DOI:
10.1039/C8QO00726H
(Research Article)
Org. Chem. Front., 2019,
6, 116-120
Graphene oxide mediated thiolation of indoles in water: a green and sustainable approach to synthesize 3-sulfenylindoles†
Received
17th July 2018
, Accepted 26th November 2018
First published on 26th November 2018
Abstract
An atom economical and transition-metal and iodine free procedure of graphene oxide (a heterogeneous, recyclable, inexpensive, and environment-friendly carbocatalyst) mediated thiolation of indoles, using thiols as a thiolating reagent, has been developed. This methodology provides a catalyst-recyclable and organic-solvent-free protocol to afford 3-sulfenylindoles with good functional group tolerance and broad substrate scope.
Introduction
Indoles and their derivatives are ubiquitously found in a diverse array of biologically active molecules. One of them is 3-sulfenylindoles, which exhibit excellent pharmacological properties, including anti-HIV,1 anti-nociceptive,2 and anti-allergic activities,3 and acting as 5-lipoxygenase and tubulin polymerization inhibitors.4,5 As a result, there have long been extensive efforts toward the development of efficient reactions to synthesize this framework. Among the reported methods, nonetheless, most of them require transition-metals,6 iodine,7 bases,8 acids9 or complex ligands10 and are costly or require harsh reaction conditions. Most importantly, in these coupling reactions employing thiolating agents, including N-(thiophenyl)succinimide,11 benzenesulfonyl chlorides,12 disulfides,13S-phenyl benzenesulfonothioate14 and benzene-sulfonhydrazide,15 generation of stoichiometric byproducts still cannot be avoided under the conditions used (Scheme 1a).
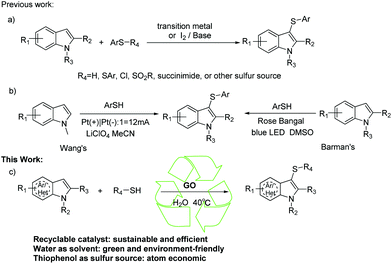 |
| Scheme 1 Thiolation of indoles with thiolating agents for the synthesis of 3-sulfenylindoles. | |
With the increasing awareness to protect our fragile environment and advances in green and sustainable chemistry in recent years (Scheme 1a), photochemical reactions and electrocatalytic protocols have been reported to obtain 3-sulfenylindoles. In 2017, Wang and his coworkers16 developed an electrocatalytic oxidant-free method to obtain 3-sulfenylindoles. Shortly thereafter, Barman's team17 reported visible-light-induced regioselective sulfenylation of imidazopyridines with thiols under transition metal-free conditions (Scheme 1b). No doubt these reported procedures have some merits, yet they suffered from some drawbacks, such as the usage of volatile toxic organic solvents and the requirement of special equipment. Thus, the development of environmentally friendly and atom-economical methods for sulfenylindole formation under mild reaction conditions is still highly desirable.
In recent years, macromolecular materials have attracted great attention in organic synthesis, due to their outstanding merits of simple preparation recyclability and low toxicity. Our group has been working on the application of macromolecular materials, including hexagonal boron nitride(h-BN), hydroxide-functionalized h-BN (h-BN@OH), cyclodextrin (CD), γ-cyclodextrin (γ-CD) and graphene oxide (GO),18 in organic synthesis for a long time. Enlightened by this, we were eager to find macromolecular materials that could mediate the synthesis of 3-sulfenylindoles. Finally, we have found graphene oxide (GO), which is a two-dimensional material with a hydrophobic carbon lattice, and when functionalized with a wide range of functional groups, such as epoxy (C–O–C), hydroxyl (OH), and carboxyl (COOH) groups on the edges and the basal plane,19 it can mediate the reaction of an indole and thiophenol to yield 3-sulfenylindoles. Meanwhile its excellent stabilities, high specific surface areas20 and amphiphilicity provide the possibility to conduct the reaction in water.21 In this work, we describe how highly efficient and sustainable GO-mediated thiolation of indoles with thiols enables the synthesis of 3-sulfenylindoles in water (Scheme 1c).
Results and discussion
The initial experiment was carried out with 1a and 2a in the presence of h-BN (30 mg) at 30 °C in H2O (5 mL) for 24 h (Table 1, entry 1). Unfortunately, the desired target product 3aa was not obtained under the initial conditions. Gratifyingly, h-BN@COOH and GO afforded the product 3aa in 35% and 55% yields, respectively, when other functionalized macromolecular materials h-BN@OH, h-BN@COOH and GO were investigated (Table 1, entries 2–4). Encouraged by this result, we further optimized the reaction conditions with GO (Table 1). First, different solvents were investigated (Table 1, entries 5–8). No products were observed for the tested solvents, except for DCM, which provided a moderate yield of 51% (Table 1, entry 8). Thus, H2O was selected as the best solvent because of its efficiency and green nature. Then variations of the temperature revealed that 40 °C was the most effective temperature with the yield increasing to 65% (Table 1, entries 4, 9, and 10). After that, we focused our attention on the amount of GO. The results showed that 100 mg GO could give an isolated yield of 81% (Table 1, entries 9 and 11–13). In addition, we found that 24 h was the essential time for this reaction (Table 1, entries 13–15). The amount of GO could be reduced to 30 mg, but the higher reaction temperature was necessary (Table 1, entries 16 and 17). Considering the reusablility of GO and to avoid harsh reaction conditions, we chose 100 mg GO as the optimal amount which gave an excellent isolated yield at 40 °C. Then, the control experiment confirmed that GO was essential for this reaction (Table 1, entry 18). Finally, we found that the isolated yield of 3aa did not change too much when the reactions were conducted under O2/Ar/air (Table 1, entries 13, 19 and 20).
Table 1 Reaction optimizationa
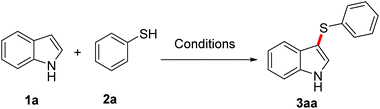
|
Entry |
Catalyst |
Amount (mg) |
Solvent |
T (°C) |
Time |
Yieldb |
Reaction conditions: 1a (0.26 mmol), 2a (0.39 mmol), GO (100 mg), solvent (5 mL), under air.
Isolated yield.
Under O2.
Under Ar.
|
1 |
h-BN |
30 |
H2O |
30 |
24 h |
N.R |
2 |
h-BN@OH |
30 |
H2O |
30 |
24 h |
N.R |
3 |
h-BN@-COOH |
30 |
H2O |
30 |
24 h |
35% |
4 |
GO |
30 |
H2O |
30 |
24 h |
55% |
5 |
GO |
30 |
DMF |
30 |
24 h |
N.R |
6 |
GO |
30 |
DMSO |
30 |
24 h |
N.R |
7 |
GO |
30 |
IPA |
30 |
24 h |
N.R |
8 |
GO |
30 |
DCM |
30 |
24 h |
51% |
9 |
GO |
30 |
H2O |
40 |
24 h |
65% |
10 |
GO |
30 |
H2O |
60 |
24 h |
66% |
11 |
GO |
50 |
H2O |
40 |
24 h |
63% |
12 |
GO |
80 |
H2O |
40 |
24 h |
67% |
13 |
GO |
100 |
H2O |
40 |
24 h |
81% |
14 |
GO |
100 |
H2O |
40 |
18 h |
63% |
15 |
GO |
100 |
H2O |
40 |
12 h |
50% |
16 |
GO |
30 |
H2O |
100 |
24 h |
71% |
17 |
GO |
30 |
H2O |
120 |
24 h |
75% |
18 |
— |
0 |
H2O |
40 |
24 h |
N.R |
19 |
GO |
100 |
H2O |
40 |
24 h |
79%c |
20 |
GO |
100 |
H2O |
40 |
24 h |
80%d |
With the optimal conditions in hand, we then investigated the substrate scope of indoles in this transformation with thiols (Scheme 2). First, indoles with various substituents were tested with 2a. Gratifyingly, indoles with various electron-withdrawing (NO2, CN, Cl, and Br) and electron-donating groups (Me and OMe) at the different positions of the indole ring were fully tolerated giving the desired products in good to excellent yields (Scheme 2, 3aa, 3ba, and 3da–3la), whereas 4-(benzyloxy)-1H-indole 1c resulted in slightly lower respective yields of 60% (Scheme 2, 3ca). We then investigated the coupling efficiency of N-methyl-indoles (1m–1o) and 2-phenyl-1H-imidazo-[1,2-a] pyridine (1p). These substrates also showed high reactivity in this transformation (Scheme 2, 3ma–3pa). Furthermore, thiols with various substituents were tested with 1a. All reactions proceeded smoothly when the thiophenol was bearing regardless of electron-donating groups (Me, t-Bu, OMe) or electron-withdrawing groups (F, Cl, Br, and CN) on the phenyl ring, the 3-sulfenylindoles were obtained in good to excellent yields (Scheme 2, 3ab–3ai). In addition, phenylmethanethiol and 1H-imidazole-2-thiol were tested and the corresponding products 3aj and 3ak were obtained in moderate yields (Scheme 2, 3aj and 3ak). Finally we were pleased to observe that this reaction could be conducted on a gram scale (1a: 8.5 mmol and 2a: 12.8 mmol) and afforded 3aa in 70% yield. This showed the great potential of this reaction in industrial syntheses. The results from this section demonstrated that this approach was broadly applicable for the synthesis of 3-sulfenylindoles.
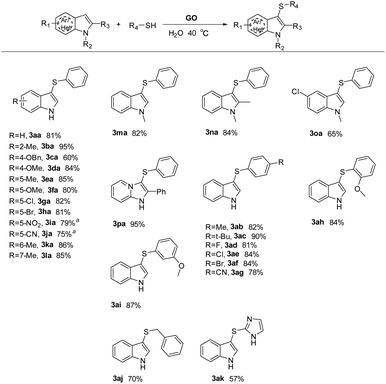 |
| Scheme 2 Substrate scope of indoles and thiols. General reaction conditions: indoles (0.26 mmol), thiophenols (0.39 mmol) and GO (100 mg) in 5 mL H2O, 40 °C, under air, 24 h; all yields refer to isolated products based on indoles; aGO (100 mg) in 5 mL H2O, 100 °C, under air, 24 h. | |
Excellent stability and recyclability are important features of GO.22 To clarify this issue, the feasibility of recycling GO was examined. Upon the completion of the reaction, GO was simply separated by filtration and washed with ethyl acetate, until no residual products were obtained. The catalyst was further subjected to vacuum to remove the rest of the solvent before it was reused in subsequent reactions. Even if it was reused ten times, there was no notable loss of its catalytic activity (Fig. 1).
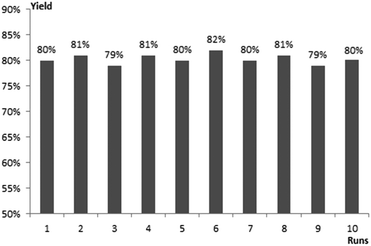 |
| Fig. 1 Reuse of GO in the synthesis of 3aa. | |
We further performed several selective and control experiments to gain insight into the reaction mechanism. The reaction did not proceed when GO was replaced by graphene (Scheme 3a). This indicated that the functional groups on GO might be involved in this transformation. Then, in order to neutralize carboxyl groups of GO, we added trimethylamine into a new reaction mixture to adjust the pH to 8
23 and found that the reaction did not proceed at all. This suggested that the carboxyl (COOH) group might play a major role in this reaction (Scheme 3b). Furthermore, graphene oxide was replaced by reduced graphene oxide under standard conductions and no product was found.
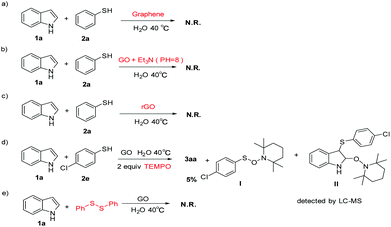 |
| Scheme 3 Mechanistic investigations of the reaction. | |
This indicated that the oxygenated groups on GO were essential for our reactions (Scheme 3c). In addition, the reaction was performed with 2,2,6,6-tetramethylpiperidin-1-oxyl (TEMPO) as a radical scavenger while 3ae was obtained in 5% yield only under the standard conditions (Scheme 3d). Meanwhile, we detected 2,2,6,6-tetramethyl-1-((4-chlorobenzenethiol)oxy)piperidine(I) and 3-(4-chlorobenzenethiol)-2-((2,2,6,6-tetramethyl piperidin-1-yl)oxy) indoline(II) by LC-MS which indicated that the reaction probably proceeded through a radical pathway. Finally, 1,2-diphenyldisulfane was used to react with the indole (1a) under standard conditions, and the product 3aa was not detected, which is probably because the hemolysis of disulfide cannot occur under these conditions17 (Scheme 3e).
On the basis of previous studies17,24 and our control experiments, we proposed a plausible reaction pathway for this reaction as shown in Fig. 2. Graphene oxide might act as a radical initiator;25 first, promoted by the functional groups on the surface of GO, benzenethiol transformed into phenylthiophenol radical A. Next, the thioyl radical A interacted with 1a to produce the radical intermediate B. After that, B was oxidized to the intermediate C. Finally, deprotonation of intermediate C led to the formation of 3-sulfenylindoles. GO probably plays a crucial role during the process of oxidation and deprotonation.
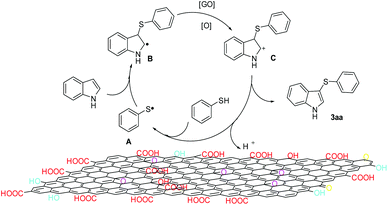 |
| Fig. 2 The proposed mechanism for the reaction. | |
Experimental
General procedure for synthesis of compound 3aa
A 15 mL sealed tube, equipped with a magnetic stirring bar and a rubber septum, was charged with indole 1a (30 mg, 0.26 mmol, 1.0 equiv.), thiophenol 2a (42.3 mg, 0.39 mmol, 1.5 equiv.), and graphene oxide (100 mg) and water was added into the vial. Then the resulting solution was stirred at 40 °C for 24 h. The reaction mixture was filtered and the residue was washed with ethyl acetate (3 × 15 mL) monitored by thin-layer chromatography (TLC) until all the organic residue was completely removed. The combined organic extracts were dried over anhydrous Na2SO4, and concentrated at reduced pressure. The crude product was purified by flash column chromatography on silica gel using a mixture of PE/EA (100
:
1) as the eluent to provide the product 3aa (white solid) in 81% yield.
Conclusion
In summary, we have developed a procedure of GO-mediated thiolation of indoles with thiols in water. This methodology provided an organic-solvent-free, transition metal and iodine free, catalyst-recyclable, mild condition, high atom economical, environment-friendly and sustainable protocol for the direct synthesis of 3-sulfenylindoles. In addition, this method shows a broad substrate scope and good scalability. We believe that this green and sustainable method has promising applications in the industrial syntheses of sulfenylindole-containing drugs and intermediates.
Conflicts of interest
There are no conflicts to declare.
Acknowledgements
This work was supported by the National Natural Science Foundation of China (Grant No: 81773577; 81573286; 81502989; and 81602954), and the Fundamental Research Funds for the Central Universities (Grant No: YJ201561).
Notes and references
-
(a) V. Famiglini, G. L. Regina, A. Coluccia, S. Pelliccia, A. Brancale, G. Maga, E. Crespan, R. Badia, E. Riveira-Muñoz, J. A. Esté, R. Ferretti, R. Cirilli, C. Zamperini, M. Botta, D. Schols, V. Limongelli, B. Agostino, E. Novellino and R. Silvestri, J. Med. Chem., 2014, 57, 9945 CrossRef CAS PubMed;
(b) G. L. Regina, A. Coluccia, A. Brancale, F. Piscitelli, V. Gatti, G. Maga, A. Samuele, C. Pannecouque, D. Schols, J. Balzarini, E. Novellino and R. Silvestri, J. Med. Chem., 2011, 54, 1587 CrossRef PubMed;
(c) R. Ragno, A. Coluccia, G. L. Regina, G. D. Martino, F. Piscitelli, A. Lavecchia, E. Novellino, A. Bergamini, C. Ciaprini, A. Sinistro, G. Maga, E. Crespan, M. Artico and R. Silvestri, J. Med. Chem., 2006, 49, 3172 CrossRef CAS PubMed;
(d) G. D. Martino, G. L. Regina, A. Coluccia, M. C. Edler, M. C. Barbera, A. Brancale, E. Wilcox, E. Hamel, M. Artico and R. Silvestri, J. Med. Chem., 2004, 47, 6120 CrossRef PubMed;
(e) R. Silvestri, G. D. Martino, G. L. Regina, M. Artico, S. Massa, L. Vargiu, M. Mura, A. G. Loi, T. Marceddu and P. L. Colla, J. Med. Chem., 2003, 46, 2482 CrossRef CAS.
- D. Potin, V. Parnet, J. M. Teulon, F. Camborde, F. Caussade, J. Meignen, D. Provost and A. Cloarec, Bioorg. Med. Chem. Lett., 2000, 10, 805 CrossRef CAS.
- P. C. Unangst, D. T. Connor, S. R. Stabler, R. J. Weikert, M. E. Carethers, J. A. Kennedy, D. O. Thueson, J. C. Chestnut, R. L. Adolphson and M. C. Conroy, J. Med. Chem., 1989, 32, 1360 CrossRef CAS.
- J. H. Hutchinson, D. Riendeau, C. Brideau, C. Chan, D. Delorme, D. Denis, J. P. Falgueyret, R. Fortin, J. Guay, P. Hamel, T. R. Jones, D. Macdonald, C. S. McFarlane, H. Piechuta, J. Scheigetz, P. Tagari, M. Thérien and Y. Girard, J. Med. Chem., 1993, 36, 2771 CrossRef CAS PubMed.
-
(a) G. L. Regina, T. Sarkar, R. Bai, M. C. Edler, R. Saletti, A. Coluccia, F. Piscitelli, L. Minelli, V. Gatti, C. Mazzoccoli, V. Palermo, C. Mazzoni, C. Falcone, A. I. Scovassi, V. Giansanti, P. Campiglia, A. Porta, B. Maresca, E. Hamel, A. Brancale, E. Novellino and R. Silvestri, J. Med. Chem., 2009, 52, 7512 CrossRef PubMed;
(b) G. D. Martino, M. C. Edler, G. L. Regina, A. Coluccia, M. C. Barbera, D. Barrow, R. I. Nicholson, G. Chiosis, A. Brancale, E. Hamel, M. Artico and R. Silvestri, J. Med. Chem., 2006, 49, 947 CrossRef PubMed;
(c) G. D. Martino, G. L. Regina, A. Coluccia, M. C. Edler, M. C. Barbera, A. Brancale, E. Wilcox, E. Hamel, M. Artico and R. Silvestri, J. Med. Chem., 2004, 47, 6120 CrossRef PubMed;
(d) Y. Maeda, M. Koyabu, T. Nishimura and S. Uemura, J. Org. Chem., 2004, 69, 7688 CrossRef CAS PubMed.
-
(a) S. Vasquez-Cespedes, A. Ferry, L. Candish and F. Glorius, Angew. Chem., Int. Ed., 2015, 54, 5772 CrossRef CAS PubMed;
(b) Z. Wu, Y. C. Li, W. Z. Ding, T. Zhu, S. Z. Liu, X. Ren and L. H. Zou, Asian J. Org. Chem., 2016, 5, 625 CrossRef CAS;
(c) Y. J. Guo, R. Y. Tang, J. H. Li, P. Zhong and X. G. Zhang, Adv. Synth. Catal., 2009, 351, 2615 CrossRef CAS;
(d) Z. Li, L. Hong, R. Liu, J. Shen and X. Zhou, Tetrahedron Lett., 2011, 52, 1343 CrossRef CAS.
-
(a) W. Ge and Y. Wei, Green Chem., 2012, 14, 2066 RSC;
(b) X. Liu, H. Cui, D. Yang, S. Dai, G. Zhang, W. Wei and H. Wang, Catal. Lett., 2016, 146, 1743 CrossRef CAS;
(c) P. Katrun, S. Hongthong, S. Hlekhlai, M. Pohmakotr, V. Reutrakul, D. Soorukram, T. Jaipetchb and C. Kuhakarn, RSC Adv., 2014, 4, 18933 RSC;
(d) G. Kumaraswamy, R. Rajua and V. Narayanarao, RSC Adv., 2015, 5, 22718 RSC;
(e) T. Guo and X.-N. Wei, Synlett, 2017, 28, 2499 CrossRef CAS;
(f) J. B. Azeredo, M. Godoi, G. M. Martins, C. C. Silveira and A. L. Braga, J. Org. Chem., 2014, 79, 4125 CrossRef CAS PubMed;
(g) P. Choudhury, B. Roy and B. Basu, Asian J. Org. Chem., 2017, 6, 1569 CrossRef CAS;
(h) H. Zhang, X. Bao and Y. Song, Tetrahedron, 2015, 71, 8885 CrossRef CAS.
-
(a) Y. Liu, Y. Zhang, C. Hu, J. P. Wan and C. Wen, RSC Adv., 2014, 4, 35528 RSC;
(b) P. Sang, Z. Chen, J. Zou and Y. Zhang, Green Chem., 2013, 15, 2096 RSC.
- T. Hostier, V. Ferey, G. Ricci, D. G. Pardo and J. Cossy, Chem. Commun., 2015, 51, 13898 RSC.
-
(a) Y. Cai, J. Li, W. Chen, M. Xie, X. Liu, L. Lin and X. Feng, Org. Lett., 2012, 14, 2726 CrossRef CAS;
(b) C. J. Nalbandian, E. M. Miller, S. T. Toenjes and J. L. Gustafson, Chem. Commun., 2017, 53, 1494 RSC.
-
(a) T. Hostier, V. Ferey, G. Ricci, D. G. Pardo and J. Cossy, Chem. Commun., 2015, 51, 13898 RSC;
(b) C. J. Nalbandian, E. M. Miller, S. T. Toenjes and J. L. Gustafson, Chem. Commun., 2017, 53, 1494 RSC.
-
(a) Q. Wu, D. Zhao, X. Qin, J. Lan and J. You, Chem. Commun., 2011, 47, 9188 RSC;
(b) M. Chen, Z. T. Huang and Q. Y. Zheng, Chem. Commun., 2012, 48, 11686 RSC;
(c) R. Rahaman, N. Devi, J. R. Bhagawati and P. Barman, RSC Adv., 2016, 6, 18929 RSC;
(d) G. Tocco, M. Begala, F. Esposito, P. Caboni, V. Cannas and E. Tramontano, Tetrahedron Lett., 2013, 54, 6237 CrossRef CAS.
-
(a) P. Sang, Z. Chen and J. Zou, Green Chem., 2013, 15, 2096 RSC;
(b) G. L. Regina, V. Gatti, V. Famiglini, F. Piscitelli and R. Silvestri, ACS Comb. Sci., 2012, 14, 258 CrossRef;
(c) S. Yi, M. Li, W. Mo, X. Hu, B. Hu, N. Sun, L. Jin and Z. Shen, Tetrahedron Lett., 2016, 57, 1912 CrossRef CAS;
(d) Q. Shi, P. Li, Y. Zhang and L. Wang, Org. Chem. Front., 2017, 4, 1322–1330 RSC.
-
(a) C. Ravi, A. Joshi and S. Adimurthy, Eur. J. Org. Chem., 2017, 3646 CrossRef CAS;
(b) F. X. Wang, S. D. Zhou, C. Wang and S.-K. Tian, Org. Biomol. Chem., 2017, 15, 5284 RSC.
-
(a) Y. Yang, S. Zhang, L. Tang, Y. Hu, Z. Zha and Z. Wang, Green Chem., 2016, 18, 2609 RSC;
(b) F.-L. Yang and S.-K. Tian, Angew. Chem., Int. Ed., 2013, 52, 4929 CrossRef CAS PubMed;
(c) J.-K. Qiu, W. J. Hao, D. C. Wang, P. Wei, J. Sun, B. Jiang and S.-J. Tu, Chem. Commun., 2014, 50, 14782 RSC;
(d) X. Li, Y. Xu, W. Wu, C. Jiang, C. Qi and H. Jiang, Chem. – Eur. J., 2014, 20, 7911 CrossRef CAS;
(e) U. Nookaraju, E. Begari, R. R. Yetra and P. Kumar, ChemistrySelect, 2016, 1, 81 CrossRef CAS.
- P. Wang, S. Tang, P. Huang and A. Lei, Angew. Chem., Int. Ed., 2017, 56, 3009 CrossRef CAS.
- R. Rahaman, S. Das and P. Barman, Green Chem., 2018, 20, 141 RSC.
-
(a) R. Nie, R. Sang, X. Ma, Y. Zheng, X. Cheng, W. Li, L. Guo, H. Jin and Y. Wu, J. Catal., 2016, 344, 286 CrossRef CAS;
(b) X. Cheng, W. Li, R. Nie, X. Ma, R. Sang, L. Guo and Y. Wu, Adv. Synth. Catal., 2017, 359, 454 CrossRef CAS;
(c) X. Ma, G. Lv, X. Cheng, W. Li, R. Sang, Y. Zhang, Q. Wang, L. Hai and Y. Wu, Appl. Organomet. Chem., 2017, 31, 3854 CrossRef;
(d) W. Li, G. Lv, X. Cheng, R. Sang, X. Ma, Y. Zhang, R. Nie, J. Li, M. Guan and Y. Wu, Tetrahedron, 2016, 72, 8557 CrossRef CAS;
(e) R. Sang, X. Cheng, W. Li, P. Luo, Y. Zhang, R. Nie, L. Hai and Y. Wu, Chem. Res. Chin. Univ., 2017, 33, 811 CrossRef CAS;
(f) Y. Wang, R. Sang, Y. Zheng, L. Guo, M. Guan and Y. Wu, Catal. Commun., 2017, 89, 138 CrossRef CAS.
-
(a) P. Xie, X. Ge, B. Fang, Z. Li, Y. Liang and C. Yang, Colloid Polym. Sci., 2013, 291, 1631 CrossRef CAS;
(b) X. Song, Y. Yang, J. Liu and H. Zhao, Langmuir, 2011, 27, 1186 CrossRef CAS;
(c) P. Guo, H. Song and X. Chen, J. Mater. Chem., 2010, 20, 4867 RSC;
(d) L. J. Cote, J. Kim, V. C. Tung, J. Luo, F. Kim and J. Huang, Pure Appl. Chem., 2010, 83, 95 Search PubMed;
(e) X. Yi, A. Feng, W. Shao and Z. Xiao, High Perform. Polym., 2016, 28, 505 CrossRef CAS.
- S. Park and R. S. Ruoff, Nat. Nanotechnol., 2009, 4, 217 CrossRef CAS.
-
(a) W. Cai, R. D. Piner, F. J. Stadermann, S. Park, M. A. Shaibat, Y. Ishii, D. Yang, A. Velamakanni, S. J. An, M. Stoller, J. An, D. Chen and R. S. Ruoff, Science, 2008, 321, 1815 CrossRef CAS;
(b) D. A. Dikin, S. Stankovich, E. J. Zimney, R. D. Piner, G. H. B. Dommett, G. Evmenenko, S. T. Nguyen and R. S. Ruoff, Nature, 2007, 448, 457 CrossRef CAS;
(c) D. Li, M. B. Muller, S. Gilje, R. B. Kaner and G. G. Wallace, Nat. Nanotechnol., 2008, 3, 101 CrossRef CAS;
(d) H. V. Kumar, K. Y. S. Huang, S. P. Ward and D. H. Adamson, J. Colloid Interface Sci., 2017, 493, 365 CrossRef CAS.
-
(a) S. Linley, Y. Liu, C. J. Ptacek, D. W. Blowes and F. X. Gu, ACS Appl. Mater. Interfaces, 2014, 6, 4658 CrossRef CAS;
(b) J. Li, M. Wang, Y. Zhang, Z. Fan, W. Zhang, F. Sun and N. Ma, ACS Sustainable Chem. Eng., 2016, 4, 3189 CrossRef CAS;
(c) Z. Zhou, M. Su and K. Shih, J. Alloys Compd., 2017, 725, 302 CrossRef CAS.
-
(a) J. Kim, L. J. Cote, F. Kim, W. Yuan, K. R. Shull and J. Huang, J. Am. Chem. Soc., 2010, 132, 8180 CrossRef CAS;
(b) P. Xie, X. Ge, B. Fang, Z. Li, Y. Liang and C. Yang, Colloid Polym. Sci., 2013, 291, 1631 CrossRef CAS.
-
(a) D. R. Dreyer, S. Park, C. W. Bielawski and R. S. Ruoff, Chem. Soc. Rev., 2010, 39, 228 RSC;
(b) Saima, D. Equbal, A. G. Lavekar and A. K. Sinha, Org. Biomol. Chem., 2016, 14, 6111 RSC;
(c) W. Guo, W. Tan, M. Zhao, K. Tao, L. Y. Zheng, Y. Wu, D. Chen and X. L. Fan, RSC Adv., 2017, 7, 37739 RSC.
- D. Voylov, T. Saito, B. Lokitz, D. Uhrig, Y. Wang, A. Agapov, A. Holt, V. Bocharova, Al. Kisliuk and Al. P. Sokolov, ACS Macro Lett., 2016, 5, 199 CrossRef CAS.
Footnote |
† Electronic supplementary information (ESI) available: All experimental details, details of chemical synthesis, additional figures and tables. See DOI: 10.1039/c8qo00726h |
|
This journal is © the Partner Organisations 2019 |
Click here to see how this site uses Cookies. View our privacy policy here.