DOI:
10.1039/C9RA04410H
(Paper)
RSC Adv., 2019,
9, 27398-27405
The first demonstration of a novel isolated fungus Eutypella sp. BJ associated with the biodegradation of polyvinyl alcohol
Received
12th June 2019
, Accepted 25th August 2019
First published on 30th August 2019
1. Introduction
Polyvinyl alcohol (PVA), a synthetic water-soluble polymer, is widely used in textile, food, leather, medical and paper industries for its excellent properties, such as good adhesion, film toughness and wear resistance.1 However, PVA is not readily degraded in the natural environment, resulting in serious environmental problems. For example, once dispersed in aqueous systems, PVA can directly interfere with the life cycles of aquatic organisms and convert them into a major organic pollution source that is subsequently preyed upon by fish, which may then die due to toxicity. In addition, when mixed into the soil, it can affect the absorption of nutrients and water by crops, leading to reduced crop yields. Therefore, in recent years, researchers have been investigating environmentally friendly methods to degrade PVA.
Research on the biodegradation of PVA can be traced back to 1936, when Nord found that PVA can be degraded under extracellular attack by a dehydratase of Fusarium lini.2 Since Suzuki and co-workers successfully isolated the first strain capable of utilizing PVA as the sole carbon source in 1973, researchers have conducted extensive research on the biodegradation of PVA.3,4 Pseudomonas sp. 113P3 isolated by Hatanaka was able to grow in a medium with PVA as the sole carbon source and produce a PQQ-dependent PVA dehydrogenase.5 Sakai et al. found that Pseudomonas secreted PVA oxidase, which could catalyse the oxidation of PVA and make it hydrolyse into a small molecular compound.6 However, most research to date has focused on the degradation of PVA by bacteria, and limited information is available regarding PVA degradation by fungi. Actually, the use of fungi and other versatile microbes to remove or mitigate the effects of macromolecular contaminants in the environment is especially important for environmental protection.7
In recent years, the degradation of PVA by fungi has been investigated. Yeast and basidiomycetes able to degrade lignin were founded to assimilate PVA.8,9 Furthermore, Flammulina velutipes was shown to exhibit PVA degradation ability and to preferentially assimilate the syndiotactic structure of PVA, suggesting that the mycelia produced PVA-degrading enzymes.10 A brown-rot fungus, Fomitopsis pinicola, could degrade PVA via the Fenton reaction in unsubmerged culture.11 Mejía et al. found that enzymatic extracts of the Phanerochaete chrysosporium fungus could promote the degradation of PVA and reduce the average molecular weight by approximately 80% by forming carbonyl groups and double bonds.12 Following infrared and ultraviolet analysis, they proposed a degradation route: modification of the free radical into an epoxide, followed by hydrolysis to form a double bond. A fungal strain belonging to Penicillium sp. isolated from activated sludge was reported able to completely degrade PVA after 12 days and secrete PVA oxidase to decompose H2O2.13 In addition, the fungus Aspergillus niger was demonstrated to grow vigorously on the surface of PVA films and assimilate them by SEM techniques.14 Two white-rot fungi, Pycnoporus cinnabarinus and Phanerochaete chrysosporium, have been reported able to degrade PVA.15 Following their discovery of a bacterial strain (BX1) and a fungal strain (WF9101) in activated sludge, Mori et al. studied the biodegradation mechanism of PVA oligomers and found that the strains could synergistically degrade PVA to vinyl alcohol oligomers.16–18 They proposed that the hydroxyl groups of PVA oligomers were oxidized by secondary alcohol oxidase to carbonyl groups, and subsequently, β-diketone hydrolase catalysed the cleavage of C–C bonds of oxidized vinyl alcohol oligomers.19 Lignolytic fungi might contribute to non-metabolic assimilation of PVA by extracellular oxidizing systems in soil environments.20 The biodegradation of composites based on PVA have also been studied. Trichothecium roseum fungus was found able to degrade PVA and PVA-graft-lactic acid copolymers, and copolymers with greater numbers of lactic acid grafted units were more conducive to attack by this fungus than those with fewer units.21
For this study we isolated and identified a novel fungus capable of degrading PVA from soil compost. To assess the potential of the isolate in assimilating PVA, we (1) compared the degradation characteristics of PVA 1788, 1799 and 2488, which exhibit different degrees of polymerization and saponification; (2) characterized the chemical structure changes of PVA 1788 during degradation by FTIR analysis; and (3) investigated the molecular weight and the molecular weight distribution of PVA 1788 at different times. Compared with fungi able to degrade PVA (Table 1), the isolated fungus Eutypella sp. BJ showed the ability to assimilate PVA with high efficiency in a shorter period of time. This study provides the first demonstration that Eutypella can successfully degrade PVA.
Table 1 The comparation of fungi reported able to degrade PVA
Fungus |
Efficiency of PVA degradation |
Degradation time (days) |
Researchers |
Flammulina velutipes |
53.5% |
14 |
Tsujiyama et al.10 |
Phanerochaete chrysosporium |
79.3% |
15 |
Mejía et al.12 |
Penicillium sp. WSH02-21 |
100% |
12 |
Ding et al.13 |
Aspergillus niger |
The surface being eroded |
7 |
Jecu et al.14 |
Pycnoporus cinnabarinus |
Nearly 50% |
30 |
Larking et al.15 |
Geotrichum sp. WF9101 |
80% |
8 |
Sakimoto et al.16–18 |
2. Materials and methods
2.1 Materials
PDA, MEA, CYA medium and mold liquid medium were purchased from Guangdong HuanKai Microbial Sci. & Tech. Co., Ltd. The commercial PVA powders (degree of polymerization, 1700 or 2400; degree of saponification, 88 or 99%) were purchased from Chang-Chun Petrochemical, Taiwan, China. Poly vinyl formal (PVF) compounded from PVA and formaldehyde was generously supplied by Professor Youyi Zhang from Tunghai University in Taiwan, China. Compost was obtained from Junde Biotech Co., Ltd. (Zhucheng, China). The main components and properties of the compost were as follows: chicken dung, 52.02% (w/w); straw, 28.45% (w/w); water-holding capacity, 68%; pH, 6.73.
2.2 Isolation of PVA degrading strain
Microbial samples were extracted from the surfaces of degraded PVF samples that had been buried in compost for three years in our laboratory.22 PVF particles were placed in Erlenmeyer flasks containing 50 mL sterile deionized water and shaken gently. Three millilitres of the suspension was incubated in mold liquid medium (100 mL). Then, 50 μL of culture was sprayed onto the screening agar medium using PVA as the sole carbon source and incubated at 30 °C for 7 days. Strains that successfully grew on the screening agar medium were isolated, purified, and then incubated again on the screening agar medium on sterile filter paper. Next, a 3 mL mixture of iodine and boric acid (1
:
4 by volume) was added to the plates, and the reaction was allowed to occur for 15 min in the dark.22 The assay was performed in three replicates. The colonies surrounded by a large transparent circle were selected. The composition of the screening agar medium was as follows: PVA, 1.00 g L−1; (NH4)2SO4, 1.00 g L−1; K2HPO4, 2.50 g L−1; KH2PO4, 0.20 g L−1; CaCl2, 0.05 g L−1, MgSO4·7H2O, 0.10 g L−1, FeSO4·7H2O, 0.05 g L−1; NaCl, 0.50 g L−1; chloramphenicol, 0.30 g L−1; agar, 15.00 g L−1.
2.3 DNA extraction, PCR amplification and phylogenetic analysis
Strains that grew on the screening medium and formed large transparent circles were cultured in mold liquid medium in an Erlenmeyer shaker at 30 °C and 160 rpm for 2 days. DNA of the isolated fungus was extracted from cultures by using the Ezup Column Fungi Genomic DNA Purification Kit. Two universal fungal primers ITS4 (5′-TCCTCCGCTTATTGATATGC-3′) and ITS5 (5′-GGAAGTAAAAGTCGTAACAAGG-3′) were used to amplify the DNA by PCR. The amplified PCR product was purified by electrophoresis in a 1.0% agarose gel. Purified DNA amplicons were sent to Guangzhou Qingke Biotechnology Co., Ltd., for sequencing. The DNA sequence from the fungal isolate was aligned with the sequences of closely related species from the NCBI nucleotide database by the CLUSTAL W program.23 The evolutionary tree inferred from the data was constructed using the neighbour-joining method in MEGA version 7.0.26. The stability of each branch was estimated by performing bootstrap analyses of the neighbour-joining data based on 1000 trials.
2.4 Morphology of the isolated PVA degrading fungus
The isolated fungus was separately spotted on PDA, MEA, and CYA medium and cultured at 30 °C for 7 days to observe colony morphology. The strain was inoculated into a mold liquid medium, cultured at 30 °C for 3 days, and then stained with a lactic acid carbonic acid blue staining solution to observe the morphology of the isolated fungus.
2.5 PVA degradation test
The PVA degradation test was performed in liquid cultures. The ability of the isolated strain to degrade PVA was investigated using PVA with two different degrees of polymerization and saponification. Prior to inoculum preparation, a seed culture was prepared by culturing the isolated fungus in 100 mL of mold liquid medium on a rotary shaker at 30 °C and 160 rpm for 3 days. Next, one percent of the fungal inoculum was added to the semi-synthetic medium (100 mL) and incubated in an Erlenmeyer shaker at 30 °C and 160 rpm for 8 days. Cultures were centrifuged at 10
000 rpm for 15 min at 4 °C to remove the cells for degradation analyses. The composition of the semi-synthetic medium was as follows: PVA, 1.00 g L−1; (NH4)2SO4, 1.00 g L−1; K2HPO4, 2.50 g L−1; KH2PO4, 0.20 g L−1; CaCl2, 0.05 g L−1, MgSO4·7H2O, 0.10 g L−1, FeSO4·7H2O, 0.05 g L−1; NaCl, 0.50 g L−1; chloramphenicol, 0.30 g L−1. The initial pH was neutral.
2.6 Analysis methods
The biodegradation of PVA by the isolated strain was comprehensively investigated by analysing the changes in biomass, PVA concentration, functional groups and molecular weight among time points before, as well as during and after the degradation of PVA.
2.6.1 Measurements of dry cell weight (DCW). DCW was determined to measure cell growth. The cells collected by centrifugation were washed three times with deionized water and dried to a constant weight at 105 °C.
2.6.2 Measurement of concentration of PVA. The analysis of PVA concentration was performed using a modified Finley method.22 Aqueous boric acid and iodine were added to the samples for 15 min at room temperature at a proportion of KI–I2 (2.5 + 12.7 g L−l): boric acid (4 g L−l): sample, which was equivalent to 2
:
3
:
2 (v/v/v) for PVA 1799, 1
:
3
:
1 (v/v/v) for PVA 1788 and 1
:
2
:
1 (v/v/v) for PVA 2488. The OD of each mixture was measured at 500 nm for PVA 1799, at 495 nm for PVA 1788, and at 520 nm for PVA 2488. Three measurements were taken for each sample.
2.6.3 Gel permeation chromatography analysis. Molecular weight distribution was analysed by GPC. The centrifugal supernatant was sent to Guangzhou Zhebo Testing Technology Co., Ltd., for testing by gel permeation chromatography Waters 5510, equipped with a refractive index detector/triple detector. The conditions for GPC were as follows: 10 μm mixed-b column, 1,2,4-trichlorobenzene as the elution solvent, and an effluent flow rate of 1.0 mL min−1. Calibration was performed using a polystyrene standard.
2.6.4 Fourier transform infrared spectroscopy analysis. The culture supernatant was filtered through a membrane filter (pore size, 0.22 μm). The PVA-containing supernatant was dried by a freeze dryer (Guangzhou Kepeng Scientific Instrument Co., Ltd.), and its infrared spectra at different biodegradation times were measured by using a Bruker FTIR Vector 33 spectrophotometer with the spectral regions from 400 to 4000 cm−1 at room temperature.
3. Results and discussion
3.1 Identification of the isolated PVA degrading strain
A novel fungus forming a large transparent circle on the screening agar medium with the ability to degrade PVA was isolated (Fig. 1). Identification of the fungus was performed through ITS sequence analysis and the observation of cell morphology.
 |
| Fig. 1 Agar plate assay for PVA degradation by Eutypella sp. BJ. | |
The isolated fungus was identified as Eutypella sp. by ITS sequence analysis, which had 100% similarity. Its position in a phylogenetic tree is shown in Fig. 2. The ITS sequence of Eutypella sp. BJ was submitted to GenBank under accession number MK651593.
 |
| Fig. 2 Phylogenetic analysis of the Eutypella sp. BJ based on ITS sequence. | |
After one week of growth, Eutypella sp. BJ was densely spread on the surfaces of the CYA, MEA and PDA media, with its colonies exhibiting a white, cotton-like morphology. The original inoculation point on the central of CYA medium had turned black and hard (Fig. 3(a)), and branching hyphae were visible on the edges of MEA medium (Fig. 3(b)). The mycelium of Eutypella sp. BJ incubated on the PDA medium tended to spread to the cover of the culture dish, and the mycelium was densely distributed at the centre of the medium and sparsely distributed at the edges (Fig. 3(c)). No spores were detected when Eutypella sp. BJ was cultured in mold liquid medium. After staining Eutypella sp. BJ, mycelia were observed with an optical microscope (Fig. 4).
 |
| Fig. 3 Photographs of 7 days fungal cultures incubated in Petri plates. (a) Growth of Eutypella sp. BJ on CYA medium; (b) growth of Eutypella sp. BJ on MEA medium; (c) growth of Eutypella sp. BJ on PDA medium. | |
 |
| Fig. 4 Micrographs of Eutypella sp. BJ (×1000). | |
Recent research on Eutypella has mainly focused on its metabolites, which have excellent antibacterial and antitumour activities.24–26 However, little research on the degradation of materials by Eutypella has been conducted. One report concerning its degradation ability was that Lee Jung-Bok et al. isolated Eutypella sp. KEF-1 from endosulfan-polluted soils, and it had the potential to degrade organochlorine insecticide endosulfan.27 To the best of our knowledge, Eutypella sp. BJ is the first fungus discovered to have the ability to independently degrade PVA. In addition to having metabolites with antitumour, immunosuppressive and other biological activities, Eutypella sp. may have great potential for polymer degradation.
3.2 Degradation of different types of PVA
Eutypella sp. BJ was cultivated in semi-synthetic medium at 30 °C to study its biodegradation of PVA with different degrees of polymerization and saponification. As shown in Fig. 5, degradation speed of Eutypella sp. BJ to three types of PVA on the first day was faster than that on other 7 days, and the slow growth of Eutypella sp. BJ accompanied with the tardy increase of PVA removal on the 2nd to 8th day. With the increase of DCW, the removal of the three types of PVA increased. These results indicated that the growth of Eutypella sp. BJ was closely related to the biodegradation of PVA. Eutypella sp. BJ degraded 87.40% and 86.31% of PVA 1788 and PVA 1799, respectively, after 8 days. When Eutypella sp. BJ was cultivated on PVA 1788 and PVA 1799, more cell growth was observed compared to cells grown on PVA 2488, which was consistent with their high removal rates. The inconspicuous degradation difference indicated that the degree of saponification had little effect on the degradation of PVA by Eutypella sp. BJ. Similarly, Tomoo Suzuki et al. found no marked difference in the degradation of PVA by crude enzyme of Pseudomonas O-3 among PVAs with different degrees of saponification.28 The removal rate of PVA 2488 (44.80%), which has a higher degree of polymerization than PVA 1788, was lower than PVA 1788, mainly because when the molecular chains of PVA are stretched in semi-synthetic medium, entanglement is more likely to occur between molecular chains, and higher polymerization causes greater molecular chain entanglement. In addition, PVA samples with high molecular weight had stronger intermolecular hydrogen bonding, which was beneficial to crystallization, making it more difficult to the stretch of PVA molecular chains in liquid cultures. Therefore, it was not conducive to the invasion of Eutypella sp. BJ, causing poor efficiency to degrade PVA 2488 with high molecular weight.
 |
| Fig. 5 Biodegradation curves of PVA samples with different degrees of polymerization and saponification recorded in biodegradation tests. (a) biodegradation curve of PVA 1788; (b) biodegradation curve of PVA 2488; (c) biodegradation curve of PVA 1799. | |
Previous investigations have also reported effects of the degrees of polymerization and saponification on PVA biodegradation. Roberto Solaro et al. demonstrated a moderate dependence of saponification on the degradation of PVA by a selected PVA-degrading mixed bacterial culture isolated from the sewage sludge of a paper mill treatment plant.29 Emo Chiellini found no significant differences in degradation among three PVA samples (PVA72, PVA88, PVA98) with different degrees of saponification during the biodegradation process.30 In addition, when studying the effect of PVA structure on the dehydrogenation reaction by PVA dehydrogenase (PVADH) from Pseudomonas sp. 113P3, Tadashi Hatanaka found that as the degree of polymerization decreased, the Michaelis constant of PVADH-S decreased.31 Combining with the above analysis and results of this study, the degradation of PVA depends little on the degree of saponification and is more influenced by the degree of polymerization.
3.3 GPC analysis of PVA
As PVA 1788 exhibited a relatively high removal rate, it was chosen for GPC and FTIR analysis. The GPC analysis revealed changes in the molecular weight and the molecular weight distribution of PVA 1788 over different biodegradation times. As shown in Table 2, the number average molecular weight (Mn) and the weight average molecular weight (Mw) of the degraded PVA 1788 decreased from 47
358 and 71
387 to 13
345 and 24
238, respectively, representing significant decreases of 71.82% and 66.05% over the eight-day incubation. Increases in the polydispersity index (d, d = Mw/Mn) of PVA 1788 was also observed, which changed from 1.51 (unbiodegraded PVA 1788 at 0 day) to 1.81 (biodegraded PVA 1788 at 8 days). The increase of d indicated that the molecular weight distribution of PVA 1788 had broadened. In addition, with increasing degradation time, the GPC profiles of PVA 1788 shifted towards the lower molecular weight area (Fig. 6). These behaviours can be attributed to the preferential assimilation of low molecular weight fractions of PVA 1788, which is in accordance with the literature.32 Relative to the low molecular weight fraction, the high molecular weight fraction of PVA 1788 has severe chain entanglement and strong intermolecular force, making the segments and molecular chains difficult to move; these conditions are not conducive to the invasion of Eutypella sp. BJ. Therefore, there was a tendency for Eutypella sp. BJ to assimilate the low molecular weight part of PVA 1788.
Table 2 The molecular weight and the molecular weight distribution of PVA 1788 in the semi-synthetic medium inoculated with Eutypella sp. BJ by GPC analysis
Time (days) |
Mn |
Mw |
d |
0 |
47 358 |
71 387 |
1.51 |
3 |
43 420 |
62 254 |
1.43 |
6 |
16 561 |
28 599 |
1.73 |
8 |
13 345 |
24 238 |
1.82 |
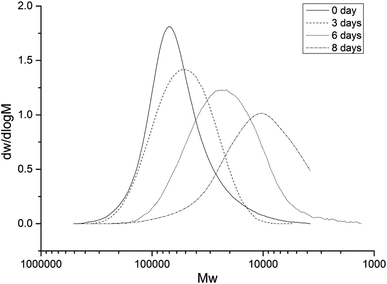 |
| Fig. 6 Molecular weight distribution of PVA 1788 in the semi-synthetic medium as recorded at different incubation times. | |
3.4 FTIR characterization
FTIR spectra analysis was performed to study the structural changes of PVA 1788 at different culture times. Compared with unbiodegraded PVA 1788 (0 day), the degraded PVA 1788 (at 3 days, 6 days, and 8 days) exhibited some differences in the infrared spectrum, as shown in Fig. 7. An absorption peak at 1715 cm−1 was observed, which represents the carbonyl group, indicating an increase in macromolecule unsaturation. The stretching vibration band of the hydroxyl group (3700–3000 cm−1) became widened and less peaked, and superimposed with the νCH stretching vibration band, which appeared at the tail of the νOH stretching vibration peak. These results indicated that some substances containing carbonyl groups (likely aldehydes or ketones) might have been produced during the biodegradation of PVA 1788, which was in accordance with the literature data.32,33 Proposed mechanisms of PVA biodegradation reported until now are mainly based on two steps: hydroxyl groups of PVA are firstly oxidized to β-diketone structures, and then C–C bonds at diketone structures are cleaved by hydrolysis. Formation of compounds containing carbonyl groups may indicate that related PVA-degrading enzymes of the fungus may act on the hydroxyl groups in PVA by oxidation or dehydrogenation, leading to the formation of carbonyl groups during the degradation. It has been reported that after microorganisms invade material, they can release enzymes that accelerate the degradation of polymers by destroying the stability of the internal structure.34,35 It could be explained that the hyphae of fungus Eutypella sp. BJ may penetrate into the interior of PVA 1788 and cause a chemical breakdown.
 |
| Fig. 7 FTIR spectra of PVA 1788 degradation at different incubation times. | |
4. Conclusions
The present study explored the potential of a novel fungus (Eutypella sp. BJ) in the biodegradation of PVA. From the results, Eutypella sp. BJ showed little difference in degradation between PVA 1788 and 1799, which have different degrees of saponification, and it exhibited relatively poor degradation ability for PVA 2488, which has a high degree of polymerization. The GPC analysis showed that Eutypella sp. BJ can assimilate PVA, decreasing its molecular weight and increasing its molecular weight distribution. The FTIR analysis indicated that some substances containing carbonyl groups may be produced during the degradation process. Unexpectedly, we discovered that Eutypella has never been reported to have the ability to degrade PVA so far, which may has promising application in the assimilation of macromolecular pollutants in the environment. With regard to research on PVA degradation, further work is needed to study its biochemistry and physiology: what enzymes and related genes have acted on the biodegradation of PVA? What are the biochemical pathways of PVA degradation by fungi? In addition, the production of industrial enzyme preparations is also an efficient strategy for the biological treatment of polymer wastes.
Conflicts of interest
All contributing authors declare that they have no conflicts of interest.
Acknowledgements
This research was financially supported by the Joint Funds of the National Natural Science Foundation of China (research project no. U1301231).
References
- E. Chiellini, A. Corti, G. D. Sarto and S. D'Antone, Oxo-biodegradable polymers-effect of hydrolysis degree on biodegradation behaviour of poly(vinyl alcohol), Polym. Degrad. Stab., 2006, 91, 3397–3406 CrossRef CAS.
- F. F. Nord, Über die Dehydrierungsleistungen von fusarium lini B, Naturwissenschaften, 1936, 24, 763 CrossRef CAS.
- T. Suzuki, Y. Ichihara, M. Yamada and K. Tonomura, Some Characteristics of Pseudomonas O-3 which Utilizes Polyvinyl Alcohol, J. Agric. Chem. Soc. Jpn., 2008, 37, 747–756 Search PubMed.
- Y. Watanabe, M. Morita, N. Hamada and Y. Tsujisaka, Formation of Hydrogen Peroxide by a Polyvinyl Alcohol Degrading Enzyme, Agric. Biol. Chem., 1975, 39, 2447–2448 CAS.
- T. Hatanaka, N. Asahi and M. Tsuji, Purification and Characterization of Poly(vinyl alcohol) Dehydrogenase from Pseudomonas sp. 113P3, Biosci., Biotechnol., Biochem., 1995, 59, 1813–1816 CrossRef CAS.
- M. Morita, N. Hamada, K. Sakai and Y. Watanabe, Purification and Properties of Secondary Alcohol Oxidase from a Strain of Pseudomonas, Agric. Biol. Chem., 1979, 43, 1225–1235 CAS.
- D. Jendrossek, in Biopolyesters, ed. W. Babel and A. Steinbüchel, Springer, Heidelberg, 2001, vol. 71, ch. 10, pp. 293–325 Search PubMed.
- M. Amann and O. Minge, Synthetic Biodegradable Polymers, in Advances in Polymer Science, ed. B. Rieger, A. Künkel, G. W. Coates, R. Reichardt, E. Dinjus and T. A. Zevaco, Springer, Heidelberg, 2011, ch. 5, vol. 245, pp. 137–172 Search PubMed.
- M. Shimao, S. Kishida, S. Harayama and T. Tamogami, The gene pvaB encodes oxidized polyvinyl alcohol hydrolase of Pseudomonas sp. strain VM15C and forms an operon with the polyvinyl alcohol dehydrogenase gene pvaA, Microbiology, 2000, 146, 649–657 CrossRef CAS PubMed.
- S.-i. Tsujiyama, T. Nitta and T. Maoka, Biodegradation of polyvinyl alcohol by Flammulina velutipes in an unsubmerged culture, J. Biosci. Bioeng., 2011, 112, 58–62 CrossRef CAS PubMed.
- S. Tsujiyama and A. Okada, Biodegradation of polyvinyl alcohol by a brown-rot fungus, Fomitopsis pinicola, Biotechnol. Lett., 2013, 35, 1907–1911 CrossRef CAS PubMed.
- G. A. I. Mejía, O. B. L. López and P. A. Mulet, Biodegradation of poly(vinyl alcohol) with enzymatic extracts of phanerochaete chrysosporium, Macromol. Symp., 2015, 148, 131–147 CrossRef.
- Q. Ding, G. Du and J. Chen, Isolation and Culture Characterization of a New Polyvinyl Alcohol-Degrading Strain: Penicillium sp. WSH02-21, World J. Microbiol. Biotechnol., 2004, 20, 587 CrossRef.
- L. Jecu, A. Gheorghe, A. Rosu, I. Raut, E. Grosu and M. Ghiurea, Ability of Fungal Strains to Degrade PVA Based Materials, J. Polym. Environ., 2010, 18, 284–290 CrossRef CAS.
- L. D. C. Rj, C. Gby and G. T. Lonergan, Enhanced degradation of polyvinyl alcohol by Pycnoporus cinnabarinus after pretreatment with Fenton's reagent, Appl. Environ. Microbiol., 1999, 65, 1798–1800 Search PubMed.
- T. Mori, M. Sakimoto, T. Kagi and T. Sakai, Enantioselective Oxidation of Diols by Secondary Alcohol Dehydrogenase from Geotrichum sp. WF9101, Biosci., Biotechnol., Biochem., 1996, 60, 1191–1192 CrossRef CAS PubMed.
- T. Mori, M. Sakimoto, T. Kagi and T. Sakai, Isolation and Characterization of a Strain of Bacillus megaterium That Degrades Poly(vinyl alcohol), Biosci., Biotechnol., Biochem., 1996, 60, 330–332 CrossRef CAS PubMed.
- T. Mori, M. Sakimoto, T. Kagi and T. Sakai, Degradation of Vinyl Alcohol Oligomers by Geotrichum sp. WF9101, Biosci., Biotechnol., Biochem., 1996, 60, 1188–1190 CrossRef CAS PubMed.
- T. Mori, M. Sakimoto, T. Kagi and T. Sakai, Secondary alcohol dehydrogenase from a vinyl alcohol oligomer-degrading Geotrichum fermentans; stabilization with Triton X-100 and activity toward polymers with polymerization degrees less than 20, World J. Microbiol. Biotechnol., 1998, 14, 349–356 CrossRef CAS.
- F. Kawai and X. Hu, Biochemistry of microbial polyvinyl alcohol degradation, Appl. Microbiol. Biotechnol., 2009, 84, 227 CrossRef CAS PubMed.
- R. Lipsa, N. Tudorachi, V. C. Grigoraş and C. Vasile, Degradation of poly(vinyl alcohol)-graft-lactic acid copolymers by Trichothecium roseum fungus, J. Appl. Polym. Sci., 2015, 132 DOI:10.1002/app.41777.
- Y. Liu, Y. Deng, P. Chen, M. Duan, X. Lin and Y. Zhang, Biodegradation analysis of polyvinyl alcohol during the compost burial course, J. Basic Microbiol., 2019, 59, 368–374 CrossRef CAS PubMed.
- G. P. Rédei, in Encyclopedia of Genetics, Genomics, Proteomics and Informatics, Springer, Dordrecht, 3rd edn, 2008, ch. 3187, DOI:10.1007/978-1-4020-6754-9_3188.
- M. Isaka, S. Palasarn, W. Prathumpai and P. Laksanacharoen, Pimarane Diterpenes from the Endophytic Fungus Eutypella sp BCC 13199, Chem. Pharm. Bull., 2011, 59, 1157–1159 CrossRef CAS PubMed.
- X. L. Wang, K. L. Sun and B. Wang, Bioactive Pimarane Diterpenes from the Arctic Fungus Eutypella sp D-1, Chem. Biodivers., 2018, 15, 7 Search PubMed.
- Y. Zhou, Y.-X. Zhang, J.-P. Zhang, H.-B. Yu, X.-Y. Liu, X.-L. Lu and B.-H. Jiao, A new sesquiterpene lactone from fungus Eutypella sp. D-1, Nat. Prod. Res., 2017, 31, 1–6 CrossRef PubMed.
- J.-B. Lee, S.-Y. Park, K. Shin, C.-P. Jeon, J.-E. Kim and G.-S. Kwon, Biodegradation of Organochlorine Insecticide Endosulfan by the Fungus Eutypella sp. KEF-1, Korean Journal of Environmental Agriculture, 2011, 30, 202–208 CrossRef.
- T. Suzuki, Y. Ichihara, M. Yamada and K. Tonomura, Some Characteristics of Pseudomonas O-3 which Utilizes Polyvinyl Alcohol, Agric. Biol. Chem., 1973, 37, 747–756 CAS.
- R. Solaro, A. Corti and E. Chiellini, Biodegradation of poly(vinyl alcohol) with different molecular weights and degree of hydrolysis, Polym. Adv. Technol., 2000, 11, 873–878 CrossRef CAS.
- E. Chiellini, A. Corti and R. Solaro, Biodegradation of poly(vinyl alcohol) based blown films under different environmental conditions, Polym. Degrad. Stab., 1999, 64, 305–312 CrossRef CAS.
- T. Hatanaka, T. Kawahara, N. Asahi and M. Tsuji, Effects of the Structure of Poly(vinyl alcohol) on the Dehydrogenation Reaction by Poly(vinyl alcohol) Dehydrogenase from Pseudomonas sp. 113P3, Biosci., Biotechnol., Biochem., 1995, 59, 1229–1231 CrossRef CAS.
- R. Solaro, A. Corti and E. Chiellini, Biodegradation of poly(vinyl alcohol) with different molecular weights and degree of hydrolysis, Polym. Adv. Technol., 2000, 11, 873–878 CrossRef CAS.
- M. Ullah, C. H. Weng, H. Li, S. W. Sun, H. Zhang, A. H. Song and H. Zhu, Degradation of polyvinyl alcohol by a novel bacterial strain Stenotrophomonas sp. SA21, Environ. Technol., 2017, 39, 1–20 Search PubMed.
- E. Chiellini, A. Corti, S. D'Antone and R. Solaro, Biodegradation of poly(vinyl alcohol) based materials, Prog. Polym. Sci., 2003, 28, 963–1014 CrossRef CAS.
- H. C. Flemming, Relevance of biofilms for the biodeterioration of surfaces of polymeric materials, Polym. Degrad. Stab., 1998, 59, 309–315 CrossRef CAS.
|
This journal is © The Royal Society of Chemistry 2019 |
Click here to see how this site uses Cookies. View our privacy policy here.