Glutathione as a photo-stabilizer of avobenzone: an evaluation under glass-filtered sunlight using UV-spectroscopy†
Received
27th July 2018
, Accepted 25th October 2018
First published on 31st October 2018
Abstract
Avobenzone is the most widely used UVA filter in sunscreen lotion and it is prone to degradation in the presence of sunlight/UV radiation. To overcome the photo-instability of avobenzone, various photostabilizers have been used as additives, including antioxidants such as vitamin C, vitamin E, and ubiquinone. In the present study, the well known antioxidant, glutathione, was evaluated for protecting avobenzone from photodegradation in the presence of glass-filtered sunlight. The features of glutathione as a skin whitener and a radical scavenger in cells have prompted the assessment of the photostabilzing activity of glutathione on avobenzone. Glutathione significantly attenuated the glass-filtered sunlight-induced degradation of avobenzone at equimolar or higher ratios of glutathione and avobenzone. Mutational studies have been undertaken to investigate the role of the thiol group and the isopeptide bond of glutathione on its photoprotection activity towards avobenzone. The thiol group of glutathione plays a vital role in exhibiting the photoprotection activity, which was further supported by the studies on photodegradation of avobonzone in the presence of β-mercaptoethanol. The dual role of glutathione as a skin whitening agent and a photostabilizer of avobenzone may be useful for the development of multipurpose cosmetic lotions.
Introduction
Sunscreens have been evolved not only to combat sunburn, but also to reduce the harmful effects of UVA (320–400 nm) radiation, such as photocarcinogenesis and photoaging.1–4 An important ingredient in sunscreen lotion is the UVA filter that prevents the UVA radiation from reaching deep into the skin.5–8 Avobenzone is one of the most widely used UVA filters in a majority of sunscreen formulations.9,10 It is still an extensively used UVA filter because of the cost effectiveness and the broad spectrum absorbance of UVA radiation.11,12 The major drawback associated with avobenzone is its photo-instability, i.e., it undergoes photodegradation upon exposure to sunlight/radiation.13–16 The photodegradation products of avobenzone cause deleterious side effects to the skin, including the oxidative damage by the radicals.17–19 In order to overcome the photodegradation of avobenzone and its associated side effects on the skin, various stabilizers were employed, including triplet quenchers such as octocrylene, and methylbenzylidine camphor.20–25 A triazine derivative was also employed as a stabilizer to block the diketo form of avobenzone.26 Recently, antioxidants such as vitamin C, vitamin E and ubiquinone were demonstrated as photostabilizers of avobenzone.13,27–29 Glutathione is a well-known peptidic antioxidant in the cellular environment (Fig. 1a) and is widely distributed across the phyla.30–34 The present study demonstrates the utility of glutathione as a photostabilzer of avobenzone. Even though peptides have proven applications in the cosmetic field,35,36 they were not tested thus far to improve the photostability of avobenzone.
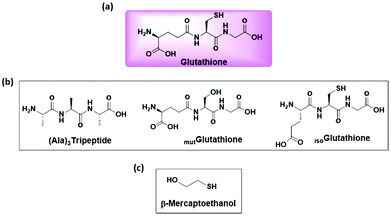 |
| Fig. 1 Chemical structures of the molecules investigated in the present study for their effect on the photodegradation of avobenzone. (a) Glutathione of cytosol, (b) synthetic analogous peptides of glutathione, and (c) β-mercaptoethanol. | |
Glutathione is widely used in the cosmetic field as a skin whitening or skin lightening agent.37,38 Interestingly, it is associated with the modulation of skin complexation and is indeed present in the skin. It has been well documented in the literature that glutathione protects the cellular system from oxidative damage by directly reacting with reactive oxygen species (ROS), such as hydroxyl radicals, hydrogen peroxide and nitroso radicals.39–41 The feature of radical scavenging activity of glutathione and the radical-mediated degradation of avobenzone have prompted us to assess the ability of glutathione to protect avobenzone from sunlight-induced photodegradation. The present report evaluates the photostabilizing activity of glutathione towards avobenzone in ethanol/acetonitrile under glass-filtered sunlight. Synthetic mutant peptides of glutathione (Fig. 1b) and β-mercaptoethanol (Fig. 1c) were also evaluated to confirm the involvement of the sulfur atom of glutathione in the photostabilization activity.
Results and discussion
Sunlight induced degradation of avobenzone
The UV spectrum of avobenzone shows a predominant peak (λmax) at 356 nm. The absorbance value corresponding to the λmax has been widely used to estimate the extent of photodegradation of avobenzone.13,42,43 The present study also adopted a similar approach and used UV spectrophotometry as a tool to measure the extent of photodegradation of avobenzone under glass-filtered sunlight. Fig. 2a shows the UV spectra of avobenzone in ethanol drawn at regular intervals of time from a solution constantly exposed to glass-filtered sunlight. It is apparent from Fig. 2a that the degradation of avobenzone increases with the increase in time of exposure to glass-filtered sunlight. Higher the exposure to glass-filtered sunlight, higher will be the extent of degradation. Similar results were obtained with the polar aprotic solvent, acetonitrile (Fig. 2b). Approximately 50% of photodegradation of avobenzone (Fig. 2c) was observed in ethanol as well as in acetonitrile upon 5 h of exposure to glass-filtered sunlight. However, complete photodegradation of avobenzone was observed during the peak summer season at Central University of Karnataka (Fig. S1a and S1b†). Even though avobenzone degraded completely in ethanol (Fig. S1a†), ∼15% of avobenzone still remained non-degraded in the acetonitrile solvent system (Fig. S1b†) under the same conditions of glass-filtered sunlight. These observations confirm the role of proticity of the solvent on the extent of photodegradation of avobenzone. This result was consistent with a previous report on the effect of polarity and proticity of the solvent on avobenzone degradation using a high pressure-mercury lamp as the UV source.42 These results confirm that the photoprotection activity of glutathione on avobenzone can be assessed in the presence of glass-filtered sunlight.
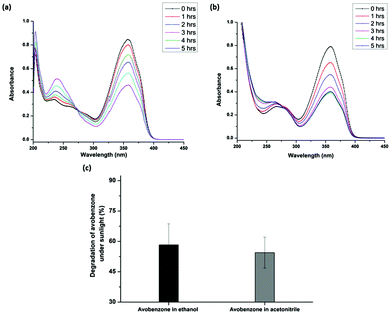 |
| Fig. 2 Glass-filtered sunlight-induced photodegradation of avobenzone. (a) UV spectra of avobenzone in ethanol drawn at regular intervals of time from a solution constantly exposed to the glass-filtered sunlight. (b) UV spectra of avobenzone in acetonitrile drawn at regular intervals of time from a solution constantly exposed to the glass-filtered sunlight. (c) Extent of photodegradation of avobenzone in polar protic/aprotic solvents under glass-filtered sunlight. Error bars represent five independent experiments on different days of glass-filtered sunlight. | |
Solar and UV radiation measurements
The dose of solar and ultraviolet (UV) radiation reaching the test center/test solution was measured using solar and UV power meters, respectively, as described in the Experimental section. The solar radiation predominantly contains UV, visible, and infrared (IR) radiation.44–46 Visible and IR radiation constitute a major portion of the solar spectrum. The ozone layer present in the stratosphere allows only UVA (320–400 nm) and UVB (285–320 nm) to reach the earth surface. Hence, the intensity of UV radiation was recorded from 290–390 nm. In order to estimate the actual solar/UV radiations reaching the test solution, the intensity of solar/UV radiation was measured by the transmission of sunlight through samples of the experimental glassware. The glass plate was found to be opaque to radiation <325 nm, and the conical flask glass was opaque to radiation <308 nm, indicating that the glass filtration predominantly produced a UVA source.
Table 1 shows the average intensity of solar and UV radiation at selected time points that caused ≥50% photodegradation of avobenzone upon constant exposure to sunlight for 6 h. Table 1 also shows the corresponding altered intensity of solar and UV radiation in the test solution when passed through the glass plate and the conical flask glass. The following inference can be drawn from the data in Table 1: (i) ∼85% and ∼94% solar radiation reached the test solution through the glass plate and the conical flask, respectively; (ii) ∼ 62% and ∼88% UV radiation reached the test solution through the glass plate and the conical flask, respectively; (iii) ∼3.2% UV radiation was present in the sunlight reaching the test center; and (iv) difference in the intensity of UV radiation before and after passing through the conical flask (≤308 nm) was ∼12%, which corresponded to UVB radiation in the sunlight. The intensity of solar and UV radiation mentioned in Table 1 correspond to the conditions of glass-filtered sunlight (hereafter, sunlight indicates glass-filtered sunlight) used to assess the effect of the additives on the photodegradation of avobenzone.
Table 1 Average intensity of solar and UV radiations at selected time points which caused ≥50% photodegradation of avobenzone over 6 h of constant exposure to sunlight
Time point |
Solar radiation (W m−2) |
UV radiation (W m−2) |
Normal |
Glass filter |
Normal |
Glass filter |
Glass plate |
Conical glass |
Glass plate |
Conical glass |
Note: Average values are from four independent readings on different days of sunlight. |
10:00 AM–12:00 PM |
1141 |
976 |
1082 |
38.23 |
23.91 |
34.13 |
12:00 PM–2:00 PM |
1222 |
1050 |
1161 |
41.84 |
25.46 |
36.67 |
2:00 PM–4:00 PM |
964 |
824 |
909 |
27.20 |
16.98 |
24.37 |
Assessing the photoprotection activity of glutathione on avobenzone under sunlight
To assess the photoprotection activity of glutathione on avobenzone, 1
:
2 ratio and various molar ratios (as seen in Fig. 4d) of avobenzone and glutathione in ethanol/acetonitrile were exposed to sunlight. Avobenzone alone was also exposed to sunlight under identical conditions to serve as the control. The extent of photodegradation of avobenzone in the presence of glutathione was monitored by recording the UV spectra of the avobenzone and glutathione mixture drawn at regular intervals of time from a solution constantly exposed to sunlight. It should be noted that the assessment of the photoprotection activity of glutathione on avobenzone was considered only if the degradation of the control avobenzone alone was >25% under identical sunlight. Fig. 3 shows the UV spectra of avobenzone and the extent of its degradation in the absence as well as in the presence of glutathione under identical conditions of sunlight. Interestingly, a significant time-dependent attenuation of the photodegradation of avobenzone was observed in the presence of glutathione. The prominent degradation of avobenzone in the absence of the additive (Fig. 3a) and the near non-degradation in the presence of glutathione (Fig. 3b) under identical conditions of sunlight confirm that glutathione protects avobenzone from photodegradation. Fig. 3c shows the percentage of degradation of avobenzone in the absence as well as in the presence of glutathione in ethanol/acetonitrile. It is evident from Fig. 3c that glutathione acts as a photostabilizer of avobenzone under sunlight. The extent of photodegradation of avobenzone in the presence of glutathione was also investigated during the peak summer season at Central University of Karnataka (Fig. S1c and S1d†). The complete photoprotection of avobenzone was observed in the ethanol solvent system (Fig. S1c†), whereas in acetonitrile, a slight initial degradation of avobenzone occurred before its photoprotection by glutathione (Fig. S1d†).
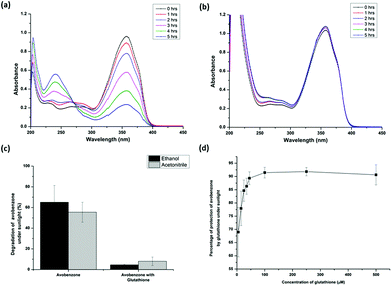 |
| Fig. 3 Assessing the ability of glutathione to protect avobenzone from the sunlight induced photodegradation in ethanol/acetonitrile. UV spectra of (a) avobenzone alone and (b) avobenzone in the presence of glutathione at different intervals of time under identical conditions of sunlight. (c) Extent of photodegradation of avobenzone in the presence of glutathione in ethanol/acetonitrile. (d) Dependence of the photoprotection of avobenzone on the concentration of glutathione. Bar graph represents the results of five/three independent experiments conducted on different days of sunlight. | |
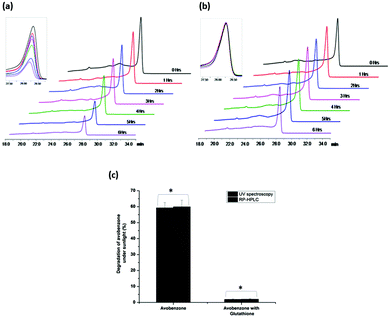 |
| Fig. 4 Comparison of photodegradation of avobenzone using UV spectroscopy and RP-HPLC. (a) RP-HPLC elution profiles of avobenzone alone and (b) RP-HPLC elution profiles of avobenzone with glutathione at different intervals of time upon exposure to identical conditions of sunlight. Inset shows the overlapping of the corresponding elution profiles. (c) Extent of photodegradation of avobenzone alone and avobenzone with glutathione as per UV spectroscopy and RP-HPLC methods. Asterisk indicates the P < 0.05 by two sample t-test between percents derived from statistic calculator (StatPac, Inc.). | |
To identify the minimal concentration of glutathione required for exhibiting the photoprotection activity on avobenzone, the sunlight-induced degradation of avobenzone was monitored in the presence of varied concentrations of glutathione in ethanol. The percentage of the protection offered by glutathione was calculated, as shown in the Experimental section. Fig. 3d shows the dependence of the extent of protection of avobenzone on the concentration of glutathione. As the concentration of glutathione increases, the photoprotection of avobenzone by glutathione also increases gradually. At the 0.2
:
1 ratio of glutathione and avobenzone, 69% photoprotection was conferred by glutathione. As the concentration of glutathione increases, the extent of photoprotection also increases (Fig. 3d) and the maximum protection of 90% is observed at the 2
:
1 ratio of glutathione and avobenzone. A similar extent of photoprotection of avobenzone was also observed with the further increase in the concentration of glutathione.
Comparison of percentage degradation of avobenzone using UV spectroscopy and RP-HPLC
To further authenticate the UV-based estimation of photodegradation of avobenzone, RP-HPLC was employed to analyse the samples drawn at regular intervals of time from the avobenzone solutions constantly exposed to sunlight. Avobenzone alone and avobenzone with glutathione in ethanol, upon exposure to sunlight, were subjected to UV spectroscopy, followed by RP-HPLC, as described in the Experimental section. Fig. 4 shows the RP-HPLC elution profiles of avobenzone alone and avobenzone with glutathione drawn at regular intervals of time from a solution exposed to identical conditions of sunlight. The photodegradation of avobenzone was evident from the RP-HPLC elution profile (Fig. 4a). Similarly, the photoprotection of avobenzone by glutathione was also evident from the RP-HPLC elution profiles (Fig. 4b). Table 2 shows the estimated concentrations of avobenzone using the UV spectra and the RP-HPLC elution profiles at given time intervals of exposure of solutions to sunlight. The deduced concentrations of avobenzone in the samples exposed to sunlight were similar according to both UV spectroscopy and RP-HPLC methods. Fig. 4c shows the percentage degradation of avobenzone upon exposure to sunlight for avobenzone alone and avobenzone with glutathione, deduced using both UV spectroscopy and RP-HPLC methods. No significant differences in the estimation of concentrations of avobenzone were observed between two methods according to the statistical analysis of data derived from three independent experiments conducted on different days. These results further confirm glutathione as a photostabilizer of avobenzone.
Table 2 Comparison of estimated concentrations of avobenzone by UV spectroscopy and RP-HPLC at regular time intervals during the constant exposure to sunlight
Time (Hours) |
Avobenzone alone |
Avobenzone with glutathione |
Concentration (μM) |
% Degradation |
Concentration (μM) |
% Degradation |
UV |
RP-HPLC |
UV |
RP-HPLC |
UV |
RP-HPLC |
UV |
RP-HPLC |
0 |
36.18 |
— |
0.00 |
— |
35.29 |
— |
0.00 |
— |
1 |
31.47 |
31.02 |
13.02 |
14.26 |
33.82 |
33.74 |
4.16 |
4.39 |
2 |
29.70 |
29.42 |
17.91 |
18.68 |
33.53 |
33.22 |
4.99 |
5.86 |
3 |
26.18 |
25.64 |
27.64 |
29.13 |
34.12 |
33.86 |
3.31 |
4.05 |
4 |
21.76 |
22.12 |
39.86 |
38.86 |
34.12 |
33.82 |
3.31 |
4.16 |
5 |
13.53 |
13.34 |
62.60 |
63.13 |
34.41 |
34.14 |
2.50 |
3.26 |
6 |
12.65 |
11.98 |
65.03 |
66.89 |
34.70 |
34.54 |
1.67 |
2.12 |
Characterization of synthetic mutglutathione, isoglutathione and (Ala)3-tripeptide
Analogous peptides of glutathione were synthesized to assess the role of thiol and iso-peptide bonds on the photoprotection activity of glutathione. Table 3 shows the list of synthetic peptides. Fig. 5a shows the ESI-MS/MS data of mutglutathione. The observed y2 ion at m/z 163 and the concomitant loss of 18 Da to yield the [y2-H2O] ion correspond to the Ser-Gly dipeptide fragment. The difference between the mass of the molecular ion peak and the mass of the y2 ion is 129 Da, which corresponds to the residual mass of glutamic acid. These observations were further supported by the presence of the b2 ion at m/z 217. These results are consistent with mutglutathione having the sequence Gluγ-Ser-Gly. Fig. 5b shows the ESI-MS/MS data of isoglutathione. The observed mass at m/z 233, 215, and 187 correspond to the b2 ion, [b2-H2O], and [b2-H2O-CO], respectively. These fragments confirm the presence of Glu-Cys dipeptide fragments in isoglutathione. The presence of b3 ion at m/z 290 and [b3-H2O] at m/z 272 confirm the sequence of isoglutathione as Gluα-Cys-Gly. Fig. S2† shows the ESI-MS/MS data of glutathione. Distinct differences in the ESI fragmentation patterns of isoglutathione and glutathione further authenticate the sequence of isoglutathione. Fig. 5c shows the ESI-MS/MS data of (Ala)3-tripeptide. The observed b2 ion at m/z 143 and the concomitant loss of 28 Da to yield the [b2-CO] ion correspond to the alanine dipeptide fragment. The difference between the mass of the molecular ion peak and the mass of the b2 ion is 89 Da. This corresponds to the residual mass of alanine and the mass of a water molecule. These observed fragment ions are consistent with (Ala)3-tripeptide, confirming the sequence of synthetic (Ala)3-tripeptide. The synthetic peptides helped to draw the following inferences. (1) Glutathione and mutglutathione differ by the mutation of cysteine to serine residue, i.e., a switch from sulfur to oxygen atom. Thus, mutglutathione helps to dissect the role of the sulfur atom of glutathione on the photoprotection activity. (2) Glutathione and isoglutathione differed by the shift in the Gluγ-Cys amide bond to the Gluα-Cys amide bond. isoGlutathione helps to reveal the role of the unique feature of the γ-peptide bond on the photoprotection activity of glutathione on avobenzone. (3) (Ala)3-tripeptide and glutathione share similar chain lengths; therefore, it may be used as a control to assess the photoprotection activity of analogous peptides of glutathione.
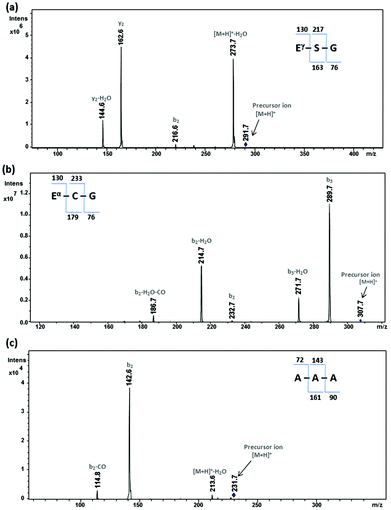 |
| Fig. 5 ESI-MS/MS data of the synthetic analogous peptides of glutathione. (a) mutGlutathione, (b) isoglutathione and (c) (Ala)3-tripeptide. Inset shows the expected fragment ions. Observed fragment ions are indicated. | |
Table 3 Characterization of analogous synthetic peptides of glutathione
Sl. no. |
Peptide name |
Sequence |
Calculated mass [M + H]+ (Da) |
Observed mass [M + H]+ (Da) |
1 |
mutGlutathione |
Gluγ-Ser-Gly |
292.1 |
291.7 |
2 |
isoGlutathione |
Gluα-Cys-Gly |
308.0 |
307.7 |
3 |
(Ala)3-tripeptide |
Ala-Ala-Ala |
232.1 |
231.7 |
Assessing the photoprotection activity of analogous peptides of glutathione on avobenzone
Fig. 6 shows the sunlight-induced degradation of avobenzone in the presence of synthetic peptides under identical conditions of sunlight. As revealed from Fig. 6b and c, attenuation of the photodegradation of avobenzone is less in the presence of mutglutathione compared with glutathione under identical conditions of sunlight. This observation confirms the involvement of the sulfur atom of glutathione on its photoprotection activity. Interestingly, a similar extent of photoprotection of avobenzone was observed in the cases of both glutathione (Fig. 6c) and isoglutathione (Fig. 6d), indicating that the γ-peptidic nature of glutathione may not have an influence on the photoprotection activity of glutathione. Surprisingly, ∼20% more avobenzone degradation was observed in the presence of (Ala)3-tripeptide (Fig. 6e). This anomaly may be ascribed to the hydrophobicity of the peptide. Vallejo et al. reported that ∼90% degradation of avobenzone was observed in hexane, while only 20% degradation was observed in ethanol/acetonitrile.43 These observations confirm that the hydrophobicity of the medium including both solvent and substrate may play a vital role on the extent of radiation-induced degradation of avobenzone.
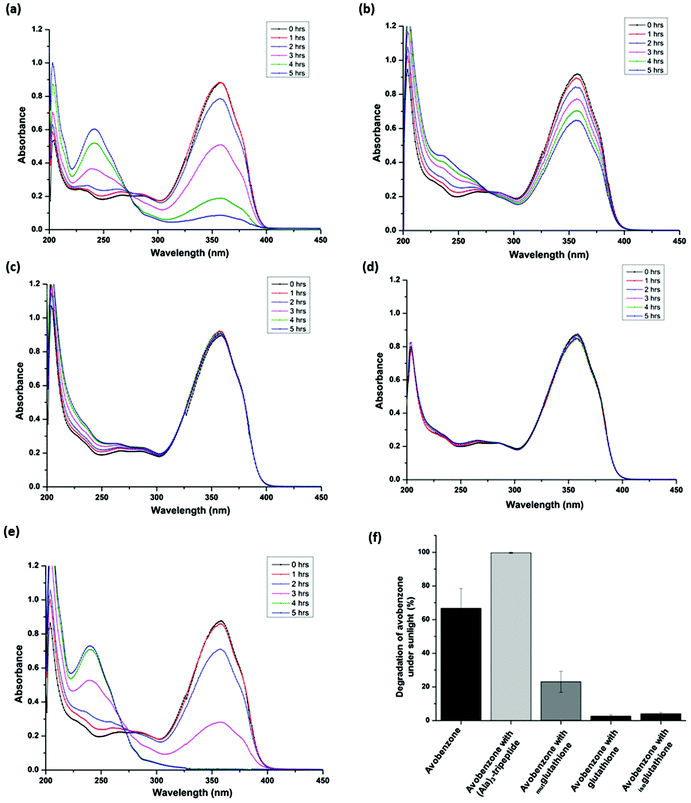 |
| Fig. 6 Assessing the photoprotection activity of glutathione and its analogous peptides on avobenzone under identical conditions of sunlight. UV spectra of (a) avobenzone alone, (b) avobenzone with mutglutathione, (c) avobenzone with glutathione, (d) avobenzone with isoglutathione and (e) avobenzone with (Ala)3-tripeptide at regular intervals of time. (f) Extent of photodegradation of avobenzone in the presence of various peptide additives. Error bars represent data of three independent experiments conducted on different days of sunlight. | |
Assessing the photoprotection activity of β-mercaptoethanol on avobenzone
To further support the involvement of the thiol group of glutathione in its photoprotection activity on avobenzone, the sunlight-induced degradation of avobenzone was monitored in the presence of β-mercaptoethanol. The structural difference between the solvent ethanol and β-mercaptoethanol is the thiol group. Fig. 7 shows the UV spectra of avobenzone and the extent of its degradation in the presence of β-mercaptoethanol in ethanol. It is evident from Fig. 7 that fair attenuation of photodegradation of avobenzone is observed in the presence of β-mercaptoethanol.
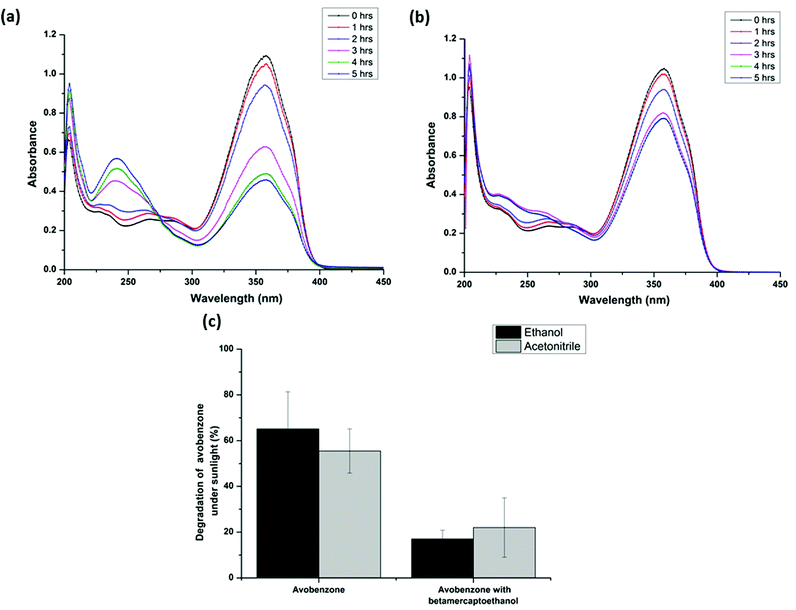 |
| Fig. 7 Assessing the ability of β-mercaptoethanol to protect avobenzone from the sunlight-induced degradation under identical conditions of sunlight. UV spectra of (a) avobenzone and (b) avobenzone in the presence of β-mercaptoethanol in ethanol at regular intervals of time. (c) Extent of photodegradation of avobenzone in the presence of β-mercaptoethanol in ethanol/acetonitrile. Error bars represent data of three independent experiments conducted on different days of sunlight. | |
Similar results were also observed in acetonitrile (Fig. S3†). These observations further support the involvement of the thiol group in the photoprotection activity on avobenzone. It should be noted that the extent of attenuation of photodegradation of avobenzone was more in the presence of glutathione than β-mercaptoethanol. Mturi and Martincigh have reported that polarity and proticity of the medium have a direct influence on the extent of photodegradation of avobenzone.42 Thus, apart from the thiol group, the backbone skeleton of glutathione may also influence the photoprotection activity.
Conclusions
Avobenzone is one of the most commonly used UVA filters in sunscreen lotions. An important drawback of avobenzone is its photo-instability, i.e., it undergoes degradation in the presence of radiations/sunlight. Various stabilizers, including antioxidants, were evaluated to impart stability to avobenzone against photodegradation. The present report has demonstrated the ability of glutathione to protect avobenzone from sunlight-induced photodegradation. Analysis of avobenzone photoprotection in the presence of glutathione using both UV spectrophotometry and RP-HPLC has clearly established glutathione as a photo-stabilizer of avobenzone. Equimolar concentrations of glutathione and avobenzone significantly attenuated the photodegradation of avobenzone under sunlight. Analogous peptides of glutathione were chemically synthesized and assessed for photoprotection activity. These results clearly indicated that the sulfur atom of glutathione plays a vital role in exhibiting the photoprotection activity. The dual role of glutathione as a skin whitening agent and a photo-stabilizer of avobenzone may find applications in the design of multi-purpose sunscreen lotion. The peptidic nature of glutathione has an advantage in developing novel cosmetic peptides to protect skin from harmful radiations.
Experimental
Materials
Avobenzone was obtained from Tokyo Chemical Industry (TCI), China. Ethanol was obtained from Changshu Hongsheng Fine Chemical Co., Ltd (CS), China. Acetonitrile was obtained from Merck, India. Glutathione was obtained from HiMedia, India. Trifluoroacetic acid was obtained from S.D. Fine Chemicals, India. Fmoc-Gly-OH and Fmoc-Cys(Trt)-OH were obtained from GL Biochem, USA. Fmoc-Glu(OH)-OtBu was obtained from Avra, India. Chromatographic grade water was obtained from Merck, India. Strata C18-columns were purchased from Phenomenex, India.
Measurement of intensity of solar and UV radiations of sunlight
Irradiance of solar radiation was measured using a Tenmars TM-207 solar power meter, which covers ultraviolet, visible, and infrared radiations of sunlight. The intensity of the UV radiation in sunlight was measured using a Leutron UV-340A light metre, which registers UV radiation within the spectral range of 290–390 nm. The photodegradation of avobenzone was also conducted in parallel during the measurements of intensity of solar and UV radiations. The directions of the meters were regularly changed in accordance to the direction of the sun during the course of exposure. Radiation intensity was also recorded through the glassware used in the experiments, namely, glass plate and piece of conical flask. Averaged radiation intensity corresponds to that obtained on four different sunny days.
Glass-filtered sunlight irradiation
Initially, 25 μM avobenzone, 50 μM glutathione, 50 μM β-mercaptoethanol, and 50 μM of peptide were prepared in 40 mL of ethanol/acetonitrile. Various concentrations of glutathione were prepared from a 1 mM stock solution by serial dilution. Note: 200 μL solution of glutathione/peptide in water was suspended in a total 40 mL of ethanol or acetonitrile, which yielded a homogeneous solution. The samples were taken in 250 mL conical flasks and covered with glass plates to avoid solvent evaporation during the exposure to glass-filtered sunlight. The samples were exposed to the peak period of 10 AM–4 PM for glass-filtered sunlight at Central University of Karnataka, Kalaburagi. The samples were occasionally stirred during the course of exposure to sunlight. The samples were drawn to record the UV spectra and the RP-HPLC elution profiles at regular intervals of time from solution constantly exposed to the sunlight. Since UV content in the sunlight varies, avobenzone alone was used as a control in all the experiments. The judgment on the photodegradation/photoprotection of avobenzone in the presence of an additive was considered only if the degradation of avobenzone alone was >25%.
UV spectroscopy
The spectra of the solutions were recorded using a PerkinElmer Lambda 25 double beam UV-visible spectrophotometer. The spectra were recorded over the wavelength range of 200–500 nm. The measurements were made immediately after the samples were withdrawn from sunlight irradiation at regular intervals during the 0–5 h time period. The absorbance intensity at 356 nm was used as a read-out to estimate the extent of photodegradation of avobenzone under sunlight. The percentage of photodegradation of avobenzone was calculated as follows:
where CI is the concentration of avobenzone before the irradiation. CF is the concentration of avobenzone after irradiation.
The percentage of photoprotection on avobenzone by glutathione was calculated as follows:
where
PA is the percentage degradation of avobenzone alone.
PB is the percentage degradation of avobenzone in the presence of glutathione under identical conditions of sunlight.
The error bars were derived from the independent experiments conducted during different days of sunlight. The statistical analysis of the data was performed on the Origin Pro 8.5 graphical platform.
Reverse phase-high performance liquid chromatography
The samples, namely, avobenzone alone and avobenzone with glutathione in ethanol, drawn at regular intervals of time were quantified using both UV spectroscopy and RP-HPLC. RP-HPLC was performed using an UFLC Shimadzu HPLC system equipped with binary LC-20AD pumps, an SIL-20AC autosampler and an SPD-20A detector. In brief, 500 μL of a sample was injected using the autosampler, eluted from a Luna phenomenix C-18 column (250 mm × 4.6 mm, 5 μm), and monitored at 356 nm. The linear gradient of acetonitrile containing 0.1% TFA was used as the mobile phase: 0 min-0%; 5 min-0%; 10 min-65%; 40 min-100%. Area under the curve was estimated using the LabSolutions software provided by the manufacturer. With respect to the area under the curve of a known concentration of avobenzone, the concentration of avobenzone in test solutions was estimated. Three independent experiments on different days of sunlight were conducted (six hours of exposure to identical sunlight) and quantified by performing both UV spectroscopy and RP-HPLC. The statistical difference between the percentages of degradation of avobenzone estimated by UV spectroscopy and RP-HPLC was analysed using the Stat. Pac Software.
Solid phase peptide synthesis
mutGlutathione, isoglutathione and (Ala)3-tripeptide were synthesized manually by the standard solid phase peptide synthesis methodology using Fmoc-(9-fluorenylmethyloxycarbonyl) chemistry and the activation of the C-terminus by HBTU. After the synthesis, the peptides were cleaved from the resin using reagent K and precipitated using ether. The precipitated peptides were washed thoroughly multiple times with cold ether. The peptide was dissolved and purified using a phenomenex solid phase extraction cartridge using the protocol provided by the manufacturer. The purified peptides were dried using a vacuum concentrator and subjected to mass spectrometry. The dried purified peptides were used to assess the photoprotection activity.
Conflicts of interest
There are no conflicts to declare.
Acknowledgements
This article is dedicated to Prof. S. S. Murthy and Prof. M. N. Sudheendra Rao for their contribution to the Department of Chemistry, Central University of Karnataka, Kalaburagi. PChVG is supported by the award of a Senior Research Fellowship (SRF) from the University Grant Commission (UGC), India. B. H. acknowledges DST-SERB-NPDF grant. V. D. acknowledges IAS-INSA-NIAS summer fellowship. We acknowledge the help of Ms. Priyanka Nelogi. This research work is supported by the DST-INSPIRE faculty research grant. We acknowledge proteomic facility, MBU, IISc, Bengalure.
Notes and references
- H. S. Black, F. R. deGruijl, P. D. Forbes, J. E. Cleaver, H. N. Ananthaswamy, E. C. deFabo, S. E. Ullrich and R. M. Tyrrell, Photocarcinogenesis: An overview, J. Photochem. Photobiol., B, 1997, 40, 29–47 CrossRef CAS.
- C. A. Elmets and C. Y. Anderson, Sunscreens and photocarcinogenesis: An objective assessment, Photochem. Photobiol., 1996, 63, 435–440 CrossRef CAS PubMed.
- M. Ichihashi, M. Ueda, A. Budiyanto, T. Bito, M. Oka, M. Fukunaga, K. Tsuru and T. Horikawa, UV-induced skin damage, Toxicology, 2003, 189, 21–39 CrossRef CAS PubMed.
- K. Scharffetter-Kochanek, M. Wlaschek, P. Brenneisen, M. Schauen, R. Blaudschun and J. Wenk, UV-induced reactive oxygen species in photocarcinogenesis and photoaging, Biol. Chem., 1997, 378, 1247–1257 CAS.
- A. Fourtanier, D. Moyal and S. Seite, Sunscreens containing the broad-spectrum UVA absorber, Mexoryl SX, prevent the cutaneous detrimental effects of UV exposure: A review of clinical study results, Photodermatol., Photoimmunol. Photomed., 2008, 24, 164–174 CrossRef CAS PubMed.
- J. B. Mancuso, R. Maruthi, S. Q. Wang and H. W. Lim, Sunscreens: An Update, Am. J. Clin. Dermatol., 2017, 18, 643–650 CrossRef PubMed.
- J. F. Nash, P. R. Tanner and P. J. Matts, Ultraviolet A radiation: Testing and labeling for sunscreen products, Dermatol. Clin., 2006, 24, 63–74 CrossRef CAS PubMed.
- A. R. Young, J. Claveau and A. B. Rossi, Ultraviolet radiation and the skin: Photobiology and sunscreen photoprotection, J. Am. Acad. Dermatol., 2017, 76, S100–S109 CrossRef CAS PubMed.
- A. Fourtanier, F. Bernerd, C. Bouillon, L. Marrot, D. Moyal and S. Seite, Protection of skin biological targets by different types of sunscreens, Photodermatol., Photoimmunol. Photomed., 2006, 22, 22–32 CrossRef CAS PubMed.
- C. Tuchinda, H. W. Lim, U. Osterwalder and A. Rougier, Novel emerging sunscreen technologies, Dermatol. Clin., 2006, 24, 105–117 CrossRef CAS PubMed.
- P. Kullavanijaya and H. W. Lim, Photoprotection, J. Am. Acad. Dermatol., 2005, 52, 937–958 CrossRef PubMed.
- R. Rai, S. C. Shanmuga and C. Srinivas, Update on photoprotection, Indian J. Dermatol., 2012, 57, 335–342 CrossRef PubMed.
- S. Afonso, K. Horita, J. P. Sousa e Silva, I. F. Almeida, M. H. Amaral, P. A. Lobão, P. C. Costa, M. S. Miranda, J. C. G. Esteves da Silva and J. M. Sousa Lobo, Photodegradation of avobenzone: Stabilization effect of antioxidants, J. Photochem. Photobiol., B, 2014, 140, 36–40 CrossRef CAS PubMed.
- H. Gonzalez, N. Tarras-Wahlberg, B. Stromdahl, A. Juzeniene, J. Moan, O. Larko, A. Rosen and A. M. Wennberg, Photostability of commercial sunscreens upon sun exposure and irradiation by ultraviolet lamps, BMC Dermatol., 2007, 7, 1 CrossRef PubMed.
- A. Cantrell and D. J. McGarvey, Photochemical studies of 4-tert-butyl-4′-methoxydibenzoylmethane (BM-DBM), J. Photochem. Photobiol., B, 2001, 64, 117–122 CrossRef CAS.
- S. P. Huong, E. Rocher, J.-D. Fourneron, L. Charles, V. Monnier, H. Bun and V. Andrieu, Photoreactivity of the sunscreen butylmethoxydibenzoylmethane (DBM) under various experimental conditions, J. Photochem. Photobiol., A, 2008, 196, 106–112 CrossRef CAS.
- E. Damiani, P. Astolfi, J. Giesinger, T. Ehlis, B. Herzog, L. Greci and W. Baschong, Assessment of the photo-degradation of UV-filters and radical-induced peroxidation in cosmetic sunscreen formulations, Free Radical Res., 2010, 44, 304–312 CrossRef CAS PubMed.
- K. M. Hanson, E. Gratton and C. J. Bardeen, Sunscreen enhancement of UV-induced reactive oxygen species in the skin, Free Radicals Biol. Med., 2006, 41, 1205–1212 CrossRef CAS PubMed.
- I. Karlsson, L. Hillerstrom, A. L. Stenfeldt, J. Martensson and A. Borje, Photodegradation of dibenzoylmethanes: Potential cause of photocontact allergy to sunscreens, Chem. Res. Toxicol., 2009, 22, 1881–1892 Search PubMed.
- M. Avenel-Audran, H. Dutartre, A. Goossens, M. Jeanmougin, C. Comte, C. Bernier, L. Benkalfate, M. Michel, M. C. Ferrier-Lebouedec, M. Vigan, J. L. Bourrain, O. Outtas, J. L. Peyron and L. Martin, Octocrylene, an emerging photoallergen, Arch. Dermatol., 2010, 146, 753–757 Search PubMed.
- I. Karlsson, K. Vanden Broecke, J. Martensson, A. Goossens and A. Borje, Clinical and experimental studies of octocrylene's allergenic potency, Contact Dermatitis, 2011, 64, 343–352 CrossRef PubMed.
- V. Lhiaubet-Vallet, M. Marin, O. Jimenez, O. Gorchs, C. Trullas and M. A. Miranda, Filter-filter interactions. Photostabilization, triplet quenching and reactivity with singlet oxygen, Photochem. Photobiol. Sci., 2010, 9, 552–558 RSC.
- R. M. Sayre, J. C. Dowdy, A. J. Gerwig, W. J. Shields and R. V. Lloyd, Unexpected photolysis of the sunscreen octinoxate in the presence of the sunscreen avobenzone, Photochem. Photobiol., 2005, 81, 452–456 CrossRef CAS PubMed.
- S. Scalia and M. Mezzena, Photostabilization effect of quercetin on the UV filter combination, butyl methoxydibenzoylmethane-octylmethoxycinnamate, Photochem. Photobiol., 2010, 86, 273–278 CrossRef CAS PubMed.
- L. R. Gaspar and P. M. Maia Campos, Evaluation of the photostability of different UV filter combinations in a sunscreen, Int. J. Pharm., 2006, 307, 123–128 CrossRef CAS PubMed.
- P. Cecilia, L.-V. Virginie, J. Oscar, T. Carles and M. M. Ángel, A blocked diketo form of avobenzone: Photostability, photosensitizing properties and triplet quenching by a triazine-derived UVB-filter, Photochem. Photobiol., 2009, 85, 178–184 CrossRef PubMed.
- J. Y. Lin, M. A. Selim, C. R. Shea, J. M. Grichnik, M. M. Omar, N. A. Monteiro-Riviere and S. R. Pinnell, UV photoprotection by combination topical antioxidants vitamin C and vitamin E, J. Am. Acad. Dermatol., 2003, 48, 866–874 CrossRef PubMed.
- F. Afaq and H. Mukhtar, Photochemoprevention by botanical antioxidants, Skin Pharmacol. Appl. Skin Physiol., 2002, 15, 297–306 CrossRef CAS PubMed.
- R. K. Chaudhuri, Z. Lascu, G. Puccetti, A. A. Deshpande and S. K. Paknikar, Design of a photostabilizer having built-in antioxidant functionality and its utility in obtaining broad-spectrum sunscreen formulations, Photochem. Photobiol., 2006, 82, 823–828 CrossRef CAS PubMed.
- R. Exner, B. Wessner, N. Manhart and E. Roth, Therapeutic potential of glutathione, Wien. Klin. Wochenschr., 2000, 112, 610–616 CAS.
- D. M. Townsend, K. D. Tew and H. Tapiero, The importance of glutathione in human disease, Biomed. Pharmacother., 2003, 57, 145–155 CrossRef CAS.
- A. Meister and M. E. Anderson, Glutathione, Annu. Rev. Biochem., 1983, 52, 711–760 CrossRef CAS PubMed.
- C. Kerksick and D. Willoughby, The antioxidant role of glutathione and N-acetyl-cysteine supplements and exercise-induced oxidative stress, J. Int. Soc. Sports Nutr., 2005, 2, 38–44 CrossRef PubMed.
- J. Pizzorno, Glutathione!, Integrative Med. Clin. J., 2014, 13, 8–12 Search PubMed.
- B. Łubkowska, B. Grobelna and Z. Maćkiewicz, The use of synthetic polypeptides in cosmetics, Copernican Lett., 2010, 1, 75–82 CrossRef.
- S. Schagen, Topical peptide treatments with effective anti-aging results, Cosmetics, 2017, 4, 16 CrossRef.
- S. Sonthalia, D. Daulatabad and R. Sarkar, Glutathione as a skin whitening agent: Facts, myths, evidence and controversies, Indian J. Dermatol., Venereol., Leprol., 2016, 82, 262–272 CrossRef PubMed.
- C. D. Villarama and H. I. Maibach, Glutathione as a depigmenting agent: An overview, Int. J. Cosmet. Sci., 2005, 27, 147–153 CrossRef CAS PubMed.
- A. Galano and J. R. Alvarez-Idaboy, Glutathione: Mechanism and kinetics of its non-enzymatic defense action against free radicals, RSC Adv., 2011, 1, 1763–1771 RSC.
- F. Franzoni, R. Colognato, F. Galetta, I. Laurenza, M. Barsotti, R. Di Stefano, R. Bocchetti, F. Regoli, A. Carpi, A. Balbarini, L. Migliore and G. Santoro, An in vitro study on the free radical scavenging capacity of ergothioneine: comparison with reduced glutathione, uric acid and trolox, Biomed. Pharmacother., 2006, 60, 453–457 CrossRef CAS PubMed.
- C. A. Wakulich and B. L. Tepperman, Role of glutathione in nitric oxide-mediated injury to rat gastric mucosal cells, Eur. J. Pharmacol., 1997, 319, 333–341 CrossRef CAS PubMed.
- G. J. Mturi and B. S. Martincigh, Photostability of the sunscreening agent 4-tert-butyl-4′-methoxydibenzoylmethane (avobenzone) in solvents of different polarity and proticity, J. Photochem. Photobiol., A, 2008, 200, 410–420 CrossRef CAS.
- J. J. Vallejo, M. Mesa and C. Gallardo, Evaluation of the avobenzone photostability in solvents used in cosmetic formulations, Vitae, 2011, 18, 63–71 CAS.
- IARC Working Group on the Evaluation of Carcinogenic Risk to Humans. Radiation. International Agency for Research on Cancer, Lyon (FR), 2012. (IARC Monographs on the Evaluation of Carcinogenic Risks to Humans, No. 100D.) Solar and ultraviolet radiation.
- P. Balasaraswathy, U. Kumar, C. R. Srinivas and S. Nair, UVA and UVB in sunlight, Optimal Utilization of UV rays in Sunlight for phototherapy, Indian J. Dermatol., Venereol., Leprol., 2002, 68, 198–201 CAS.
- N. Sahba and T. J. Rockett, Infrared Absorption Coefficients of Silica Glasses, J. Am. Ceram. Soc., 1992, 75, 209–212 CrossRef CAS.
Footnotes |
† Electronic supplementary information (ESI) available. See DOI: 10.1039/c8pp00343b |
‡ These two authors are equally contributed to the work. |
|
This journal is © The Royal Society of Chemistry and Owner Societies 2019 |