DOI:
10.1039/C9RA01200A
(Paper)
RSC Adv., 2019,
9, 7718-7722
PIDA-mediated intramolecular oxidative C–N bond formation for the direct synthesis of quinoxalines from enaminones†
Received
16th February 2019
, Accepted 18th February 2019
First published on 7th March 2019
Abstract
A intramolecular oxidative C(sp2)–N bond formation mediated by hypervalent iodine(III) to obtain quinoxalines from readily available N-(2-acetaminophenyl)enaminones was developed. A tandem process involving PIDA-mediated intramolecular condensation cyclization and a subsequent elimination was postulated, which was highly efficient and metal-free under mild conditions. Moreover, flexible structural modifications of quinoxalines bearing carbonyl groups are of interest for further transformations as building blocks in organic synthesis.
Quinoxaline represents one of the most prevalent heterocycles in natural products.1 It has enjoyed extensive applications in pharmaceuticals due to its various biological activities such as antimicrobial, antiviral, antidiabetic, anti-inflammatory, anticancer, and antidepressant properties.2,3 Moreover, quinoxalines are the building blocks used in the preparation of porphyrins,4 dyes,5 electroluminescent materials,6 cavitand and salen ligands.7 Among these, quinoxalines bearing carbonyl groups are important structural motifs in bioactive molecules (Fig. 1), such as compounds a and b, which are used as potential photoprotective drugs and anticancer and hypoxia-selective agents.8 They are also the building blocks of some quinoxaline metal complexes, such as compounds c and d, which possess significant antimicrobial and anticancer activities.9
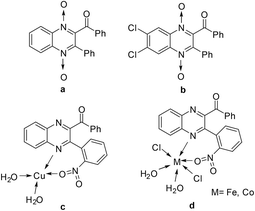 |
| Fig. 1 Selected bioactive molecules with quinoxaline bearing a carbonyl group moiety. | |
Consequently, a variety of strategies have been developed to prepare such a core. The conventional procedure for the synthesis of quinoxalines involves the condensation of o-disubstituted benzene with two-carbon synthons such as 1,2-dicarbonyl compounds,10 α-hydroxy ketones,11 vicinal diols,12 phenacyl bromides,13 epoxides,14 and alkynes.15 Recently, transition metal-catalyzed domino cyclization reactions16 have been found to be efficient. However, there are still some respective limitations such as the use of problematically available or dangerous starting materials, harsh reaction conditions, poor regioselectivity, and the requirement of noble metals as catalysts, which may lead to potential contamination in products and impede their applications, especially in the pharmaceutical industry. Therefore, the development of an efficient and metal-free route to obtain quinoxalines under mild reaction conditions remains highly desirable.
Owing to the didentate nucleophilicity and electrophilicity of enaminones, various heterocycles can be synthesized from enaminones.17,18 In 2016, a convenient regiospecific synthesis of quinoxalines involving base-promoted C-α-CH2-extrusion from enaminones under metal-free conditions was developed by our group.19 Due to our continued interest in the development of new strategies for the synthesis of heterocycles based on enaminones under metal-free conditions,20a−h we recently reported the preliminary results of a simple, mild and highly atom-efficient synthesis of 2-hydroxy-benzo[b][1,4]oxazins from N-(2-hydroxylaryl) enaminones via a hypervalent iodine(III)-promoted intramolecular iminoenol trapping reaction (Scheme 1a).20g,21 As an extension of this methodology and the synthesis of versatile quinoxalines, N-(2-acetaminophenyl)enaminones as starting materials under appropriate hypervalent iodine(III)-mediated conditions were explored (Scheme 1b). It was proven to be an expedient and simple strategy to access quinoxalines.
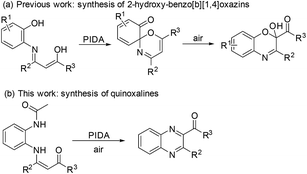 |
| Scheme 1 Hypervalent iodine(III)-mediated synthesis of heterocycles from enaminones. | |
Initially, N-(2-acetaminophenyl)enaminone 1a was used as the standard substrate to search for suitable reaction conditions. We were pleased to find that the reaction of 1a with PIDA (iodobenzene diacetate) in EtOH at 80 °C successfully afforded the desired product quinoxaline 2a in 58% isolated yield (Table 1, entry 1). Then, a series of solvents such as DCE, 1,4-dioxane, DMF, and toluene were tested; toluene was found to be the most efficient solvent (entries 2–5). Subsequent studies showed that other hypervalent iodine reagents (PIFA, PhI + m-CPBA) were less effective for the formation of quinoxalines compared to PIDA (entries 6 and 7). When the loading of PIDA was reduced to 0.3 equiv., the yield dropped dramatically (entry 8). Temperature screening experiments revealed that the reaction at 80 °C gave the best yield (entries 9 and 10). The screening of further additives showed that AcOH, TsOH, Et3N and K2CO3 were inferior to PhCOOH (entries 11–15). The reaction did not improve significantly by increasing the loading of PhCOOH (entry 16). No satisfactory result was obtained when a catalytic amount of PhCOOH was used (entry 17). Oxygen seemed to be indispensable to the reaction since a decreased yield was obtained when the reaction was carried out under an N2 atmosphere (entries 18 and 19). Finally, the optimal reaction conditions for the PIDA-promoted synthesis of quinoxaline derivatives were identified as follows: 1.1 equiv. of PIDA as the oxidant, 1.0 equiv. of PhCOOH as the additive and toluene as the solvent at 80 °C under air.
Table 1 Optimization of the reaction conditionsa
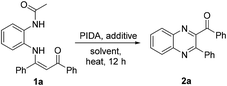
|
Entry |
Solvent |
T (°C) |
[O] (equiv.) |
Additive (equiv.) |
Yieldsb (%) |
Reaction conditions: 1a (0.2 mmol) and iodine compound in solvent (2 mL) at a corresponding temperature for 12 h under air atmosphere. Isolated yields. Under O2. Under N2. PIFA = phenyliodine(III) bis(trifluoroacetate), m-CPBA = 3-chloroperoxybenzoic acid. |
1 |
EtOH |
80 |
PIDA (1.1) |
— |
58 |
2 |
DCE |
80 |
PIDA (1.1) |
— |
72 |
3 |
1,4-Dioxane |
80 |
PIDA (1.1) |
— |
62 |
4 |
DMF |
80 |
PIDA (1.1) |
— |
34 |
5 |
Toluene |
80 |
PIDA (1.1) |
— |
79 |
6 |
Toluene |
80 |
PIFA (1.1) |
— |
67 |
7 |
Toluene |
80 |
PhI (10 mol%) + m-CPBA (2) |
— |
Trace |
8 |
Toluene |
80 |
PIDA (0.3) |
— |
36 |
9 |
Toluene |
60 |
PIDA (1.1) |
— |
75 |
10 |
Toluene |
100 |
PIDA (1.1) |
— |
70 |
11 |
Toluene |
80 |
PIDA (1.1) |
AcOH (1.0) |
59 |
12 |
Toluene |
80 |
PIDA (1.1) |
TsOH (1.0) |
21 |
13 |
Toluene |
80 |
PIDA (1.1) |
PhCOOH (1.0) |
90 |
14 |
Toluene |
80 |
PIDA (1.1) |
Et3N (1.0) |
49 |
15 |
Toluene |
80 |
PIDA (1.1) |
K2CO3 (1.0) |
72 |
16 |
Toluene |
80 |
PIDA (1.1) |
PhCOOH (2.0) |
92 |
17 |
Toluene |
80 |
PIDA (1.1) |
PhCOOH (0.1) |
80 |
18c |
Toluene |
80 |
PIDA (1.1) |
PhCOOH (1.0) |
88 |
19d |
Toluene |
80 |
PIDA (1.1) |
PhCOOH (1.0) |
25 |
Under the optimized reaction conditions (Table 1, entry 13), we evaluated the scope of this developed method by using various substituted N-(2-acetaminophenyl)enaminones. As shown in Scheme 2, R1 and R2 in substrate 1 can be either electron-rich or electron-deficient aryl groups and provide the corresponding quinoxalines in 44–93% yields (2b–2k, 2n–2r). N-(2-Acetaminophenyl)enaminones 1 with electron-donating groups at the para position of the phenyl ring reacted smoothly to afford the expected quinoxalines in desirable yields (2d, 2f, and 2g), while the yields for the substrates bearing electron-withdrawing groups were relatively lower (2h, 2i). Due to steric hindrance, the substrates with para-substituents (2d) gave slightly higher yields than those with ortho- and meta-substituents (2b, 2c). Halogens, including F, Cl, and Br, worked well (2i–2k, 2p–2r, 53–73% yields), which could be further extended to subsequent transition metal-catalyzed coupling reactions. Heteroaryl groups, such as furyl and thienyl groups, were also suitable and gave the desired products in 42% and 43% yields, respectively (2l, 2m). Moreover, when the R2 group was a cyclopropyl group, this transformation could still proceed smoothly, giving the desired product in 35% yield (2s). A trace amount of the desired product was obtained when R2 was an aliphatic group and enamino esters were used as the substrate.
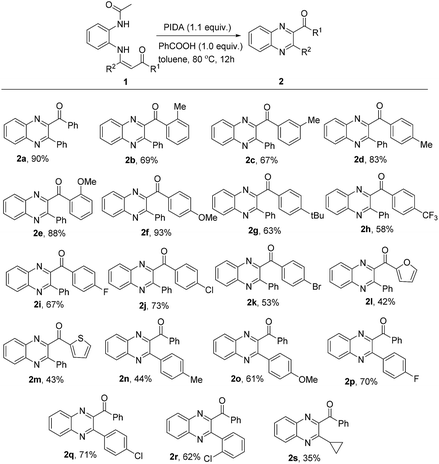 |
| Scheme 2 Substrate scope of enaminones. aReaction conditions: 1a (0.2 mmol), PIDA (0.22 mmol), PhCOOH (0.2 mmol) in toluene (2 mL) at 80 °C for 12 h. bIsolated yields. | |
The flexible structural modification of quinoxalines bearing carbonyl groups indicates their further transformations to useful molecules.22 For example, the reduction of 2a with KBH4 furnished 3a in 85% yield, which showed some activity to prevent brain damage and neurodegenerative diseases (Scheme 3a).23 Subsequent cyclization in the presence of concentrated H2SO4 afforded the condensed quinoxaline 4a, which is a pharmacophoric structure motif with antifungal activity (Scheme 3b).24
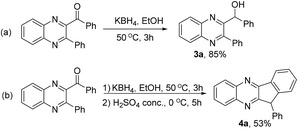 |
| Scheme 3 Further transformations of 2a. | |
To probe the possible reaction mechanism, some control experiments were carried out (Scheme 4). The radical scavengers TEMPO (2,2,6,6-tetramethylpiperidine, 1-oxy) and DPE (1,1-diphenylethylene) resulted in yields of 68% and 55%, respectively (eqn (1)), which indicated that a radical pathway might not be involved in this reaction. When N-(2-acetaminophenyl)enaminone 1a was reacted under the standard conditions for 2 h, intermediate 5a was isolated in 32% yield, which could be transformed into the desired product 2a in 93% yield (eqn (2)). As expected, the intermediate 5a could be transformed into the desired product 2a in 41% yield under standard conditions without PIDA and PhCOOH (eqn (3)), while a higher yield (84%) was obtained in the presence of PhCOOH (eqn (4)), indicating that PhCOOH could promote the process from intermediate 5a to the desired product 2a.
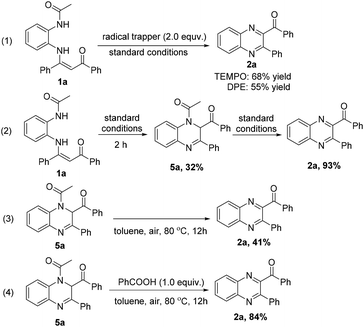 |
| Scheme 4 Control experiments. | |
A plausible mechanism is proposed in Scheme 5 according to the aforementioned results and reported literatures.25 The initial reaction of 1a and PIDA afforded α-iodo iminoketone A. Then, intramolecular condensation cyclization of A with the concomitant release of PhI and CH3COOH afforded 5a. Subsequently, the oxidation of 5a provided B, which was detected by HRMS (ESI, Fig. S1†) in the presence of O2. Finally, the elimination of CH3COOH from B generated the final product 2a.
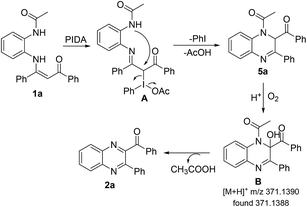 |
| Scheme 5 Proposed reaction mechanism. | |
In conclusion, an efficient hypervalent iodine(III)-mediated approach to substituted quinoxalines from readily available N-(2-acetaminophenyl)enaminones was developed. This reaction tolerated a wide range of functional groups in moderate to excellent yields under mild and metal-free conditions. Due to the importance of quinoxalines, this protocol can be further expanded to the synthesis of biologically and medicinally relevant compounds.
Conflicts of interest
There are no conflicts to declare.
Acknowledgements
This research was supported by NSF of China (21572072), 111 project (BC2018061), Xiamen Southern Oceanographic Center (15PYY052SF01).
Notes and references
-
(a) J. B. Laursen, P. C. de Visser, H. K. Nielsen, K. J. Jensen and J. Nielsen, Bioorg. Med. Chem. Lett., 2002, 12, 171 CrossRef CAS PubMed;
(b) M. S. Abdelfattah, T. Kazufumi and M. Ishibashi, J. Nat. Prod., 2010, 73, 1999 CrossRef CAS PubMed.
-
(a) G. Sakata and K. Makino, Heterocycles, 1988, 27, 2481 CrossRef CAS;
(b) T. Mathew, A. Á. Papp, F. Paknia, S. Fustero and G. K. S. Prakash, Chem. Soc. Rev., 2017, 46, 3060 RSC;
(c) J. A. Pereira, A. M. Pessoa, M. N. D. S. Cordeiro, R. Fernandes, C. Prudêncio, J. P. Noronha and M. Vieira, Eur. J. Med. Chem., 2015, 97, 664 CrossRef CAS PubMed.
-
(a) A. Gomtsyan, E. K. Bayburt, R. G. Schmidt, G. Z. Zheng, R. J. Perner, S. Didomenico, J. R. Koenig, S. Turner, T. Jinkerson and I. Drizin, J. Med. Chem., 2005, 48, 744 CrossRef CAS PubMed;
(b) W. He, M. R. Myers, B. Hanney, A. P. Spada, G. Bilder, H. Galzcinski, D. Amin, S. Needle, K. Page, Z. Jayyosi and M. H. Perrone, Bioorg. Med. Chem. Lett., 2003, 13, 3097 CrossRef CAS PubMed;
(c) A. Jaso, B. Zarranz, I. Aldana and A. Monge, J. Med. Chem., 2005, 48, 2019 CrossRef CAS PubMed;
(d) R. Sarges, H. R. Howard, R. G. Browne, L. A. Lebel, P. A. Seymour and B. K. Koe, J. Med. Chem., 1990, 33, 2240 CrossRef CAS PubMed;
(e) L. E. Seitz, W. J. Suling and R. C. Reynolds, J. Med. Chem., 2002, 45, 5604 CrossRef CAS PubMed;
(f) R. Rajule, V. C. Bryant, H. Lopez, X. Luo and A. Natarajan, Bioorg. Med. Chem., 2012, 20, 2227 CrossRef CAS PubMed.
-
(a) J. L. Sessler, H. Maeda, T. Mizuno, V. M. Lynch and H. Furuta, J. Am. Chem. Soc., 2002, 124, 13474 CrossRef CAS PubMed;
(b) M. J. Crossley, P. J. Sintic, J. A. Hutchinson and K. P. Ghiggino, Org. Biomol. Chem., 2005, 3, 852 RSC.
-
(a) N. D. Sonawane and D. W. Rangnekar, J. Heterocycl. Chem., 2002, 39, 303 CrossRef CAS;
(b) T. Hirayama, S. Yamasaki, H. Ameku, T. Ishii, T. Thiemann and S. Mataka, Dyes Pigm., 2005, 67, 105 CrossRef CAS.
-
(a) S. Dailey, J. W. Feast, R. J. Peace, R. C. Sage, S. Till and E. L. Wood, J. Mater. Chem., 2001, 11, 2238 RSC;
(b) K. R. J. Thomas, M. Velusamy, J. T. Lin, C. H. Chuen and Y. T. Tao, Chem. Mater., 2005, 17, 1860 CrossRef CAS;
(c) S. Achelle, C. Baudequin and N. Plé, Dyes Pigm., 2013, 98, 575 CrossRef CAS.
-
(a) P. P. Castro, G. Zhao, G. A. Masangkay, C. Hernandez and L. M. Gutierrez-Tunstad, Org. Lett., 2004, 6, 333 CrossRef CAS PubMed;
(b) X. H. Wu, A. E. V. Gorden, S. A. Tonks and J. Z. Vilseck, J. Org. Chem., 2007, 72, 8691 CrossRef CAS PubMed.
-
(a) J. Mouawad, F. Saadeh, H. A. Tabosh, M. J. Haddadin and H. Gali-Muhtasib, Med. Oncol., 2016, 33, 86 CrossRef PubMed;
(b) H. Gali-Muhtasib, M. Haddadin, D. Rahhal and I. Younes, Oncol. Rep., 2001, 8, 679 CAS;
(c) M. Diab-Assef, M. J. Haddadin, P. Yared, C. Assaad and H. Gali-Muhtasib, Mol. Carcinog., 2002, 33, 198 CrossRef CAS PubMed;
(d) W. Itani, F. Geara, J. Haykal, M. Hadadin and H. Gali-Muhtasib, Radiat. Oncol., 2007, 2, 1 CrossRef PubMed;
(e) M. El-khatib, F. Geara, M. J. Haddadin and H. Gali-Muhtasib, Radiat. Oncol., 2010, 5, 107 CrossRef PubMed.
- S. J. Kirubavathy, R. Velmurugan, K. Parameswari and S. Chitra, Int. J. Pharma Sci. Res., 2014, 5, 2508 Search PubMed.
-
(a) Z. Zhao, D. D. Wisnoski, S. E. Wolkenberg, W. H. Leister, Y. Wang and C. W. Lindsley, Tetrahedron Lett., 2004, 45, 4873 CrossRef CAS;
(b) R. S. Bhosale, S. R. Sarda, S. S. Ardhapure, W. N. Jadhav, S. R. Bhusare and R. P. Pawar, Tetrahedron Lett., 2005, 46, 7183 CrossRef CAS;
(c) S. V. More, M. N. V. Sastry and C. F. Yao, Green Chem., 2006, 8, 91 RSC;
(d) C. Srinivas, C. N. S. S. P. Kumar, V. J. Rao and S. Palaniappan, J. Mol. Catal. A: Chem., 2007, 265, 227 CrossRef CAS;
(e) M. Ayaz, Z. Xu and C. Hulme, Tetrahedron Lett., 2014, 55, 3406 CrossRef CAS PubMed;
(f) K. B. Harsha and K. S. Rangappa, RSC Adv., 2016, 6, 57154 RSC;
(g) K. S. Indalkar, C. K. Khatri and G. U. Chaturbhuj, J. Chem. Sci., 2017, 129, 141 CrossRef CAS.
-
(a) S. Y. Kim, K. H. Park and Y. K. Chung, Chem. Commun., 2005, 1321 RSC;
(b) V. Jeena and R. S. Robinson, Beilstein J. Org. Chem., 2009, 5, 24 Search PubMed;
(c) A. Shaabani and A. Maleki, Chem. Pharm. Bull., 2008, 56, 79 CrossRef CAS PubMed;
(d) S. Sithambaram, Y. Ding, W. Li, X. Shen, F. Gaenzler and S. L. Suib, Green Chem., 2008, 10, 1029 RSC;
(e) J. Richard, Chem. Commun., 2003, 2286 Search PubMed;
(f) A. Kamal, K. S. Babu, S. Faazil, S. A. Hussaini and A. B. Shaik, RSC Adv., 2014, 4, 46369 RSC;
(g) A. Kamal, K. S. Babu, S. A. Hussaini, R. Mahesh and A. Alarifi, Tetrahedron Lett., 2015, 56, 2803 CrossRef CAS;
(h) V. Jeena and R. S. Robinson, Tetrahedron Lett., 2014, 55, 642 CrossRef CAS;
(i) M. Jeganathan, A. Dhakshinamoorthy and K. Pitchumani, Tetrahedron Lett., 2014, 55, 1616 CrossRef CAS.
- C. S. Cho and S. G. Oh, Tetrahedron Lett., 2006, 47, 5633 CrossRef CAS.
-
(a) K. Kumar, S. R. Mudshinge, S. Goyal, M. Gangar and V. A. Nair, Tetrahedron Lett., 2015, 56, 1266 CrossRef CAS;
(b) B. Madhav, S. N. Murthy, V. P. Reddy, K. R. Rao and Y. Nageswar, Tetrahedron Lett., 2009, 50, 6025 CrossRef CAS;
(c) H. Meshram, G. S. Kumar, P. Ramesh and B. C. Reddy, Tetrahedron Lett., 2010, 51, 2580 CrossRef CAS;
(d) B. Tanwar, P. Purohit, B. N. Raju, D. Kumar, D. N. Kommi and A. K. Chakraborti, RSC Adv., 2015, 5, 11873 RSC;
(e) J. P. Wan, S. F. Gan, J. M. Wu and Y. Pan, Green Chem., 2009, 11, 1633 RSC.
-
(a) S. Antoniotti and E. Dũnach, Tetrahedron Lett., 2002, 43, 3971 CrossRef CAS;
(b) M. M. Ibrahim, D. Grau, F. Hampel and S. B. Tsogoeva, Eur. J. Inorg. Chem., 2014, 14015 Search PubMed;
(c) E. C. Taylor, C. A. Maryanoff and J. S. Skotnicki, J. Org. Chem., 1980, 45, 2512 CrossRef CAS;
(d) A. Kumar, S. kumar, A. Saxena, A. De and S. Mozumdar, Catal. Commun., 2008, 9, 778 CrossRef CAS.
-
(a) W. Wang, Y. Shen, X. Meng, M. Zhao, Y. Chen and B. Chen, Org. Lett., 2011, 13, 4514 CrossRef CAS PubMed;
(b) S. Okumura, Y. Takeda, K. Kiyokawa and S. Minakata, Chem. Commun., 2013, 49, 9266 RSC;
(c) S. Shi, T. Wang, W. Yang, M. Rudolph and A. S. K. Hashmi, Chem.–Eur., 2013, 19, 6576 CrossRef CAS PubMed;
(d) C. Y. Chen, W. P. Hu, M. C. Liu, P. C. Yan, J. J. Wang and M. I. Chung, Tetrahedron, 2013, 69, 9735 CrossRef CAS;
(e) K. K. D. R. Viswanadham, M. P. Reddy, P. Sathyanarayana, O. Ravi, R. Kant and S. R. Bathula, Chem. Commun., 2014, 50, 13517 RSC;
(f) Z. Wang, G. Hu, J. Liu, W. Liu, H. Zhang and B. Wang, Chem. Commun., 2015, 51, 5069 RSC.
-
(a) R. P. Pandit, S. H. Kim and Y. R. Lee, Adv. Synth. Catal., 2016, 358, 3586 CrossRef CAS;
(b) H. Ma, D. Li and W. Yu, Org. Lett., 2016, 18, 868 CrossRef CAS PubMed;
(c) H. Liu, T. Duan, Z. Zhang, C. Xie and C. Ma, Org. Lett., 2015, 17, 2932 CrossRef CAS PubMed;
(d) C. Deraedt, R. Ye, W. T. Ralston, F. D. Toste and G. A. Somorjai, J. Am. Chem. Soc., 2017, 139, 18084 CrossRef CAS PubMed;
(e) T. F. Chen, X. Chen, J. Wei, D. G. Lin, Y. Xie and W. Zeng, Org. Lett., 2016, 18, 2078 CrossRef CAS PubMed.
- For recent reviews, see:
(a) A.-Z. A. Elassar and A. A. El-Khair, Tetrahedron, 2003, 59, 8463 CrossRef CAS;
(b) B. Stanovnik and J. Svete, Chem. Rev., 2004, 104, 2433 CrossRef CAS PubMed;
(c) B. Govindh, B. S. Diwakar and Y. L. Murthy, Org. Commun., 2012, 5, 105 Search PubMed;
(d) A. K. Chattopadhyay and S. Hanessian, Chem. Commun., 2015, 51, 16437 RSC;
(e) A. K. Chattopadhyay and S. Hanessian, Chem. Commun., 2015, 51, 16450 RSC;
(f) J. P. Wan and Y. Gao, Chem. Rec., 2016, 16, 1164 CrossRef CAS PubMed.
- For recent examples, see:
(a) Z. Gu, C. Jia, S. Wang and S. Ji, Chem. Sci., 2016, 7, 4067 RSC;
(b) K. Goutham, D. A. Kumar, S. Suresh, B. Sridhar, R. Narender and G. V. Karunakar, J. Org. Chem., 2015, 80, 11162 CrossRef CAS PubMed;
(c) R. L. Yan, X. N. Li, X. D. Yang, X. Kang, L. K. Xiang and G. S. Huang, Chem. Commun., 2015, 51, 2573 RSC;
(d) L. Z. Yu, H. Z. Wei and M. Shi, Adv. Synth. Catal., 2018, 360, 1967 CrossRef CAS;
(e) J. Liu, W. Wei, T. Zhao, X. Y. Liu, J. Wu, W. Q. Yu and J. B. Chang, J. Org. Chem., 2016, 81, 9326 CrossRef CAS PubMed;
(f) H. C. Ma, D. J. Li and W. Yu, Org. Lett., 2016, 18, 868 CrossRef CAS PubMed;
(g) C. Zheng, Y. Wang and R. H. Fan, Org. Lett., 2015, 17, 916 CrossRef CAS PubMed;
(h) X. Q. Sun, Y. R. Lyu, D. Zhang-Negrerie, Y. F. Du and K. Zhao, Org. Lett., 2013, 15, 6222 CrossRef CAS PubMed;
(i) J. Ke, C. He, H. Y. Liu, M. J. Li and A. W. Lei, Chem. Commun., 2013, 49, 7549 RSC;
(j) J. P. Wan, Y. Y. Zhou, Y. Y. Liu and S. R. Sheng, Green Chem., 2016, 18, 402 RSC;
(k) G. Cheng, W. Lv and L. Xue, Green Chem., 2018, 20, 4414 RSC;
(l) X. Mao, T. Tong, S. Fan, L. Fang, J. Wu, X. Wang, H. Kang and X. Lv, Chem. Commun., 2017, 53, 4718 RSC;
(m) W. Fan, Y. R. Li, Q. Li, B. Jiang and G. G. Li, Tetrahedron, 2016, 47, 4867 CrossRef.
- J. H. Shen, X. D. Wang, X. Lin, Z. H. Yang, G. L. Cheng and X. L. Cui, Org. Lett., 2016, 18, 1378 CrossRef CAS PubMed.
-
(a) G. L. Cheng, X. B. Zeng, J. H. Shen, X. S. Wang and X. L. Cui, Angew. Chem., Int. Ed., 2013, 52, 13265 CrossRef CAS PubMed;
(b) J. H. Shen, G. L. Cheng and X. L. Cui, Chem. Commun., 2013, 49, 10641 RSC;
(c) G. L. Cheng, Y. X. Weng, X. F. Yang and X. L. Cui, Org. Lett., 2015, 17, 3790 CrossRef CAS PubMed;
(d) J. H. Shen, D. D. Cai, C. S. Kuai, Y. Q. Liu, M. E. Wei, G. L. Cheng and X. L. Cui, J. Org. Chem., 2015, 80, 6584 CrossRef CAS PubMed;
(e) J. H. Shen, L. L. Xue, X. Lin, G. L. Cheng and X. L. Cui, Chem. Commun., 2016, 52, 3293 Search PubMed;
(f) J. H. Shen, X. F. Yang, F. Y. Wang, Y. Wang, G. L. Cheng and X. L. Cui, RSC Adv., 2016, 6, 48905 RSC;
(g) H. zhang, J. H. Shen, G. L. Cheng, Y. D. Feng and X. L. Cui, Org. Lett., 2018, 20, 664 CrossRef CAS PubMed;
(h) H. Zhang, J. H. Shen, G. L. Cheng, B. J. Wu and X. L. Cui, Asian J. Org. Chem., 2018, 7, 1089 CrossRef CAS.
- A. Yoshimura and V. V. Zhdankin, Chem. Rev., 2016, 116, 3328 CrossRef CAS PubMed.
- V. Nicole and M. Pierre, Bull. Soc. Chim. Fr., 1977, 11–12, 1245 Search PubMed.
- G. Moshe, V. J. Arieh, S. Alex and M. Ilan, US Pat. 8541428, 2013.
- G. Matolcsy, M. Kovacs, M. Tueske and B. Toth, Neth. J. Plant Pathol., 1977, 83(suppl. 1), 39 CrossRef CAS.
-
(a) F. F. Zhao, X. Liu, R. Qi, D. Zhang-Negrerie, J. H. Huang, Y. F. Du and K. Zhao, J. Org. Chem., 2011, 76, 10338 CrossRef CAS PubMed;
(b) X. Q. Sun, Y. R. Lyu, D. Zhang-Negrerie, Y. F. Du and K. Zhao, Org. Lett., 2013, 15, 6222 CrossRef CAS PubMed;
(c) B. S. P. A. Kumar, B. Madhav, K. H. V. Reddy and Y. V. D. Nageswar, Tetrahedron Lett., 2011, 56, 2862 CrossRef.
Footnote |
† Electronic supplementary information (ESI) available. See DOI: 10.1039/c9ra01200a |
|
This journal is © The Royal Society of Chemistry 2019 |