DOI:
10.1039/C9RA01103J
(Paper)
RSC Adv., 2019,
9, 13757-13764
Efficient resolution of 3-aryloxy-1,2-propanediols using CLEA-YCJ01 with high enantioselectivity†
Received
12th February 2019
, Accepted 14th March 2019
First published on 3rd May 2019
Abstract
The lipase YCJ01 from Burkholderia ambifaria is an organic solvent-stable enzyme and its activity can be activated by a hydrophobic solvent due to the “interface activation” mechanism. The activity of lipase YCJ01 increased by 2.1-fold with t-butanol as the precipitant even after cross-linking. The cross-linked enzyme aggregates of lipase YCJ01 (CLEAs-YCJ01) were found to be efficient for resolving 3-(4-methylphenoxy)-1,2-propanediol (MPPD) through sequential esterification. Excellent enantioselectivity towards MPPD (E > 400), excellent enantiomeric excess (ee) values of 99.2% for S-diacetates and 99.1% for R-monoacetate, and high yield (49.9%) were achieved using a high substrate concentration (180 mmol L−1). Thus, R- and S-type compounds with excellent ee values were simultaneously obtained, and MPPD was resolved by CLEAs-YCJ01. CLEAs-YCJ01 also showed high operational stability and maintained 91.2% residual activity after ten batches. To further evaluate the substrate specificity of CLEAs-YCJ01, a series of 3-aryloxy-1,2-propanediols (six analogues of MPPD) was applied as substrates for resolution. Under the optimized reaction conditions of reaction temperature of 35 °C, MPPD concentration of 180 mmol L−1, molar ratio of vinyl acetate to MPPD of 3
:
1, and isopropyl ether as the solvent, CLEAs-YCJ01 exhibited relatively strict enantioselectivity towards all the analogues of MPPD with a high yield (≥49.3%), favourable ee values (94.8–99.4%) for S-diacetates, and high ee values (92.1–99.2%) for R-monoacetate, which shows potential prospects for industrial applications.
Introduction
Enantiopure 3-aryloxy-1,2-propanediols (APPDs) comprise a class of important precursors for chiral drugs and pesticides. S-type APPDs are synthetic intermediates for the synthesis of cardiac propranolol, which is a drug for the treatment of cardiovascular diseases.1,2 They can also be used to synthesize phosphodiesterase inhibitors3,4 for the treatment of heart disease. Furthermore, R-type APPDs are precursors for a synthetic insect growth regulator.5,6 Therefore, considerable research has been performed on preparation methods for chiral APPDs, which can be divided into two categories.
The first category comprises chemical methods. The enantioselective esterification of the secondary hydroxyl group needs a chiral chemical catalyst. Chiral nanoporous metal–metallosalen frameworks7 are used as catalysts to produce R-3-benzoxy-1,2-propanediols, and the ee values of the products range from 87% to 99%. However, these frameworks have complex structures and their preparation includes complex steps. Therefore, the second category of methods for the preparation of chiral APPDs, namely enzymatic methods, is becoming increasingly attractive. Several epoxides at a 10 mM concentration were resolved to produce S-3-benzoxyl-1,2-propanediols using an epoxide hydrolase derived from Aspergillus niger,8 and the enantioselectivity values ranged between 22 and 60 after 2 h. In another study, a recombinant epoxide hydrolase from Yarrowia lipolytica9 was applied as a catalyst for the preparation of S-3-phenoxy-1,2-propanediols, and the ee values of the products were 95%. However, water-dependent side reactions such as the non-catalytic hydrolysis of epoxides resulted in a decrease in the ee value and yield of the product.10,11 On the other hand, most epoxide hydrolases preferentially hydrolyze racemic epoxides into the S configuration of the formed diol, which limits their applications in the preparation of R-type diols.
Lipases (esterases) are often applied for the synthesis, hydrolysis, and transesterification of esters and exhibit enantioselective properties.12 For example, the dicarboxyesters of 1,2-propanediols were sequentially hydrolyzed by an esterase13 to obtain (S)-1-phenyl-1,2-ethanediol with an ee of 96%. Also, chiral 3-aryloxy-1,2-propanediols can be directly obtained through sequential esterification with a lipase. Theil's group14,15 used Amano lipase PS to separate 3-aryloxy-1,2-propanediols, yielding R-type and S-type compounds with ee values in the range of 55–99.8% and 79–96.8%, respectively, resulting in moderate enantioselectivity towards the S-type compounds. The lipase PS is hard to apply in the preparation of S-type compounds.
The immobilization of the enzyme affords improved operational stability and allows its facile separation and reuse.16 Thus, effective methodologies for enzyme immobilization as CLEAs (cross-linked enzyme aggregates) are applicable to a broad range of enzymes, and CLEAs are proven to be significantly more stable to denaturation by heat, organic solvents and proteolysis than the corresponding soluble enzyme or lyophilized (freeze-dried) powder.17 In this work, CLEAs of lipase YCJ01 from Burkholderia ambifaria18 (CLEAs-YCJ01) were prepared and evaluated for the resolution of 3-(4-methylphenoxy)-1,2-propanediol (MPPD). CLEAs-YCJ01 showed strict enantioselectivity towards MPPD and excellent ee values were simultaneously obtained for both R- and S-type compounds. A series of 3-aryloxy-1,2-propanediols was also used to evaluate the substrate specificity of CLEAs-YCJ01.
Results and discussion
Selection of precipitating agent for preparation of CLEAs-YCJ01
The selection of an appropriate precipitating agent is very important for the preparation of CLEAs-YCJ01. According to the previous study on the stability of lipase YCJ01 in various organic solvents (25%, v/v) by Yao,18 the lipase YCJ01 is stable in ethanol, 1-propyl alcohol, 2-propyl alcohol, t-butanol and acetone. In addition, the commonly used protein precipitant, saturated ammonium sulfate solution, was considered. Therefore, these solvents were selected as the precipitants in our present study. Their effects on the activity recovery of CLEAs-YCJ01 are shown in Table 1. The type of precipitant used has a great influence on the activity recovery of CLEAs-YCJ01. When saturated ammonium sulfate solution was used as the precipitant, the activity recovery of CLEAs-YCJ01 was 77.9%; however, when the precipitant was changed to organic solvents, the activity recovery of CLEAs-YCJ01 was higher than 100%. Specially, 214.8% of activity recovery was achieved using t-butanol as the precipitant. These results may be closely related to the “interface activation” mechanism of lipase. Most lipases have an activity center featuring an α-helical amphipathic “lid”.19 In a hydrophobic environment or on an oil–water interface, the lid is opened outwards from the active center so that it does not hinder the entry of the substrate into the active center and the lipase shows an active form. In this study, the organic solvents used as precipitants could trigger the “interface activation” mechanism. In the solvent t-butanol with higher hydrophobicity, the activity of lipase YCJ01 may be activated efficiently and the active form was kept after cross-linking. Moreover, the solvent t-butanol with higher hydrophobicity may induce the aggregation of enzyme proteins in a shorter time, which reduced the loss of enzymatic activity.20
Table 1 Special activity and activity recovery of CLEAs-YCJ01 in different precipitantsc
Precipitant |
Specific activitya (×104 U g−1) |
Activity recoveryb (%) |
Special activity = (activity in the CLEAs/weight of CLEAs) × 100%. Activity recovery = (activity in the CLEAs/activity of lipase solution used) × 100%. Conditions: protein concentration of enzyme solution: 4.0 mg mL−1, activity of lipase YCJ01: 176.6 U mL−1, volume of precipitant : volume of enzyme solution = 3 : 1, cross-linking time = 4 h and glutaraldehyde amount = 0.25% (v/v, %). |
Ethanol |
4.44 ± 0.20 |
100.6 ± 4.6 |
1-Propanol |
5.26 ± 0.30 |
119.1 ± 6.9 |
2-Propanol |
5.63 ± 0.24 |
127.5 ± 5.5 |
Acetone |
4.83 ± 0.32 |
109.3 ± 7.2 |
t-Butanol |
10.86 ± 0.37 |
214.8 ± 8.3 |
Saturated ammonium sulfate solution |
3.44 ± 0.17 |
77.9 ± 3.8 |
Glutaraldehyde is the most commonly used cross-linker for the preparation of CLEAs. The effect of glutaraldehyde concentration on the activity recovery of CLEAs-YCJ01 is shown in Table 2. As the glutaraldehyde concentration increased from 0.05% (v/v) to 0.25% (v/v), the activity recovery reached a high level (214.8%). Further increasing the amount of glutaraldehyde caused a great loss in the activity of CLEAs-YCJ01 and a low activity recovery. Therefore, 0.25% was selected as the optimum glutaraldehyde amount.
Table 2 Effect of the amount of glutaraldehyde on CLEAs-YCJ01 activity
Amounta (v/v, %) |
Specific activitya (×104 U g−1) |
Activity recoveryb (%) |
Amount of glutaraldehyde = glutaraldehyde volume/enzyme volume × 100%. Reaction conditions: enzyme protein concentration = 4.0 mg mL−1, activity of enzyme solution = 176.6 U mL−1, volume of t-butanol : volume of enzyme = 3 : 1, and cross-linking time = 4 h. |
0.05 |
13.02 ± 0.46 |
165.8 ± 10.4 |
0.15 |
11.87 ± 0.28 |
192.3 ± 6.3 |
0.2 |
11.53 ± 0.37 |
211.3 ± 8.4 |
0.25 |
10.86 ± 0.37 |
214.8 ± 8.3 |
0.3 |
9.99 ± 0.37 |
202.8 ± 8.4 |
0.35 |
7.97 ± 0.48 |
166.4 ± 10.8 |
0.375 |
6.12 ± 0.41 |
130.0 ± 9.3 |
Optimization for the resolution of MPPD by CLEAs-YCJ01
As shown in the Scheme 1, the reaction was carried out with vinyl acetate in the presence of CLEAs-YCJ01. 3-(4-Methylphenoxy)-1,2-propanediol (MPPD, substrate 2) was firstly formed into primary monoacetate, and the formed monoacetate was enantioselectively converted into the S-diacetates. In the next experiment, the conditions of resolution were optimized to improve the reaction rate and ee values.
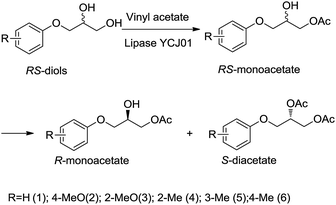 |
| Scheme 1 Enzymatic resolution of racemic 3-aryloxy-1,2-propanediols. | |
The effect of organic solvents on the resolution of MPPD. In non-aqueous enzymology, medium engineering is an effective means to control and regulate the structure and function of enzymes.21 Thus, the effects of six solvents with different hydrophobicities (log
P, n-hexane/water distribution coefficient) on the activity and enantioselectivity of CLEAs-YCJ01 were investigated. As shown in Table 3, in the moderately hydrophobic isopropyl ether, the conversion of MPPD reached 49.1%; however, in the hydrophilic solvents tetrahydrofuran (THF) and acetone, the conversions were 41.6% and 43.2%, respectively. In the hydrophilic solvents, the “critical water” layer at the enzymatic surface is stripped off by the solvent,22 resulting in an increase in the rigidity of the enzyme structure and a decrease in its reaction activity. In isopropyl ether, the “critical water” layer on the enzymatic surface was maintained, which favored the flexibility of the enzyme, and thus the enzyme retained its high activity. Meanwhile, in hexane and toluene with higher hydrophobicity, the activity of CLEAs-YCJ01 decreased, which may be because these highly hydrophobic solvents altered the microenvironment of the active center, which hindered the proper orientation of the substrates in the active center and led to a reduction in the reaction rate.23 Thus, a solvent with moderate hydrophobicity is beneficial to maintain the “critical water” of the enzymatic surface and also facilitate the proper orientation of substrates in its active center.
Table 3 Resolution of racemic MPPD in non–aqueous solvents
Catalyst |
Solvent |
log P |
Initial rates (μmol min−1) |
ee of S-diacetates |
c (%) |
Ea |
E = ln[(1 − c)(1 − ees)/(1 − c)(1 + ees)], reaction conditions: 50 mM MPPD and 100 mM vinyl acetate was catalyzed in 20 mL solvent containing 20 mg of CLEAs-YCJ01, the mixture was incubated at 30 °C and rotated at 180 rpm, and the reaction time was 12 h. |
Lipase YCJ01 |
Diisopropyl ether |
1.9 |
67.2 ± 1.9 |
99.1 ± 0.1 |
40.4 ± 1.2 |
406 ± 23 |
t-Butanol |
0.6 |
51.1 ± 2.3 |
94.6 ± 0.4 |
33.4 ± 1.5 |
58 ± 3 |
THF |
0.49 |
32.3 ± 2.2 |
73.7 ± 0.6 |
25.9 ± 1.8 |
9 ± 2 |
Acetone |
−0.23 |
41.6 ± 2.2 |
82.7 ± 0.5 |
30.1 ± 1.6 |
15 ± 2 |
CLEAs-YCJ01 |
Diisopropyl ether |
1.9 |
81.8 ± 0.2 |
99.7 ± 0.1 |
49.1 ± 0.1 |
1190 ± 35 |
t-Butanol |
0.6 |
74.2 ± 1.3 |
97.1 ± 0.4 |
44.6 ± 0.8 |
105 ± 5 |
THF |
0.49 |
67.8 ± 1.9 |
85.9 ± 0.5 |
41.6 ± 1.4 |
24 ± 3 |
Acetone |
−0.23 |
71.5 ± 2.0 |
89.4 ± 0.6 |
43.2 ± 1.2 |
37 ± 3 |
The values of ee for the S-diacetates were determined and also presented in Table 3. The solvents markedly affected the enantioselectivity of CLEAs-YCJ01. Fortunately, in isopropyl ether, CLEAs-YCJ01 not only showed the highest activity, but also the highest enantioselectivity towards MPPD. When the hydrophobic solvents hexane and toluene were used as solvents, the enantioselectivity was 126 and 89, respectively. When diisopropyl ether was used as the solvent, the enantioselectivity rapidly increased to 1190. However, upon changing the solvents to the hydrophilic solvents t-butanol, THF and acetone, the enantioselectivity decreased to 105, 24 and 37, respectively. Clearly, isopropyl ether proved to be the most suitable solvent for the resolution of MPPD through transesterification. Isopropyl ether also favored the Penicillium roqueforti lipase24 and porcine pancreatic lipase,25 which exhibited the best catalytic performances for the resolution of secondary alcohols through transesterification. However, the reason for these lipases showing their best catalytic performances in isopropyl ether is unclear. The enantioselectivity of lipases may change, sometimes dramatically, as a function of the nature of the solvents and for other reasons.26
The effect of temperature on the resolution of MPPD. The effect of temperature on the enzymatic reaction was investigated and the results are shown in Fig. 1. The catalytic activity increased from 42.6 to 68.2 μmol (min g)−1 in the range of 30–50 °C. However, when the temperature exceeded 35 °C, the ee values of S-diacetates decreased to below 99%. High temperature conditions change the structure of the catalytic center of lipases,28 which explains the reduction in the enantioselectivity of CLEAs-YCJ01 towards MPPD at high temperatures. Thus, the optimum reaction temperature was determined to be 35 °C.
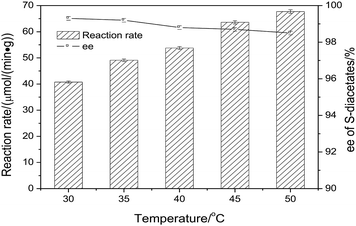 |
| Fig. 1 Effect of temperature on the resolution of MPPD. Reaction conditions: the resolution of MPPD was performed in 20 mL isopropyl ether containing 50 mM MPPD, 100 mM vinyl acetate and 20 mg CLEAs-YCJ01. The mixture was rotated at 180 rpm and the reaction time was 6 h. | |
The effect of the water content of the solvent on the resolution of MPPD. As shown in Fig. 2, CLEAs-YCJ01 showed high catalytic activity when the water content was between 0.56 and 0.75, and the conversion reached more than 49% at 3 h. However, the conversion at 3 h was less than 42% at the low water contents of 0.11, 0.24, and 0.33. Additionally, the ee values of the S-diacetates decreased slightly to 98.2% at a water content of 0.75. The above results indicated that a high water content improved the flexibility and catalytic activity of CLEAs-YCJ01 while decreasing its enantioselectivity,29 leading to a decrease in the ee values of S-diacetates. Therefore, the appropriate water content is 0.56.
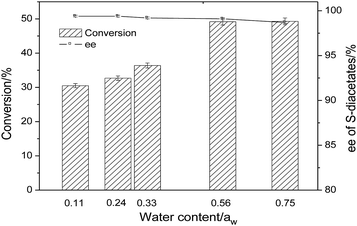 |
| Fig. 2 Effect of water content on the resolution of MPPD. Reaction conditions: the resolution of MPPD was performed in 20 mL isopropyl ether containing 50 mM MPPD, 150 mM vinyl acetate and 20 mg CLEAs-YCJ01. The mixture was incubated at 35 °C, rotated at 180 rpm and the reaction time was 3 h. | |
The effect of substrate concentration on the resolution of MPPD. The effect of substrate concentration on the resolution of MPPD is shown in Fig. 3. The reaction rate increased with an increase in the substrate concentration. However, when the substrate concentration exceeded 180 mmol L−1, the rate decreased sharply from 246.5 to 182.7 μmol (min g)−1. There may be two reasons for the decline in the reaction rate. First, when the substrate concentration reached more than 180 mmol L−1, MPPD was not completely dissolved in the solvent. Second, a high concentration of formed monoacetate may increase the viscosity of the reaction system, leading to a decrease in the reaction rate. Meanwhile, the change in concentration had little effect on the enantioselectivity of the enzyme, and the ee value of S-diacetates was 99.2% at 180 mmol L−1.
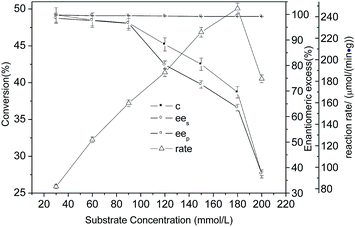 |
| Fig. 3 Effect of substrate concentration on the resolution of MPPD. Reaction conditions: the resolution of MPPD was performed in 20 mL isopropyl ether containing 20 mg CLEAs-YCJ01 and the molar ratio of MPPD to vinyl acetate was 3 : 1. The mixture was incubated at 35 °C, rotated at 180 rpm with a water content 0.56, and the reaction time was 6 h. | |
MPPD enzymatic reaction process curve. According to the results for the resolution of MPPD with CLEAs-YCJ01, the optimum conditions for enzyme catalysis included the use of isopropyl ether as the solvent, MPPD concentration of 180 mmol L−1, molar ratio of vinyl acetate to substrate of 3
:
1, reaction temperature of 35 °C and water content of 0.56. Under these optimum conditions, the resolution process curve of MPPD with CLEAs-YCJ01 was obtained, as shown in Fig. 4. At the beginning of the reaction, S-monoacetate was rapidly transformed into S-diacetate. As the reaction progressed further, the substrate concentration gradually decreased, which led to a slow increase in conversion. At the reaction time of 12 h, the yield of S-diacetates was 49.9% and the ee values for monoacetate and S-diacetates were 99.1% and 99.2%, respectively. Thus, excellent ee values were simultaneously obtained for both the R- and S-type compounds, and the resolution of MPPD by CLEAs-YCJ01 gave 49.9% yield for S-diacetates and 49.3 yield for R-monoacetate.
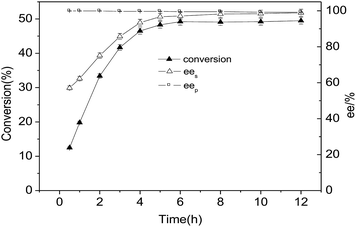 |
| Fig. 4 Time course of resolution of MPPD by CLEAs-YCJ01. Reaction conditions: the resolution of MPPD was performed in 20 mL isopropyl ether containing 180 mM MPPD, 540 mM vinyl acetate and 20 mg CLEAs lipase YCJ01. The mixture was incubated at 35 °C and rotated at 180 rpm with a water content of 0.56. | |
Operational stability of CLEAs-YCJ01. The operational stabilities of CLEAs-YCJ01 and lipase YCJ01 for the resolution of MPPD are shown in Fig. 5. When the reaction was catalyzed by CLEAs-YCJ01, the highest conversion and enantioselectivity were achieved in the first batch. Thereafter, the activity of lipase YCJ01 decreased. The residual activity of CLEAs-YCJ01 after ten batches remained at 91.2% of the initial activity, and the ee values of S-diacetates remained above 99.0% after every batch. These results showed that CLEAs-YCJ01 has good operational stability and the reaction time does not change the enantioselectivity of CLEAs-YCJ01, indicating that the two configurations of substrate with high ee values could be achieved by prolonging the reaction time or adding catalyst at every batch reaction.
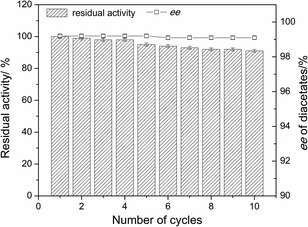 |
| Fig. 5 Operational stabilities of the lipase YCJ01 and CLEAs-YCJ01. Reaction conditions: the resolution of MPPD was performed in 20 mL isopropyl ether containing 180 mM MPPD, 540 mM vinyl acetate and 20 mg CLEAs-YCJ01. The mixture was incubated at 35 °C and rotated at 180 rpm with a water content 0.56. Residual activity = (the conversion of MPPD/the conversion of MPPD for the first cycle) × 100%. Operational conditions: the first cycle ended with the conversion of 49.2% for MPPD at 12 h, and the other cycles ended with the same time of the first cycle. | |
The methods for the preparation of enantiomerically pure MPPD include asymmetric catalysis and enzyme catalysis. Zhu et al.7 applied a chiral nanoporous metal–organic catalyst for the preparation of S-MPPD with a substrate concentration of 80 mmol L−1 and the reaction reached 57% conversion and 87% ee after 24 h. Bendigiri et al.9 reported a recombinant epoxide hydrolase from Yarrowia lipolytica for the preparation of S-MPPD and the ee was 95%. Zhao et al.30 applied an epoxide hydrolase for the preparation of R-MPPD and the ee was 73%. Theil et al.15 reported a transformation in the presence of Amano lipase PS from B. cepacia, in which the yield of S-diacetates reached 42% and the ee for S-diacetates was 93%. In the above studies, the concentration of substrate was in the range of 10–80 mmol L−1. In this work, a high enantioselectivity of S-diacetates and substrate concentration were obtained using CLEAs-YCJ01 as a catalyst. The ee values for monoacetate and S-diacetates were 99.1% and 99.2%, respectively, and the substrate concentration reached 180 mmol L−1, which shows potential prospects for industrial applications.
Resolution of 3-aryloxy-1,2-propanediols by CLEAs-YCJ01
Since CLEAs-YCJ01 showed excellent enantioselectivity towards MPPD, it was evaluated for the resolution of a series of 3-aryloxy-1,2-propanediols (six analogues of MPPD). The reactions are shown in Scheme 1 and the results are shown in Table 5. Every S-monoacetate of 3-aryloxy-1,2-propanediols preferentially transformed into S-diacetates with a high conversion (≥49.3%), favorable ee values (94.8–99.4%) for S-diacetates and high ee values (92.1–99.2%) for R-monoacetate. This means that CLEAs-YCJ01 has relatively strict enantioselectivity towards all the tested 3-aryloxy-1,2-propanediols. Especially, CLEAs-YCJ01 had strict enantioselectivity towards 3-(4-methylphenoxy)-1,2-propanediol (substrate 2, Table 5), and the ee values for monoacetate and S-diacetates were 99.1% and 99.2%, respectively. Also, 99.2% ee of monoacetate and 99.4% ee of S-diacetates were obtained for 3-phenoxy-1,2-propanediol (substrate 1, Table 5) by CLEAs-YCJ01.
Table 5 Resolution of racemic 3-aryloxy-1,2-propanediols in non-aqueous solventsa
No. |
Substrate |
Catalyst |
ee |
c (%) |
E |
Monoacetate |
Diacetates |
Reaction conditions: 180 mM 3-phenoxy-1,2-propanediols and 540 mM vinyl acetate were catalyzed in 20 mL isopropyl ether containing 20 mg of CLEAs-YCJ01 (or 43 mg of lipase YCJ01 powder with the same activity of CLEAs-YCJ01), the mixture was incubated at 35 °C and rotated at 180 rpm with a water content 0.56. The reaction was monitored by HPLC. |
1 |
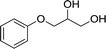 |
Lipase YCJ01 |
78.5 ± 1.0 |
99.1 ± 0.1 |
44.2 ± 0.3 |
529 ± 12 |
CLEAs-YCJ01 |
99.2 ± 0.1 |
99.4 ± 0.1 |
49.9 ± 0.1 |
1836 ± 132 |
2 |
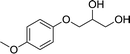 |
Lipase YCJ01 |
83.4 ± 1.0 |
99.1 ± 0.1 |
45.7 ± 0.3 |
601 ± 18 |
CLEAs-YCJ01 |
99.1 ± 0.1 |
99.2 ± 0.1 |
49.9 ± 0.1 |
1347 ± 78 |
3 |
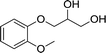 |
Lipase YCJ01 |
57.8 ± 1.0 |
96.2 ± 0.6 |
37.5 ± 0.3 |
94 ± 18 |
CLEAs-YCJ01 |
95.2 ± 0.1 |
96.0 ± 0.2 |
49.8 ± 0.1 |
184 ± 21 |
4 |
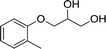 |
Lipase YCJ01 |
44.8 ± 1.6 |
94.4 ± 0.7 |
32.2 ± 0.6 |
54 ± 9 |
CLEAs-YCJ01 |
92.1 ± 0.2 |
94.8 ± 0.2 |
49.3 ± 0.1 |
124 ± 11 |
5 |
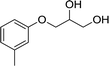 |
Lipase YCJ01 |
60.4 ± 1.4 |
95.4 ± 0.5 |
38.8 ± 0.4 |
78 ± 12 |
CLEAs-YCJ01 |
95.2 ± 0.2 |
95.3 ± 0.2 |
49.9 ± 0.1 |
156 ± 11 |
6 |
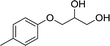 |
Lipase YCJ01 |
74.5 ± 1.8 |
96.7 ± 0.1 |
43.5 ± 0.7 |
137 ± 16 |
CLEAs-YCJ01 |
95.6 ± 0.2 |
96.5 ± 0.2 |
49.8 ± 0.1 |
219 ± 17 |
The six types of 3-aryloxy-1,2-propanediols have the common structure of 3-phenoxy-1,2-propanediols with different substituents on their benzene ring. As shown in Table 5, substrate 4 has an ortho benzene methyl moiety and substrate 6 has a para benzene methyl moiety. CLEAs-YCJ01 showed different enantioselectivities to these two substrates. Overall, the substrates having a para substituent on the benzene ring did not greatly affect the enantioselectivity, while that having an ortho substituent showed a large enantioselective effect. It may be possible that the ortho substitution of the substrate near the catalytic active center slightly affects the substrate orientation in the active center, thus reducing the enantioselectivity of the enzyme towards ortho-substituted compound–lipase complexes.15
Theil's group14,15 used Amano lipase PS to resolve 3-aryloxy-1,2-propanediols. The ee values of R-type compounds ranged from 55% to 99.8%, while that of S-type compounds ranged from 79% to 96.8%. In summary, CLEAs-YCJ01 showed good enantioselectivity towards 3-aryloxy-1,2-propanediols with high ee values for both R- and S-type compounds, indicating that CLEAs-YCJ01 has relatively strict substrate selectivity towards all the used analogues of MPPD.
Experimental
Materials
The lipase YCJ01 from B. ambifaria was characterized in our previous report, and its amino acid sequence was assigned GenBank accession no. JQ733583. B. ambifaria YCJ01 was deposited in CCTCC (Wuhan, China) with the accession number CCTCC M 2011058.18 3-Aryloxy-1,2-propanediols were purchased from Heowns Biochemical Technology LLC (Tianjin, China). p-Nitrophenyl palmitate (pNPP) was purchased from Sigma-Aldrich (St. Louis, MO, USA). Vinyl acetate, isopropyl ether and all other chemicals were purchased from Sinopharm Chemical Reagent Co., Ltd. (Shanghai, China). All reagents were used directly without any further treatment.
Enzyme activity assay
The hydrolytic activity of the free lipase was assayed according to reported literature procedures and pNPP was used as the substrate.31 CLEAs-YCJ01 was suspended in phosphate buffer (pH = 7.5, 50 mM) and samples of this suspension were used to calculate its activity according to the procedure reported for the free enzyme.
Transesterification reaction
The enzymatic transesterification reactions were performed in 50 mL conical flasks with stoppers. The appropriate amounts of solvent, 3-aryloxy-1,2-propanediols, vinyl acetate, and enzyme were introduced into the conical flasks and incubated at a constant temperature and shaking at 180 rpm. Aliquots of samples (30 μL) were withdrawn from the reaction mixture at intervals and the samples were analyzed via high-performance liquid chromatography (HPLC) according the following method.
Analytical methods
The reaction mixture was sampled at intervals and the solvent in each sample was volatilized and the remains were redissolved in a mobile phase composed of n-hexane/isopropanol (85/15, v/v) (200 μL) then filtered using a 0.45 μm membrane filter. After filtration, the treated samples were analyzed via HPLC using a Chiralcel® OD-H column (Daicel, 4.6 × 250 mm) and detected at the ultraviolet wavelength of 270 nm. The mobile phase flow rate was 1 mL min−1 and 20 μL of each sample was injected at 30 °C. The retention times of all the substrates are presented in the ESI.†
The ee values of R-monoacetate (ees) and S-diacetates (eep) were calculated by HPLC. The yield of S-diacetates was determined by HPLC. The conversion of 3-aryloxy-1,2-propanediols (c) was calculated as c (%) = ees/(ees + eep) × 100 and the enantioselectivity (E) was calculated as E = ln[(1 − c)(1 − ees)/(1 − c)(1 + ees)].
Water content
The substrates and solvents were separately pre-equilibrated at different water contents (aw) prior to the resolution. All samples were placed in a closed vessel above saturated salts, and equilibrium was reached for 48 h at room temperature. The salts used in this test included LiCl, aw = 0.11; CH3COOK, aw = 0.24; MgCl2, aw = 0.33; Mg(NO3)2, aw = 0.56, and NaCl, aw = 0.75.
Preparation of CLEAs of lipase YCJ01 (CLEAs-YCJ01)
The preparation of CLEAs-YCJ01 in this work was done according to the procedures described by Schoevaart20 with some modifications. Crude lipase YCJ01 powder was prepared in our previous work18 and dissolved in phosphate buffer (pH = 7.5 and 0.05 M) to a concentration of 1.2 mg protein per mL (53 U). Aggregates of lipase YCJ01 were prepared using precipitants and glutaraldehyde was added to a total concentration of 0.25% (v/v), then the resulting suspensions were stirred at 0 °C for 4 h. The CLEAs of lipase YCJ01 was separated by centrifugation and obtained by volatilizing the residual solvent. All portions of the filtrate were retained for the determination of protein concentration and activity.
Bioconversion and determination of operational stability
In a typical reaction, 20 mL isopropyl ether, CLEAs-YCJ01 (20 mg), MPPD (50 mM), and vinyl acetate (100 mM) were loaded into 50 mL conical flasks with stoppers. The mixture was kept at 35 °C and rotated at 180 rpm. The samples were withdrawn from the mixture and analyzed by HPLC, as described above. When the determination of operational stability was performed, the filtered CLEAs-YCJ01 was washed with fresh isopropyl ether (3 × 5 mL) and loaded back into the conical flasks for the next cycle.
Conclusions
In this work, cross-linked enzyme aggregates of lipase YCJ01 (CLEAs-YCJ01) were prepared with t-butanol as the precipitant, and 214.8% of activity recovery was achieved. CLEAs-YCJ01 showed excellent enantioselectivity towards 3-(4-methylphenoxy)-1,2-propanediol with ee values of 99.2% for S-diacetates and 99.1% for monoacetate. For the first time, R- and S-type compounds with excellent ee values were simultaneously obtained. CLEAs-YCJ01 also showed high operational stability and maintained 91.2% residual activity after ten batches. These results showed that CLEAs-YCJ01 has good operational stability and the reaction time does not change the enantioselectivity of CLEAs-YCJ01, indicating that the two configurations of substrate with high ee values could be achieved by prolonging the reaction time or adding catalyst at every batch reaction.
Further, CLEAs-YCJ01 was evaluated for the resolution of a series of 3-aryloxy-1,2-propanediols (six analogues of MPPD) under the optimized reaction conditions for the resolution of MPPD. The results showed that CLEAs-YCJ01 has relatively strict enantioselectivity towards 3-aryloxy-1,2-propanediols with a high yield (≥49.3%), favorable ee values (94.8–99.4%) for S-diacetates and high ee values (92.1–99.2%) for R-monoacetate. Especially, towards 3-(4-methylphenoxy)-1,2-propanediol and 3-phenoxy-1,2-propanediol, both of R- and S-type compounds with >99% ee were obtained.
Conflicts of interest
There are no conflicts to declare.
Acknowledgements
This work was supported by the National High Technology Research and Development Key Program of China (2012AA022205).
Notes and references
- H.-S. Byun, J. A. Sadlofsky and R. Bittman, J. Org. Chem., 1998, 63, 2560–2563 CrossRef CAS PubMed.
- S. Maccari, M. Buoncervello, A. Rampin, M. Spada, D. Macchia, L. Giordani, T. Stati, C. Bearzi, L. Catalano, R. Rizzi, L. Gabriele and G. Marano, Br. J. Pharmacol., 2017, 174, 139–149 CrossRef CAS PubMed.
- B. Liu, X. Qian, Q. Wu and X. Lin, Enzyme Microb. Technol., 2008, 43, 375–380 CrossRef CAS.
- B. L. Linhares, N. R. F. Nascimento, L. F. Gonzaga-Silva, C. F. Santos, M. O. Moraes, L. B. Marinho, A. P. G. Silva, M. C. Fonteles and R. Reges, Eur. J. Pharmacol., 2018, 833, 425–431 CrossRef CAS PubMed.
- J. Agustian, A. H. Kamaruddin and S. Bhatia, Process Biochem., 2010, 45, 1587–1604 CrossRef CAS.
- A. Y. Ridwan, R. Matoba, J. Wu, J.-H. Choi, H. Hirai and H. Kawagishi, Tetrahedron Lett., 2018, 59, 2559–2561 CrossRef CAS.
- C. Zhu, G. Yuan, X. Chen, Z. Yang and Y. Cui, J. Am. Chem. Soc., 2012, 134, 8058–8061 CrossRef CAS PubMed.
- M. T. Reetz, M. Bocola, L.-W. Wang, J. Sanchis, A. Cronin, M. Arand, J. Zou, A. Archelas, A.-L. Bottalla, A. Naworyta and S. L. Mowbray, J. Am. Chem. Soc., 2009, 131, 7334–7343 CrossRef CAS PubMed.
- C. Bendigiri, K. Harini, S. Yenkar, S. Zinjarde, R. Sowdhamini and A. RaviKumar, RSC Adv., 2018, 8, 12918–12926 RSC.
- Z. Sun, L. Wu, M. Bocola, H. C. S. Chan, R. Lonsdale, X.-D. Kong, S. Yuan, J. Zhou and M. T. Reetz, J. Am. Chem. Soc., 2018, 140, 310–318 CrossRef CAS PubMed.
- Å. Janfalk Carlsson, P. Bauer, H. Ma and M. Widersten, Biochemistry, 2016, 55, 1940 CrossRef PubMed.
- Z. S. Seddigi, M. S. Malik, S. A. Ahmed, A. O. Babalghith and A. Kamal, Coord. Chem. Rev., 2017, 348, 54–70 CrossRef CAS.
- X. Tian, G.-W. Zheng, C.-X. Li, Z.-L. Wang and J.-H. Xu, J. Mol. Catal. B: Enzym., 2011, 73, 80–84 CrossRef CAS.
- F. Theil, J. Weidner, S. Ballschuh, A. Kunath and H. Schick, Tetrahedron Lett., 1993, 34, 305–306 CrossRef CAS.
- F. Theil, J. Weidner, S. Ballschuh, A. Kunath and H. Schick, J. Org. Chem., 1994, 59, 388–393 CrossRef CAS.
- S. Cao, P. Xu, Y. Ma, X. Yao, Y. Yao, M. Zong, X. Li and W. Lou, Chin. J. Catal., 2016, 37, 1814–1823 CrossRef CAS.
- S. Talekar, A. Joshi, G. Joshi, P. Kamat, R. Haripurkar and S. Kambale, RSC Adv., 2013, 3, 12485–12511 RSC.
- C. Yao, Y. Cao, S. Wu, S. Li and B. He, J. Mol. Catal. B: Enzym., 2013, 85–86, 105–110 CrossRef CAS.
- J. Skjold-Jørgensen, J. Vind, A. Svendsen and M. J. Bjerrum, Biochemistry, 2014, 53, 4152–4160 CrossRef PubMed.
- R. Schoevaart, M. W. Wolbers, M. Golubovic, M. Ottens, A. P. Kieboom, F. van Rantwijk, L. A. van der Wielen and R. A. Sheldon, Biotechnol. Bioeng., 2004, 87, 754–762 CrossRef CAS PubMed.
- M. Agarwal, P. K. Dikshit, J. B. Bhasarkar, A. J. Borah and V. S. Moholkar, Chem. Eng. J., 2016, 295, 254–267 CrossRef CAS.
- J. Abildskov, M. B. van Leeuwen, C. G. Boeriu and L. A. M. van den Broek, J. Mol. Catal. B: Enzym., 2013, 85–86, 200–213 CrossRef CAS.
- M. D. Alves, F. M. Aracri, É. C. Cren and A. A. Mendes, Chem. Eng. J., 2017, 311, 1–12 CrossRef CAS.
- T. Miyazawa, S. Kurita, S. Ueji and T. Yamada, Biocatal. Biotransform., 2009, 17, 459–473 CrossRef.
- V. Athawale, N. Manjrekar and M. Athawale, Biotechnol. Prog., 2003, 19, 298–302 CrossRef CAS PubMed.
- M. Z. Kamal, P. Yedavalli, M. V. Deshmukh and N. M. Rao, Protein Sci., 2013, 22, 904–915 CrossRef CAS PubMed.
- B. Wang, C. Zhang, Q. He, H. Qin, G. Liang and W. Liu, Mol. Catal., 2018, 448, 116–121 CrossRef CAS.
- H. Cao, L. Deng, M. Lei, F. Wang and T. Tan, J. Mol. Catal. B: Enzym., 2014, 109, 101–108 CrossRef CAS.
- R. Sato, A. Sato and H. Tokuyama, J. Appl. Polym. Sci., 2015, 132, 1–5 CrossRef.
- J. Zhao, Y.-Y. Chu, A.-T. Li, X. Ju, X. Kong, J. Pan, Y. Tang and J.-H. Xu, Adv. Synth. Catal., 2011, 353, 1510–1518 CrossRef CAS.
- B. Wang, W. Li, B. Wu and B. He, J. Mol. Catal. B: Enzym., 2014, 106, 105–110 CrossRef CAS.
Footnote |
† Electronic supplementary information (ESI) available. See DOI: 10.1039/c9ra01103j |
|
This journal is © The Royal Society of Chemistry 2019 |
Click here to see how this site uses Cookies. View our privacy policy here.