DOI:
10.1039/C9RA05855A
(Paper)
RSC Adv., 2019,
9, 40378-40387
Self-assembly modification of polyurethane sponge for application in oil/water separation†
Received
28th July 2019
, Accepted 22nd November 2019
First published on 5th December 2019
Abstract
A polyurethane (PU) sponge with excellent oil/water separation property has been successfully prepared by modifying with octadecyltrichlorosilane (OTS) self-assemblies. The chemical structure, surface topography, and surface wettability of the sponge were characterized by Fourier transform infrared spectroscopy (FTIR), scanning electron microscopy, and contact angle experiments, respectively. The prepared sponge could completely absorb oil within a few seconds. In addition, it also possessed excellent selectivity for oil, high absorption capacity (25 times the self-weight), high oil retention (92.6%), and good recyclability. The sponge was synthesized by a facile, mild and inexpensive method, and has excellent potential for use in practical applications because of its desirable property of oil/water separation.
1. Introduction
In recent decades, there has been an increase in the number of oil spillages as well as the industrial discharge of oils/organic solvents, which pose a significant threat to marine ecosystems.1–9 Therefore, there is a growing demand for the preparation of oil-absorbing materials.
Recently, a number of traditional materials, including activated carbon,10,11 carbon nanotubes,12,13 zeolites,14,15 fibers,16 and straw17 have been used to remove and collect spilled oil. However, these materials have a number of drawbacks, such as low absorption capacity, none or poor selectivity, and poor reusability. Therefore, it is urgent to develop absorbent materials with better performance for the efficient removal of oils/organic solvents.
Hydrophobicity is desirable and crucial for an oil absorbent, since it will absorbs oils selectively while repelling water.18 To date, many studies have focused on developing materials with both hydrophobicity and lipophilicity. These include mesh/membranes,19 carbon nanotubes,20,21 polymer gels, and nanoporous polymers.22,23 Various materials with three-dimensional (3D) structure have been reported with high porosity, low density, and recyclability and showed great advantages in the absorption of oils and organic solvents; these include CNT- and graphene-based absorbent materials and bacterial cellulose.24 Unfortunately, complicated processes and high costs prevent the use of these materials in practical applications.
PU sponge is an inexpensive, highly porous, good elasticity, 3D material with a large internal surface area, and has been modified for oil/water separation applications.25–28 At present, the preparation of hydrophobic sponges has attracted considerable attention. For example, Zhu et al.29 prepared a superhydrophobic PU sponge through a solution immersion method. The as-prepared sponges absorbed various kinds of oils up to above 13 times the sponges' weight. Nguyen et al.30 modified sponges with graphene by a dip-coating method, and they showed superhydrophobicity and excellent absorption performance for a broad range of oils. However, the oil absorption capacity drastically dropped after the first absorption-squeezing cycle. Zhu et al.31 coated polysiloxane onto a PU sponge, which improved the durability in a corrosive environment. Although these sponge absorbent materials exhibited great absorption capacity for oils/organic solvents, they were still limited in application because of some limits, such as expensive materials and complicated procedures. Therefore, it is necessary to establish a facile and mild method for preparing hydrophobic porous materials. The modification of a sponge from superhydrophilic to superhydrophobic while maintaining its capability of absorbing oils/organic solvents, thus realizing its application for removal of oils or organic solvents from water is desirable.32 The hydrophilicity of a PU sponge is a major problem in the modification process, because it is composed of carbonyl and amino groups.33
In this study, a PU sponge with a great absorption capacity was prepared using OTS self-assemblies. The schematic illustrations of the preparation method and oil/water separation process are presented in Fig. 1. The as-prepared sponge has an excellent potential to be used in practical applications because of its desirable property of oil/water separation. More interestingly, the as-prepared sponge could completely absorb oil within a few seconds. In addition, it also possessed excellent selectivity for oil, high absorption capacity (25 times the self-weight), and high oil retention (92.6%). This study provides a simple and inexpensive method for fabricating a hydrophobic and lipophilic sponge that might be applied to oil/water separation.
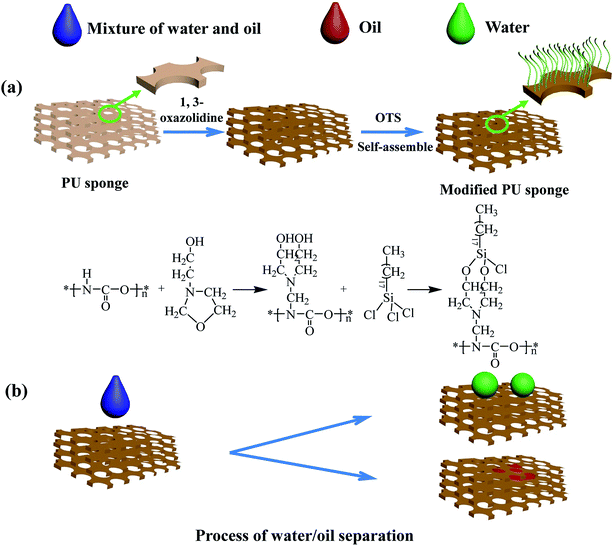 |
| Fig. 1 (a) Schematic of the PU sponge modifying process; (b) the process of oil/water separation. | |
2. Experimental
2.1. Materials
1,3-oxazolidine was synthesized according to the previous literature.34 Analytical reagent grade OTS was purchased from Aladdin Industrial Inc., Shanghai, China. Diethanolamine, formaldehyde, anhydrous ethanol, toluene, and hexane were obtained from Sinopharm Chemical Reagent Co., Ltd, China and used as received without further purification. The sponge used in this study was commercially available polyurethane sponge. Deionized water was used in all preparations.
2.2. Preparation of the hydrophobicity and lipophilic PU sponges
First, the PU sponge was cut into a square shapes (2 × 2 × 2 cm), and they were ultrasonically cleaned with ethanol and deionized water, respectively, for 30 min to remove surface stains and oils. Then, the sponges were placed in an oven at 60 °C for 24 h to dry completely.
The dried sponge was immersed in 200 mL of water solution, which contained 10% of 1,3-oxazolidine at 120 °C for 1 h. Finally, the sponge was washed with ethanol and distilled water repeatedly to remove the unreacted 1,3-oxazolidine solution, and then dried at 60 °C for 24 h.
The dried sponges modified with 1,3-oxazolidine were immersed in an anhydrous hexane solution of 3 mM OTS for 24 h at ambient temperature. Then, the sponges were washed with anhydrous hexane to remove the residuals, and then dried at 60 °C for 24 h. Finally, the long-chain OTS with –CH3 terminal groups self-assembled on the hydroxyl. The schematic of the fabrication process for the assembly of OTS SAMs on the sponges are presented in detail in Fig. 1.
2.3. Test of oil/water separation property
The modified sponge was immersed into an oil/water mixture (oil was dyed red for clear observation) until it was completely filled with oil. Then, the sponge was removed from the water surface to test the weight. Oil absorption capacities were calculated by testing the weight of the modified sponge before and after absorption, according to eqn (1): |
R (%) = [(m − m0)/m0] × 100%
| (1) |
where R (%) is the oil absorption capacity, m and m0 are the weight of sponge after absorption with oil and the weight of the control sponge, respectively.
The separation efficiency of the as-prepared sponge for different oil/water mixtures was calculated by testing the weight of oil before and after absorption, according to eqn (2):
|
Q (%) = [(n0 − n)/n0] × 100%
| (2) |
where
Q (%) is the separation efficiency,
n and
n0 are the weight of the absorbed oil and the weight of the oil before being absorbed, respectively.
2.4. Oil retention rate test
A sponge was immersed into an oil/water mixture and placed until it achieved complete absorption saturation with oil. Then, the saturated sponge was wiped with a filter paper to remove the excessive oil. The oil retention rate R of the sponge was calculated by testing the weight of the saturated sponge before and after centrifugation at 3000 rpm for 1 min, according to eqn (3): |
T (%) = [(ma − m0)/(mb − m0)] × 100%
| (3) |
where T (%) is the oil retention capacity, ma, m0, and mb are the weight of sponge after centrifugation, the weight of the control sponge, and the weight of the saturated sponge with oil (g), respectively.
2.5. Characterizations
The chemical structures were investigated using an FTIR instrument (Nicolet 170SX). SEM images were obtained by a scanning electron microscope (SEM, Korea EM-30). Contact angles were measured using an OCA50 machine (Data-Physics, Germany) under ambient temperature. The continuous oil/water separation process was investigated using a peristaltic pump (BT300-01, Longer Precision Pump Co., Ltd). The water-in-toluene emulsion particle size value was determined by dynamic light scattering (DLS) using a Zetasizer-HS (Malvern Instruments, UK). All the photos in this study were taken by a Canon camera.
3. Results and discussion
3.1. Analysis of SEM morphology
The morphologies of the PU sponge before and after copolymerization were examined by SEM. As shown in Fig. 2a, the results clearly showed that the sponge had a 3D porous structure. The pore size was about 500 μm and the skeleton had a diameter of about 50 μm. The 3D network structure is gainful for the absorption of a liquid. Meanwhile, the modified sponge had the same porous structure after the polymer modification, which confirms that the modification process couldn't destroy the porous structure of the sponge (Fig. 2c). However, compared to the pristine sponge, it is clearly observed that the 3D skeleton became rough after modification because of the presence for polymers, and the interconnected porous structure could be maintained. Surface roughness is very important for successfully preparing a hydrophobic and lipophilic surface. The results indicated that the simple functionalization process couldn't change the original structure of the pristine sponge.
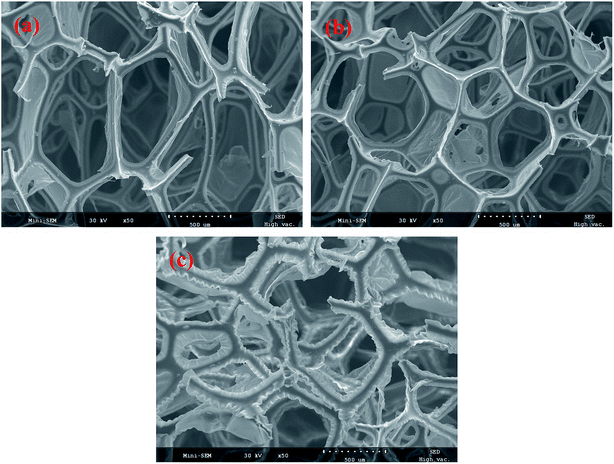 |
| Fig. 2 SEM images of the PU sponge: (a) pristine sponge; (b) after ring-opening reaction of 1,3-oxazolidine with PU sponge; (c) the PU sponge after modifying with OTS self-assemblies. | |
3.2. FTIR spectroscopy analysis
In this study, two hydroxyl groups were introduced on one secondary amine N–H bond of the PU surface by a grafting reaction, which could significantly improve the surface reactivity of the PU sponge. Then, the long-chain OTS self-assembled on the hydroxyl group, which was obtained from a ring-opening reaction of 1,3-oxazolidine and the secondary amine N–H bonds of the PU sponge. Finally, the OTS SAMs with –CH3 terminal groups were prepared on the substrates of the sponges, which could remarkably increase the hydrophobicity and lipophilicity.
The chemical structures were further characterized by FTIR. Fig. 3 shows the FTIR spectra of the pristine sponge and polymer-modified sponge. As shown in Fig. 3, the vibration peaks appear at about 1100, 1460, and 1541 cm−1, which are assigned to C–O stretching vibrations, C–H bending vibrations, C–N stretching vibrations, respectively.35 The peaks appearing at about 2843 and 2920 cm−1 are attributed to the C–H stretching vibrations of methyl and methylene groups, respectively. The results confirmed that copolymerization took place as expected and the sponge was covered with OTS SAMs on the surface.
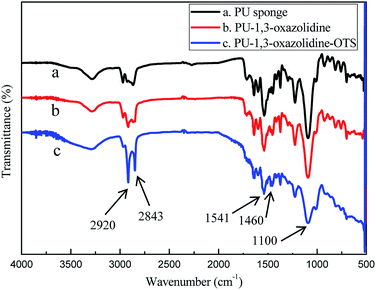 |
| Fig. 3 FTIR spectra of PU sponge: (a) pristine sponge; (b) after ring-opening reaction of 1,3-oxazolidine with PU sponge; (c) after modifying with OTS self-assemblies. | |
3.3. Properties of hydrophobicity and lipophilic
Oil/water separation typically concerns the interface, and the effective strategy is to design novel materials with special wettability.36 To further estimate the surface wettability of the sponges, contact angle measurements were performed by placing droplets of oil and water on the surface of sponges. As shown in Fig. 4, the unmodified sponge had a good hydrophilic property based on the shape of a water droplet (Fig. 4a). However, the water droplet remained stable on the surface of the modified sponge, indicating that it displayed hydrophobicity because of the alkyl chain of OTS SAMs with the low surface tension, and the water contact angle was about 156° (Fig. 4c). However, the bean oil droplet was almost absorbed into the pores of the modified sponge, and the bean oil contact angle was about 0° (Fig. 4d). The bean oil could spread on the surface and permeate into the inner part of the modified sponge. This phenomenon was due to the strong lipophilicity of the functional alkyl chain attached to the sponge and the capillary force of the pores, resulting in great oil absorption performance.
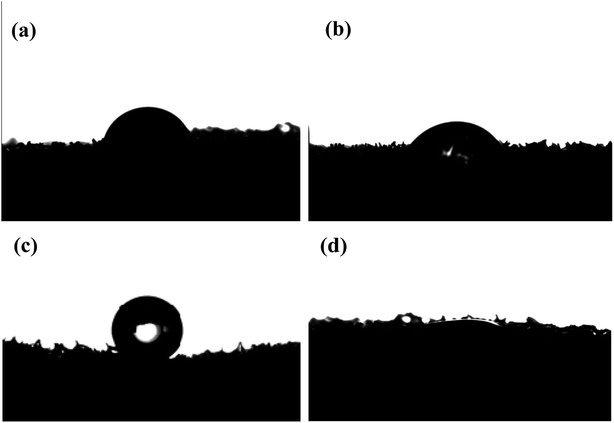 |
| Fig. 4 The water contact angle (CA) tests of PU sponges: (a) the image of a water droplet (2 μL) on the pristine sponge with a contact angle of about 63°; (b) the image of a bean oil droplet (2 μL) on the pristine sponge with a contact angle of about 46°; (c) the image of a water droplet (2 μL) on the modified sponge with a contact angle of 156°; (d) bean oil droplet (2 μL) spread and permeated quickly on the modified sponge. | |
The photographs of the modified sponge with hydrophobicity and lipophilic wettability were also obtained. As shown in Fig. 5, the modified sponge had special surface wettability. The water droplets (dyeing using a disperse dye for obvious comparison) stood on the modified sponge surface like balls, and it could very easily roll off the surface (Fig. 5c). Nevertheless, the water droplets were absorbed by the pristine sponge as soon as they were placed on the surface (Fig. 5a). In contrast, when the bean oil droplet was dropped on the surface of the sponge modified with OTS SAMs, it was immediately absorbed by the sponge very quickly, as indicated in Fig. 5d, demonstrating the superoleophilic property.
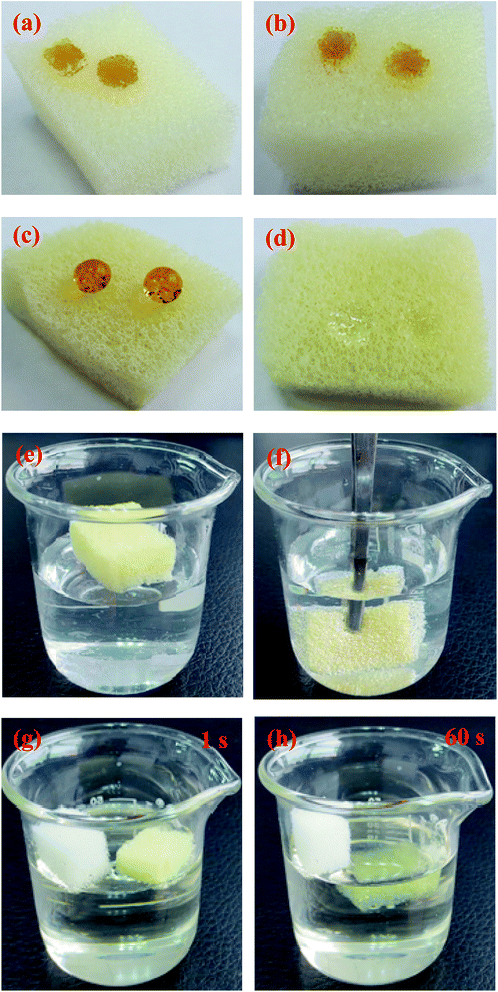 |
| Fig. 5 The hydrophobicity and lipophilic photographs of the modified sponges: (a) the image of a water droplet (10 μL) on the pristine sponge; (b) the image of a water droplet (10 μL) on the sponge after modifying with 1,3-oxazolidine; (c) the image of a water droplet (10 μL) on the sponge after modifying with OTS self-assemblies; (d) the image of a bean oil droplet (10 μL) on the sponge after modifying with OTS self-assemblies; (e) optical image of the grafted PU sponge with OTS self-assemblies floating on water due to its hydrophobicity and light weight; (f) optical image of the grafted sponge immersed in water by an external force, exhibiting a silver mirror-like surface due to the surrounding air bubbles; (g and h) optical image of the immersion process of the pristine (left) and modified sponges (right) in the bean oil. The pristine sponge floated on the surface of the oil with poor wettability, while the modified sponge was completely immersed in oil with excellent wettability after 60 s. | |
When the modified sponge was placed on water, it could float on the water surface due to hydrophobicity and its light weight (Fig. 5e). In addition, when the sponge was immersed in water, it could exhibit a typical silver mirror-like surface as a result of a uniform air layer trapped between the hydrophobic surface and water. After releasing the external force, it would instantaneously float on the surface of water again without any water being absorbed into the sponge (Fig. 5f). This phenomenon was referred to the non-wetting Cassie–Baxter model.37,38 Moreover, the lipophilic property of the sponges was investigated. When both the sponges were put on a bean oil surface simultaneously, the pristine sponge floated on the surface of oil due to its low oil wettability. In contrast, the modified sponge was completely immersed into oil because of its excellent wettability after 60 s (Fig. 5g and h). Based on the above discussions, we conclude that the modified sponge has excellent potential to be used in oil/water separation.
3.4. Absorption capacity and oil retention rate of the modified sponge
The absorption capacity of the modified sponge for oil was investigated. The amount of absorbed oil was determined using the mass method, as shown in eqn (1). The results are shown in Fig. 6a. The modified sponge could exhibit good absorption for kinds of oils and organic solvents, such as lubrication oil, bean oil, acetone, peanut oil, and n-hexane. It was revealed that the modified sponge exhibited excellent absorption capacity in the range 20–25 times the amount of its own weight at a saturated state. The 3D hierarchical porous structure and lipophilic property ensure excellent absorption capacity. Furthermore, the as-prepared sponge modified with OTS SAMs exhibited higher absorption capacity than previously reported absorbent materials, such as PDMS foam (4–11 times)39 and superhydrophobic sponges (13–18 times).36 Therefore, the sponge had greater potential for oil absorption applications.
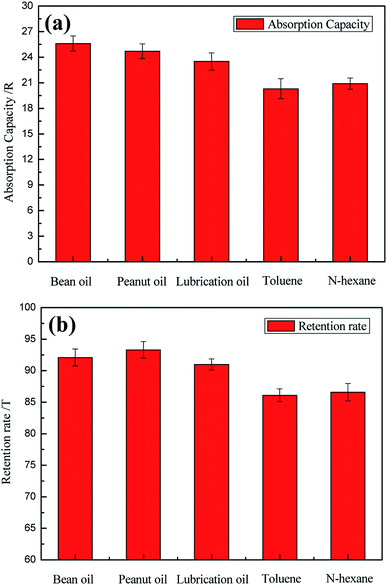 |
| Fig. 6 (a) The absorption capacities of the modified sponge for different kinds of oils; (b) the oil retention rates of the modified sponge. | |
As we know, in the process of oil clean up, the saturated sponge needs to be recycled. This requires the modified sponge to have a good oil retention property to prevent the desorption of oil caused by some external force. The oil retention rates for several oils and organic solvents were tested. As shown in Fig. 6b, the modified sponge exhibited nearly perfect oil retention (92.6% for bean oil as an example).
3.5. Separation of oil/water
Oil/water separation and the recyclability of the modified sponge are important in oils/organic solvents cleanup applications. Oil/water separation of the modified sponge was investigated. As shown in Fig. 7a, when a piece of modified sponge was placed on the surface of oil/water mixtures, bean oil (dyed by oil red) could be absorbed within a few seconds, resulting in the transparent region (clean water) that was originally contaminated by the oil. The modified sponge floated on the water surface during the whole absorption process because of its low density and superhydrophobicity. Subsequently, the oil was separated entirely from the water simply by taking the sponge out, and the absorbed oil could be collected from the sponge through a simple mechanical squeezing process. The as-prepared sponge also effectively absorbed some oils and organic solvents, such as peanut oil, lubrication oil, toluene, and n-hexane.
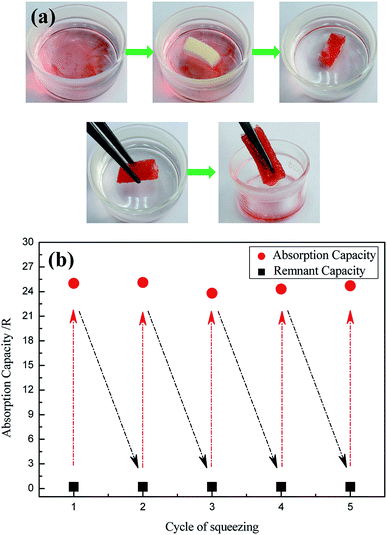 |
| Fig. 7 (a) The process of oil/water separation for the modified sponge, and the collection of bean oil (dyed by oil red) from the water surface; (b) the changes in the adsorption capacity of bean oil for the modified sponge after 5 squeezing cycles. | |
For practical applications, oil absorption capacity and recyclability of the modified sponge are important factors. The reusability of the modified sponge was investigated by repeated absorption–desorption process. The changes in the absorption capacity of bean oil for the modified sponge with squeezing cycles are presented in Fig. 7b. Because of its excellent elasticity and durability, it was very interesting to note that the modified sponge still maintained high absorption capacity after being squeezed for 5 cycles. The excellent properties implied that the as-prepared sponge has excellent potential for use in practical applications.
The separation efficiency of the as-prepared sponge for different oil/water mixtures was calculated, as shown in eqn (2). The mixtures of bean oil/water, peanut oil/water, lubrication oil/water, toluene/water, and n-hexane/water were successfully separated with high efficiency. Almost no visible oil existed in the water after separation. The separation efficiency of the as-prepared sponge for different oil/water mixtures is presented in Fig. 8. It can be seen that the separation efficiency is high regardless of the mixture of oil and water. The excellent performances imply that the as-prepared sponge is a prospective candidate for oil/water separation.
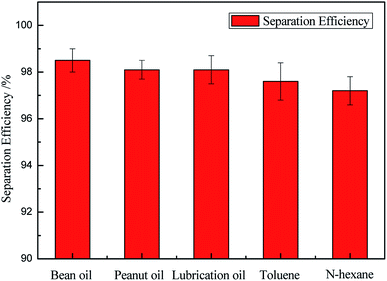 |
| Fig. 8 The separation efficiency of the as-prepared sponge for different oil/water mixtures. | |
Industrial waste often contains some surfactants. In order to further investigate the separation performance for surfactant-stabilized emulsions, a surfactant-stabilized water-in-toluene emulsion was prepared. The size of the particles in the water-in-toluene emulsion was investigated by DLS. As shown in Fig. 9a, the average size of the emulsion particles was about 710 nm. After separation of the emulsion through modified sponge absorption, the separated emulsion became much transparent compared to the original milky white emulsion by the removal of oil, as shown in Fig. 9b. The significant difference in phase composition before and after separation illustrates that the emulsion could be separated effectively.
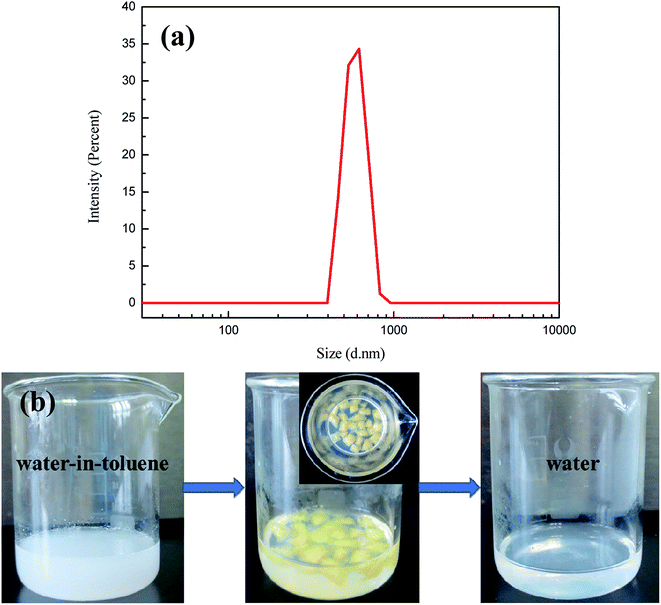 |
| Fig. 9 (a) Droplet size distribution of surfactant-stabilized water-in-toluene emulsion; (b) photo of a surfactant-stabilized water-in-toluene emulsion before and after separation. | |
3.6. Continuous oil/water separation
The continuous oil/water separation was investigated using a peristaltic pump. Large amounts of oil (the toluene was dyed with oil red for clear observation) were continuously collected from oil/water mixtures, as illustrated in Fig. 10. When the tube tied with the modified sponge was placed at the oil/water interface, it quickly absorbed oil and repelled water completely due to its hydrophobicity and lipophilic wettability. Furthermore, when the peristaltic pump was turned on, a stream of oil (toluene) was formed in the tube, because toluene was continuously absorbed through the modified sponge, and the thickness of the oil layer gradually decreased. Finally, the oil could be successfully collected by a small piece of modified sponge under the peristaltic pump, and no water could be found in the collected oil (Fig. 10 and Video S1 in ESI†). The good selectivity of the modified sponge for oil/water mixtures was probably owing to its high capillary action as well as its hydrophobicity and lipophilic wettability. These results indicated that the modified sponge has potential in application for oil/water separation.
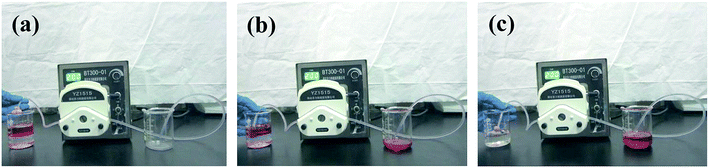 |
| Fig. 10 A peristaltic pump-assisted continuous oil/water separation of the modified sponge. Toluene was dyed with oil red for clear observation. (a) Before separation; (b) during separation; (c) after separation. | |
4. Conclusions
A sponge with excellent oil/water separation property has been successfully prepared by modifying with OTS self-assemblies. The sponge exhibiting hydrophobicity and lipophilicity was synthesized by a facile, mild and inexpensive method, and has excellent potential for use in the separation of oils or organic solvents from water. The oil absorption process of the sponge was completed within a few seconds and no water was detected in the sponge. In addition, it also possessed excellent selectivity for oil, high absorption capacity (25 times the self-weight), high oil retention (92.6%), and good recyclability. The sponge developed in this study will be a promising material for large-scale removal of oils and organic contaminants from water.
Conflicts of interest
On behalf of all authors, the corresponding author states that there is no conflict of interest.
Acknowledgements
The authors gratefully acknowledge the financial support of the National Natural Science Foundation of China (Grant no. 51703130), and the International Science and Technology Cooperation Project of Shaoxing University (Grant no. 2019LGGH1004).
References
- C. Yang, U. Kaipa, Q. Z. Mather, X. Wang, V. Nesterov, A. F. Venero and M. A. Omary, Fluorous metal-organic frameworks with superior adsorption and hydrophobic properties toward oil spill cleanup and hydrocarbon storage, J. Am. Chem. Soc., 2011, 133, 18094–18097 CrossRef CAS PubMed.
- J. Schaum, M. Cohen, S. Perry, R. Artz, R. Draxler, J. B. Frithsen, D. Heist, M. Lorber and L. Phillips, Screening level assessment of risks due to dioxin emissions from burning oil from the BP Deepwater Horizon Gulf of Mexico spill, Environ. Sci. Technol., 2010, 44, 9383–9389 CrossRef CAS PubMed.
- E. B. Kujawinski, M. C. Kido Soule, D. L. Valentine, A. K. Boysen, K. Longnecker and M. C. Redmond, Fate of dispersants associated with the Deepwater Horizon Oil Spill, Environ. Sci. Technol., 2011, 45, 1298–1306 CrossRef CAS PubMed.
- S. T. Nguyen, J. Feng, N. T. Le, A. T. T. Le, N. Hoang, V. B. C. Tan and H. M. Duong, Cellulose Aerogel from Paper Waste for Crude Oil Spill Cleaning, Ind. Eng. Chem. Res., 2013, 52, 18386–18391 CrossRef CAS.
- L. Guterman, Exxon Valdez Turns 20, Science, 2009, 323, 1558–1559 CrossRef CAS PubMed.
- X. Wang, M. Li, Y. Shen, Y. Yang, H. Feng and J. Li, Facile preparation of loess-coated membranes for multifunctional surfactant-stabilized oil-in-water emulsion separation, Green Chem., 2019, 21, 3190–3199 RSC.
- H. Zhang, Y. Shen, M. Li, G. Zhu, H. Feng and J. Li, Egg Shell Powders-Coated Membrane for Surfactant-Stabilized Crude Oil-in-Water Emulsions Efficient Separation, ACS Sustainable Chem. Eng., 2019, 7, 10880–10887 CrossRef CAS.
- J. Li, C. Xu, C. Guo, H. Tian, F. Zha and L. Guo, Underoil superhydrophilic desert sand layer for efficient gravity-directed water-in-oil emulsions separation with high flux, J. Mater. Chem. A, 2018, 6, 223–230 RSC.
- Y. Long, Y. Shen, H. Tian, Y. Yang, H. Feng and J. Li, Superwettable Coprinus comatus coated membranes used toward the controllable separation of emulsified oil/water mixtures, J. Membr. Sci., 2018, 565, 85–94 CrossRef CAS.
- L. Yang, S. Wu and J. P. Chen, Modification of Activated Carbon by Polyaniline for Enhanced Adsorption of Aqueous Arsenate, Ind. Eng. Chem. Res., 2007, 46, 2133–2140 CrossRef CAS.
- A. Bayat, S. F. Aghamiri, A. Moheb and G. R. Vakili-Nezhaad, Oil Spill Cleanup from Sea Water by Sorbent Materials, Chem. Eng. Technol., 2005, 28, 1525–1528 CrossRef CAS.
- R. Q. Long and R. T. Yang, Carbon Nanotubes as Superior Sorbent for Dioxin Removal, J. Am. Chem. Soc., 2001, 123, 2058–2059 CrossRef CAS PubMed.
- S. Huang and J. Shi, Monolithic Macroporous Carbon Materials as High-Performance and Ultralow-Cost Sorbents for Efficiently Solving Organic Pollution, Ind. Eng. Chem. Res., 2014, 53, 4888–4893 CrossRef CAS.
- H. M. Choi and R. M. Cloud, Natural sorbents in oil spill cleanup, Environ. Sci. Technol., 1992, 26, 772–776 CrossRef CAS.
- Q. Wen, J. Di, L. Jiang, J. Yu and R. Xu, Zeolite-coated mesh film for efficient oil–water separation, Chem. Sci., 2013, 4, 591–595 RSC.
- M. Zhang, C. Wang, S. Wang, Y. Shi and J. Li, Fabrication of coral-like superhydrophobic coating on filter paper for water–oil separation, Appl. Surf. Sci., 2012, 261, 764–769 CrossRef CAS.
- Y. P. Patil, B. Gajre, D. Dusane, S. Chavan and S. Mishra, Effect of maleic anhydride treatment on steam and water absorption of wood polymer composites prepared from wheat straw, cane bagasse, and teak wood sawdust using Novolac as matrix, J. Appl. Polym. Sci., 2000, 77, 2963–2967 CrossRef CAS.
- Q. Liu, K. Meng, K. Ding and Y. Wang, A Superhydrophobic Sponge with Hierarchical Structure as an Efficient and Recyclable Oil Absorbent, Chempluschem, 2015, 80, 1435–1439 CrossRef CAS.
- J. Drelich, E. Chibowski, D. D. Meng and K. Terpilowski, Hydrophilic and superhydrophilic surfaces and materials, Soft Matter, 2011, 7, 9804–9828 RSC.
- L. Guo, W. Yuan, J. Li, Z. Zhang and Z. Xie, Stable superhydrophobic surfaces over a wide pH range, Appl. Surf. Sci., 2008, 254, 2158–2161 CrossRef CAS.
- M. Li, J. Xu and Q. Lu, Creating superhydrophobic surfaces with flowery structures on nickel substrates through a wet-chemical-process, J. Mater. Chem., 2007, 17, 4772–4776 RSC.
- J. Wu, J. Chen, K. Qasim, J. Xia, W. Lei and B.-p. Wang, A hierarchical mesh film with superhydrophobic and superoleophilic properties for oil and water separation, J. Chem. Technol. Biotechnol., 2012, 87, 427–430 CrossRef CAS.
- C. Wang, T. Yao, J. Wu, C. Ma, Z. Fan, Z. Wang, Y. Cheng, Q. Lin and B. Yang, Facile Approach in Fabricating Superhydrophobic and Superoleophilic Surface for Water and Oil Mixture Separation, ACS Appl. Mater. Interfaces, 2009, 1, 2613–2617 CrossRef CAS PubMed.
- Z.-Y. Wu, C. Li, H.-W. Liang, J.-F. Chen and S.-H. Yu, Ultralight, Flexible, and Fire-Resistant Carbon Nanofiber Aerogels from Bacterial Cellulose, Angew. Chem., 2013, 125, 2997–3001 CrossRef.
- P. Calcagnile, D. Fragouli, I. S. Bayer, G. C. Anyfantis, L. Martiradonna, P. D. Cozzoli, R. Cingolani and A. Athanassiou, Magnetically Driven Floating Foams for the Removal of Oil Contaminants from Water, ACS Nano, 2012, 6, 5413–5419 CrossRef CAS PubMed.
- Y. Liu, J. Ma, T. Wu, X. Wang, G. Huang, Y. Liu, H. Qiu, Y. Li, W. Wang and J. Gao, Cost-Effective Reduced Graphene Oxide-Coated Polyurethane Sponge As a Highly Efficient and Reusable Oil-Absorbent, ACS Appl. Mater. Interfaces, 2013, 5, 10018–10026 CrossRef CAS PubMed.
- J. Li, L. Shi, Y. Chen, Y. Zhang, Z. Guo, B.-l. Su and W. Liu, Stable superhydrophobic coatings from thiol-ligand nanocrystals and their application in oil/water separation, J. Mater. Chem., 2012, 22, 9774–9781 RSC.
- C.-F. Wang and S.-J. Lin, Robust Superhydrophobic/Superoleophilic Sponge for Effective Continuous Absorption and Expulsion of Oil Pollutants from Water, ACS Appl. Mater. Interfaces, 2013, 5, 8861–8864 CrossRef CAS PubMed.
- Q. Zhu, Q. Pan and F. Liu, Facile Removal and Collection of Oils from Water Surfaces through Superhydrophobic and Superoleophilic Sponges, J. Phys. Chem. C, 2011, 115, 17464–17470 CrossRef CAS.
- D. D. Nguyen, N.-H. Tai, S.-B. Lee and W.-S. Kuo, Superhydrophobic and superoleophilic properties of graphene-based sponges fabricated using a facile dip coating method, Energy Environ. Sci., 2012, 5, 7908–7912 RSC.
- Q. Zhu, Y. Chu, Z. Wang, N. Chen, L. Lin, F. Liu and Q. Pan, Robust superhydrophobic polyurethane sponge as a highly reusable oil-absorption material, J. Mater. Chem. A, 2013, 1, 5386–5393 RSC.
- D. D. Nguyen, N. H. Tai, S. B. Lee and W. S. Kuo, Superhydrophobic and superoleophilic properties of graphene-based sponges fabricated using a facile dip coating method, Energy Environ. Sci., 2012, 5, 7908–7912 RSC.
- H. A. Mohamed, B. M. Badran, A. M. Rabie and S. M. M. Morsi, Synthesis and characterization of aqueous (polyurethane/aromatic polyamide sulfone) copolymer dispersions from castor oil, Prog. Org. Coat., 2014, 77, 965–974 CrossRef CAS.
- I. Rotaru, M. Ionescu, D. Dan and M. Vuluga, Synthesis of a New Mannich Polyether Polyol with Isocyanuric Structure, Mater. Plast., 2008, 45, 23–28 CAS.
- V. H. Pham and J. H. Dickerson, Superhydrophobic Silanized Melamine Sponges as High Efficiency Oil Absorbent Materials, ACS Appl. Mater. Interfaces, 2014, 6, 14181–14188 CrossRef CAS PubMed.
- Q. Zhu, Q. Pan and F. Liu, Facile Removal and Collection of Oils from Water Surfaces through Superhydrophobic and Superoleophilic Sponges, J. Phys. Chem. C, 2011, 115, 17464–17470 CrossRef CAS.
- I. A. Larmour, S. E. J. Bell and G. C. Saunders, Remarkably Simple Fabrication of Superhydrophobic Surfaces Using Electroless Galvanic Deposition, Angew. Chem., Int. Ed., 2007, 46, 1710–1712 CrossRef CAS PubMed.
- N. Verplanck, Y. Coffinier, V. Thomy and R. Boukherroub, Wettability Switching Techniques on Superhydrophobic Surfaces, Nanoscale Res. Lett., 2007, 2, 577–596 CrossRef CAS.
- S.-J. Choi, T.-H. Kwon, H. Im, D.-I. Moon, D. J. Baek, M.-L. Seol, J. P. Duarte and Y.-K. Choi, A Polydimethylsiloxane (PDMS) Sponge for the Selective Absorption of Oil from Water, ACS Appl. Mater. Interfaces, 2011, 3, 4552–4556 CrossRef CAS PubMed.
Footnote |
† Electronic supplementary information (ESI) available. See DOI: 10.1039/c9ra05855a |
|
This journal is © The Royal Society of Chemistry 2019 |