DOI:
10.1039/C9RA06120G
(Paper)
RSC Adv., 2019,
9, 29721-29725
Oxidative cyanation of N-aryltetrahydroisoquinoline induced by visible light for the synthesis of α-aminonitrile using potassium thiocyanate as a “CN” agent†
Received
6th August 2019
, Accepted 12th September 2019
First published on 19th September 2019
Abstract
A novel method for the synthesis of α-aminonitrile through visible-light-induced oxidative cyanation of N-aryltetrahydroisoquinoline with potassium thiocyanate has been developed. The process does not require the use of a photocatalyst, transition metal reagent, strong oxidizing agent, or toxic cyano-containing compound, which makes the reaction simple and green.
α-Aminonitriles are versatile intermediates which can be readily converted into a range of multifunctional organics,1 such as α-aminocarbonyl compounds, α-amino acids, 1,2-diamines and many others. Moreover, many nitrile-containing compounds have been widely used as drugs for treating various diseases.2 For example, amphetaminil3 is a stimulant that can be employed to treat obesity and narcolepsy; others like Saxagliptin,4 NVP-DPP728 (ref. 5) and Vildagliptin6 are potent reversible inhibitors of dipeptidyl peptidase-4 (DPP-4), and can be applied as novel antidiabetic drugs. Therefore, the development of novel, efficient and clean methods for the synthesis of α-aminonitriles has attracted wide attention.
Traditionally, α-aminonitriles are synthesized through the Strecker reaction.7 Another momentous strategy for synthesis of α-aminonitriles is oxidative cyanation of tertiary amines with the involvement of transition metal reagents (such as Ru,8 Au,9 Cu,10 Fe,11 Co,12 V,13 Mo,14 etc.) and non-metallic reagents (such as PhI(OAc)2,15 tropylium salt,16 AIBN,17 TBAI,18 AcOH,19 PIFA,20 TBHP,21 thiourea,22 DDQ,23 TBPB,24a etc.). Among the reported cyanidation of tertiary amines, the cyano sources mainly includes (i) metal-cyanides such as NaCN,8a–8c,10c KCN,16 K3[Fe(CN)6],25 KSCN,21 etc., (ii) organic cyanides such as trimethylcyanosilane,9a,10e,11a–11c,11f malononitrile,10a,15,23 ethyl cyanoformate,11d,8e,11d,12 benzoyl cyanide,11e phenylacetonitrile,18a cyanoacetic acid,18b tetrabutylammonium cyanide,19 cyanobenziodoxolones,24 AIBN,26 etc., and (iii) combined cyano source such as the combination of 1,2-dichloroethane and trimethylsilyl azide.10b (Scheme 1). However, most of these methods require transition metal reagents, strong oxidizing agents, and cyano sources that are highly toxic and difficult to obtain. Therefore, it is necessary to find a cyanide source that is easily available and of low toxicity for the cyanidation of tertiary amines.
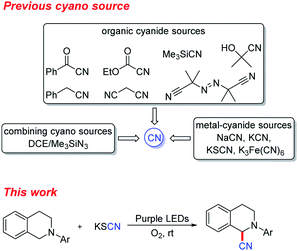 |
| Scheme 1 Cyano sources for cyanidation reactions of tertiary amine. | |
In organic synthesis, thiocyanate has received extensive attention due to its low toxicity, low cost and easy availability.27 Using the thiocyano group of thiocyanate, thiocyanation of organic molecules can be achieved by nucleophilic substitution or through a free radical pathway.28 More importantly, thiocyanate can be utilized as a clean and safe source of cyano group.21,29 However, these methods have the following disadvantages: using a transition metal catalyst, or a strong oxidizing agent, or heating. The utilization of light energy in organic synthesis is highly commendable because it is sustainable, clean, environmentally benign, as well as widely and easily available. In recent years, great progresses have been made in the formation of carbon–carbon bond that is induced by visible light,30 among which the oxidative cyanation of tertiary amine is a good example.31 However, to the best of our awareness, the cyanidation of tertiary amine with thiocyanate induced by visible light has not been documented yet. Herein, we report the visible-light-induced oxidative cyanation reaction of tertiary amines with potassium thiocyanate.
We began our investigation with the use of N-phenyltetrahydroisoquinoline (1a) and potassium thiocyanate (2a) as the model reaction (Table 1). First, the reaction was carried out in absolute ethanol illuminated by the compact fluorescent lamps (CFL) of 30 W at room temperature under air atmosphere. Fortunately, the desired product 2-phenyl-1,2,3,4-tetrahydroisoquinoline-1-carbonitrile (3a) was obtained in 50% yield after 24 h (entry 1). Then, we explored the effects of oxidants such as O2, TBHP, H2O2, K2S2O8, DDQ, and PhI(OAc)2 on the reaction (entries 2–7). Among them, O2 showed the best result (entry 2). The light source also had an important influence on this reaction. When the compact fluorescent lamps was replaced by a red LEDs, the yield of the reaction significantly reduced from 55% to 6% (entry 8). When green LEDs was chosen as the light source, the yield of the reaction was increased to 68% (entry 9). Besides, we investigated the effect of other visible light sources on the reaction, and purple LEDs was proved to be the best choice, up to 83% yield (entries 10 and 11). Based on the above experimental results, it is deduced that the shorter the visible-light wavelength, the higher is the reaction efficiency. In addition, we investigated the effect of solvents such as CH3OH, DMSO, PhMe, and CH3CN on the reaction (entries 12–15). Among them, CH3CN was the most suitable, giving 3a in 92% yield. When KSCN was replaced with NH4SCN, the yield of the corresponding product was 71% (entry 16). It is worth noting that the reaction did not proceed in the absence of either light or oxidant (entries 17 and 18).
Table 1 Optimization of cyanidation of N-phenyltetrahydroisoquinoline (1a) with potassium thiocyanate (2a)a
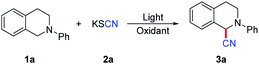
|
Entry |
Oxidant |
Light |
Solvent |
Yieldb (%) |
Reaction conditions: 1a (0.2 mmol), 2a (0.1 mmol), oxidant (1.5 equiv.), solvent (1.5 mL), rt, 24 h. Isolated yields based on 2a. NH4SCN was used instead of KSCN. |
1 |
Air |
30 W CFL |
C2H5OH |
50 |
2 |
O2 |
30 W CFL |
C2H5OH |
55 |
3 |
TBHP |
30 W CFL |
C2H5OH |
25 |
4 |
H2O2 |
30 W CFL |
C2H5OH |
17 |
5 |
K2S2O8 |
30 W CFL |
C2H5OH |
19 |
6 |
DDQ |
30 W CFL |
C2H5OH |
3 |
7 |
PhI(OAc)2 |
30 W CFL |
C2H5OH |
5 |
8 |
O2 |
30 W red LEDs |
C2H5OH |
6 |
9 |
O2 |
30 W green LEDs |
C2H5OH |
68 |
10 |
O2 |
30 W blue LEDs |
C2H5OH |
74 |
11 |
O2 |
30 W purple LEDs |
C2H5OH |
83 |
12 |
O2 |
30 W purple LEDs |
CH3OH |
11 |
13 |
O2 |
30 W purple LEDs |
DMSO |
8 |
14 |
O2 |
30 W purple LEDs |
PhMe |
11 |
15 |
O2 |
30 W purple LEDs |
CH3CN |
92 |
16c |
O2 |
30 W purple LEDs |
CH3CN |
71 |
17 |
— |
30 W purple LEDs |
C2H5OH |
0 |
18 |
O2 |
— |
C2H5OH |
0 |
With the optimized conditions, we explored the substrate scope of this visible-light-induced cyanation reaction between N-aryltetrahydroisoquinoline (1) and potassium thiocyanate (2a) (Scheme 2). The N-aryltetrahydroisoquinolines with either an electron-withdrawing or electron-donating group reacted well with potassium thiocyanate to afford the desired products in moderate to good yields. Specifically, when the benzene ring attached to the nitrogen atom had an electron donating group (Me, Et, and Ph) at the para position, the desired products were in 79%, 86% and 62% yields, respectively (3b–d). In the cases of having an electron-withdrawing group (F, Cl, Br, CN, CF3, and OCF3) attached to the benzene ring at para position, the desired products were obtained in moderate to good yields, and the highest one was up to 88% (3e–j). Besides, ortho- and meta-substituted N-aryltetrahydroisoquinolines also exhibited high reactivity, and the target products were obtained in moderate yields (3k–n).
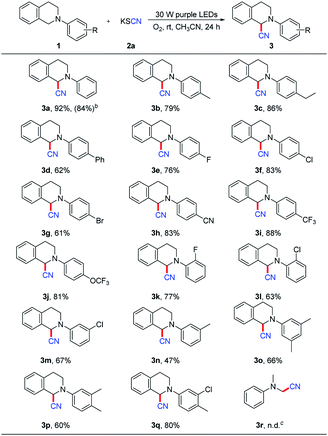 |
| Scheme 2 Substrate scope for cyanation of N-aryltetrahydroisoquinolines (1) with potassium thiocyanate (2a)a. aConditions: 1 (0.2 mmol), 2a (0.1 mmol), CH3CN (1.5 mL), 24 h, isolated yields of column chromatography based on 2a. bThe yield was based on 1 mmol of KSCN. cNo product was detected by GC-MS. | |
What's more, the substrates with two identical or different substituents on the phenyl ring were also suitable for this reaction, and the yields of the products vary from 60% to 80% (3o–q). Regrettably, dialkyl substituted aromatic amine (such as N,N-dimethylaniline) was not suitable for this reaction (3r).
To gain reasonable insight into the reaction mechanism, we conducted the following control experiments (Scheme 3). When 2,2,6,6-tetramethyl-1-piperidinyloxy (TEMPO) and 2,6-di-tert-butyl-4-methylphenol (BHT), both are radical quenchers, was added to the reaction under the standard conditions, the product 3a was obtained in 3% and 9% yield, respectively, detected by GC. The results reveal that the reaction may proceed with the participation of free radicals.
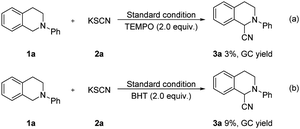 |
| Scheme 3 Control experiments. | |
Based on our experimental results and those of previous reports,19,21,32,33 a mechanism for this visible-light-induced oxidative cyanation reaction is proposed as illustrated in Scheme 4. Initially, substrate 1 is transformed into cationic radical I in the presence of visible light and oxygen, and anionic superoxide radical O2˙− is generated simultaneously. Then, cationic radical I reacts with O2˙− to generate radical II which loses an electron to form intermediate V. Under the action of HOO−, thiocyanate is converted to cyano anion which then reacts with intermediate V to generate the desired product 3. At the same time, the intermediate II is combined with HOO˙ to form III, and the latter III is dehydrated to form a by-product IV.
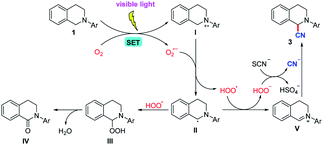 |
| Scheme 4 Possible mechanism. | |
Conclusion
In summary, we have developed a novel method for the synthesis of α-aminonitrile using N-aryltetrahydroisoquinoline and potassium thiocyanate as raw materials. The product α-aminonitriles can be obtained in moderate to good yields under the illumination of 30 W purple LEDs at room temperature for 24 h in the oxygen atmosphere. The process does not involve any photocatalysts, transition metal reagents, strong oxidizing agents, and cyano-containing compounds that are highly toxic and difficult to obtain. This reaction provides a simple, green, and efficient method for the synthesis of α-aminonitriles. Further studies on reaction mechanism and synthetic applications are currently underway.
Conflicts of interest
There are no conflicts to declare.
Acknowledgements
This work was supported by the National Natural Science Foundation of China (No. 21772035), and the Provincial Natural Science Foundation of Hunan (No. 2019JJ50104).
Notes and references
-
(a) P. Anbarasan, T. Schareina and M. Beller, Chem. Soc. Rev., 2011, 40, 5049 RSC;
(b) Y.-Y. Ping, Q.-P. Ding and Y.-Y. Peng, ACS Catal., 2016, 6, 5989 CrossRef CAS;
(c) D. Enders and J. P. Shilvock, Chem. Soc. Rev., 2000, 29, 359 RSC;
(d) N. Otto and T. Opatz, Chem.–Eur. J., 2014, 20, 13064 CrossRef CAS PubMed;
(e) L. K. Kinthada, S. R. Kinthada, A. Kinthada, K. N. Kinthada and A. Kinthada, J. Org. Chem., 2017, 82, 8548 CrossRef CAS PubMed;
(f) L. K. Kinthada, S. Ghosh, K. N. Babu, M. Sharique, S. Biswas and A. Kinthada, Org. Biomol. Chem., 2014, 12, 8152 RSC;
(g) L. K. Kinthada, S. Ghosh, S. De, S. Bhunia, D. Dey and A. Kinthada, Org. Biomol. Chem., 2013, 11, 6984 RSC.
-
(a) F. F. Fleming, L. Yao, P. C. Ravikumar, L. Funk and B. C. Shook, J. Med. Chem., 2010, 53, 7902 CrossRef CAS PubMed;
(b) B. Kuhn, M. Hennig and P. Mattei, Curr. Top. Med. Chem., 2007, 7, 609 CrossRef CAS PubMed;
(c) L. Qiao, C. A. Baumann, C. S. Crysler, N. S. Ninan, M. C. Abad, J. C. Spurlino, R. L. DesJarlais, J. Kervinen, M. P. Neeper, S. S. Bayoumy, R. Williams, I. C. Deckman, M. Dasgupta, R. L. Reed, N. D. Huebert, B. E. Tomczuk and K. J. Moriarty, Bioorg. Med. Chem. Lett., 2006, 16, 123 CrossRef CAS.
-
(a) J. J. Klosa, Prakt. Chem., 1963, 20, 283 CrossRef CAS;
(b) P. M. Beardsley, R. L. Balster and L. S. Harris, Drug Alcohol Depend., 1986, 18, 149 CrossRef CAS PubMed.
-
(a) S. A. Savage, G. S. Jones, S. Kolotuchin, S. A. Ramrattan, T. Vu and R. E. Waltermire, Org. Process Res. Dev., 2009, 13, 1169 CrossRef CAS;
(b) G. S. Jones, S. A. Savage, S. Ivy, P. L. Benitez and A. J. Ramirez, J. Org. Chem., 2011, 76, 10332 CrossRef CAS PubMed;
(c) D. J. Augeri, J. A. Robl, D. A. Betebenner, D. R. Magnin, A. Khanna, J. G. Robertson, A. Wang, L. M. Simpkins, P. Taunk, Q. Huang, S.-P. Han, B. Abboa-Offei, M. Cap, L. Xin, L. Tao, E. Tozzo, G. E. Welzel, D. M. Egan, J. Marcinkeviciene, S. Y. Chang, S. A. Biller, M. S. Kirby, R. A. Parker and L. G. Hamann, J. Med. Chem., 2005, 48, 5025 CrossRef CAS PubMed.
- T. E. Hughes, M. D. Mone, M. E. Russell, S. C. Weldon and E. B. Villhauer, Biochemistry, 1999, 38, 11597 CrossRef CAS PubMed.
- B. Ahren, M. Landin-Olsson, P. A. Jansson, M. Svensson, D. Holmes and A. Schweizer, J. Clin. Endocrinol. Metab., 2004, 89, 2078 CrossRef CAS PubMed.
-
(a) H. Groeger, Chem. Rev., 2003, 103, 2795 CrossRef CAS PubMed;
(b) G. K. Friestad and A. K. Mathies, Tetrahedron, 2007, 63, 2541 CrossRef CAS;
(c) S. C. Pan and B. List, Chem.–Asian J., 2008, 3, 430 CrossRef CAS PubMed;
(d) P. Merino, E. Marques-Lopez, T. Tejero and R. P. Herrera, Tetrahedron, 2009, 65, 1219 CrossRef CAS;
(e) J. Martens, ChemCatChem, 2010, 2, 379 CrossRef CAS;
(f) J. Wang, X. Liu and X. Feng, Chem. Rev., 2011, 111, 6947 CrossRef CAS PubMed.
-
(a) H. Terai, N. Komiya and T. Nakae, J. Am. Chem. Soc., 2003, 125, 15312 CrossRef;
(b) S. I. Murahashi, N. Komiya and H. Terai, Angew. Chem., Int. Ed., 2005, 44, 6931 CrossRef CAS PubMed;
(c) S. Murahashi, T. Nakae, H. Terai and N. Komiya, J. Am. Chem. Soc., 2008, 130, 11005 CrossRef CAS PubMed;
(d) S. Verma, S. L. Jain and B. Sain, Catal. Lett., 2011, 141, 882 CrossRef CAS;
(e) K. Harsha, V. Reddy, G. Satish, V. P. Reddy, B. S. P. A. Kumara and Y. V. D. Nageswar, RSC Adv., 2012, 2, 11084 RSC.
-
(a) Y. Zhang, H. Peng, M. Zhang, Y.-X. Cheng and C.-J. Zhu, Chem. Commun., 2011, 47, 2354 RSC;
(b) W. Yang, L. Wei, F. Yi and M.-Z. Cai, Tetrahedron, 2016, 72, 4059 CrossRef CAS.
-
(a) Z. Li and C.-J. Li, Eur. J. Org. Chem., 2005, 2005, 3173 CrossRef;
(b) G. Zhang, Y.-X. Ma, G.-B. Cheng, D.-B. Liu and R. Wang, Org. Lett., 2014, 16, 656 CrossRef CAS;
(c) E. Boess, C. Schmitz and M. Klussmann, J. Am. Chem. Soc., 2012, 134, 5317 CrossRef CAS;
(d) W.-S. Yang, L. Wei, F.-Y. Yi and M.-Z. Cai, Tetrahedron, 2016, 72, 4059 CrossRef CAS;
(e) Y. Liu, C. Wang, D. Xue, M. Xiao, C.-Q. Li and J.-L. Xiao, Chem.–Eur. J., 2017, 23, 3051 CrossRef CAS PubMed.
-
(a) W. Han and A. R. Ofial, Chem. Commun., 2009, 0, 5024 RSC;
(b) P. Liu, Y.-G. Liu, E. L. M. Wong, S. Xiang and C. M. Che, Chem. Sci., 2011, 2, 2187 RSC;
(c) A. Wagner, W. Han, P. Mayer and A. R. Ofial, Adv. Synth. Catal., 2013, 355, 3058 CrossRef CAS;
(d) M. Patil, A. R. Kapdi and A. V. Kumar, RSC Adv., 2015, 5, 54505 RSC;
(e) L. Zhang, X. Gu, P. Lu and Y.-G. Wang, Tetrahedron, 2016, 72, 2359 CrossRef CAS;
(f) M. Huang, Q. Deng, Q. Gao, J. Shi, X.-H. Zhang and Y. Xiong, Asian J. Org. Chem., 2018, 7, 404 CrossRef CAS.
- M. R. Patil, N. P. Dedhia, A. R. Kapdi and A. V. Kumar, J. Org. Chem., 2018, 83, 4477 CrossRef CAS PubMed.
- S. Singhal, S. L. Jain and B. Sain, Chem. Commun., 2009, 2371 RSC.
- K. Alagiri and K. R. Prabhu, Org. Biomol. Chem., 2012, 10, 835 RSC.
- X.-Z. Shu, X.-F. Xia, Y.-F. Yang, K.-G. Ji, X.-Y. Liu and Y.-M. Liang, J. Org. Chem., 2009, 74, 7464 CrossRef CAS PubMed.
- J. M. Allen and T.-H. Lambert, J. Am. Chem. Soc., 2011, 133, 1260 CrossRef CAS PubMed.
- L. Liu, Z. Wang, X. Fu and C.-H. Yan, Org. Lett., 2012, 14, 5692 CrossRef CAS PubMed.
-
(a) C. Zhang, C. Liu, Y. Shao, X.-G. Bao and X.-B. Wan, Chem.–Eur. J., 2013, 19, 17917 CrossRef CAS PubMed;
(b) H.-X. Wang, Y. Shao, H. Zheng, H.-H. Wang, J. Cheng and X.-B. Wan, Chem.–Eur. J., 2015, 21, 18333 CrossRef CAS.
- H. Ued, K. Yoshida and H. Tokuyama, Org. Lett., 2014, 16, 4194 CrossRef PubMed.
- H. Shen, X.-H. Zhang, Q. Liu, J. Pan, W. Hu, Y. Xiong and X.-M. Zhu, Tetrahedron Lett., 2015, 56, 5628 CrossRef CAS.
- A. Wagner and A. R. Ofial, J. Org. Chem., 2015, 80, 2848 CrossRef CAS PubMed.
- Z. Zhang, K. Gu, Z. Bao, H.-B. Xing, Q.-W. Yang and Q.-L. Ren, Tetrahedron, 2017, 73, 3118 CrossRef CAS.
- Q. Liu, S.-C. Yu, L.-Z. Hu, M. I. Hussain, X.-H. Zhang and Y. Xiong, Tetrahedron, 2018, 74, 7209 CrossRef CAS.
-
(a) M.-X. Sun, F.-Y. Wang, B.-H. Xu, X.-Q. Ma and S.-J. Zhang, Org. Biomol. Chem., 2018, 16, 1971 RSC;
(b) V. V. Zhdankin, C. J. Kuehl, A. P. Krasutsky, J. T. Bolz, B. Mismash, J. K. Woodward and A. J. Simonsen, Tetrahedron Lett., 1995, 36, 7975 CrossRef CAS.
- A. M. Nauth, N. Otto and T. Opatz, Adv. Synth. Catal., 2015, 357, 3424 CrossRef CAS.
- P.-Y. Liu, C. Zhang, S.-C. Zhao, F. Yu, F. Li and Y.-P. He, J. Org. Chem., 2017, 82, 12786 CrossRef CAS.
- T. Castanheiro, J. Suffert, M. Donnard and M. Gulea, Chem. Soc. Rev., 2016, 45, 494 RSC.
-
(a) B. Chen, S. Guo, X. Guo, G. Zhang and Y. Yu, Org. Lett., 2015, 17, 4698 CrossRef CAS PubMed;
(b) H. Yang, X. H. Duan, J.-F. Zhao and L.-N. Guo, Org. Lett., 2015, 17, 1998 CrossRef CAS PubMed;
(c) W. Fan, Q. Yang, F. Xu and P. Li, J. Org. Chem., 2014, 79, 10588 CrossRef CAS PubMed;
(d) S. Mitra, M. Ghosh, S. Mishra and A. Hajra, J. Org. Chem., 2015, 80, 8275 CrossRef CAS PubMed;
(e) A. K. Yadav and L. D. S. Yadav, Tetrahedron Lett., 2015, 56, 6696 CrossRef CAS;
(f) L.-N. Guo, Y.-R. Gu, H. Yang and J. Hu, Org. Biomol. Chem., 2016, 14, 3098 RSC.
-
(a) G.-Y. Zhang, J.-T. Yu, M.-L. Hu and J. Cheng, J. Org. Chem., 2013, 78, 2710 CrossRef CAS;
(b) Y.-B. Huang, Y. Yu, Z.-Z. Zhu, C.-L. Zhu, J.-H. Cen, X.-W. Li, W.-Q. Wu and H.-F. Jiang, J. Org. Chem., 2017, 82, 7621 CrossRef CAS PubMed;
(c) W. Guo, W. Tan, M.-M. Zhao, L.-Y. Zheng, K.-L. Tao, D.-L. Chen and X.-L. Fan, J. Org. Chem., 2018, 83, 6580 CrossRef CAS PubMed;
(d) A. R. Sardarian, I. D. Inaloo, A. R. Modarresi-Alam, E. Kleinpeter and U. Schilde, J. Org. Chem., 2019, 84, 1748 CrossRef CAS.
-
(a) N. A. Romero and D. A. Nicewicz, Chem. Rev., 2016, 116, 10075 CrossRef CAS PubMed;
(b) J. P. Goddard, C. Ollivier and L. Fensterbank, Acc. Chem. Res., 2016, 49, 1924 CrossRef CAS PubMed;
(c) M. H. Shaw, J. Twilton and D. W. C. MacMillan, J. Org. Chem., 2016, 81, 6898 CrossRef CAS PubMed;
(d) L. Revathi, L. Ravindar, W.-Y. Fang, K. P. Rakesh and H.-L. Qin, Adv. Synth. Catal., 2018, 360, 4652 CrossRef CAS;
(e) C.-S. Wang, P. H. Dixneuf and J. F. Soule, Chem. Rev., 2018, 118, 7532 CrossRef CAS;
(f) Y.-Y. Chen, L.-Q. Lu, D.-G. Yu, C.-J. Zhu and W.-J. Xiao, Sci. China: Chem., 2019, 62, 24 CrossRef CAS.
-
(a) Y. Pan, S. Wang, C. W. Kee, E. Dubuisson, Y.-Y. Yang, K. P. Loh and C. H. Tan, Green Chem., 2011, 13, 3341–3344 RSC;
(b) M. Rueping, S. Zhu and R. M. Koenigs, Chem. Commun., 2011, 47, 12709 RSC;
(c) D. P. Hari and B. König, Org. Lett., 2011, 13, 3852 CrossRef CAS PubMed;
(d) W. To, G. Tong, W. Lu, C.-S. Ma, J. Liu, A. L. F. Chow and C.-M. Che, Angew. Chem., Int. Ed., 2012, 51, 2654 CrossRef CAS PubMed;
(e) M. Rueping, J. Zoller, D. C. Fabry, K. Poscharny, R. M. Koenigs, T. E. Weirich and J. Mayer, Chem.–Eur. J., 2012, 18, 3478 CrossRef CAS PubMed;
(f) D. B. Ushakov, K. Gilmore, D. Kopetzki, D. T. McQuade and P. H. Seeberger, Angew. Chem., Int. Ed., 2014, 53, 557 CrossRef CAS;
(g) J. C. O. Pacheco, A. Lipp, A. M. Nauth, F. Acke, J. P. Dietz and T. Opatz, Chem.–Eur. J., 2016, 22, 5409 CrossRef PubMed;
(h) A. M. Nauth, A. Lipp, B. Lipp and T. Opatz, Eur. J. Org. Chem., 2017, 2017, 2099 CrossRef CAS;
(i) A. M. Nauth, J. C. O. Pacheco, S. Pusch, S. Pusch and T. Opatz, Eur. J. Org. Chem., 2017, 2017, 6966 CrossRef CAS;
(j) T. Ide, K. Shimizu, H. Egami and Y. Hamashima, Tetrahedron Lett., 2018, 59, 3258 CrossRef CAS;
(k) A. M. Nauth, E. Schechtel, R. Dören, W. Tremel and T. Opatz, J. Am. Chem. Soc., 2018, 140, 14169 CrossRef CAS PubMed;
(l) O. Yilmaz, M. S. Oderinde and M. H. Emmert, J. Org. Chem., 2018, 83, 11089 CrossRef CAS PubMed.
-
(a) I. R. Wilson and G. M. Harris, J. Am. Chem. Soc., 1960, 82, 4515 CrossRef CAS;
(b) I. R. Wilson and G. M. Harris, J. Am. Chem. Soc., 1961, 83, 286 CrossRef CAS;
(c) A. A. Christy and P. K. Egeberg, Talanta, 2000, 51, 1049 CrossRef CAS;
(d) J. N. Figlar and D. M. Stanbury, Inorg. Chem., 2000, 39, 5089 CrossRef CAS PubMed;
(e) A. L. Collado and M. Diaz, Ind. Eng. Chem. Res., 2009, 48, 9902 CrossRef.
-
(a) H. Bartling, A. Eisenhofer, B. Konig and R. M. Gschwind, J. Am. Chem. Soc., 2016, 138, 11860 CrossRef CAS PubMed;
(b) X.-J. Wang, Q.-Y. Li, Z. Ma, W.-Q. Zhang, J.-L. Xu, W. Wei, H. Lu and X.-S. Zhao, Chem. Commun., 2016, 52, 11284 RSC;
(c) W.-Q. Ji, P.-H. Li, S.-A. Yang and L. Wang, Chem. Commun., 2017, 53, 8482 RSC.
Footnote |
† Electronic supplementary information (ESI) available. See DOI: 10.1039/c9ra06120g |
|
This journal is © The Royal Society of Chemistry 2019 |
Click here to see how this site uses Cookies. View our privacy policy here.