DOI:
10.1039/C9RA02258A
(Review Article)
RSC Adv., 2019,
9, 17921-17932
Metastasis manners and the underlying mechanisms of ALK and ROS1 rearrangement lung cancer and current possible therapeutic strategies
Received
24th March 2019
, Accepted 1st June 2019
First published on 7th June 2019
Abstract
The rearrangements of anaplastic lymphoma kinase (ALK) and the c-ros oncogene 1 (ROS1) have both been important driving factors in non-small-cell lung cancer (NSCLC). They have already been defined in 3–5% of NSCLC patients. ALK and ROS1 rearrangements are associated with unique clinical and pathological features, especially patients are usually younger, with milder or never smoking history, and adenocarcinoma histology. Also, they have both been found to contribute to the metastasis of NSCLC by cell migration and invasion. It has recently been recognized that the brain can be considered as a primary site for metastasis in cancers with ALK or ROS1 rearrangements. The present review summarizes the current status of NSCLC metastasis and possible mechanisms based on available evidence, and then we list possible therapeutic strategies so that an increase in control of ALK and ROS1 rearrangement of NSCLC metastases by combination therapy can be translated in an increase in overall survival and prognosis.
1. Introduction
Compared with other types of cancer, lung cancer has the highest morbidity and mortality.1 Cancer metastasis is a complex process with multiple processes that make it one of the most difficult aspects to understand in oncology. Metastatic disease has become the main reason for the low survival rate of non-small-cell lung cancer (NSCLC). Therefore, it is important to understand the NSCLC metastasis process and its potential invasion and migration pathways.2,3 Identifying targets that inhibit lung cancer invasiveness and metastasis will help develop new anti-lung cancer strategies.
Approximately 20–25% of advanced NSCLC, particularly adenocarcinoma subtypes, have viable carcinogenic drive mutations.4 Activating mutations or translocations of the anaplastic lymphoma kinase gene (ALK) have been identified in several types of cancer, including NSCLC.5 An additional receptor tyrosine kinase (RTK) recently identified to be involved in recurring gene rearrangements in NSCLC is c-ros oncogene 1 (ROS1), an orphan receptor whose physiologic functions are still poorly understood.6 ROS1-positive patients, representing approximately 1–2% of NSCLC cases, tend to possess typical clinicopathologic features similar to those described for ALK-positive NSCLC patients.6–8 One study showed that in Caucasian patients, the common genetic alteration in advanced NSCLC was KRAS mutations in approximately 29% of patients, epidermal growth factor receptor (EGFR) mutations accounting for approximately 11%, ALK rearrangements accounting for approximately 5%,9 and mesenchymal–epithelial transition (MET) mutations (exon 14) accounted for 4%,4 and ROS1 rearrangement accounted for 1%.8
According to Pan et al., in patients with NSCLC who are positive for ALK or ROS1, bone metastasis accounted for 43%, intrapulmonary lesions accounted for 37%, pleura metastasis accounted for 30%, and brain metastasis accounted for 21%. These four metastases are the most common in NSCLC.10 Another study showed that NSCLC patients aged 40 years or younger accounted for about 4% of the total number of NSCLC, of which 54.5% were women, 86.1% of patients were with adenocarcinoma, and 72.5% of patients were non-smokers.2 About 35.7% of patients with stage IV NSCLC had central nervous system (CNS) metastasis, and about 31.5% had bone metastases. For patients with ALK-positive mutations, overall survival (OS) was 9.8 months, and patients with ALK-negative mutations had an OS of 5.6 months.2 These data suggest that it is necessary to summarize the data on ALK- or ROS1-positive NSCLC metastases, analyze the metastases mechanism, and propose some constructive treatment strategies.
2. Metastasis manners of ALK and ROS1 rearrangement NSCLC
2.1 Metastasis in the CNS
In NSCLC patients with ALK rearrangements, the CNS appears to be one of the most common metastatic sites during the patient's treatment, such as the brain, pia mater, and spinal cord.11 NSCLC brain metastases are generally considered to be the final stage of advanced disease and an ominous sign of disease progression and death,12 and the incidence of brain metastases is as high as 50%,13,14 whereas the incidence of spinal cord metastases is 0.4%. Of note, 35–50% of ALK-positive patients were found to have brain metastases when participating in one study.11,15–17 And previous reports have described a high proportion of CNS metastases in Latin American patients at diagnosis.18 Moreover, the brain metastasis of ALK- or ROS1-positive NSCLC has not been significantly improved after treatment with drugs such as crizotinib, which is closely related to the difficulty in crossing the blood–brain barrier (BBB).19 Table 1 provides a summary of recent clinical trials for the treatment of ALK/ROS1 positive NSCLC brain metastases.
Table 1 Treatment of ALK/ROS1 positive NSCLC brain metastasis with TKIs-summary of the latest clinical trialsa
Agent |
Molecular targets |
Clinical trials. gov identifier |
Patients |
Clinical stage |
Dosage |
Complete intracranial response rate (%) |
Intracranial PFS |
Reference |
PFS, progression-free survival; ALK, anaplastic lymphoma kinase; ROS1, c-ros oncogene 1; MET, mesenchymal–epithelial transition. |
Crizotinib |
ALK/ROS1/MET |
NCT01154140 (PROFILE 1014) |
39 |
Phase III trial |
250 mg per os twice daily |
12 weeks: 85%, 24 weeks: 56% |
9 months |
77 |
Ceritinib |
ALK/ROS1 |
NCT01828099 (ASCEND-4) |
54 |
Phase III trial |
750 mg per os daily |
24 weeks or longer: 46.3% (n = 54) |
10.7 months (n = 189) |
141 |
Alectinib |
ALK |
NCT02075840 (J-ALEX) |
15 |
Phase III trial |
300 mg per os twice daily |
33% |
26.3 months |
117 and 144 |
Brigatinib |
ALK/ROS1 |
NCT02094573 |
73 |
Phase II trial |
180 mg per os daily |
— |
18.4 months |
145 |
Lorlatinib |
ALK/ROS1 |
NCT01970865 |
81 |
Phase II trial |
100 mg per os daily |
20% |
— |
146 |
Ensartinib |
ALK/ROS1 |
NCT01625234 |
14 |
Phase I/II trial |
225 mg per os daily |
57% |
16.6 months |
120 |
2.1.1 Leptomeningeal carcinomatosis (LC). LC is also known as leptomeningeal metastasis (LM). It occurs in approximately 3.8% of patients with unselected NSCLC,20 and is seen in about 5% of patients with ALK rearrangement. Since the discovery of LC, its mechanism has not been clearly elucidated.21 There are a variety of ways by which cancer cells can invade the leptomeninges: it can be invaded directly by cells adjacent to the spinal and cranial nerves,22 also, the cells involved in the venous blood circulation can enter the space through the Batson plexus,23 and those within the arterial circulation can go across the choroid plexus to enter the leptomeninges.22 These cancer cells proliferate in suspension and they are in direct contact with pia mater, which is a thin mesenchymal tissue layer covering the nerve axis, including the spinal cord and root. Once identified, pial membrane metastasis may invade the parenchyma and produce focal nerve damage.24 In a previous study, it was observed that patients with LC usually had very poor prognosis, and neither chemotherapy nor targeted therapy was able to significantly improve the outcomes. The median survival of untreated patients was only 4–6 weeks.25
2.1.2 Intradural extramedullary spinal cord metastases (IESCM) and intradural spinal cord metastases (ISCM). NSCLC often metastasizes to the intracranial central nervous system, but rarely metastasizes to the spinal cord, which occurs in only about 2% of NSCLC patients with less than 150 cases described in the medical case literatures.26 According to previous findings, the spinal cord metastasis may have been caused by dissemination and seeding via the cerebrospinal fluid.26–28IESCM, also known as spinal cord pial metastases, are a special clinical manifestation of LC-related spinal cord involvement.29,30 Tumor cells spread and spread in the subarachnoid space outside the spinal cord, resulting in a series of symptoms of spinal cord and spinal nerve root involvement, manifested as metastatic spinal cord compression syndrome. The incidence of such metastasis is low, but the clinical symptoms of patients are heavy. Once they occur, the quality of life of patients is seriously affected, and the prognosis is extremely poor.31
Like LC, ISCM is a rare disease, but ISCM is common in lung cancer and breast cancer metastasis, while cervical, thoracic and lumbar spine are also affected. In NSCLC, ISCM occurs in 2–4.2% of patients.11,32,33 Justin and colleagues recently reported the ISCM in the first ALK-positive NSCLC patient.11 In their study, 3 out of 96 other ALK-positive patients were also diagnosed with ISCM, but LC was not found in these patients. The average time from diagnosis of NSCLC to ISCM in all patients exceeded 15 months, indicating that the longer the survival period, the greater the risk of developing ISCM.11
Most reports are retrospective and limited in scale, so there is no uniform conclusion on the best treatment for patients with ISCM.32,33 In general, management strategies already include radiation therapy, surgery resection, chemotherapy, or a combination of the above methods.33 For patients with intramedullary tumors, ISCM patients had the worst results, with a median survival of approximately 4 months from the time of diagnosis.34
2.2 Bone metastasis
Kuijpers et al. in their study identified 784 KRAS+, 160 EGFR+ (exon 19del, exon 21 L858R), 42 ALK+ and 1008 triple negative tumors. From this study, they reported that the bone was the most common metastatic organ (34%).35 Skeleton is one of the common metastatic sites of lung cancer. Bone metastasis of lung cancer interferes with local bone homeostasis, reduces bone formation or/and increases bone resorption.36,37 Patients with lung cancer and bone metastases have a poor prognosis with a median survival of less than 1 year.38 The mechanism of NSCLC bone metastasis is not fully understood, but there is evidence that lung cancer cells invade the bone surface and through the secretion of certain growth factors or cytokines, thereby destroy the steady state of the bone.39
Receptor Activator of Nuclear Factor-κ B Ligand (RANKL) plays a critical role in the formation, survival, and function of osteoclasts. Previous studies showed that RANKL is very important for the processes leading to bone metastases proceeded to skeletal destruction.40 The RANK receptor is responsible for RANKL functions, it is expressed on osteoclasts and osteoclast precursors, and is inhibited by the soluble decoy receptor osteoprotegerin (OPG).41,42 Using diverse signals including interleukin (IL)-1β, IL-6, IL-8, IL-11, IL-17, macrophage inflammatory protein-1 alpha (MIP1α), tumor necrosis factor-α, parathyroid hormone-related protein (PTHrP), and prostaglandin E2 (PGE2) metastatic tumor cells cause an up-regulation of RANKL within bone stromal cell.43 Subsequently, in response to PTHrP, IL-1, PGE2, Dickkopf-related protein 1 (DKK-1) or epidermal growth factor (EGF), tumor involvement in bone can also down-regulate local OPG levels in stroma and osteoblasts.44 In any case, an increase in the ratio of RANKL to OPG in the local bone microenvironment can lead to invasive osteolytic bone destruction.
Some preclinical studies have demonstrated that the inhibition of RANKL leads to a significant reduction of bone tumor burden. Moreover, the combination of RANKL inhibitors with chemotherapy not only targets the bone environment to treat NSCLC bone metastases but also supports further clinical evaluation, thus establishing that the combinatorial approach is better than the use of either drug alone.45
2.3 Lymph nodes metastasis
Lymph nodes (LNs) are common site of metastasis and it is important for triggering an anti-tumor immune response. Therefore, cancer cells must evade the immune response before they can be transferred to LNs.46 The process of lymphatic metastasis begins with the orchestration of lymphangiogenesis and the preparation of a premetastatic niche.46 A premetastatic niche is a LN microenvironment favorable for tumor growth. Cancer cells then invade tumor-associated lymphatic vessels at the primary site on their way to tumor-draining LNs (TDLNs), where they survive and grow.46
The mechanism by which LN lymphangiogenesis results in metastasis has not yet been identified, but one hypothesis suggests that increased lymph flow which is an effect of LN lymph angiogenesis may lead to a more efficient delivery of cancer cells to LNs and distant organs, and the expression of both vascular endothelial growth factor (VEGF)-C and VEGF-D have been linked with increased LN metastasis.46 A study conducted by Zhang et al. showed that the frequency of lung cancer patients with lymph node metastasis expressing ALK and ROS1 fusions was 10.7% and 1.8% respectively.47
Gao et al. analyzed ALK fusions from paired primary and metastatic lymph node tumors in 78 lung cancer patients who had not received small molecular tyrosine kinase inhibitors (TKIs), and proved that the ALK fusions of primary lung cancers and those in corresponding metastatic lymph node tumors are highly consistent.48
2.4 Ovarian metastasis
Ovarian metastasis from lung cancer is extremely rare, approximately 0.3–0.4% of metastatic ovarian tumors metastasize from lung cancer, and about 33% of the lung carcinomas that metastasize to the ovary are adenocarcinomas.49–51 Ovarian metastasis has been observed to be more common among young women with lung cancer, this is a feature usually common in ALK- and ROS1-positive patients. A few cases of ovarian involvement from ALK-positive NSCLCs have been reported. Jing and colleagues presented a case of a 41 year-old woman who was initially diagnosed with NSCLC adenocarcinoma on histology.52 After receiving a 6-course first-line chemotherapy and 8-course maintenance therapy, emergency surgery was performed and the resected tissues were sent for pathological evaluation and immunohistochemistry (IHC) analysis. The pathological diagnosis of the resected ovarian was metastatic pulmonary adenocarcinoma and a positive indication for ALK rearrangement was found in a metastatic ovarian lesion. Hence, it was deduced that specimens from metastatic lesions and primary tumors are equally suitable for use in detecting ALK rearrangement for therapeutic strategies in patients with advanced NSCLC. After the detection of ALK rearrangement, crizotinib was administered, and the disease remained stable after 10 months of crizotinib therapy.52
Although several cases of ovarian metastasis involving ALK rearrangement have been presented, only one case of bilateral ovarian metastasis from ROS1 translocated lung adenocarcinoma has been reported so far. Franchina et al. presented a case of a 43 years old never smoking woman diagnosed with metastatic lung adenocarcinoma.53 Transvaginal ultrasound indicated the presence of dysfunctional cysts in both ovaries. After undergoing EGFR, KRAS and ALK testing, no actionable mutation was found and she commenced first-line chemotherapy with cisplatin-pemetrexed. After 19 months FISH assay detected the presence of ROS1 rearrangement. They reported a lengthy progression-free survival (PFS) on pemetrexed-based chemotherapy, but after re-staging and pathological confirmation of ROS1 translocation, she enrolled in a phase II study of crizotinib.
2.5 Choroidal metastasis
Lung cancer has been reported to metastasize to the eye in 0.2% to 7% of patients based on clinical studies.54 There is abundant blood supply to the choroid, this may present a suitable microenvironment for cancer cell growth. Thus, the choroid is the most common ocular tissue affected by metastatic disease.55,56 Lu and colleagues reported a case of a patient with ROS1-rearranged NSCLC that presented with choroidal metastases and did not respond to initial chemotherapy but had a rapid and complete response to crizotinib.57 Feng and colleagues reported a similar case in an ALK-positive patient.58 However, another study presents a case where the metastatic tumor was first responsive to crizotinib and a decrease in tumor size and improved vision was observed.59 The condition was stable until the 16th month of crizotinib therapy when a new superior metastasis was detected. Crizotinib therapy was continued for 2 weeks and then discontinued after the patients' vision worsened. Treatment with the second generation anti-ALK agent, brigatinib was initiated. After brigatinib treatment, the patient's ocular symptoms were resolved and vision improved.59 Also, the second generation ALK inhibitors alectinib and ensartinib have shown to be effective against of crizotinib resistant choroidal metastasis in patients with ALK NSCLC.56,59
2.6 Other metastasis
Most incidence of NSCLC is accompanied by multiple metastases (pleura, adrenal gland, muscle, bone, liver). This is also reflected in the pathology of patients with ALK-positive NSCLC.60 An increase in the incidence of liver metastases in ALK-positive patients was recently reported.61,62 Mikes et al. observed a higher incidence of pericardial, pleural, and liver metastasis in ALK-positive patients compared to patients without an EGFR, KRAS or ALK oncogene abnormality.63 Beside that Di Ma et al. analyzed the clinicopathological features and treatment outcomes of 52 patients with ALK-positive advanced NSCLC admitted to the Oncology Department of the Cancer Hospital of the Chinese Academy of Medical Sciences. They reported that pleural metastases occurred in 36.5% of patients.64 But no reports of relevant mechanisms were found.
3. The metastasis mechanisms of ALK and ROS1 rearrangement NSCLC
A novel circRNA F-circEA-2a produced from the EML4-ALK fusion gene which is mainly located in the cytoplasm was identified, although F-circEA-2a does not affect the proliferation of NSCLC cells, it can significantly affect the migration and invasion of cancer cells.65 It has been reported that metformin increases cell sensitivity to crizotinib by inhibiting cell proliferation and invasion to promote apoptosis, which is likely to be related to down-regulation of insulin-like growth factor 1 receptor (IGF-1R) signaling.66 Epithelial–mesenchymal transition (EMT) is closely related to the invasion and metastasis of cancer cells, previous findings suggest that EMT was acquired during ALK inhibitor therapy.67 And this process is related to EMT-related proteins E-cadherin and vimentin.68 As another major regulator of EMT, slug is up-regulated by up-regulation of hypoxia inducible factor (HIF)-1α during hypoxia. Expression of EML4-ALK also increases expression of EMT-inducing transcription factors, snail and slug, suggesting that EMT is ongoing.69 And blocking extracellular regulated protein kinases (ERK) 1/2 can antagonize cell migration mediated by EML4-ALK.70 An and colleagues have shown that Crk-like protein (CRKL) is a key downstream response factor for ALK. Although knockdown of CRKL has no effect on ALK phosphorylation and expression in cells, it hinders the expression of the downstream genes RAS and RAC1 of ALK, which leads to a decrease in cell viability and migration ability.71 In addition, studies have shown that by directly targeting zinc finger E-box-binding homeobox 1 (ZEB1) and indirectly up-regulating E-cadherin, high expression of miR-200c significantly inhibits proliferation, migration and invasion of NSCLC cells.72
In the study of invasion and metastasis of the high expression of ALK or ROS1 NSCLC, Jun et al. observed that ROS1 cells expressing the CD74-ROS1 fusion were highly invasive in vitro and metastatic in vivo. Expression of CD74-ROS1 resulted in the phosphorylation of the extended synaptotagmin-like protein E-Syt1.73 A recent study conducted by our group further demonstrated that CD74-ROS1 or CD74-ROS1 G2032R mutations induce cell EMT in A549 cells by constructing an A549-CD74-ROS1 crizotinib-resistant cell line with the G2032R mutation. This promotes migration and increases expression of matrix metalloproteinase (MMP)-9 and Twist1 transcription factors.74 Fig. 1 summarizes possible pathways for ALK/ROS1 positive NSCLC invasion and metastasis.
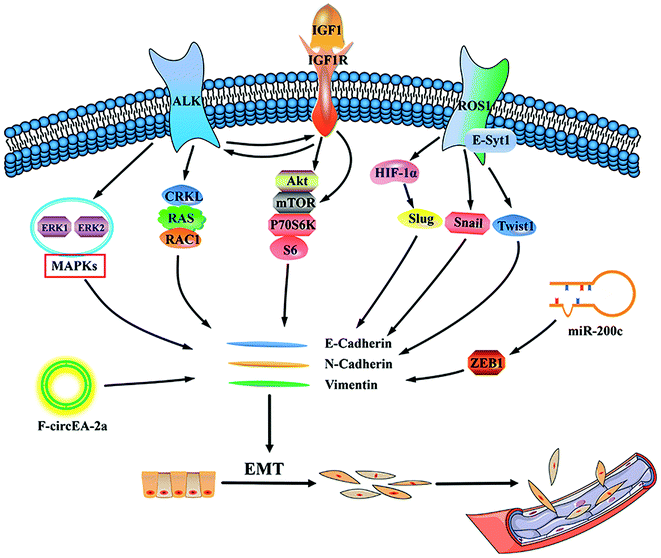 |
| Fig. 1 Diagram of the possible pathways for ALK/ROS1 rearrangement NSCLC invasion and metastasis. IGF1, insulin-like growth factor 1; IGF1R, insulin-like growth factor 1 receptor; ERK, extracellular signal-regulated kinases; MAPK, mitogen-activated protein kinases; CRKL, Crk-like protein; RAS, RAC1 (downstream genes of ALK); mTOR, mammalian target of rapamycin; E-Syt1, extended synaptotagmin-like protein 1; HIF-1α, hypoxia inducible factor-1α; ZEB1, zinc finger E-box-binding homeobox 1; EMT, epithelial–mesenchymal transition. | |
4. Conventional treatments
The side effects caused by conventional chemotherapy make it very limited in controlling ALK- or ROS1-positive NSCLC metastasis compared to targeted therapy and immunotherapy. Due to cumulative toxicity, patients often fail to receive multiple courses of conventional chemotherapy including platinum-based chemotherapy, pemetrexed chemotherapy or both, while targeted therapy and immunotherapy can be more easily maintained.75 Increasing the chemotherapy cycle can significantly prolong progression-free survival (PFS), but the OS cannot be improved, while adverse reactions increase, and quality of life declines.76 The PROFILE 1014 phase III study reported a median PFS of 7.0 months in patients receiving conventional chemotherapy (pemetrexed plus cisplatin carboplatin), which was significantly lower than patients receiving crizotinib with a median survival of 10.9 months.77 In patients who received bevacizumab plus platinum-containing dual-drug chemotherapy in the first-line, and bevacizumab plus pemetrexed for dual-drug maintenance therapy prolonged PFS compared with bevacizumab monotherapy maintenance therapy.78 Even if maintenance treatment can prolong PFS, OS cannot be prolonged and the quality of life cannot be improved.79
5. Treatments targeting metastasis of ALK and ROS1 rearrangement NSCLC
5.1 Crizotinib
Crizotinib (Xalkori, PF-02341066) is an oral small-molecule inhibitor which can inhibit the tyrosine kinases ALK, ROS1, and MET factor and it was also the first direct ALK inhibitor tested in humans with ALK rearrangement-positive lung cancer.80,81 Reports on the use of crizotinib for CNS metastasis are contradictory and the mechanisms involved are not fully understood.82–84
However, in the PROFILE 1014 phase III study, a comparison study between crizotinib and chemotherapy85 showed that intracranial efficacy was prospectively assessed using protocol-specified brain imaging at baseline in patients with brain metastasis, crizotinib showed a clinically and statistically significant improvement in efficacy compared to platinum-pemetrexed chemotherapy.77,86 As a result of this study, crizotinib was approved as first-line agent by the FDA in 2013.87,88 In another study, crizotinib had less effect on the control of CNS metastasis, this is mainly due to the role of the BBB, because crizotinib is a known P-glycoprotein substrate that can be excreted by the BBB.89 Toyokawa et al. also observed that the concentration of crizotinib in cerebrospinal fluid was extremely low,90 thus suggesting that crizotinib may not reach the therapeutic concentration that should be found in the CNS. Takeda et al. reported that isolated radiotherapy of CNS lesions in patients whose disease had progressed on crizotinib led to favorable outcomes following resumption of crizotinib therapy, indicating that a combination of isolated radiotherapy with crizotinib therapy may improve the prognosis of patients with CNS metastases.9
Zhang et al. reported a case in which the use of crizotinib combined with surgery, whole brain radiotherapy (WBRT) and residual focus supplemental radiotherapy for the treatment of ROS1-positive lung adenocarcinoma with symptomatic brain metastases was successful.91 If the concentration of crizotinib in cerebrospinal fluid can be monitored regularly, crizotinib combined with WBRT may be an alternative strategy for CNS metastasis in patients with ROS1-positive NSCLC.92
Crizotinib has proven to be effective against LC because disruption of the BBB with WBRT results in elevated concentrations of crizotinib in the cerebrospinal fluid.93 The OS of patients treated in this manner is approximately ∼5 years.92 There have even been reports of profound effects of ALK inhibitors on EML4-ALK-positive lung cancer patients, and almost all bone metastases and lymph node metastases disappeared shortly after crizotinib administration.94
5.2 Ceritinib
Ceritinib (Zykadia, LDK378) is a second-generation, small-molecule, ATP-competitive ALK tyrosine kinase inhibitor (TKI). It is structurally different from crizotinib and has proven to be 20 times more effective than crizotinib.95 Ceritinib is effective in patients with metastatic NSCLC including but not limited to brain metastasis. It showed significant CNS activity96 compared to the first-generation ALK inhibitor. Its anti-brain metastasis effect in ALK-positive NSCLC, suggests it has a better BBB penetrating ability. Ceritinib is known to have 15% CSF penetration.97 This is also verified in ASCEND-1 Trial (#8003).96
Moreover, studies have shown that ceritinib can prolong PFS in patients. This study envisions the application of ceritinib in ROS1 rearranged NSCLC patients.98 Ceritinib also showed excellent anti-IESCM activity in patients with ALK-positive NSCLC.99 Although there is a current lack of case support, but evidence from pathology reports and some preclinical studies, suggest that second-generation ALK inhibitors, especially ceritinib, may be a good choice for treating LC.97 It is worth noting that ceritinib may be highly effective in patients whose disease has progressed on crizotinib and has metastasized regardless of where the metastatic site is.100
5.3 Alectinib
Alectinib (CH5424802/RO5424802) is a highly selective second-generation ALK inhibitor. In the phase I/II trial in the United States, alectinib has significant activity on brain metastases of ALK rearranged NSCLC with an initial total response rate of 52%.101–103 In November 2017, the FDA approved the use of alectinib for first-line treatment of patients with ALK-positive metastatic NSCLC.104 This is based on the 3rd clinical trial test (ALEX). In the ALEX trial, 45% of patients in the crizotinib-treated group developed CNS progression and this number is only 12% in the alectinib-treated group.105
When it comes to LC or ISCM, a large number of experimental conclusions tells us that alectinib has a beneficial effect on CNS lesions.96,101,106–108 In the report by Justin et al., alexinib showed significant anti-tumor activity in ALK-positive LC patients. In addition, they found that alectinib had a good control of patients with recurrent CNS disease after treatment with crizotinib and ceritinib.106 Alectinib's phase II global study demonstrated its effective clinical activity in patients with advanced ALK-positive NSCLC. Alectinib also led to a reduction in CNS metastasis, and CNS objective response rate in patients with CNS metastases is 57%, and the CNS complete response rate is 27%.107
Therefore, as a more effective ALK receptor inhibitor, its real therapeutic advantage lies in the treatment of CNS metastatic disease. Its mechanism is that, unlike other ALK inhibitors, alectinib is not a substrate for P-glycoprotein, so it can reach higher concentrations in the cerebrospinal fluid.102 Unfortunately, there are few reports on the treatment of ROS1-positive NSCLC with alectinib.
5.4 Brigatinib
The targeted therapy drug brigatinib (Alunbrig™) for patients with metastatic NSCLC was granted accelerated approve by the US Food and Drug Administration (FDA) in April 2017.109 In patients with ALK-positive NSCLC who had not previously received an ALK inhibitor, PFS was significantly longer among patients who received brigatinib than among those who received crizotinib. In a phase 3 trial, brigatinib, as compared with crizotinib, had superior efficacy against systemic and intracranial disease.110 So brigatinib is another second-generation ALK inhibitor that has enhanced antitumor activity and broad anti-mutation activity compared to other second-generation ALK inhibitors.111
Importantly, according to the phase I/II trial results, patients with brain metastases had an objective response rate of 67% and a median progress free survival of 15.6 months or even higher, indicating that brigatinib may have excellent CNS activity.112
5.5 Lorlatinib
Lorlatinib (PF-06463922), a third-generation small molecule TKI, is a novel, reversible, ATP-competitive inhibitor of ALK and ROS1. Inhibition acts by mediating the disruption of the signaling pathways of ALK and ROS1.113 Lorlatinib has been approved for the treatment of ALK-positive NSCLC in the United States and Europe.114 It is important that lorlatinib also has a good effect on patients with brain metastases through the BBB.115 Animal studies have shown that lorlatinib is more effective against CNS lesions than alectinib.115 Clinical studies showed that at any time point, the cumulative incidence of CNS progression and non-CNS progression was lower in the alectinib group than in the crizotinib group.105,116,117
NCT01970865 was an open-label multicentre phase I/II study118 which demonstrated that alectinib is systemically active in patients with advanced ALK- or ROS1-positive NSCLC and can prevent intracranial metastasis. Therefore, lorlatinib is very promising as the treatment of choice for ALK- or ROS1-positive NSCLC patients with tumor metastasis.
5.6 Ensartinib
Ensartinib is a new generation of uniquely effective ALK inhibitors with good clinical activity. Ensartinib not only inhibits wild-type ALK, but also inhibits ALK-mutants (F1174, C1156Y, L1196M, S1206R, T1151, and G1202R mutants).119 Phase I/II study of ensartinib (X-396) demonstrated that in 14 patients with brain metastases, brain tumors disappeared completely in 2 patients, tumors in 7 patients were significantly reduced, and it had an intracranial response of 64.3%. There were 4 patients with stable tumor progression, so the total brain tumor control rate was as high as 92.9%. Therefore, its remarkable CNS activity is worthy of our attention.120
5.7 Erlotinib
Although erlotinib is a selective inhibitor of EGFR tyrosine kinase domain, it has been reported that it may have improved CNS metastasis activity, the CSF penetration rate of erlotinib in patients with CNS metastases is 5.1%, which suggests that it can be one of the options for the treatment of CNS metastasis in NSCLC.121 The overall response rate is about 50%, which is better than standard second-line chemotherapy.122 In addition, there are reports recommending the use of bevacizumab in combination with erlotinib as a first-line treatment for brain metastases.123
5.8 Immunotherapy
Several research groups have found that programmed cell death 1 ligand 1 (PD-L1) expression is associated with driving mutations such as EGFR mutations and EML4-ALK fusion genes in NSCLC and that overexpression of EML4-ALK fusion gene increases PD-L1 expression via a common downstream pathway mediated by mitogen-activated protein kinases/extracellular signal-regulated kinases (MAPK/ERK) and phosphoinositide 3-kinases-protein kinase B (PI3K-AKT).124 Hong et al. found that ALK fusion protein-mediated PD-L1 increased T cell apoptosis in tumor cell and dendritic cells-cytokine induced killer (DC-CIK) cell co-culture systems.124–126 One report claims that clinically, in patients with NSCLC, there are fewer studies on programmed cell death protein 1 (PD-1) axis inhibitors in patients with ALK mutations. However, patients with EGFR mutations have a lower response rate (about 10%) to PD-1 axis inhibitors.127 Anti-PD-L1 drugs include atezolizumab and durvalumab, as well as anti-PD-1 drugs, nivolumab and pembrolizumab have been approved in patients with NSCLC.128 In one study, the addition of atezolizumab to bevacizumab plus chemotherapy (carboplatin plus paclitaxel) significantly improved PFS and OS among patients with metastatic non-squamous NSCLC, regardless of PD-L1 expression and EGFR or ALK genetic alteration status.129 Compared with docetaxel, atezolizumab has a higher efficacy in advanced and metastatic NSCLC progressing.130,131 The US Food and Drug Administration approved nivolumab in March 2015 for the treatment of patients with metastatic squamous NSCLC.132 Moreover, in patients with advanced squamous cell NSCLC, nivolumab was significantly superior to docetaxel in OS, response rate, and PFS regardless of PD-L1 expression levels.133 Pembrolizumab, a monoclonal antibody that binds to the PD-1 receptor, is approved for the treatment of patients with metastatic non-squamous NSCLC who do not have EGFR or ALK genomic tumor mutations.134 And a sustained intracranial response was achieved in 33% of NSCLC patients.135 Similarly, Kamath et al. reported an intracranial response rate of 20–30% in patients with melanoma or NSCLC brain metastases treated with the PD-1 inhibitor pembrolizumab.136 These studies have shown that pembrolizumab was well tolerated and that intracranial and extracranial responses were highly consistent. The PD-1/PD-L1 inhibitor nivolumab and the cytotoxic T lymphocyte-associated antigen-4 (CTLA-4) inhibitor ipilimumab are currently the most widely used combined immunotherapy. Nivolumab and ipilimumab are the first PD-1/CTLA-4 combinations to show safety and superior efficacy in metastatic melanoma,137 however these types of combination therapy failed to improve PFS and OS in metastatic NSCLC and is not recommended for the treatment of relapsed or metastatic NSCLC.138
6. Discussion
In this review, we thoroughly summarized the invasion and metastasis pathways of ALK and ROS1 overexpressed NSCLC and current possible therapeutic strategies. Although NSCLC patients with ALK and/or ROS1 mutations account for a small fraction of the overall NSCLC, they have become a research hotspot in recent years. Compared with other parts of the metastasis, the occurrence of brain metastasis makes treatment difficult because of the existence of the BBB,19 however, after crizotinib, many second-generation TKIs have been developed, such as ceritinib, alectinib, etc., all of which can cross the BBB and maintain a certain concentration in CSF, which improves the prognosis of patients with NSCLC brain metastases.95,101 From the perspective of historical treatment, chemotherapy plus stereotactic radiosurgery (SRS), WBRT and surgery were the mainstream treatments of brain metastases,139 but we now have more choices. For example, erlotinib plus bevacizumab may be used as first-line therapy for brain metastasis (BM) in NSCLC, even in patients with BM-related neurologic symptoms and multiple BMs;123 microsurgical resection plus SRS is a good treatment for metastatic intramedullary spinal cord tumors, while also avoiding damage to surrounding tissues;139 crizotinib combined with intrathecal chemotherapy may have a better effect on pia mater disease in patients with ALK-positive NSCLC;140 pemetrexed is used as a control in some clinical studies and has been shown to be less effective than TKIs, but does not rule out the possibility of combination therapy.141 And also some immunotherapies have provided us with new ideas, in patients with PD-L1-negative tumors, the combination of pembrolizumab with chemotherapy showed high levels of activity compared to chemotherapy alone142,143
Despite we can't solve the problem of lung cancer metastasis right away, we can take a different approach to get a better prognosis. The new ALK/ROS1 inhibitor should not only be more effective than crizotinib and able to inhibit the clinically relevant acquired resistance mutations in ALK or ROS1 but also confer sustained clinical activity in the CNS. We should focus more on reducing drug toxicity and reducing related side effects. Although diseases such as brain metastasis are serious and complicated diseases, the management of NSCLC in recent years has extended the life of patients, and it is necessary to develop more personalized treatment plans and continuously optimize treatment plans.
7. Conclusion & future perspectives
Cases of tumor metastasis in ALK/ROS1 rearrangement NSCLC patients are common, which are associated with acquired drug resistance, once the disease is present, the prognosis is poor, and the mortality is high. Therefore, we summarized the most common metastasis manners for ALK/ROS1 rearrangement NSCLC and its mechanisms based on the existing researches and intended to provide ideas for future researches. We also reviewed the latest clinical trial data which give a clearer understanding of research progress in developing the most effective treatment options.
Crizotinib combined with WBRT may have a better effect on the ROS1-positive lung adenocarcinoma with symptomatic brain metastases; after treatment with crizotinib and ceritinib, alectinib can effectively control recurrent central nervous system diseases; erlotinib plus bevacizumab can be used as first-line treatment for BM in ALK-positive NSCLC. We suggest more researches should focus on the related mechanisms of increased invasion and metastasis rate caused by ALK/ROS1 rearrangement NSCLC and exploring personalized treatment strategies is urgent.
Conflicts of interest
The authors declare that they have no financial, personal or professional conflict of interest.
Acknowledgements
This work was funded by the National Natural Science Foundation of China (81872394), Young and middle age backbone personnel training program of Shenyang Pharmaceutical University (ZQN2015003) and Liaoning BaiQianWan Talents Supporting Program (2016921065).
References
- S. Sun, J. H. Schiller and A. F. Gazdar, Nat. Rev. Cancer, 2007, 7, 778–790 CrossRef CAS PubMed.
- L. C. Christine and A. W. Robert, Science, 2011, 331, 1559–1564 CrossRef PubMed.
- P. S. Steeg, Nat. Med., 2006, 12, 895–904 CrossRef CAS PubMed.
- J. Mazieres, J. P. Merlio, D. Debieuvre, J. Mosser, H. Lena, L. Ouafik, B. Besse, I. Rouquette, V. Westeel, F. Escande, I. Monnet, A. Lemoine, R. Veillon, H. Blons, C. Audigier-Valette, P. P. Bringuier, R. Lamy, M. Beau-Faller, J. L. Pujol, J. C. Sabourin, F. Penault-Llorca, M. G. Denis, S. Lantuejoul, F. Morin, Q. Tran, P. Missy, A. Langlais, B. Milleron, J. Cadranel, J. C. Soria and G. Zalcman, Lancet Oncol., 2016, 387, 1415–1426 Search PubMed.
- M. Soda, Y. L. Choi, M. Enomoto, S. Takada, Y. Yamashita, S. Ishikawa, S. Fujiwara, H. Watanabe, K. Kurashina, H. Hatanaka, M. Bando, S. Ohno, Y. Ishikawa, H. Aburatani, T. Niki, Y. Sohara, Y. Sugiyama and H. Mano, Nature, 2007, 448, 561–566 CrossRef CAS PubMed.
- J. F. Gainor and A. T. Shaw, Oncologist, 2013, 18, 865–875 CrossRef CAS PubMed.
- K. Bergethon, A. T. Shaw, S. H. Ou, R. Katayama, C. M. Lovly, N. T. McDonald, P. P. Massion, C. Siwak-Tapp, A. Gonzalez, R. Fang, E. J. Mark, J. M. Batten, H. Chen, K. D. Wilner, E. L. Kwak, J. W. Clark, D. P. Carbone, H. Ji, J. A. Engelman, M. Mino-Kenudson, W. Pao and A. J. Iafrate, J. Clin. Oncol., 2012, 30, 863–870 CrossRef CAS PubMed.
- B. Gitlitz, B. Addario, A. Sable-Hunt, S. Novello, T. Vavala, R. Chen, M. Bittoni, S. L. Park, M. Jennings and G. Oxnard, J. Clin. Oncol., 2016, 11, S174 Search PubMed.
- M. Takeda, I. Okamoto and K. Nakagawa, J. Clin. Oncol., 2013, 8, 654–657 CAS.
- X. Pan, T. Lv, F. Zhang, H. Fan, H. Liu and Y. Song, Clin. Transl. Oncol., 2018, 20, 1168–1174 CrossRef CAS PubMed.
- J. F. Gainor, S. H. Ou, J. Logan, L. F. Borges and A. T. Shaw, J. Thorac. Oncol., 2013, 8, 1570–1573 CrossRef CAS PubMed.
- P. W. Sperduto, N. Kased, D. Roberge, Z. Xu, R. Shanley, X. Luo, P. K. Sneed, S. T. Chao, R. J. Weil, J. Suh, A. Bhatt, A. W. Jensen, P. D. Brown, H. A. Shih, J. Kirkpatrick, L. E. Gaspar, J. B. Fiveash, V. Chiang, J. P. Knisely, C. M. Sperduto, N. Lin and M. Mehta, J. Clin. Oncol., 2012, 30, 419–425 CrossRef PubMed.
- K. Deepak, B. Paul, L. Jing and M. P. Mehta, J. Clin. Oncol., 2015, 24, 1295–1304 Search PubMed.
- J. L. Hubbs, J. A. Boyd, H. Donna, J. P. Chino, S. Mert and C. R. Kelsey, Cancer, 2010, 116, 5038–5046 CrossRef PubMed.
- I. B. Muller, A. J. de Langen, E. Giovannetti and G. J. Peters, OncoTargets Ther., 2017, 10, 4535–4541 CrossRef PubMed.
- M. Nishio, D. W. Kim, Y. L. Wu, K. Nakagawa, B. J. Solomon, A. T. Shaw, S. Hashigaki, E. Ohki, T. Usari, J. Paolini, A. Polli, K. D. Wilner and T. Mok, Cancer Res. Treat., 2018, 50, 691–700 CrossRef CAS PubMed.
- A. T. Shaw, D. W. Kim, R. Mehra, D. S. Tan, E. Felip, L. Q. Chow, D. R. Camidge, J. Vansteenkiste, S. Sharma, T. De Pas, G. J. Riely, B. J. Solomon, J. Wolf, M. Thomas, M. Schuler, G. Liu, A. Santoro, Y. Y. Lau, M. Goldwasser, A. L. Boral and J. A. Engelman, N. Engl. J. Med., 2014, 370, 1189–1197 CrossRef CAS PubMed.
- O. Arrieta, L. A. Ramirez-Tirado, R. Baez-Saldana, O. Pena-Curiel, G. Soca-Chafre and E. O. Macedo-Perez, Lung Cancer, 2015, 90, 161–166 CrossRef PubMed.
- A. Sgambato, F. Casaluce, P. Maione and C. Gridelli, Expert Rev. Anticancer Ther., 2018, 18, 71–80 CrossRef CAS PubMed.
- J. Remon, E. Le Rhun and B. Besse, Cancer Treat. Rev., 2017, 53, 128–137 CrossRef CAS PubMed.
- C. J. J. A. F. P. A. U. P. U. F. K. M. Eberth, Virchows Arch. A: Pathol. Anat., 1969, 49, 51–63 CrossRef.
- C. P. Kokkoris, Cancer, 1983, 51, 154–160 CrossRef CAS PubMed.
- J. Yang, S. Shin, M. Fowkes, S. Krieger, J. A. Strauchen, M. Smethurst and A. Demopoulos, J. Neurol., 2011, 77, e75 CrossRef CAS PubMed.
- A. Boire, Y. Zou, J. Shieh, D. G. Macalinao, E. Pentsova and J. Massague, Cell, 2017, 168, 1101–1113 CrossRef CAS PubMed.
- S. S. Lee, M. K. Kim, S. J. Sym, S. W. Kim, W. K. Kim, S. B. Kim and J. H. Ahn, J. Neuro-Oncol., 2007, 84, 85–89 CrossRef PubMed.
- H. Okamoto, T. Shinkai, Y. Matsuno and N. Saijo, Cancer, 2015, 72, 2583–2588 CrossRef.
- E. S. Connolly Jr, C. J. Winfree, P. C. Mccormick, M. Cruz and B. M. Stein, Surgical Neurology, 1996, 46, 329–337 CrossRef CAS.
- J. M. Findlay, M. Bernstein, R. G. Vanderlinden and L. Resch, Neurosurgery, 1987, 21, 911 CrossRef CAS PubMed.
- M. Kizawa, N. Mori, Y. Hashizume and M. Yoshida, Neuropathology, 2010, 28, 295–302 CrossRef PubMed.
- K. Steven, P. Johan, L. R. Emilie, P. Fabrice, I. Gueorgui, R. François-Xavier, P. Philippe, M. Jean-François, E. Evelyne and D. Bertrand, Neurosurgery, 2013, 73, 923–931 CrossRef PubMed.
- Y. Xu, W. Zhong, J. Zhao, M. Chen, L. Li and M. Wang, Zhongguo Feiai Zazhi, 2016, 19, 539–544 Search PubMed.
- H. Okamoto, T. Shinkai, Y. Matsuno and N. Saijo, Cancer, 2015, 72, 2583–2588 CrossRef.
- W. S. Sung, M. J. Sung, J. H. Chan, B. Manion, J. Song, A. Dubey, A. Erasmus and A. Hunn, World Neurosurg., 2013, 79, 576–584 CrossRef PubMed.
- M. Karsy, J. Guan, W. Sivakumar, J. A. Neil, M. H. Schmidt and M. A. Mahan, Neurosurg. Focus, 2015, 39, E3 Search PubMed.
- C. Kuijpers, L. E. L. Hendriks, J. L. Derks, A. C. Dingemans, A. S. R. van Lindert, M. M. van den Heuvel, R. A. Damhuis and S. M. Willems, Lung Cancer, 2018, 121, 76–81 CrossRef CAS PubMed.
- L. R. Patel, D. F. Camacho, Y. Shiozawa, K. J. Pienta and R. S. Taichman, Future Oncol., 2011, 7, 1285–1297 CrossRef CAS PubMed.
- S. Peters and E. Meylan, Curr. Opin. Oncol., 2013, 25, 137–144 CrossRef CAS PubMed.
- C. D'Antonio, A. Passaro, B. Gori, E. Del Signore, M. R. Migliorino, S. Ricciardi, A. Fulvi and F. de Marinis, Ther. Adv. Med. Oncol., 2014, 6, 101–114 CrossRef PubMed.
- R. Faccio, Ann. N. Y. Acad. Sci., 2011, 1237, 71–78 CrossRef CAS PubMed.
- W. J. Boyle, W. S. Simonet and D. L. Lacey, Nature, 2003, 423, 337 CrossRef CAS PubMed.
- Y. Y. Kong, H. Yoshida, I. Sarosi, H. L. Tan, E. Timms, C. Capparelli, S. Morony, A. J. Oliveira-Dos-Santos, G. Van and A. Itie, Nature, 1999, 397, 315–323 CrossRef CAS PubMed.
- E. E. Mcgrath, J. Thorac. Oncol., 2011, 6, 1468–1473 CrossRef PubMed.
- G. R. Mundy, Nat. Rev. Cancer, 2002, 2, 584–593 CrossRef CAS PubMed.
- L. Xin, W. Qiongqing, H. Guohong, V. P. Catherine, F. Martin, R. Michael, M. Joan and K. Yibin, Genes Dev., 2009, 23, 1882 CrossRef PubMed.
- R. E. Miller, J. C. Jones, T. Mark, M. L. Blake and W. C. Dougall, J. Thorac. Oncol., 2014, 9, 345–354 CrossRef CAS PubMed.
- D. Jones, E. R. Pereira and T. P. Padera, Front Oncol., 2018, 8, 36 CrossRef PubMed.
- S. Zhang, B. Yan, J. Zheng, J. Zhao and J. Zhou, Oncotarget, 2016, 7, 63758–63766 Search PubMed.
- Q. Gao, H. Ma, B. Wang, Y. Yao, J. Zhou and J. Zhou, Oncotarget, 2017, 8, 108840–108847 Search PubMed.
- J. A. Irving and R. H. Young, Am. J. Surg. Pathol., 2005, 29, 997 Search PubMed.
- K. Fujiwara, Y. Ohishi, H. Koike, S. Sawada, T. Moriya and I. Kohno, Gynecol. Oncol., 1995, 59, 124–128 CrossRef CAS PubMed.
- K. A. Lee, J. S. Lee, J. K. Min, H. J. Kim, W. S. Kim and K. Y. Lee, Tuberc. Respir. Dis., 2014, 77, 258–261 CrossRef PubMed.
- X. Jing, F. Li, X. Meng, Z. Liu, J. Yu and B. Liu, Cancer Biol. Ther., 2017, 18, 279–284 CrossRef CAS PubMed.
- T. Franchina, A. Russo, G. R. Ricciardi, G. Liguori, A. Herberg, N. Normanno and V. Adamo, Cancer Biol. Ther., 2016, 1–5, DOI:10.1080/15384047.2016.1219822.
- K. M. Kreusel, N. E. Bechrakis, T. Wiegel, L. Krause and M. H. Foerster, Acta Ophthalmol., 2008, 86, 515–519 CrossRef PubMed.
- Y. Okuma, Y. Hosomi, K. Kitamura, M. Iguchi, T. Okamura, S. Fukami, T. Hishima and M. Shibuya, Int. J. Clin. Oncol., 2009, 14, 541–544 CrossRef PubMed.
- Y. Okuma, Y. Tanaka, T. Kamei, Y. Hosomi and T. Okamura, OncoTargets Ther., 2015, 8, 1321–1325 CrossRef CAS PubMed.
- S. Lu, M. C. Azada and S. H. Ou, Lung Cancer, 2015, 87, 207–209 CrossRef PubMed.
- Y. Feng, A. D. Singh, C. Lanigan, R. R. Tubbs and P. C. Ma, J. Thorac. Oncol., 2013, 8, e109–111 CrossRef PubMed.
- Z. H. Cui, Y. Zhang, L. L. Liang, Z. H. Li, I. Abramova and Q. Hao, Int. J. Ophthalmol., 2017, 10, 310–314 Search PubMed.
- R. E. Mikes, F. Jordan, G. Hutarew and M. Studnicka, Lung Cancer, 2015, 90, 614–616 CrossRef PubMed.
- M. Varella-Garcia, J. A. Iafrate and W. Pao, J. Thorac. Oncol., 2011, 6(6), S291 Search PubMed.
- P. Yang, J. Thorac. Oncol., 2011, 6(6), S50 Search PubMed.
- R. C. Doebele, X. Lu, C. Sumey, D. A. Maxson, A. J. Weickhardt, A. B. Oton, P. A. Bunn Jr, A. E. Baron, W. A. Franklin, D. L. Aisner, M. Varella-Garcia and D. R. Camidge, Cancer, 2012, 118, 4502–4511 CrossRef CAS PubMed.
- M. Di, X. Hao, W. Yan, P. Xing and J. Li, Thorac. Cancer, 2016, 7, 452–458 CrossRef PubMed.
- S. Tan, D. Sun, W. Pu, Q. Gou, C. Guo, Y. Gong, J. Li, Y. Q. Wei, L. Liu, Y. Zhao and Y. Peng, Mol. Cancer, 2018, 17, 138 CrossRef PubMed.
- L. Li, Y. Wang, T. Peng, K. Zhang, C. Lin, R. Han, C. Lu and Y. He, Oncotarget, 2016, 7, 34442–34452 Search PubMed.
- J. F. Gainor, L. Dardaei, S. Yoda, L. Friboulet, I. Leshchiner, R. Katayama, I. Dagogo-Jack, S. Gadgeel, K. Schultz, M. Singh, E. Chin, M. Parks, D. Lee, R. H. DiCecca, E. Lockerman, T. Huynh, J. Logan, L. L. Ritterhouse, L. P. Le, A. Muniappan, S. Digumarthy, C. Channick, C. Keyes, G. Getz, D. Dias-Santagata, R. S. Heist, J. Lennerz, L. V. Sequist, C. H. Benes, A. J. Iafrate, M. Mino-Kenudson, J. A. Engelman and A. T. Shaw, Cancer Discovery, 2016, 6, 1118–1133 CrossRef CAS PubMed.
- B. De Craene and G. Berx, Nat. Rev. Cancer, 2013, 13, 97–110 CrossRef CAS PubMed.
- A. Kogita, Y. Togashi, H. Hayashi, S. Sogabe, M. Terashima, M. A. De Velasco, K. Sakai, Y. Fujita, S. Tomida, Y. Takeyama, K. Okuno, K. Nakagawa and K. Nishio, Int. J. Oncol., 2014, 45, 1430–1436 CrossRef CAS PubMed.
- F. Guo, X. Liu, Q. Qing, Y. Sang, C. Feng, X. Li, L. Jiang, P. Su and Y. Wang, Biochem. Biophys. Res. Commun., 2015, 459, 398–404 CrossRef CAS PubMed.
- R. An, Y. Wang, D. Voeller, A. Gower, I. K. Kim, Y. W. Zhang and G. Giaccone, Oncotarget, 2016, 7, 29199–29210 Search PubMed.
- H. X. Gao, L. Yan, C. Li, L. M. Zhao and W. Liu, Mol. Med. Rep., 2016, 14, 4135–4143 CrossRef CAS PubMed.
- H. J. Jun, H. Johnson, R. T. Bronson, S. de Feraudy, F. White and A. Charest, Cancer Res., 2012, 72, 3764–3774 CrossRef CAS PubMed.
- W. Gou, X. Zhou, Z. Liu, L. Wang, J. Shen, X. Xu, Z. Li, X. Zhai, D. Zuo and Y. Wu, Cancer Lett., 2018, 422, 19–28 CrossRef CAS PubMed.
- M. A. Socinski, T. Evans, S. Gettinger, T. A. Hensing, L. VanDam Sequist, B. Ireland and T. E. Stinchcombe, Chest, 2013, 143, e341S–e368S CrossRef CAS PubMed.
- Y. Y. Soon, M. R. Stockler, L. M. Askie and M. J. Boyer, J. Clin. Oncol., 2009, 27, 3277–3283 CrossRef PubMed.
- B. J. Solomon, F. Cappuzzo, E. Felip, F. H. Blackhall, D. B. Costa, D.-W. Kim, K. Nakagawa, Y.-L. Wu, T. Mekhail, J. Paolini, J. Tursi, T. Usari, K. D. Wilner, P. Selaru and T. S. K. Mok, J. Clin. Oncol., 2016, 34, 2858–2865 CrossRef CAS PubMed.
- J. D. Patel, M. A. Socinski, E. B. Garon, C. H. Reynolds, D. R. Spigel, M. R. Olsen, R. C. Hermann, R. M. Jotte, T. Beck, D. A. Richards, S. C. Guba, J. Liu, B. Frimodt-Moller, W. J. John, C. K. Obasaju, E. J. Pennella, P. Bonomi and R. Govindan, J. Clin. Oncol., 2013, 31, 4349–4357 CrossRef CAS PubMed.
- M. J. Edelman, T. Le Chevalier and J. C. Soria, J. Thorac. Oncol., 2012, 7, 1331–1336 CrossRef CAS PubMed.
- J. G. Christensen, H. Y. Zou, M. E. Arango, Q. Li, J. H. Lee, S. R. McDonnell, S. Yamazaki, G. R. Alton, B. Mroczkowski and G. Los, Mol. Cancer Ther., 2007, 6, 3314–3322 CrossRef CAS PubMed.
- H. Yasuda, L. L. de Figueiredo-Pontes, S. Kobayashi and D. B. Costa, J. Thorac. Oncol., 2012, 7, 1086–1090 CrossRef CAS PubMed.
- I. Hideko, Y. Masayuki, T. Nagio, H. Katsuyuki, I. Eiki, T. Akihiko, T. Shinichi, H. Shinsuke, S. Toshiaki and T. Mitsune, Jpn. J. Clin. Oncol., 2014, 44, 963–968 CrossRef PubMed.
- K. Yoshiaki, K. Yasutaka, S. Atsuhiko and H. Kouko, BMJ Case Rep., 2013, 2013 DOI:10.1136/bcr-2013-200867.
- D. Maillet, I. Martellafay, D. Arpin and M. Pérol, J. Thorac. Oncol., 2013, 8, e30–e31 CrossRef PubMed.
- B. J. Solomon, T. Mok, D. W. Kim, Y. L. Wu, K. Nakagawa, T. Mekhail, E. Felip, F. Cappuzzo, J. Paolini, T. Usari, S. Iyer, A. Reisman, K. D. Wilner, J. Tursi, F. Blackhall and P. Investigators, N. Engl. J. Med., 2014, 371, 2167–2177 CrossRef PubMed.
- A. T. Shaw, D. W. Kim, K. Nakagawa, T. Seto, L. Crino, M. J. Ahn, T. De Pas, B. Besse, B. J. Solomon, F. Blackhall, Y. L. Wu, M. Thomas, K. J. O'Byrne, D. Moro-Sibilot, D. R. Camidge, T. Mok, V. Hirsh, G. J. Riely, S. Iyer, V. Tassell, A. Polli, K. D. Wilner and P. A. Janne, N. Engl. J. Med., 2013, 368, 2385–2394 CrossRef CAS PubMed.
- https://www.cancer.gov/about-cancer/treatment/drugs/fda-crizotinib, 2013.
- J. C. Chuang and J. W. Neal, Transl. Lung Cancer Res., 2015, 4, 639–641 CAS.
- I. Dagogo-Jack and A. T. Shaw, Ann. Oncol., 2016, 27(suppl. 3), iii42–iii50 CrossRef PubMed.
- G. Toyokawa, T. Seto, M. Takenoyama and Y. Ichinose, Cancer Metastasis Rev., 2015, 34, 797–805 CrossRef CAS PubMed.
- M. Zhang, L. Nie and J. Zhang, Zhongguo Feiai Zazhi, 2016, 19, 525–529 Search PubMed.
- C. E. Onesti, D. Iacono, S. Angelini, M. Mazzotta, R. Giusti, S. Lauro and P. Marchetti, Anticancer Drugs, 2019, 30, 201–204 CrossRef CAS PubMed.
- S. Okawa, T. Shibayama, A. Shimonishi, J. Nishimura, T. Ozeki, K. Takada, H. Kayatani, D. Minami, K. Sato, K. Fujiwara, T. Yonei, T. Sato and M. Suno, Case Rep. Oncol., 2018, 11, 777–783 CrossRef PubMed.
- K. Hideki, N. Takahiro, T. Kengo, S. Manabu, M. Hiroyuki, I. Toshihiko, M. Yukiko, Y. Mitsuru, S. Masato, I. Meiji, I. Makiko, I. Dai, Y. Sana, K. Hajime, O. Miki and N. Akira, Lung Cancer, 2012, 75, 66–72 CrossRef PubMed.
- L. Friboulet, N. Li, R. Katayama, C. C. Lee, J. F. Gainor, A. S. Crystal, P. Y. Michellys, M. M. Awad, N. Yanagitani, S. Kim, A. C. Pferdekamper, J. Li, S. Kasibhatla, F. Sun, X. Sun, S. Hua, P. McNamara, S. Mahmood, E. L. Lockerman, N. Fujita, M. Nishio, J. L. Harris, A. T. Shaw and J. A. Engelman, Cancer Discovery, 2014, 4, 662–673 CrossRef CAS PubMed.
- D. W. Kim, R. Mehra, S. W. Tan, E. Felip, L. Q. M. Chow and D. R. Camidge, Oncologist, 2014, 19, 5–11 CrossRef PubMed.
- I. Zhang, N. G. Zaorsky, J. D. Palmer, R. Mehra and B. Lu, Lancet Oncol., 2015, 16, e510–e521 CrossRef CAS PubMed.
- S. M. Lim, H. R. Kim, J. S. Lee, K. H. Lee, Y. G. Lee, Y. J. Min, E. K. Cho, S. S. Lee, B. S. Kim and M. Y. Choi, J. Clin. Oncol., 2017, 35, 2613 CrossRef CAS PubMed.
- Y. Xu, W. Zhong, M. Chen, J. Zhao and M. Wang, Thorac. Cancer, 2018, 9, 1078–1081 CrossRef PubMed.
- E. Bendaly, A. A. Dalal, K. Culver, P. Galebach, I. Bocharova, R. Foster, M. Sasane, A. R. Macalalad and A. Guérin, Adv. Ther., 2017, 34, 1145 CrossRef CAS PubMed.
- S. M. Gadgeel, L. Gandhi, G. J. Riely, A. A. Chiappori, H. L. West, M. C. Azada, P. N. Morcos, R. M. Lee, L. Garcia and L. J. Yu, Lancet Oncol., 2014, 15, 1119–1128 CrossRef CAS PubMed.
- T. Kodama, M. Hasegawa, K. Takanashi, Y. Sakurai, O. Kondoh and H. Sakamoto, Cancer Chemother. Pharmacol., 2014, 74, 1023–1028 CrossRef CAS PubMed.
- H. Sakamoto, T. Tsukaguchi, S. Hiroshima, T. Kodama, T. Kobayashi, T. A. Fukami, N. Oikawa, T. Tsukuda, N. Ishii and Y. Aoki, Cancer Cell, 2011, 19, 679–690 CrossRef CAS PubMed.
- https://www.cancer.gov/news-events/cancer-currents-blog/2017/alectinib-fda-untreated-lung-cancer, 2017.
- S. Peters, D. R. Camidge, A. T. Shaw, S. Gadgeel, J. S. Ahn, D. W. Kim, S. I. Ou, M. Perol, R. Dziadziuszko, R. Rosell, A. Zeaiter, E. Mitry, S. Golding, B. Balas, J. Noe, P. N. Morcos and T. Mok, N. Engl. J. Med., 2017, 377, 829–838 CrossRef CAS PubMed.
- J. F. Gainor, C. A. Sherman, K. Willoughby, J. Logan, E. Kennedy, P. K. Brastianos, A. S. Chi and A. T. Shaw, J. Thorac. Oncol., 2015, 10, 232–236 CrossRef CAS PubMed.
- S. H. Ou, J. S. Ahn, L. De Petris, R. Govindan, J. C. Yang, B. Hughes, H. Lena, D. Moro-Sibilot, A. Bearz, S. V. Ramirez, T. Mekhail, A. Spira, W. Bordogna, B. Balas, P. N. Morcos, A. Monnet, A. Zeaiter and D. W. Kim, J. Clin. Oncol., 2016, 34, 661–668 CrossRef CAS PubMed.
- I. O. Sai-Hong, K. R. Sommers, M. C. Azada and E. B. Garon, Oncologist, 2015, 20, 224–226 CrossRef PubMed.
- A. Markham, Drugs, 2017, 77, 1131–1135 CrossRef CAS PubMed.
- D. R. Camidge, H. R. Kim, M. J. Ahn, J. C. Yang, J. Y. Han, J. S. Lee, M. J. Hochmair, J. Y. Li, G. C. Chang, K. H. Lee, C. Gridelli, A. Delmonte, R. Garcia Campelo, D. W. Kim, A. Bearz, F. Griesinger, A. Morabito, E. Felip, R. Califano, S. Ghosh, A. Spira, S. N. Gettinger, M. Tiseo, N. Gupta, J. Haney, D. Kerstein and S. Popat, N. Engl. J. Med., 2018, 379, 2027–2039 CrossRef CAS PubMed.
- D. W. Kim, M. Tiseo, M. J. Ahn, K. L. Reckamp, K. H. Hansen, S. W. Kim, R. M. Huber, H. L. West, G. Hjm and M. J. Hochmair, J. Clin. Oncol., 2017, 35, JCO2016715904 Search PubMed.
- S. N. Gettinger, L. A. Bazhenova, C. J. Langer, R. Salgia, K. A. Gold, R. Rosell, A. T. Shaw, G. J. Weiss, M. Tugnait, N. I. Narasimhan, D. J. Dorer, D. Kerstein, V. M. Rivera, T. Clackson, F. G. Haluska and D. R. Camidge, Lancet Oncol., 2016, 17, 1683–1696 CrossRef CAS PubMed.
- T. W. Johnson, P. F. Richardson, S. Bailey, A. Brooun, B. J. Burke, M. R. Collins, J. J. Cui, J. G. Deal, Y. L. Deng, D. Dinh, L. D. Engstrom, M. He, J. Hoffman, R. L. Hoffman, Q. Huang, R. S. Kania, J. C. Kath, H. Lam, J. L. Lam, P. T. Le, L. Lingardo, W. Liu, M. McTigue, C. L. Palmer, N. W. Sach, T. Smeal, G. L. Smith, A. E. Stewart, S. Timofeevski, H. Zhu, J. Zhu, H. Y. Zou and M. P. Edwards, J. Med. Chem., 2014, 57, 4720–4744 CrossRef CAS PubMed.
- https://www.cancer.gov/news-events/cancer-currents-blog/2017/fda-ceritinib-nsclc, 2017.
- H. Y. Zou, L. Friboulet, D. P. Kodack, L. D. Engstrom, Q. Li, M. West, R. W. Tang, H. Wang, K. Tsaparikos, J. Wang, S. Timofeevski, R. Katayama, D. M. Dinh, H. Lam, J. L. Lam, S. Yamazaki, W. Hu, B. Patel, D. Bezwada, R. L. Frias, E. Lifshits, S. Mahmood, J. F. Gainor, T. Affolter, P. B. Lappin, H. Gukasyan, N. Lee, S. Deng, R. K. Jain, T. W. Johnson, A. T. Shaw, V. R. Fantin and T. Smeal, Cancer Cell, 2015, 28, 70–81 CrossRef CAS PubMed.
- M. Nishio, K. Nakagawa, T. Mitsudomi, N. Yamamoto, T. Tanaka, H. Kuriki, A. Zeaiter and T. Tamura, Lung Cancer, 2018, 121, 37–40 CrossRef PubMed.
- T. Hida, H. Nokihara, M. Kondo, Y. H. Kim, K. Azuma, T. Seto, Y. Takiguchi, M. Nishio, H. Yoshioka and F. Imamura, Lancet, 2017, 390, 29–39 CrossRef CAS.
- A. T. Shaw, E. Felip, T. M. Bauer, B. Besse, A. Navarro, S. Postel-Vinay, J. F. Gainor, M. Johnson, J. Dietrich, L. P. James, J. S. Clancy, J. Chen, J.-F. Martini, A. Abbattista and B. J. Solomon, Lancet Oncol., 2017, 18, 1590–1599 CrossRef CAS PubMed.
- T. Li, W. Ma and E. C. Tian, Chin. J. Clin. Oncol., 2018 DOI:10.21037/cco.2018.10.12.
- L. Horn, J. R. Infante, K. L. Reckamp, G. R. Blumenschein, T. A. Leal, S. N. Waqar, B. J. Gitlitz, R. E. Sanborn, J. G. Whisenant, L. Du, J. W. Neal, J. P. Gockerman, G. Dukart, K. Harrow, C. Liang, J. J. Gibbons, A. Holzhausen, C. M. Lovly and H. A. Wakelee, Clin. Cancer Res., 2018, 24, 2771–2779 CrossRef CAS PubMed.
- T. Yosuke, M. Katsuhiro, F. Masahide, T. Tomohiro, F. Shiro, I. Kaoru, S. Yuichi, K. Young Hak, M. Tadashi and I. Ken-Ichi, J. Thorac. Oncol., 2010, 5, 950–955 CrossRef PubMed.
- Q. Wei-Xiang, S. Zan, L. Feng, S. Yuan-Jue, M. Da-Liu, T. Li-Na, H. Ai-Na and Y. Yang, Asian Pac. J. Cancer Prev., 2012, 13, 5177–5182 CrossRef.
- Y. Chikaishi, M. Kanayama, A. Taira, Y. Nabe, S. Shinohara, T. Kuwata, M. Takenaka, S. Oka, A. Hirai, K. Kuroda, N. Imanishi, Y. Ichiki and F. Tanaka, Ann. Transl. Med., 2018, 6, 401 CrossRef PubMed.
- K. Ota, K. Azuma, A. Kawahara, S. Hattori, E. Iwama, J. Tanizaki, T. Harada, K. Matsumoto, K. Takayama, S. Takamori, M. Kage, T. Hoshino, Y. Nakanishi and I. Okamoto, Clin. Cancer Res., 2015, 21, 4014–4021 CrossRef CAS PubMed.
- K. Azuma, K. Ota, A. Kawahara, S. Hattori, E. Iwama, T. Harada, K. Matsumoto, K. Takayama, S. Takamori and M. Kage, Ann. Oncol., 2014, 25, 1935 CrossRef CAS PubMed.
- E. A. Akbay, S. Koyama, J. Carretero, A. Altabef, J. H. Tchaicha, C. L. Christensen, O. R. Mikse, A. D. Cherniack, E. M. Beauchamp, T. J. Pugh, M. D. Wilkerson, P. E. Fecci, M. Butaney, J. B. Reibel, M. Soucheray, T. J. Cohoon, P. A. Janne, M. Meyerson, D. N. Hayes, G. I. Shapiro, T. Shimamura, L. M. Sholl, S. J. Rodig, G. J. Freeman, P. S. Hammerman, G. Dranoff and K. K. Wong, Cancer Discovery, 2013, 3, 1355–1363 CrossRef CAS PubMed.
- H. Borghaei, L. Paz-Ares, L. Horn, D. R. Spigel, M. Steins, N. E. Ready, L. Q. Chow, E. E. Vokes, E. Felip, E. Holgado, F. Barlesi, M. Kohlhaufl, O. Arrieta, M. A. Burgio, J. Fayette, H. Lena, E. Poddubskaya, D. E. Gerber, S. N. Gettinger, C. M. Rudin, N. Rizvi, L. Crino, G. R. Blumenschein Jr, S. J. Antonia, C. Dorange, C. T. Harbison, F. Graf Finckenstein and J. R. Brahmer, N. Engl. J. Med., 2015, 373, 1627–1639 CrossRef CAS PubMed.
- M. Protopapa, V. Kouloulias, S. Nikoloudi, C. Papadimitriou, G. Gogalis and A. Zygogianni, J. Oncol., 2019, 2019, 3267409 Search PubMed.
- M. A. Socinski, R. M. Jotte, F. Cappuzzo, F. Orlandi, D. Stroyakovskiy, N. Nogami, D. Rodriguez-Abreu, D. Moro-Sibilot, C. A. Thomas, F. Barlesi, G. Finley, C. Kelsch, A. Lee, S. Coleman, Y. Deng, Y. Shen, M. Kowanetz, A. Lopez-Chavez, A. Sandler and M. Reck, N. Engl. J. Med., 2018, 378, 2288–2301 CrossRef CAS PubMed.
- L. Fehrenbacher, A. Spira, M. Ballinger, M. Kowanetz, J. Vansteenkiste, J. Mazieres, K. Park, D. Smith, A. Artal-Cortes, C. Lewanski, F. Braiteh, D. Waterkamp, P. He, W. Zou, D. S. Chen, J. Yi, A. Sandler and A. Rittmeyer, Lancet, 2016, 387, 1837–1846 CrossRef CAS.
- A. Rittmeyer, F. Barlesi, D. Waterkamp, K. Park, F. Ciardiello, J. von Pawel, S. M. Gadgeel, T. Hida, D. M. Kowalski, M. C. Dols, D. L. Cortinovis, J. Leach, J. Polikoff, C. Barrios, F. Kabbinavar, O. A. Frontera, F. De Marinis, H. Turna, J. S. Lee, M. Ballinger, M. Kowanetz, P. He, D. S. Chen, A. Sandler and D. R. Gandara, Lancet, 2017, 389, 255–265 CrossRef.
- FDA expands approved use of Opdivo to treat lung cancer, Press release of the Food and Drug Administration, Bethesda, MD, 2015, http://www.fda.gov/newsevents/newsroom/pressannouncements/ucm436534.htm Search PubMed.
- J. Brahmer, K. L. Reckamp, P. Baas, L. Crinò, W. E. E. Eberhardt, E. Poddubskaya, S. Antonia, A. Pluzanski, E. E. Vokes, E. Holgado, D. Waterhouse, N. Ready, J. Gainor, O. Arén Frontera, L. Havel, M. Steins, M. C. Garassino, J. G. Aerts, M. Domine, L. Paz-Ares, M. Reck, C. Baudelet, C. T. Harbison, B. Lestini and D. R. Spigel, N. Engl. J. Med., 2015, 373, 123–135 CrossRef CAS PubMed.
- C. J. Langer, S. M. Gadgeel, H. Borghaei, V. A. Papadimitrakopoulou, A. Patnaik, S. F. Powell, R. D. Gentzler, R. G. Martins, J. P. Stevenson, S. I. Jalal, A. Panwalkar, J. C.-H. Yang, M. Gubens, L. V. Sequist, M. M. Awad, J. Fiore, Y. Ge, H. Raftopoulos and L. Gandhi, Lancet Oncol., 2016, 17, 1497–1508 CrossRef CAS PubMed.
- S. B. Goldberg, S. N. Gettinger, A. Mahajan, A. C. Chiang, R. S. Herbst, M. Sznol, A. J. Tsiouris, J. Cohen, A. Vortmeyer, L. Jilaveanu, J. Yu, U. Hegde, S. Speaker, M. Madura, A. Ralabate, A. Rivera, E. Rowen, H. Gerrish, X. Yao, V. Chiang and H. M. Kluger, Lancet Oncol., 2016, 17, 976–983 CrossRef CAS PubMed.
- S. D. Kamath and P. U. Kumthekar, Front Oncol., 2018, 8, 414 CrossRef PubMed.
- J. Larkin, V. Chiarion-Sileni, R. Gonzalez, J. J. Grob, C. L. Cowey, C. D. Lao, D. Schadendorf, R. Dummer, M. Smylie, P. Rutkowski, P. F. Ferrucci, A. Hill, J. Wagstaff, M. S. Carlino, J. B. Haanen, M. Maio, I. Marquez-Rodas, G. A. McArthur, P. A. Ascierto, G. V. Long, M. K. Callahan, M. A. Postow, K. Grossmann, M. Sznol, B. Dreno, L. Bastholt, A. Yang, L. M. Rollin, C. Horak, F. S. Hodi and J. D. Wolchok, N. Engl. J. Med., 2015, 373, 1270–1271 CrossRef PubMed.
- M. A. Gubens, L. V. Sequist, J. P. Stevenson, S. F. Powell, L. C. Villaruz, S. M. Gadgeel, C. J. Langer, A. Patnaik, H. Borghaei, S. I. Jalal, J. Fiore, S. Saraf, H. Raftopoulos and L. Gandhi, Lung Cancer, 2019, 130, 59–66 CrossRef PubMed.
- S. Hernandez-Duran, S. Hanft, R. J. Komotar and G. R. Manzano, Neurosurgical Review, 2016, 39, 175–183 CrossRef PubMed.
- H. K. Ahn, B. Han, S. J. Lee, T. Lim, J. M. Sun, J. S. Ahn, M. J. Ahn and K. Park, Lung Cancer, 2012, 76, 253–254 CrossRef PubMed.
- J.-C. Soria, D. S. W. Tan, R. Chiari, Y.-L. Wu, L. Paz-Ares, J. Wolf, S. L. Geater, S. Orlov, D. Cortinovis, C.-J. Yu, M. Hochmair, A. B. Cortot, C.-M. Tsai, D. Moro-Sibilot, R. G. Campelo, T. McCulloch, P. Sen, M. Dugan, S. Pantano, F. Branle, C. Massacesi and G. de Castro, Lancet, 2017, 389, 917–929 CrossRef CAS.
- M. Reck, Immunotherapy, 2018, 10, 93 CrossRef CAS PubMed.
- R. Hui, E. B. Garon, J. W. Goldman, N. B. Leighl, M. D. Hellmann, A. Patnaik, L. Gandhi, J. P. Eder, M. J. Ahn and L. Horn, Ann. Oncol., 2017, 28, 874–881 CrossRef CAS PubMed.
- T. Hida, H. Nokihara, M. Kondo, Y. H. Kim, K. Azuma, T. Seto, Y. Takiguchi, M. Nishio, H. Yoshioka, F. Imamura, K. Hotta, S. Watanabe, K. Goto, M. Satouchi, T. Kozuki, T. Shukuya, K. Nakagawa, T. Mitsudomi, N. Yamamoto, T. Asakawa, R. Asabe, T. Tanaka and T. Tamura, Lancet, 2017, 390, 29–39 CrossRef CAS.
- D. R. Camidge, D. W. Kim, M. Tiseo, C. J. Langer, M. J. Ahn, A. T. Shaw, R. M. Huber, M. J. Hochmair, D. H. Lee, L. A. Bazhenova, K. A. Gold, S. I. Ou, H. L. West, W. Reichmann, J. Haney, T. Clackson, D. Kerstein and S. N. Gettinger, J. Clin. Oncol., 2018, 36, 2693–2701 CrossRef CAS PubMed.
- B. J. Solomon, B. Besse, T. M. Bauer, E. Felip, R. A. Soo, D. R. Camidge, R. Chiari, A. Bearz, C. C. Lin, S. M. Gadgeel, G. J. Riely, E. H. Tan, T. Seto, L. P. James, J. S. Clancy, A. Abbattista, J. F. Martini, J. Chen, G. Peltz, H. Thurm, S. I. Ou and A. T. Shaw, Lancet Oncol., 2018, 19, 1654–1667 CrossRef CAS PubMed.
|
This journal is © The Royal Society of Chemistry 2019 |
Click here to see how this site uses Cookies. View our privacy policy here.