Photo-polymerization properties of type-II photoinitiator systems based on 2-chlorohexaaryl biimidazole (o-Cl-HABI) and various N-phenylglycine (NPG) derivatives†
Received
12th July 2018
, Accepted 26th October 2018
First published on 29th October 2018
Abstract
A series of p-substituted NPG derivatives (Cl-NPG, OMe-NPG and NO2-NPG) comprising different push–pull characteristics have been synthesized and characterized. The NPG derivatives have good thermal stability and red shifted absorption when compared with the original N-phenyl glycine (NPG) compound. These NPGs were selected in combination with 2-chlorohexaaryl biimidazole (o-Cl-HABI) for Type II free radical polymerization (FRP). Commercial NPG was also mixed with o-Cl-HABI for comparison. Their photo-polymerization properties were investigated by the gel fraction method in a nitrogen atmosphere. Electron transfer efficiencies for those Type II packages were studied by cyclic voltammetry (CV) and free energy change ΔG results.
Introduction
Photopolymerization reaction is the process of converting monomers into polymers initiated by photoinitiators (PIs) or photoinitiator packages under a suitable light irradiation.1 Owing to the change in phase or solubility, these techniques have a wide range of industrial applications such as coating, adhesives, 3D printing, microlithography, optics, medicine, and nanotechnology.2–7 Free radical polymerization (FRP) and cationic polymerization (CP) are the most common procedures used in photochemical reaction for different photocuring applications.8 Among them, the FRP was paid more attention to due to several advantages compared to CP, such as rapid curing ability, flexible monomer chemistry, and spatial and temporal control.9 FRP processes are classified into two types depending on their decompositions mechanism including (1) Type I or α-cleavage PIs, also called one-component photoinitiator, involving generation of radicals by PIs directly via a homolytic cleavage process under light irradiation, and (2) Type II PIs involving radical generation and electron transfer processes between hydrogen acceptor and hydrogen donor units.9,10 The Type II PIs are more useful than Type I PIs due to better optical absorption properties in the near-visible wavelength region.11
Photoresist for printed circuit board (PCB) was one of the industrial application using Type II PIs. These kinds of photo-imaginable materials are also called negative photoresist or dry films, which were developed by DuPont in the 1960s.12 These kinds of PIs are generally used as hexaaryl biimidazole (HABI, or lophine dimer, L2) and its derivatives such as 2-chlorohexaaryl biimidazole (o-Cl-HABI) as hydrogen acceptor compounds. The wide use of HABI compound was due to the high yield of the lophyl radical (L˙) generation, low sensitivity in the presence of oxygen and long lifetime.13 Currently, the incorporation of substituted functional group into the HABI backbone has received much attention due to the influence on UV-visible absorption and photo-reactivity.14
As for the co-initiator, which is the hydrogen donor ingredient, the most common hydrogen donor including amine or thiol derivatives were selected for industrial applications. N-Phenylglycine (NPG), a well-known amino acid, has been widely used due to biologically less-toxic, non-allergic and odorless nature compared to thiol derivatives.15 In addition, the NPG derivatives have gained considerable interest for application in various fields such as drugs,16 reprography,17 and dental restorative materials.15 To summarize, the photodecomposition of NPGs and its derivatives (NPGs) can be sensitized by lots of initiator ingredients or multicomponent photoinitiator systems under UV-light.17–19 For example, Rabek et al. presented the photoreactivity of camphorquinone (CD) and different amines. With different NPG derivatives, the photo-reactivity changes significantly. Among them, NPG showed good rate of polymerization than the others.18 Currently, researchers adopted NPG as coinitiators for two or multicomponent CP or FRP applications. The NPG-based PI packages also demonstrate excellent photoreactivity under LED as light source and can be a good candidate for 3D printing or sensors.20,21
Previously, we had studied photo-reactivity based on different weight percentages of o-Cl-HABI and NPG PIs. It was found that the NPG compound had a great impact on photo-reactivity than o-Cl-HABI.22 The corresponding proposed mechanism and scheme of this type II PIs based on o-Cl-HABI and NPG is shown below: (1) the formation of L˙ after light irradiation, (2) the lophyl radical can abstract hydrogen atom from hydrogen donor coinitiators (NPGs), (3) the NPG radical was generated by the decarboxylation process, which then initiated polymerization of monomers with the addition of vinyl groups.
To continue our study on investigating
o-Cl-HABI/NPG photoinitiator package, a series of
p-substituted NPG derivatives with different electronics push–pull characteristics,
i.e. OMe-NPG (methoxy group), Cl-NPG (chloro group) and NO
2-NPG (nitro group) as shown in
Fig. 1 were designed and synthesized. In addition, their photo-reactivity, thermal properties, solubility, photo-physical and electrochemical properties are analyzed and compared with the original coinitiator, NPG.
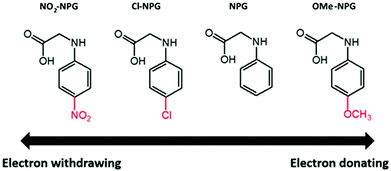 |
| Fig. 1 Structures of N-phenyl glycine (NPG) and NPG derivatives (OMe-NPG, Cl-NPG and NO2-NPG). | |
Experimental section
Materials
2-Chlorohexaaryl biimidazole (o-Cl-HABI), N-phenylglycine (NPG), and trimethylolpropane trimethacrylate (TMPTMA) were supplied by DuPont, Taiwan. 4-Chloroaniline, p-anisidine, 4-nitroaniline, ethyl chloroacetate, 2-bromoacetic acid, and sodium acetate were purchased from Sigma-Aldrich and used as received without further purification. Solvents: hexane, methanol, ethanol, tetrahydrofuran (THF), dimethylformamide (DMF), and acetone were used as received.
Synthesis of NPG derivatives (Cl-NPG, OMe-NPG and NO2-NPG) (Scheme 1)
Cl-NPG (N-(4-chlorophenyl)glycine).
4-Chloroaniline (20 g, 0.157 mol), ethyl chloroacetate (22 mL, 0.155 mol) and sodium acetate (15 g, 0.19 mol) were mixed together in 100 mL ethanol used as the solvent. The solution was refluxed for 12 h with stirring. The reaction solution was left overnight at room temperature and filtered to obtain a clear solution. The clear solution was then poured into iced water. The precipitate formed was collected by filtration and dried. The dried intermediate, ethyl ester of Cl-substituted glycine, was used for the next step without further purification. Aqueous sodium hydroxide solution (2.25 M, 50 mL) and ethyl ester of Cl-substituted glycine were mixed together and refluxed for 1 h. After cooling, the reaction mixture was acidified using aqueous hydrochloric acid to pH = 2. The precipitated light yellow Cl-substituted glycine (Cl-NPG) solid was filtered and washed thoroughly with cold water (3.6 g, 49.1%) (m.p. = 146 °C). 1H-NMR (400 MHz, CDCl3, δ, ppm): 7.16 (d, J = 9.2 Hz, 2H, Ha), 6.55 (d, J = 8.8 Hz, 2H, Hb), 3.96 (s, 2H, –CH2, Hc). HRMS m/z [M]+ calculated for C8H8ClNO2, 186.03163 found: 186.03159.
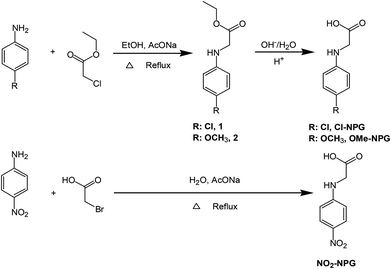 |
| Scheme 1 Synthesis route for N-phenyl glycine derivatives (OMe-NPG, Cl-NPG and NO2-NPG). | |
OMe-NPG (N-(4-methoxyphenyl)glycine).
The procedure used for this synthesis was similar to that described for Cl-NPG. p-Anisidine (8 g, 0.07 mol), ethyl chloroacetate (6 mL, 0.04 mol) and sodium acetate (5.6 g, 0.07 mol) were mixed together in 50 mL ethanol used as the solvent to obtain the OMe-NPG (2.3 g, 43%). (m.p. = 165 °C) (m.p. = 142 °C).231H-NMR (400 MHz, DMSO-d6, δ, ppm): 8.8 (1H, –COOH, Hd), 6.71 (d, J = 9.2 Hz, 2H, Ha), 6.5 (d, J = 9.2 Hz, 2H, Hb), 3.72 (s, 2H, –CH2, Hc), 3.63 (s, 3H, –OCH3, He). HRMS m/z [M]+ calculated for C9H11NO3, 182.08117 found: 182.08128.
NO2-NPG (N-(4-nitrophenyl)glycine).
4-Nitrobenzenamine (3 g, 0.02 mol), 2-bromoacetic acid (3 g, 0.02 mol) and sodium acetate (3.5 g, 0.04 mol) were mixed together in 40 mL H2O used as the solvent. The solution was refluxed for 24 h with stirring. The reaction solution was filtered hot to remove the impurity, and the filtrate was cooled to precipitate the crude product. Then, the product was subjected to column chromatography using ethyl acetate as the eluent to give the yellow solid (0.7 g, 20.1%) (m.p. = 238 °C). 1H-NMR (400 MHz, DMSO-d6, δ, ppm): 12.83 (1H, –COOH, He), 8.00 (d, J = 9.2 Hz, 2H, Ha), 7.76 (1H, –NH, Hc), 6.66 (d, J = 9.2 Hz, 2H, Hb), 3.99 (3H, –CH2, Hd). EI-Mass m/z [M] calculated for C8H8N2O4, 196.16, found: 196.0483.
Degree of conversion (DC), gel fraction
The gel fraction method was selected in this study to obtain the DC value and calculated by the following eqn (1).24 Gel fraction is a convenient method to measure the insoluble fractions, such as the cross-linked or network polymers. Typical formulations (TMPTMA/o-Cl-HABI/NPGs = 97.95/2/0.05 wt%) were used in this study for gel fraction testing. First, TMPTMA, o-Cl-HABI and relative NPG derivatives were mixed together at weight ratios of 97.95
:
2
:
0.05 using CH2Cl2 as the solvent. After completely dissolution, the solvent was removed and poured into the plate with fixed area and weight (around 0.5 g). Subsequently, the formulation was exposed for 120 s under nitrogen atmosphere. Finally, the cured sample was washed by Soxhlet extraction using hexane as the solvent for 6 h. After that, the sample was filtered out and dried to get the final polymerized polymer. | 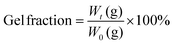 | (1) |
where W0 is the original weight and Wt is the weight after drying. To ensure the results, the average of at least three separate determinations was used.
Measurements
1H-NMR spectra were recorded using an Agilent Unity plus-400 spectrometer. High-Resolution Mass spectra (HR-MS, FAB) and Electron Ionization spectra (EI) were recorded using a Bruker APEX II mass spectrometer and a JEOL JMS-700 mass spectrometer, respectively. Ultraviolet–visible (UV-vis) spectra were recorded using a PerkinElmer Lambda 35 UV/VIS Spectrometer. Cyclic voltammetry (CV) was conducted using a BioLogic SP-150 model at a scan rate of 100 mV s−1. Melting Point (Tm) was determined using TA Instruments DSC 2920 Modulated at a heating rate of 10 °C min−1 from 30 to 250 °C in a nitrogen atmosphere. The photopolymerization was conducted using a Philips 16 W lamp (type Actinic BL) with a total light around 605 mJ cm−2 for 120 s exposure time, which was tested using a radiometer.
Results and discussion
The synthetic procedures for the series of NPG derivatives (Cl-NPG, OMe-NPG and NO2-NPG) are shown in Scheme 1. The Cl-NPG and OMe-NPG were synthesized according to the method reported previously in the literature.23 First, the p-substituted anilines were reacted with ethyl chloroacetate in the presence of base as catalyst to afford the intermediates 1 and 2. Subsequently, the relative acetic ester groups were converted into acid groups and the target compounds were obtained (Cl-NPG and OMe-NPG). On the other hand, the NO2-NPG compound was obtained directly from p-substituted aniline with 2-bromoacetic acid in the presence of base as catalyst. 1H-NMR and HR-MS were used to confirm the structural characteristics of NPG derivatives. Fig. 2 presents the 1H-NMR spectra of Cl-NPG, OMe-NPG and NO2-NPG. The aromatic protons for these three compounds resonated in the region of 6.55–7.16, 6.5–6.71, and 6.66–8.00 ppm, and their methyl protons (–CH2) appeared in the region of 3.96, 3.72, and 3.99 ppm, respectively. A slightly downfield shift of the aromatic protons for NO2-NPG compound was attributed to the electron-withdrawing characteristic of the nitro group. In addition, the carboxyl group of the OMe-NPG and NO2-NPG could be measured in regions of 8.88 and 12.83 ppm, respectively. Furthermore, the HR-MS spectroscopies were confirmed with the proposed structures. Thus, these results are in good agreement with the expected structures of Cl-NPG, OMe-NPG and NO2-NPG.
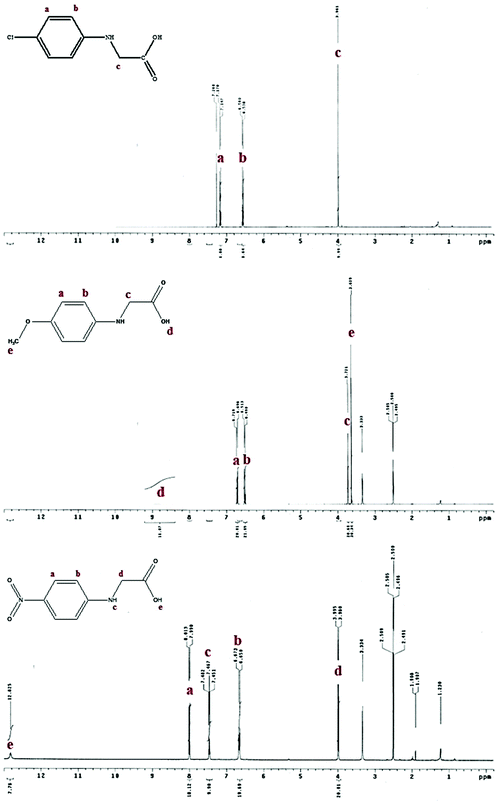 |
| Fig. 2
1H-NMR spectra of OMe-NPG, Cl-NPG and NO2-NPG. | |
The melting points of these NPG derivatives were evaluated by DSC. In addition, the o-Cl-HABI and NPG was also conducted in this study. Fig. 3 shows the DSC curves of these compounds and their relevant data are summarized in Table 1. DSC experiments were carried out at a heating rate of 10 °C min−1 in a nitrogen atmosphere. The melting points of these compounds are related to the relative ease or difficulty of overcoming the cohesive forces between the molecules. The melting point of o-Cl-HABI was 210 °C. Moreover, the melting points of the NPGs were in the order of NO2-NPG (238 °C) > OMe-NPG (165 °C) > Cl-NPG (146 °C) > NPG (127 °C). All the NPG derivatives showed higher melting point than the reference NPG sample, which indicated that all the samples showed higher thermal stability than NPG. Moreover, NO2-NPG showed the highest Tm due to the presence of the strong electron-withdrawing nitro group, resulting in larger dipole moment.25,26
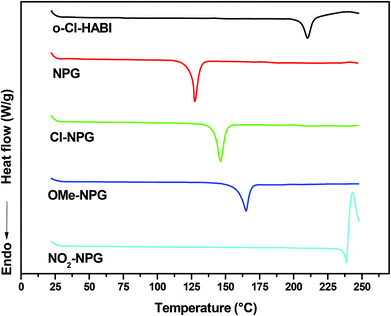 |
| Fig. 3 DSC curves of o-Cl-HABI and NPG derivatives. | |
Table 1 Characteristic parameters of o-Cl-HABI and NPG derivatives
Compound |
λ
abs
(ε) (M−1 cm−1) nm |
E
ox
(V) |
E
red
(V) |
E
g
(eV) |
ΔGe (V) |
T
m
(°C) |
Recorded in THF solutions at 10−5 M.
Recorded in THF solutions. Scan rate, 100 mV s−1; electrolyte, nBu4NPF6. The oxidation potentials were measured by cyclic voltammetry.
The reduction potential was also measured by cyclic voltammetry.
The bandgap, Eg, was derived from the offset of the absorption spectra.
From the Rehm–Weller equation. ΔG = Eox − Ered − Eg. Eox: oxidation potential of relative NPGs; Ered: reduction potential of o-Cl-HABI; Eg: band gap of the NPGs.
Baseline shift in the second heating DSC traces, at a heating rate of 10 °C min−1.
|
o-Cl-HABI
|
287 (17 460) |
— |
−1.71 |
— |
— |
210 |
NPG
|
280 (4681), 289 (4344) |
0.69 |
— |
3.94 |
−1.54 |
127 |
Cl-NPG
|
278 (2580), 308 (2080) |
0.82 |
— |
3.75 |
−1.22 |
146 |
OMe-NPG
|
280 (1094), 291 (1570), 314 (2162) |
0.44 |
— |
3.64 |
−1.49 |
165 |
NO2-NPG
|
280 (2208), 289 (1767), 371 (11 251) |
1.23 |
— |
2.99 |
−0.05 |
238 |
For ensuring well-dissolved for the formulation (TMPTMA/o-Cl-HABI/NPGs), the solubility in various organic solvents is very important. The solubility for the o-Cl-HABI and NPGs samples was determined at a concentration of 1 mg of compound in 1 mL of solvent and the results are listed in Table 2. All the samples showed good solubility in aprotic polar solvent, DMF, and were also soluble in low-boiling solvents such as THF and acetone. However, the NO2-NPG and OMe-NPG samples showed poor solubility in CH2Cl2 and dissolved in MeOH after heating. The overall solubilities of the NPG derivatives were in the following order: NPG > Cl-NPG > OMe-NPG ≈ NO2-NPG. The poor solubility of OMe-NPG ≈ NO2-NPG was due to the stronger intramolecular strength than other samples. This is also consistent with the melting point results mentioned above. Thus, to ensure the well-dispersed formulation for DC testing, we used THF as the solvent in latter formulation.
Table 2 Solubility behavior of o-Cl-HABI and NPG derivatives in various organic solventsa
Compound |
DMF |
CH2Cl2 |
THF |
CH3OH |
Acetone |
Solubility was determined with 1 mg of compound in 1 mL of solvent. ○ = soluble at room temperature; Δ = soluble on heating; X = insoluble even on heating.
|
o-Cl-HABI
|
○ |
○ |
○ |
○ |
○ |
NPG
|
○ |
○ |
○ |
○ |
○ |
Cl-NPG
|
○ |
Δ |
○ |
○ |
○ |
OMe-NPG
|
○ |
X |
○ |
Δ |
○ |
NO2-NPG
|
○ |
X |
○ |
Δ |
○ |
The UV-visible absorption spectra of all the samples of o-Cl-HABI and NPGs in THF solution are shown in Fig. 4, and their corresponding data are listed in Table 1. The o-Cl-HABI showed broader and higher absorption than NPG ranging from 250–325 nm. All the NPGs have the absorption bands shorter than 300 nm due to the localized π–π* transition. In addition, p-substituted NPG derivatives show that the long absorption at >300 nm are from more delocalized π–π* transition with charge transfer character. Thus, the absorption bands decrease in the order of NO2-NPG > OMe-NPG > Cl-NPG > NPG depending on the push–pull characteristics. In addition, the original NPG exhibited smaller absorption at >300 nm, which indicated the insignificant charge transfer for this molecule.
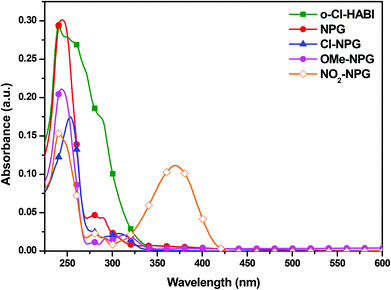 |
| Fig. 4 UV-vis absorption of o-Cl-HABI and NPG derivatives in THF solutions. | |
To investigate the DC values with o-Cl-HABI/NPGs packages, the free energy changes, ΔG, were calculated. The ΔG values illustrated the electron transfer phenomenon between o-Cl-HABI and NPGs, and these values were calculated from the classical Rehm–Weller equation,27i.e. ΔG = Eox − Ered − Eg + C, where Eox, Ered, and Eg are the oxidation potential of the electron donor (NPGs), the reduction potential of the electron acceptor (o-Cl-HABI), and the excited singlet state energies of NPGs, respectively. In addition, C is the electrostatic interaction energy for the initially formed ion pair, generally considered as negligible in polar solvents.27 Thus, Eox of NPGs, Ered of o-Cl-HABI and Eg of NPGs can be measured by cyclic voltammetry and the cutoff of the absorption spectra. The oxidation spectra of the NPGs, reduction spectra of the o-Cl-HABI and their corresponding results are summarized in Fig. 5, Fig. S1 and Table 1.† The values of the relative ΔG were also obtained based on the Rehm–Weller equation mentioned above and also summarized in Table 1. The ΔG values of o-Cl-HABI/NPG, o-Cl-HABI/Cl-NPG, o-Cl-HABI/OMe-NPG and o-Cl-HABI/NO2-NPG were −1.54, −1.22, −1.49 and −0.05 V, respectively. The ΔG value of the o-Cl-HABI/NPG package is lower than other packages, suggesting that this package has the most spontaneous electron transfer characteristic.28 Furthermore, the ΔG value of the o-Cl-HABI/NO2-NPG package is close to zero, which indicates nearly no electron transfer relative to this package.
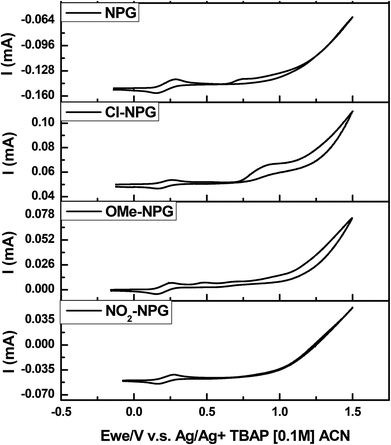 |
| Fig. 5 Cyclic voltammograms of the o-Cl-HABI and NPG derivatives in THF solutions. | |
The degree of conversion (DC %) was also tested by gel fraction method mentioned above (eqn (1)). Fig. 6 exhibits gel fraction results under a similar exposure time (120 s) with the weight composition of o-Cl-HABI
:
NPGs at 2
:
0.05. The exposed environment is a critical issue to determine the conversion efficiency of the PI package.22 In order to correctly and systematically realize the DC values of different packages, we eliminated the effects from the uncertainty of atmosphere. Thus, the gel fraction results were tested in a nitrogen atmosphere for the reduction of the NPGs diluted effects by air during the exposed period. The DC % decreases in the order of o-Cl-HABI/NPG (51.7%) > o-Cl-HABI/OMe-NPG (41.8%) > o-Cl-HABI/Cl-NPG (35.5%) > o-Cl-HABI/NO2-NPG (0%). A higher DC value implies good electron transfer from hydrogen acceptor to hydrogen donor, that is, the o-Cl-HABI/NPG has the best electron transfer efficiency than others. These results are also consistent with the free energy changes mentioned above. In addition, the o-Cl-HABI/NO2-NPG showed nearly no reaction after exposure for 120 s, which might be due to no electron transfer between o-Cl-HABI and NO2-NPG.
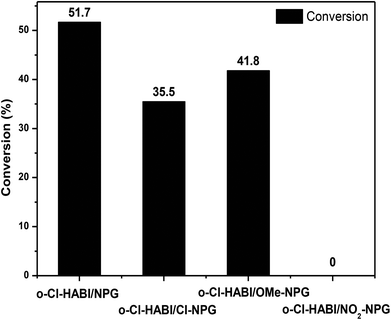 |
| Fig. 6 Conversion rate of TMPTMA monomer in the presence of o-Cl-HABI and different NPG derivatives at fixed weight ratios and exposure times (120 s) in a nitrogen atmosphere. | |
Conclusions
In conclusion, a series of p-substituted NPG derivatives (Cl-NPG, OMe-NPG and NO2-NPG) have been synthesized and characterized. The NPG derivatives have good thermal stability and red shift absorption than the pristine NPG analogue. However, the DC value of those o-Cl-HABI/NPGs package is still lower than that of o-Cl-HABI/NPG. We created the electron transfer correlation between o-Cl-HABI and NPGs, which should be useful for structural designing of these kinds of type II photoinitiator package.
Conflicts of interest
There are no conflicts to declare.
Acknowledgements
Financial support from (1) Ministry of Science and Technology, Taiwan ROC and (2) DuPont, Taiwan is gratefully acknowledged.
References
- Y. Yagci, S. Jockusch and N. J. Turro, Photoinitiated polymerization: advances, challenges, and opportunities, Macromolecules, 2010, 43, 6245–6260 CrossRef CAS
.
-
W. A. Green, Industrial photoinitiators: a technical guide, CRC Press, Boca Raton, FL, 2010 Search PubMed
.
- M. Layani, X. Wang and S. Magdassi, Novel materials for 3D printing by photopolymerization, Adv. Mater., 2018, 1706344 CrossRef PubMed
.
- I. V. Khudyakov, Fast photopolymerization of acrylate coatings: Achievements and problems, Prog. Org. Coat., 2018, 121, 151–159 CrossRef CAS
.
- J. V. Crivello and E. Reichmanis, Photopolymer materials and processes for advanced technologies, Chem. Mater., 2014, 26(1), 533–548 CrossRef CAS
.
- Y. Fuchs, O. Soppera and K. Haupt, Photopolymerization and photostructuring of molecularly imprinted polymers for sensor applications—A review, Anal. Chim. Acta, 2012, 717, 7–20 CrossRef CAS PubMed
.
- N. A. Chartrain, C. B. Williams and A. R. Whittington, A review on fabricating tissue scaffolds using vat photopolymerization, Acta Biomater., 2018, 74, 90–111 CrossRef CAS PubMed
.
- P. Xiao, J. Zhang, F. Dumur, M. A. Tehfe, F. Morlet-Savary, B. Graff, D. Gigmes, J. P. Fouassier and J. Lalevée, Visible light sensitive photoinitiating systems: Recent progress in cationic and radical photopolymerization reactions under soft conditions, Prog. Polym. Sci., 2015, 41, 32–66 CrossRef CAS
.
-
(a) H. J. Hageman, Photoinitiators for free radical polymerization, Prog. Org. Coat., 1985, 13, 123–150 CrossRef CAS
;
(b) H. F. Gruber, Photoinitiators for free radical polymerization, Prog. Polym. Sci., 1992, 17, 953–1044 CrossRef CAS
;
(c) R. S. Davidson, Polymeric and polymerisable free radical photoinitiators, J. Photochem. Photobiol., A., 1993, 69, 263–275 CrossRef
.
- M. El-Roz, J. Lalevée, X. Allonas and J. P. Fouassier, Mechanistic Investigation of the Silane, Germane, and Stannane Behavior When Incorporated in Type I and Type II Photoinitiators of Polymerization in Aerated Media, Macromolecules, 2009, 42(22), 8725–8732 CrossRef CAS
.
-
J. Lalevée, Photopolymerisation Initiating Systems, Polymer Chemistry Series, 2018, 10.1039/9781788013307
.
-
T. F. Haller Jr. and R. C. Stumpf, Institute of Printed Circuits, Evanston, IL, Proceedings of the September Fall Meeting, Los Angeles, 1968 Search PubMed
.
- X. Z. Qin, A. Liu, A. D. Trifunac and V. V. Krongauz, Photodissociation of hexaarylbiimidazole. 1. Triplet-state formation, J. Phys. Chem., 1991, 95(15), 5822–5826 CrossRef CAS
.
-
(a) D. Ahn, S. R. Zavada and T. F. Scott, Rapid, photomediated healing of hexaarylbiimidazole-based covalently cross-linked gels, Chem. Mater., 2017, 29(16), 7023–7031 CrossRef CAS
;
(b) W. L. Gong, G. F. Zhang, C. Li, M. P. Aldred and M. Q. Zhu, Design, synthesis and optical properties of a green fluorescent photoswitchable hexaarylbiimidazole (HABI) with non-conjugated design, RSC Adv., 2013, 3, 9167–9170 RSC
;
(c) D. Ahn, S. S. Sathe, B. H. Clarkson and T. F. Scott, Hexaarylbiimidazoles as visible light thiol–ene photoinitiators, Dent. Mater., 2015, 31, 1075–1089 CrossRef CAS PubMed
.
- Z. Kucybala, M. Pietrazk, J. Paczkowski, L. Å. Linden and J. F. Rabek, Kinetic studies of a new photoinitiator hybrid system based on camphorquinone-N-phenylglicyne derivatives for laser polymerization of dental restorative and stereolithographic (3D) formulations, Polymer, 1996, 37, 4585–4591 CrossRef CAS
.
- N. B. Tien, N. P. Buu-Hoï and N. D. Xuong, Tuberculostatic N-arylglycines and derivatives, J. Org. Chem., 1958, 23, 186–188 CrossRef CAS
.
- S. Ikeda and S. Murata, Photolysis of N-phenylglycines sensitized by polycyclic aromatic hydrocarbons effects of sensitizers and substituent groups and application to photopolymerization, J. Photochem. Photobiol., A, 2002, 149, 121–130 CrossRef CAS
.
- J. Jakubiak, X. Allonas, J. P. Fouassier, A. Sionkowska, E. Andrzejewska, L. Å. Linden and J. F. Rabek, Camphorquinone–amines photoinitating systems for the initiation of free radical polymerization, Polymer, 2003, 44, 5219–5226 CrossRef CAS
.
-
(a) J. P. Fouassier and J. Lalevée, Three-component photoinitiating systems: towards innovative tailor made high performance combinations, RSC Adv., 2012, 2, 2621–2629 RSC
;
(b) X. Allonas, J. P. Fouassier, H. Obeid, M. Kaji and Y. Ichihashi, Mechanistic analysis of photopolymerization reactions in the presence of HABI/Hydrogen donor photoinitiator system, J. Photopolym. Sci. Technol., 2004, 17, 35–40 CrossRef CAS
.
- J. Zhang, J. Lalevée, X. Mou, F. Morlet-Savary, B. Graff and P. Xiao, N-Phenylglycine as a versatile photoinitiator under near-UV LED, Macromolecules, 2018, 51, 3767–3773 CrossRef CAS
.
- A. A. Mousawi, P. Garra, M. Schmitt, J. Toufaily, T. Hamieh, B. Graff, J. P. Fouassier, F. Dumur and J. Lalevée, 3-Hydroxyflavone and N-phenylglycine in high performance photoinitiating systems for 3D printing and photocomposites synthesis, Macromolecules, 2018, 51, 4633–4641 CrossRef
.
- Y. C. Chen and Y. T. Kuo, Photocuring kinetic studies of TMPTMA monomer by Type II photoinitiators of different weight ratios of 2-chlorohexaaryl biimidazole (o-Cl-HABI) and N-phenyl glycine (NPG), J. Photopolym. Sci. Technol., 2018, 31, 487–492 CrossRef CAS
.
- N. S. Rai, B. Kalluraya, B. Lingappa, S. Shenoy and V. G. Puranic, Convenient access to 1,3,4-trisubstituted pyrazoles carrying 5-nitrothiophene moiety via 1,3-dipolar cycloaddition of sydnones with acetylenic ketones and their antimicrobial evaluation, Eur. J. Med. Chem., 2008, 43, 1715–1720 CrossRef PubMed
.
- D. Kunwong, N. Sumanochitraporn and S. Kaewpirom, Curing behavior of a UV-curable coating based on urethane acrylate oligomer: the influence of reactive monomers, Songklanakarin J. Sci. Technol., 2011, 33, 201–207 CAS
.
-
(a) S. Uesato, Y. Hashimoto, M. Nishino, Y. Nagaoka and H. Kuwajima, N-Substituted Hydroxyureas as Urease Inhibitors, Chem. Pharm. Bull., 2002, 50, 1280–1282 CrossRef CAS PubMed
;
(b) J. Oberski, R. Festag, C. Schmidt, G. Liissem, J. H. Wendorff, A. Greiner, M. Hopmeier and F. Motamedi, Synthesis and structure-property relationships of processable liquid crystalline polymers with arylenevinylene segments in the main chain for light-emitting applications, Macromolecules, 1995, 28, 8676–8682 CrossRef CAS
.
- P. Simamora and S. H. Yalkowsky, Group contribution methods for predicting the melting points and boiling points of aromatic compounds, Ind. Eng. Chem. Res., 1994, 33, 1405–1409 CrossRef CAS
.
- D. Rehm and A. Weller, Kinetics of fluorescence quenching by electron and H-atom transfer, Isr. J. Chem., 1970, 8, 259–271 CrossRef CAS
.
- J. Zhang, N. Zivic, F. Dumur, P. Xiao, B. Graff, D. Gigmes, J. P. Fouassier and J. Lalevée, A benzophenone-naphthalimide derivative as versatile photoinitiator of polymerization under near UV and visible lights, J. Polym. Sci., Part A: Polym. Chem., 2015, 53, 445–451 CrossRef CAS
.
Footnote |
† Electronic supplementary information (ESI) available. See DOI: 10.1039/c8pp00300a |
|
This journal is © The Royal Society of Chemistry and Owner Societies 2019 |