavior, reusability and adsorption mechanism etc of the B-PANEAPF for CV were studied in details.
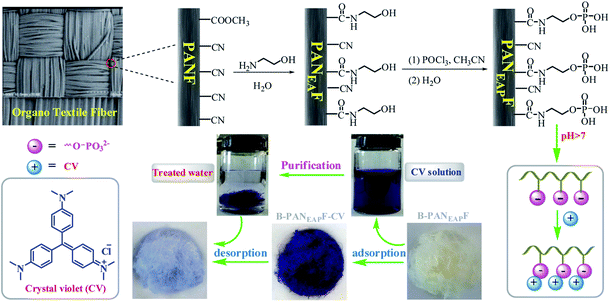 |
| Fig. 1 Phosphoric acid functionalized fiber PANEAPF as recyclable adsorbent for CV. | |
2. Materials and methods
2.1 Reagents and instruments
The details of reagents and instruments can be seen in the Section 1 of ESI.†
2.2 The synthesis of B-PANEAPF
The preparation of the functionalized fibers is as follows:
Step 1: the hydroxyethylation of PANF.
A mixture of 4.0 g PANF, 60 mL of H2O and 40 mL of 2-aminoethanol were placed in a three-necked flask (250 mL). The above reaction mixture was heated to reflux and reacted for 4 hours. After cooling to room temperature, the functionalized fiber was taken out and washed with warm H2O repeatedly. Then the prepared hydroxyethylation fiber (PANEAF) was put into the oven drying at 60 °C for 8 h.
Step 2: the phosphorylation of PANEAF.
A mixture of 1.0 g PANEAF, 20 mL of CH3CN and 2 mL of POCl3 were placed in a three-necked flask the above mixture was heated at 80 °C for 4 h. After cooling the mixture to room temperature, the fiber was taken out and put into ice water. After the fiber was stirred for one hour, it was filtered out again and washed with warm H2O repeatedly, then the fiber was dried at 60 °C over night to get phosphorylated fiber PANEAPF. Then the fiber PANEAPF was treated with 0.1 M NaOH, then it was washed with H2O and dried at 60 °C overnight to obtain the B-PANEAPF.
2.3 Dyes adsorption capacities of B-PANEAPF
First, 10 mg dried fiber was placed in 20 mL dye solution (1 × 10−3 mol L−1), the pH of the different dye solutions were adjusted to 5. Then the above solution was stirred using electromagnetic stirring for 4 h at room temperature. Then, the fiber was taken out with tweezers. In this work, the concentrations of dye after adsorption were measured by UV-vis spectrometer, before testing, the pH of dye solution was adjusted the same as that of standard curve, all adsorption tests were performed in triplicate.
2.4 Adsorption kinetics and isotherm experiments
The adsorption kinetics and isotherm experiments were carried out in 50 mL bottle with screw cap containing 20 mL CV solution (pH = 6) and 10 mg of B-PANEAPF. For the kinetics studies, B-PANEAPF was soaked in a 1 × 10−3 mol L−1 CV solution. After stirring for the desired time, the fiber was filtered out and the CV concentration in the remaining solution was measured by UV-vis spectrometer. In the adsorption isotherm tests, the initial CV concentrations were varied from 0.05 to 1 mmol L−1, the different CV solution was stirred for 3 h to guarantee the adsorption equilibrium had reached. Then the concentration of CV in each solution was measured by UV-vis spectrometer.
2.5 Desorption test
Dried fiber (100 mg) was immersed in 20 mL CV solution (0.2 mmol L−1, pH = 6). The solution was stirred for 1 h at room temperature. The fiber was taken out with tweezers and washed with deionized water. Then the CV adsorbed fiber was put into 50 mL 0.2 M HCl solution stirring for 2 h. After desorption, the fiber was taken out and washed with 0.1 M NaOH solution and deionized water, respectively. Then, it was used for the next circulate. The CV concentrations after adsorption (Ce) and desorption (Cd) were determined by UV. The desorption percentage D (%) was calculated from: D = (V2 × Cd)/[V1 × (C0 − Ce)] × 100%, and the removal efficiency was calculated from: R = [(C0 − Ce)/C0], where C0 is the initial concentration of CV, V1 and V2 are the volumes of adsorptive solution and eluent solution, respectively.
2.6 The adsorption of B-PANEAPF for CV under continuous-flow process
B-PANEAPF (300 mg) was filled into a silicone column with length of 100 mm and inside diameter of 5.6 mm. The flow rate of the continuous-flow device was adjusted to 1 mL min−1, then a CV solution (C0 = 0.2 mmol L−1, pH = 6) was pumped through the silicone column. The receptor liquid was collected in the flask and then the concentration of CV in the receptor fluid was determined by UV-vis spectrometer.
3. Results and discussion
3.1 The preparation and characterization of the fiber
3.1.1 The synthesis of phosphate functionalized fiber. Our previous work showed that the PANEAPF could be effectively modified onto the surface of PANF via a simple two-step strategy (Fig. 1). Furthermore the characterization technologies of elemental analysis, FTIR, XPS, solid-NMR, SEM, XRD, BET, and mechanical properties etc. were used to verify the success of grafting process.31 Based on previous research, phosphate functionalized fiber B-PANEAPF was easily prepared by alkalization treatment of PANEAPF. The degree of modification of the functionalized fibers were expressed by weight gain and phosphorus content, respectively. As shown in Table 1, the weight gain of the hydroxyethylated fiber PANEAF (Table 1, entry 2) is 15.7% based on PANF, and the OH content of the PANEAF is calculated as 2.34 mmol g−1 according to the weight gain. For the phosphorylated fiber PANEAPF, the weight gain easily reached to a high level of 18.6% (Table 1, entry 3), and the P content measured by ICP-OES and the acid content determined by acid–base titration were 1.72 mmol g−1 and 2.93 mmol g−1, respectively. It is obvious that the modification degree of the fiber is high (>1 mmol g−1), which will help to improve its adsorption capacity. The mechanical properties of the fibers were also tested and the results are showed in Table 1. The breaking strengths of PANEAPF and B-PANEAPF decreased slightly from 11.21 cN to 9.21 cN and 9.03 cN which all kept over 80.5% strength of the PANF. Overall, the results indicated that the phosphorylated fiber with high degree of modification and excellent strength is a potential adsorbent for water treatment.
Table 1 The weight gain of functionalized fibers and their P content, acid content, and mechanical properties
Entry |
Fiber |
Weight gaina (%) |
P contentb (mmol g−1) |
Acid contentc (mmol g−1) |
Mechanical properties |
Breaking strength |
Retention of breaking strengthd |
Weight gain = [(M2 − M1)/M1] × 100%, where M1 and M2 are the weights of original fiber and modified fiber. Determined by ICP-OES. Determined by acid–base titration. Based on PANF = 100%. |
1 |
PANF |
— |
0 |
0 |
11.21 cN |
100% |
2 |
PANEAF |
15.7 |
0 |
— |
9.94 cN |
88.6% |
3 |
PANEAPF |
18.6 |
1.72 |
2.93 |
9.21 cN |
82.2% |
4 |
B-PANEAPF |
— |
1.64 |
0 |
9.03 cN |
80.5% |
In addition, the specific surface area of the PANF and functionalized fibers are shown in Table S1†. The fibers contain nanometer sized pores with average pore diameter between 2.2 and 2.8 nm. The specific surface area of B-PANEAPF is 10.2 m2 g−1, the specific surface characteristics of B-PANEAPF may be beneficial to its adsorption capacity.
3.1.2 Surface topography. The SEM images of PANEAPF, B-PANEAPF, B-PANEAPF-CV (CV adsorbed fiber), B-PANEAPF-1 (one adsorption–desorption cycle), and B-PANEAPF-10 (the tenth adsorption–desorption cycle) are illustrated in Fig. 2. When the images are enlarged 200 times all of the fibers were continuous and showed good integrities, which indicates that the structures of the fibers were well maintained. After the SEM images were magnified to 2000 times, the surface of the PANEAPF was smooth and uniform (Fig. 2a). After treatment with dilute NaOH, the surface of the B-PANEAPF was not destroyed obviously, just slightly rougher than PANEAPF (Fig. 2b). In the SEM image of B-PANEAPF-CV (Fig. 2c), no obvious particles can be found through the high magnification (2000 times), and the phenomenon indicated that the CV dye was adsorbed onto the fiber by chemical interactions rather than simple physical attachment. After ten repetitions (Fig. 2e), the surface of the fiber had no significant changes compared with B-PANEAPF showing the excellent recycling ability of the phosphorylated fiber.
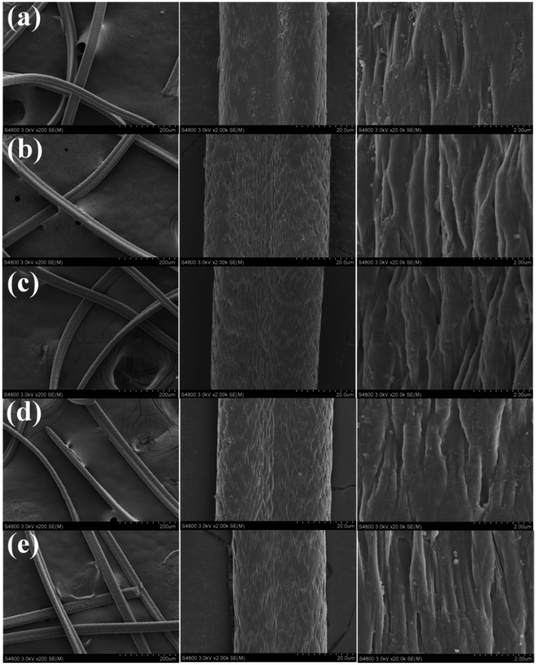 |
| Fig. 2 The SEM images of (a) PANEAPF, (b) B-PANEAPF, (c) B-PANEAPF-CV, (d) B-PANEAPF-1, (e) B-PANEAPF-10. | |
3.1.3 Composition and structure analysis. In this work, the composition and the structure analysis of the B-PANEAPF before and after use were illustrated by elemental analysis, FTIR, 13C solid-state NMR, solid UV-vis, and XRD. Firstly, the elemental analysis data of PANF, B-PANEAPF and B-PANEAPF-CV are presented in Table 2. The nitrogen (17.09%), carbon (50.87%) and hydrogen (5.41%) contents of the B-PANEAPF were all decreased due to the modification of new phosphorous-containing functional groups. After adsorption of CV, the carbon (55.59%) and hydrogen (6.19%) contents of the B-PANEAPF-CV increased and the nitrogen content (16.95%) decreased as expected. This can be the cause of higher carbon (73.5%) and hydrogen (7.4%) contents and lower nitrogen content (10.3%) in adsorbed CV molecule. Hence, it is speculated that the CV was adsorbed on the fiber adsorbent which can also be proved by the fiber photographs before and after adsorption as shown in Fig. S1.† The color change of the B-PANEAPF from light yellow to purplish blue further manifests the success of adsorption process.
Table 2 The elemental analysis data
Entry |
Sample |
C (%) |
H (%) |
N (%) |
1 |
PANF |
66.19 |
5.98 |
24.38 |
2 |
B-PANEAPF |
50.87 |
5.41 |
17.09 |
3 |
B-PANEAPF-CV |
55.59 |
6.19 |
16.95 |
The FTIR spectra of the fibers are shown in Fig. 3A and S2.† The FTIR spectra of PANF (Fig. 3A, trace a) showing the absorption peaks at 2242 cm−1 (C
N) and 1733 cm−1 (C
O), indicating the existence of the acrylonitrile and methyl acrylate units in the co-polymer of PANF. The wider absorption band of PANEAF (Fig. 3A, trace b) between 3600 and 3100 cm−1 is attributed to the stretching vibration of the O–H and N–H groups, and the C
O stretching vibration of PANEAF red shifts from 1733 to 1680 cm−1, which is also due to the formation of amide bonds (O
C–NH). After phosphate modification, the absorption peaks at 976, 1073, and 1172 cm−1 of the B-PANEAPF were corresponded to the stretching vibration of P–OH and PO2 (ref. 44) suggesting that the phosphate groups were successfully modified in the fiber surface (Fig. 3A, trace c). After adsorption of CV, a new absorption peak of B-PANEAPF-CV at 725 cm−1 was assigned to the out-of-plane bending vibration of phenyl moiety evidencing that the CV with aromatic structure was successfully adsorbed by B-PANEAPF (Fig. 3A, trace d). Furthermore, when the fiber was reused for 1 and 10 times, the spectra of B-PANEAPF-1 and B-PANEAPF-10 were almost the same as that of B-PANEAPF, indicating that the fiber had high stability and excellent reusability (Fig. 3A, traces e and f). The successful adsorption of CV can also be demonstrated by 13C solid-state NMR (Fig. 3B). The 13C solid-state NMR of PANF and B-PANEAPF (Fig. 3B, traces a and b) had similar signal peaks at 29.7, 122.1, and 176 ppm which can be assigned to the backbone carbon, ester carbonyl carbon, and cyano group carbon, respectively.45
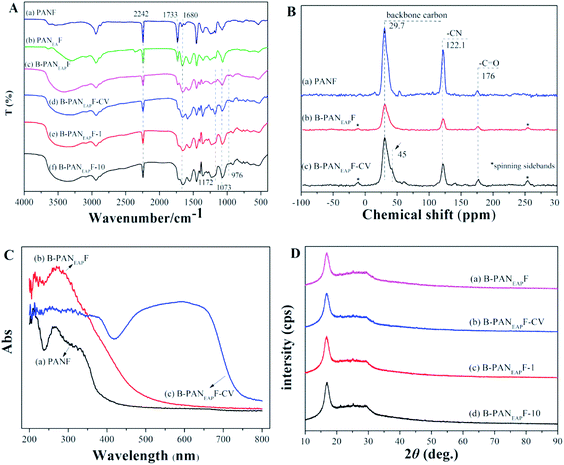 |
| Fig. 3 (A) The FTIR spectra of (c) B-PANEAPF, (d) B-PANEAPF-CV, (e) B-PANEAPF-1 and (f) B-PANEAPF-10; (B) the 13C solid-state NMR of the fibers; (C) the solid UV-vis spectra of the different fibers; (D) XRD patterns of the (a) B-PANEAPF, (b) B-PANEAPF-CV, (c) B-PANEAPF-1 and (d) B-PANEAPF-10. | |
The 13C solid-state NMR spectra of the fibers are shown in Fig. 3B. The spectra of the fibers show signals at 29.7, 122.1 and 176 ppm, which are ascribed to backbone carbon, cyano group (CN) and carbonyl (C
O), respectively. In the 13C solid-state NMR spectrum of B-PANEAPF-CV (Fig. 3B, trace c), the new signal peak from N(CH3)2 at 45 ppm appeared because the adsorbed CV contains N(CH3)2 groups46 in its chemical structure (Fig. 1). The result was further proved by the solid UV-vis (Fig. 3C). When the functionalized fiber B-PANEAPF-CV adsorbed CV, its spectrum showed a strong absorption band centered at 595 nm which is consistent with the maximum UV absorption wavelength of CV (Fig. S3†).47 X-ray powder diffraction (XRD) was used to illustrate the crystalline structure of the fibers (Fig. 3D). In the XRD spectrum of PANF, the sharp diffraction peak at 17° is the characteristic peaks for (100) crystallographic planes of PANF.48 It is obvious that the spectra of B-PANEAPF, B-PANEAPF-CV, B-PANEAPF-1, and B-PANEAPF-10 were rather similar to one of raw PANF (Fig. 3D, traces a–d). This finding suggests that the modification of the fiber mainly occurs in the surface layer of the fiber and it does not destroy the fiber structures. After repeated use, the fiber structure is still well maintained, which is consistent well with the results of SEM and FTIR, showing excellent stability of the functionalized fiber.
3.1.4 Thermal stability analysis. The thermal stability of the fiber within 800 °C were tested by thermogravimetric analysis in nitrogen atmosphere. As shown in Fig. 4, the weight loss before 100 °C is mainly caused by water evaporation. It's worth mentioning that there was no significant weight loss before 300 degrees, showing the high thermal stability of the functionalized fibers. The thermal decomposition of fibers were mainly between 300 to 500 °C, and the B-PANEAPF still showed high weight remaining of 40% at 800 °C, the result indicates that the application of phosphate functional fiber in practical water has high stability.
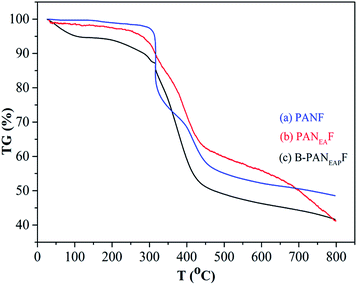 |
| Fig. 4 The thermogravimetric analysis of (a) PANF, (b) PANEAF, and (c) B-PANEAPF. | |
3.2 Comparison of adsorption capacities for different dyes by B-PANEAPF
The adsorption capacity of the fiber at equilibrium and a given time (qe and qt, mmol g−1) were utilized to evaluate the adsorption performance. The formulas are shown as follows: |
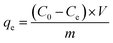 | (1) |
|
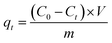 | (2) |
where C0, Ce and Ct (mmol L−1) are the concentrations at initial, equilibrium and a given time t (min), respectively, V is the solution volume (mL) and m is the mass of the fiber (g).
Various dyes such as methyl orange, rhodamine B, methyl green, Victoria blue B, methylene blue, neutral red, and crystal violet were used to test the adsorption capacities of the B-PANEAPF. To prevent precipitation, the pH of all dye solutions was adjusted to 5. The results are shown in Fig. 5, as expected, the B-PANEAPF had almost no affinity for anionic dyes such as methyl orange due to the strong charge repulsion. Nevertheless, the adsorption capacity of B-PANEAPF for cationic dyes significantly increased with the highest adsorption capacity of 0.75 mmol g−1 for CV. Furthermore, the B-PANEAPF had higher adsorption capacity for CV, neutral red, and methylene blue than that of rhodamine B, methyl green, and Victoria blue B (Fig. 5). This is probably due to the large steric hindrance of rhodamine B and Victoria blue B that can prevent their adsorption onto the fiber surface, and methyl green containing two positive centers which repel each other and lead to lower removal efficiency than that for CV. Furthermore, the adsorption capacity of neutral red is slightly higher than methylene blue due to the lower steric hindrance. Hence, the above mentioned results show that the B-PANEAPF is an excellent CV adsorbent. And the CV is selected as the research object to conduct the following researches.
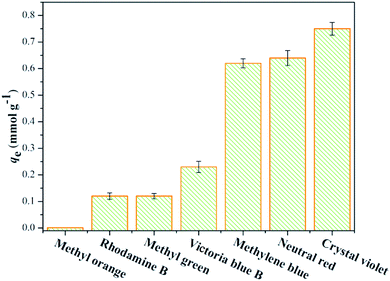 |
| Fig. 5 The adsorption capacities of the B-PANEAPF for different dyes. | |
3.3 Adsorption performance of the B-PANEAPF for CV
3.3.1 Effect of pH on adsorption capacity. It was reported that the solution pH not only affects the ionization degree of a dye, but also affects the surface charge of a adsorbent.6 Therefore, the CV adsorption by B-PANEAPF was studied in details within the pH ranged from 3 to 9 (Section 2 in ESI†). Zero charge (pHPZC) is reported at the pH in which the initial pH equals the final pH.41 As shown in Fig. 6a, the pHPZC of B-PANEAPF is equal to 7.8. At pH < pHPZC, the adsorption capacities of B-PANEAPF increase with the increase of pH values from 3 to 6. However, the adsorption capacities had no significant change when the pH was greater than 6 (Fig. 6b). This might be due to the occurrence of negative charges on the surface of fiber with increasing pH. And the positively charged CV dye showed a little competition with H+ at higher pH values. Hence, the interaction between anions and cations was more intense resulting in better B-PANEAPF removal of CV. In addition, the pKa values of the three nitrogen contained functional groups for CV are 3.29, 3.78 and 4.26, respectively.47 Hence, when the pH was above pHPZC, the surface of the fiber was completely deprotonated so that the further increase in pH had little effect on the adsorption of the fiber. This work suggests the pH 6 as the optimal pH value for future studies.
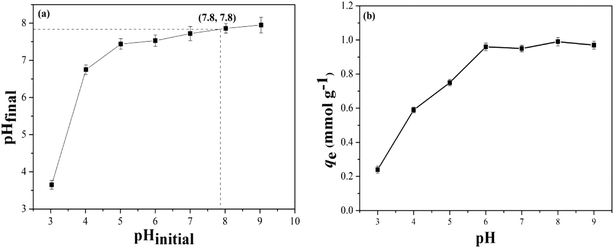 |
| Fig. 6 (a) Relationship between pH initial and pH final; (b) the effect of pH on the adsorption of CV by B-PANEAPF. | |
3.3.2 The determination of the adsorption limit of B-PANEAPF for CV. It is very important to reduce residual concentration of the CV solution by adsorbents to safety standard values in real applications.49 This work investigated the effect of adsorbent dosage for determination the limit of adsorption (Fig. S4†). The initial concentration was as high as 204 mg L−1. An adsorbent dosage of 0.5 g L−1, decreased the solution concentration to 30.6 mg L−1. Increasing the adsorbent dosage to 1 g L−1 decreased the residual concentrations of the solutions to below 2.3 mg L−1 with high removal efficiencies of above 98% which were within the wastewater discharge standards of 15 mg L−1 stipulated by China. This is likely due to an increase in adsorption dosage which, in turn, can enhance the specific surface area and the availability of adsorption sites on the fiber surface.50 Furthermore, the decrease in the concentration CV was not obvious with further increase of the dosage. It's worth mentioning that the concentration of the CV can be reduced to as low as to 0.5 mg L−1 in the range of this study showing a very low limit of adsorption. A lower dosage of 1 g L−1 was observed for fiber adsorbent compared to the biomass combustion residue.51 And the former had higher removal efficiency than the reported adsorbents. For example, sawdust and magnetic nanoparticles had lower maximum removal efficiency of 95.58% and 80.4%, respectively.52,53
3.3.3 Adsorption kinetics. Adsorption is a time determined process, the adsorption capacity increases gradually with time until the adsorption equilibrium is reached. The temporal behavior of B-PANEAPF for CV adsorption was studied and the results are shown in Fig. 7. As presented in Fig. 7a, the adsorption rate began very fast within the first 40 min, and then gradually slowed down as the contact time increased until the adsorption equilibrium was reached. The fiber took about 15 min to reach over the half saturated adsorption and 60 min to reach the equilibrium adsorption (0.91 mmol g−1). This is due to the initial existence of free adsorption sites on the B-PANEAPF which can interact with cationic dye CV. With the prolongation of adsorption time, the free adsorption sites of B-PANEAPF gradually decreased, leading to a lower adsorption rate until equilibrium. The result revealed that the B-PANEAPF can rapidly and efficiently remove CV from wastewater.
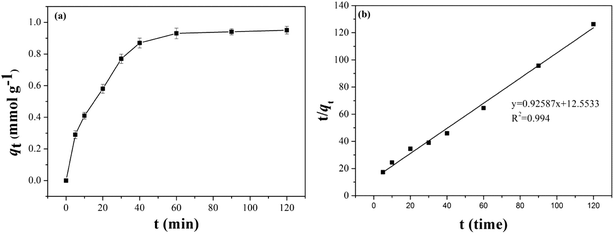 |
| Fig. 7 (a) Equilibrium adsorption capacity of B-PANEAPF at different contact time of CV, (b) pseudo second-order adsorption kinetic. | |
The adsorption process was analysed by different adsorption kinetic models. In this work, the pseudo first-order (3) and pseudo second-order (4) kinetic models were used to gain the rate constant and equilibrium adsorption of B-PANEAPF,54 the k1 (min−1) and k2 (g mmol−1 min−1) are the rate constants.
|
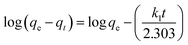 | (3) |
|
 | (4) |
Previous works have shown that if the adsorption process is more in line with the second-order kinetic model, it is more likely to be a chemical adsorption, while the first-order kinetic model is more inclined to physical adsorption.55 The fitting results are shown in Fig. 7b and S5,† the adsorption kinetics constants are summarized in Table S2.† The adsorption of CV by B-PANEAPF was more consistent with the pseudo second-order kinetics model (R2 = 0.994) rather than the first-order kinetics model (R2 = 0.976) indicating a chemical interaction between CV and phosphate anion rather a physical interaction.
3.3.4 Adsorption isotherm. The maximum adsorption capacity of adsorbents is affected by the initial concentration. Generally speaking, with the increase of initial concentration, the adsorption capacity of adsorbents increases until the maximum adsorption is achieved. As shown in Fig. 8a, the B-PANEAPF could adsorb most of the CV at a low concentration of CV. Nevertheless, there were many free active sites which could not bind CV resulting in a lower adsorption capacity. Due to the higher driving force for mass transfer, the adsorption capacity of B-PANEAPF increased along with increasing CV concentration. The maximum adsorption capacity could reach 0.95 mmol g−1 within the range of experimental concentrations which was higher than zeolite47 (0.30 mmol g−1), rice husk6 (0.10 mmol g−1), and chitosan56 (0.19 mmol g−1) etc. functionalized adsorbents.
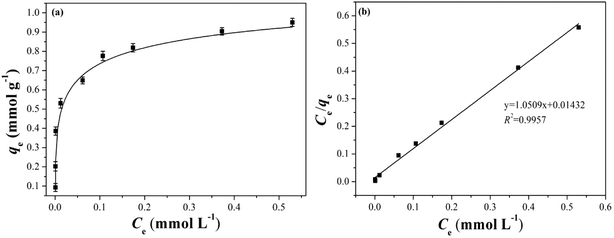 |
| Fig. 8 (a) Equilibrium adsorption capacity of B-PANEAPF under different initial concentration of CV, (b) Langmuir adsorption isotherm. | |
The adsorption process of B-PANEAPF for CV was further investigated by adsorption isotherm. The data of adsorption isotherm were analyzed by Langmuir57 (5) and Freundlich isotherm58 (6) models:
|
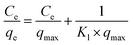 | (5) |
|
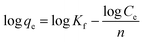 | (6) |
where
n is the heterogeneity factor,
Kl is the Langmuir adsorption constants (L mmol
−1) and
Kf is the Freundlich adsorption constant (mmol g
−1).
It was reported that Langmuir adsorption isotherm model is more suitable for single-layer adsorption, while Freundlich adsorption model is more inclined to multi-layer adsorption.59 The fitting results are shown in Fig. 8b and S6,† and the isotherm constants are summarized in Table S2.† The adsorption isotherm date fitted best with the Langmuir adsorption model when the R2 values were compared. The results showed that the removal of CV by B-PANEAPF tend to be monolayer adsorption process, it also indicated that the adsorption sites on B-PANEAPF were uniform and energetically identical.
3.3.5 Thermodynamic studies. The thermodynamic studies of B-PANEAPF for CV was studied at 283, 293, and 303 K. As shown in Fig. S7,† increasing trend in CV adsorption capacity with an increase in the temperature indicated the process to be endothermic. The temperature dependence of adsorption is associated with different thermodynamic parameters such as standard enthalpy (ΔH°, kJ mol−1), standard entropy (ΔS°, J mol−1 K−1), and Gibbs free energy (ΔG°, kJ mol−1) which can be calculated by the following equations:60 |
 | (7) |
|
ΔG° = −RT ln KC
| (8) |
|
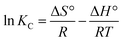 | (9) |
where KC is distribution coefficient, CAe is the amount of CV adsorbed on B-PANEAPF (mmol L−1), R is the gas constant (8.314 J mol−1 K−1), T is the temperature (K).As shown in Table S3,† the positive values of ΔG° and ΔS° indicate that the adsorption of CV on the B-PANEAPF is a non-spontaneous and an entropy-driven process. And the positive value of ΔH° (13.90 kJ mol−1) for CV adsorption by B-PANEAPF indicates the endothermic adsorption process, it also shows that chemisorption process is the rate controlling step.61 The activation energy (Ea) is the minimum kinetic energy required by the CV to react with the active sites on the B-PANEAPF, which can be obtained by the Arrhenius equation. In general, when the value of Ea is higher than 8 kJ mol−1, it can be attributed to a chemisorption.62 The Ea value calculated from the slope of the plot of ln
k2 vs.1/T (Fig. S8†) is 11.46 kJ mol−1, which is consistent with the proposed mechanism of chemisorption.
3.4 Possible adsorption mechanism of B-PANEAPF for CV
According to the above observations, the possible adsorption mechanisms of B-PANEAPF for CV removal were shown in Fig. S9.† It is known that the structure of the adsorbate and the surface properties of the fiber are the most important key points to understand the adsorption mechanism. For instance, CV can dissociate into CV+ and Cl− in aqueous solution.6 In the literature, the adsorption of cationic dyes by different adsorbents carries out by the electrostatic interaction and hydrogen bonding force.6,14,63 The fiber adsorbent B-PANEAPF has also similar adsorption forces in the adsorption process. When the pH value of CV solution is around the pHpzc of B-PANEAPF, the adsorption of CV by B-PANEAPF mainly relies on the strong electrostatic attraction due to the large amounts of anions on the surface of fiber. Additionally, numerous amide amide N–H bonds on the surface of fibers are good hydrogen-bonded donors which can interact with tertiary amine N of CV through the weak hydrogen bonds interaction. According to the kinetic characteristics of chemical adsorption discussed above, it can be concluded that the adsorption mechanism is mainly chemical interactions between anions and cations, and the interfractional hydrogen bonding force.
In order to further verify the adsorption mechanism of CV by B-PANEAPF, the X-ray photoelectron spectroscopy (XPS) of B-PANEAPF before and after the absorption of CV was tested. The results are shown in Fig. 9 and the corresponding atomic compositions are given in Table S4.† As shown in Fig. 9A, the B-PANEAPF contains C, N, O, P and Na, which indicates that the surface layer of the B-PANEAPF has sodium phosphate functional groups. Before adsorption of CV, the atomic percent of Na in B-PANEAPF is 1.5% (Table S4,† entry 1), while the content of sodium atom decreased obviously to 0.5% after adsorption of CV (Table S4,† entry 1), the result shows that the Na+ on the surface of B-PANEAPF reacts with the CV+ in the solution by ion exchange, proving the chemisorption mechanism of B-PANEAPF for CV.
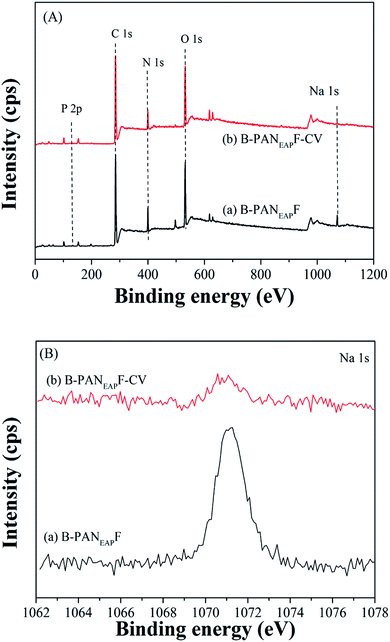 |
| Fig. 9 (A) XPS survey spectrum of (a) B-PANEAPF and (b) B-PANEAPF-CV, (B) high resolution Na 1s spectra of the fibers. | |
3.5 Desorption and reusability or the B-PANEAPF
Recycling ability is an important index for supported adsorbents. It was reported that the cationic dyes could be desorbed from the adsorbents using acid solution.35 Inorganic acids such as HCl have the apparent advantages of low-cost, high efficiency, and environment-friendly properties. Hence, it was selected as the eluent in this study. As shown in Fig. 10, the B-PANEAPF possessed high reusability of more than 10 times with high removal efficiency of above 99%. The high desorption percentage of over 95% indicates that most of the adsorbed CV could be desorbed from the fiber surface which also can be proved by the fiber pictures before and after adsorption (Fig. 1). The initial fiber B-PANEAPF was beige, after adsorption of CV the color of the fiber turned dark blue showing the successful adsorption process. It is worth mentioning that the color of the fiber was very close to the initial fiber after desorption. Furthermore, fiber was fully recovered by the tweezers possessing irreplaceable advantages over the powder or nanocarrier materials.64
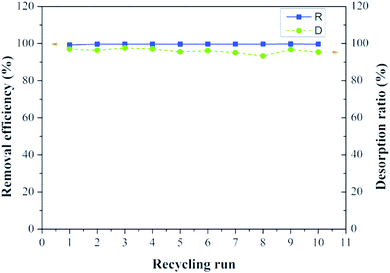 |
| Fig. 10 The recycling ability of B-PANEAPF for CV. | |
3.6 Use of PANEAPF in a flow process
Functionalized polyacrylonitrile fibers have the characteristic of soft, continuous and easy to knit, which is very suitable for continuous flow application in filled columns.29 Our previous work has shown the application potential and merits of the PANEAPF in catalytic organic reactions.31 For the purpose of practical application, the removal of CV by B-PANEAPF under continuous-flow conditions was studied (Fig. S10†). The breakthrough curve of B-PANEAPF for CV (0.2 mmol L−1) is illustrated in Fig. 11. The concentration of CV in the column effluent increased with the accumulation of effluent volume. When the volume of effluent does not exceed 210 mL, the removal efficiency of B-PANEAPF for CV was as higher as over 90%. The result show that the continuous flow device is simple and efficient for CV remediation practically. Therefore, the continuous flow device of fiber can effectively reduce the dye pollution of water body when it is placed at the sewage outfall.
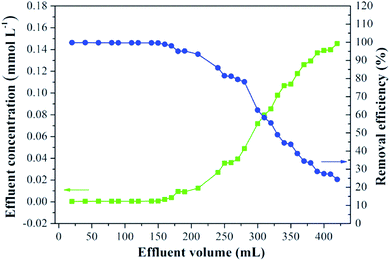 |
| Fig. 11 Breakthrough curves of B-PANEAPF for CV solution. | |
3.7 Comparison of the adsorption capacities for CV by various supported adsorbents
Finally, the removal ability for CV of recently used supported adsorbents was compared with the fiber adsorbent presented in this work (Table 3). Firstly, we compared the adsorption capacities of different adsorbents. It is noteworthy that the B-PANEAPF exhibited higher removal capacity (354.46 mg g−1) than many mineral, carbon materials, biomass, and metal oxides etc. modified adsorbents. Furthermore, the recyclability of B-PANEAPF is better than many other adsorbents. Overall, the many advantages of low-cost, excellent cyclicity and the removal of CV under continuous-flow conditions of the fiber is an attractive approach for practical application.
Table 3 The removal performances for CV by various supported adsorbents
Entry |
Adsorbent |
pH |
T (°C) |
Isotherm model |
qmax (mg g−1) |
Run |
Reference |
1 |
Multi-walled carbon nanotubes |
— |
— |
Langmuir isotherm |
100 |
— |
1 |
2 |
k-Carrageenan-g-poly (methacrylic acid) |
7 |
20 |
Langmuir isotherm |
28.24 |
— |
65 |
3 |
NaOH-modified rice husk |
— |
20 |
Langmuir isotherm |
44.87 |
— |
6 |
4 |
ZSM-5 zeolite |
7.5 |
45 |
Langmuir isotherm |
141.8 |
— |
47 |
5 |
Montmorillonite, K10 |
5.9 |
30 |
Langmuir isotherm |
400 |
— |
66 |
7 |
Modified magnetic nanoadsorbent |
6 |
— |
Langmuir isotherm |
166.67 |
— |
53 |
8 |
SiO2@MgO |
6.5 |
45 |
Langmuir isotherm |
2244.85 |
5 |
64 |
10 |
Chitosan hydrogel beads |
— |
30 |
Langmuir isotherm |
76.9 |
— |
56 |
11 |
CarAlg/MMt0.5 |
6.4 |
25 |
Langmuir isotherm |
88.8 |
— |
67 |
12 |
B-PANEAPF |
6 |
30 |
Langmuir isotherm |
354.46 |
10 |
This study |
4. Conclusion
In this study, we used polyacrylonitrile fiber, a nontoxic and inexpensive support, to synthesize a phosphate functionalized fiber adsorbent B-PANEAPF for the removal of CV. The B-PANEAPF showed higher selectivity and adsorption capacity (354.46 mg g−1) for CV over many other cationic dyes, viz. rhodamine B, methyl green, Victoria blue B, methylene blue, and neutral red. The adsorption performance tests showed that the residual concentrations of the CV solutions were below 2.3 mg mL−1 with high removal efficiency of 98% when the adsorption dosage of fiber was as low as 1 g L−1. The fiber could also easily decrease the CV below 0.5 mg L−1 which is substantially lower than 15 mg L−1 maximum effluent discharge standard in China. Furthermore, the B-PANEAPF exhibited high adsorption efficiency with semi-saturated adsorption of 15 min. The adsorption data fitted better with the pseudo second-order kinetic and Langmuir adsorption model indicating the monolayer chemisorption between CV and adsorption sites in the fiber surface. Moreover, the phosphate functionalized fiber can be applied in a continuous-flow system for CV adsorption with high removal efficiency of 90% when the effluent volume is less than 210 mL. In addition, the fiber can be easily recovered and separated from the solution showing excellent reusability for 10 times. The adsorption mechanisms revealed that the electrostatic interaction between cationic dye and cationic active sites and hydrogen bonding force of amide N–H played the key role in adsorption process. In summary, the B-PANEAPF characterized by its simplicity, efficiency, eco-friendliness, and reusability is an attractive adsorbent for CV removal in wastewater treatment.
Conflicts of interest
There are no conflicts to declare.
Acknowledgements
This work was financially supported by the National Natural Science Foundation of China (No. 21777111 and 21572156), the Natural Science Foundation of Anhui Province (No. 1908085QB87), and the Youth Science Fund of Anhui Agricultural University (No. 2018zd05).
References
- V. Sabna, S. G. Thampi and S. Chandrakaran, Ecotoxicol. Environ. Saf., 2016, 134, 390–397 CrossRef CAS PubMed.
- I. Ali, C. Peng, D. Lin and I. Naz, in Green Processing and Synthesis, 2019, vol. 8, p. 256 Search PubMed.
- A. Mittal, J. Mittal, A. Malviya, D. Kaur and V. K. Gupta, J. Colloid Interface Sci., 2010, 343, 463–473 CrossRef CAS PubMed.
- W. T. Tsai, C. Y. Chang, C. H. Ing and C. F. Chang, J. Colloid Interface Sci., 2004, 275, 72–78 CrossRef CAS PubMed.
- O. J. Hao, H. Kim and P.-C. Chiang, Crit. Rev. Environ. Sci. Technol., 2000, 30, 449–505 CrossRef CAS.
- S. Chakraborty, S. Chowdhury and P. Das Saha, Carbohydr. Polym., 2011, 86, 1533–1541 CrossRef.
- Y.-R. Jiang, H.-P. Lin, W.-H. Chung, Y.-M. Dai, W.-Y. Lin and C.-C. Chen, J. Hazard. Mater., 2015, 283, 787–805 CrossRef CAS PubMed.
- J. L. Marco-Brown, L. Guz, M. S. Olivelli, B. Schampera, R. M. Torres Sánchez, G. Curutchet and R. Candal, Chem. Eng. J., 2018, 333, 495–504 CrossRef CAS.
- M. G. Peleyeju and O. A. Arotiba, Environ. Sci.: Water Res. Technol., 2018, 4, 1389–1411 RSC.
- I. Ali, C. Peng, I. Naz, Z. M. Khan, M. Sultan, T. Islam and I. A. Abbasi, RSC Adv., 2017, 7, 40158–40178 RSC.
- I. Ali, C. Peng, I. Naz, D. Lin, D. P. Saroj and M. Ali, RSC Adv., 2019, 9, 3625–3646 RSC.
- A. Sharma, Z. Syed, U. Brighu, A. B. Gupta and C. Ram, J. Cleaner Prod., 2019, 220, 23–32 CrossRef CAS.
- S. Wong, H. H. Tumari, N. Ngadi, N. B. Mohamed, O. Hassan, R. Mat and N. A. Saidina Amin, J. Cleaner Prod., 2019, 206, 394–406 CrossRef CAS.
- Y. Xiong, J. Chen, M. Duan and S. Fang, Phys. Chem. Chem. Phys., 2018, 20, 19208–19220 RSC.
- S.-S. Yang, Y.-d. Chen, J.-H. Kang, T.-R. Xie, L. He, D.-F. Xing, N.-Q. Ren, S.-H. Ho and W.-M. Wu, J. Cleaner Prod., 2019, 227, 33–47 CrossRef CAS.
- I. Ali, C. Peng, Z. M. Khan, M. Sultan and I. Naz, Arabian J. Sci. Eng., 2018, 43, 6245–6259 CrossRef CAS.
- H. Ma, A. Kong, Y. Ji, B. He, Y. Song and J. Li, J. Cleaner Prod., 2019, 214, 89–94 CrossRef CAS.
- N. Ghaemi and P. Safari, J. Hazard. Mater., 2018, 358, 376–388 CrossRef CAS PubMed.
- H. Zhang, D. Fang, Z. Kong, J. Wei, X. Wu, S. Shen, W. Cui and Y. Zhu, Chem. Eng. J., 2018, 331, 406–415 CrossRef CAS.
- C. Liu, X. Liang, J. a. Liu, Y. Liu, J. Luo and H. Zhu, RSC Adv., 2016, 6, 38430–38436 RSC.
- O. Mapazi, K. P. Matabola, R. M. Moutloali and C. J. Ngila, Polymer, 2018, 149, 106–116 CrossRef CAS.
- R. Yang, Y. Su, K. B. Aubrecht, X. Wang, H. Ma, R. B. Grubbs, B. S. Hsiao and B. Chu, Polymer, 2015, 60, 9–17 CrossRef CAS.
- S. Deng, G. Zhang and P. Wang, Environ. Sci.: Water Res. Technol., 2018, 4, 487–492 RSC.
- X.-L. Shi, B. Sun, Y. Chen, Q. Hu, P. Li, Y. Meng and P. Duan, J. Catal., 2019, 372, 321–329 CrossRef CAS.
- R. Zhao, Y. Li, X. Li, Y. Li, B. Sun, S. Chao and C. Wang, J. Colloid Interface Sci., 2018, 514, 675–685 CrossRef CAS PubMed.
- P. Meng, S. Deng, B. Wang, J. Huang, Y. Wang and G. Yu, Chem. Eng. J., 2017, 315, 108–114 CrossRef CAS.
- R. Xu, J. Cui, R. Tang, F. Li and B. Zhang, Chem. Eng. J., 2017, 326, 647–655 CrossRef CAS.
- R. Zhao, Y. Wang, X. Li, B. Sun, Y. Li, H. Ji, J. Qiu and C. Wang, ACS Sustainable Chem. Eng., 2016, 4, 2584–2592 CrossRef CAS.
- J. Du, G. Xu, H. Lin, G. Wang, M. Tao and W. Zhang, Green Chem., 2016, 18, 2726–2735 RSC.
- J. Cao, P. Li, G. Xu, M. Tao, N. Ma and W. Zhang, Chem. Eng. J., 2018, 349, 456–465 CrossRef CAS.
- G. Xu, J. Cao, Y. Zhao, L. Zheng, M. Tao and W. Zhang, Chem.–Asian J., 2017, 12, 2565–2575 CrossRef CAS PubMed.
- G. Xu, M. Jin, Y. K. Kalkhajeh, L. Wang, M. Tao and W. Zhang, J. Cleaner Prod., 2019, 231, 77–86 CrossRef CAS.
- R. Gao, G. Xu, L. Zheng, Y. Xie, M. Tao and W. Zhang, J. Mater. Chem. C, 2016, 4, 5996–6006 RSC.
- G. Xu, L. Wang, M. Li, M. Tao and W. Zhang, Green Chem., 2017, 19, 5818–5830 RSC.
- Y. Fan, H.-J. Liu, Y. Zhang and Y. Chen, J. Hazard. Mater., 2015, 283, 321–328 CrossRef CAS PubMed.
- A. Almasian, M. E. Olya and N. M. Mahmoodi, J. Taiwan Inst. Chem. Eng., 2015, 49, 119–128 CrossRef CAS.
- Y. Zhang, Y. Ye, X. Zhou, Z. Liu, G. Zhu, D. Li and X. Li, J. Mater. Chem. A, 2016, 4, 838–846 RSC.
- Z. Wang, J. Guo, J. Ma and L. Shao, J. Mater. Chem. A, 2015, 3, 19960–19968 RSC.
- D. Shetty, I. Jahovic, J. Raya, F. Ravaux, M. Jouiad, J.-C. Olsen and A. Trabolsi, J. Mater. Chem. A, 2017, 5, 62–66 RSC.
- I. Ali, C. Peng, T. Ye and I. Naz, RSC Adv., 2018, 8, 8878–8897 RSC.
- R. Zhao, X. Li, B. Sun, M. Shen, X. Tan, Y. Ding, Z. Jiang and C. Wang, Chem. Eng. J., 2015, 268, 290–299 CrossRef CAS.
- Q. Gao, J.-F. Xie, Y.-T. Shao, C. Chen, B. Han, K.-S. Xia and C.-G. Zhou, Chem. Eng. J., 2017, 313, 197–206 CrossRef CAS.
- F. J. García-Mateos, R. Berenguer, M. J. Valero-Romero, J. Rodríguez-Mirasol and T. Cordero, J. Mater. Chem. A, 2018, 6, 1219–1233 RSC.
- T. Y. Ma and S. Z. Qiao, ACS Catal., 2014, 4, 3847–3855 CrossRef CAS.
- Y. H. Choi, C. M. Choi, D. H. Choi, Y. Paik, B. J. Park, Y. K. Joo and N. J. Kim, J. Membr. Sci., 2011, 371, 84–89 CrossRef CAS.
- J. Zhang, Y. Wang, N. Luo, Z. Chen, K. Wu and G. Yin, Dalton Trans., 2015, 44, 9847–9859 RSC.
- G. V. Brião, S. L. Jahn, E. L. Foletto and G. L. Dotto, J. Colloid Interface Sci., 2017, 508, 313–322 CrossRef PubMed.
- E. Zussman, X. Chen, W. Ding, L. Calabri, D. A. Dikin, J. P. Quintana and R. S. Ruoff, Carbon, 2005, 43, 2175–2185 CrossRef CAS.
- B. C. S. Ferreira, F. S. Teodoro, A. B. Mageste, L. F. Gil, R. P. de Freitas and L. V. A. Gurgel, Ind. Crops Prod., 2015, 65, 521–534 CrossRef CAS.
- W. Hamza, N. Dammak, H. B. Hadjltaief, M. Eloussaief and M. Benzina, Ecotoxicol. Environ. Saf., 2018, 163, 365–371 CrossRef CAS PubMed.
- S. K. Ghosh and A. Bandyopadhyay, J. Environ. Chem. Eng., 2017, 5, 2415–2430 CrossRef CAS.
- S. Shakoor and A. Nasar, Groundwater for Sustainable Development, 2018, 7, 30–38 CrossRef.
- C. Muthukumaran, V. M. Sivakumar and M. Thirumarimurugan, J. Taiwan Inst. Chem. Eng., 2016, 63, 354–362 CrossRef CAS.
- Y. S. Ho and G. McKay, Process Biochem., 1999, 34, 451–465 CrossRef CAS.
- L. Bai, H. Hu, W. Zhang, J. Fu, Z. Lu, M. Liu, H. Jiang, L. Zhang, Q. Chen and P. Tan, J. Mater. Chem., 2012, 22, 17293–17301 RSC.
- A. Pal, S. Pan and S. Saha, Chem. Eng. J., 2013, 217, 426–434 CrossRef CAS.
- I. Langmuir, J. Am. Chem. Soc., 1918, 40, 1361–1402 CrossRef CAS.
- H. Freundlich, Z. Phys. Chem., Stoechiom. Verwandtschaftsl., 1906, 57, 385–470 CAS.
- N. Uenlue and M. Ersoz, Sep. Purif. Technol., 2007, 52, 461–469 CrossRef CAS.
- A. K. Singha Deb, V. Dwivedi, K. Dasgupta, S. Musharaf Ali and K. T. Shenoy, Chem. Eng. J., 2017, 313, 899–911 CrossRef CAS.
- C. Xiong, Y. Li, G. Wang, L. Fang, S. Zhou, C. Yao, Q. Chen, X. Zheng, D. Qi, Y. Fu and Y. Zhu, Chem. Eng. J., 2015, 259, 257–265 CrossRef CAS.
- K. Z. Elwakeel and E. Guibal, Chem. Eng. J., 2015, 281, 345–359 CrossRef CAS.
- A. Masoumi, K. Hemmati and M. Ghaemy, Chemosphere, 2016, 146, 253–262 CrossRef CAS PubMed.
- Y. Pei, M. Wang, D. Tian, X. Xu and L. Yuan, J. Colloid Interface Sci., 2015, 453, 194–201 CrossRef CAS PubMed.
- M. Gholami, M. T. Vardini and G. R. Mahdavinia, Carbohydr. Polym., 2016, 136, 772–781 CrossRef CAS PubMed.
- G. K. Sarma, S. Sen Gupta and K. G. Bhattacharyya, J. Environ. Manage., 2016, 171, 1–10 CrossRef CAS PubMed.
- G. R. Mahdavinia, H. Aghaie, H. Sheykhloie, M. T. Vardini and H. Etemadi, Carbohydrate Polymers, 2013, 98, 358–365 CrossRef CAS PubMed.
Footnote |
† Electronic supplementary information (ESI) available. See DOI: 10.1039/c9ra07199g |
|
This journal is © The Royal Society of Chemistry 2019 |