DOI:
10.1039/C8NJ05577G
(Paper)
New J. Chem., 2019,
43, 130-134
Organocatalytic enantioselective functionalization of indoles in the carbocyclic ring with cyclic imines†
Received
2nd November 2018
, Accepted 13th November 2018
First published on 13th November 2018
Abstract
An organocatalytic enantioselective functionalization in the carbocyclic ring of indoles with benzoxathiazine 2,2-dioxides is described using a quinine-derived bifunctional organocatalyst. This aza-Friedel–Crafts reaction provides 4-indolyl, 5-indolyl and 7-indolyl sulfamidate derivatives in good yields (up to 99%) and with moderate to high enantioselectivities (up to 86% ee).
Introduction
The catalytic asymmetric Friedel–Crafts reaction1 of aromatic compounds with imines is one of the most important methodologies for the synthesis of enantiopure chiral benzylic amines, which are present in a wide range of pharmaceutical and natural products.2 In this context, the enantioselective addition of indoles to imines is one of the most studied asymmetric Friedel–Crafts reactions,3,4 due to the importance of the indole scaffold in natural product synthesis, medicinal and agrochemical industries and materials science.5 However, the majority of methods on the enantioselective aza-Friedel–Crafts reaction of indoles involve the functionalization of the C-3 position.4 Additionally, different examples of the asymmetric functionalization at the C-2 position of indoles with imines have been described.6 In contrast, the enantioselective functionalization in the carbocyclic ring of indoles is hardly studied in the literature and represents a great challenge in asymmetric catalysis (Fig. 1A).7 We have recently presented a methodology for the organocatalytic enantioselective functionalization of the carbocyclic ring of indoles using as an activating/directing group, a hydroxy group.8–10 Hydroxyindoles, in the presence of bifunctional organocatalysts, react as phenols, even when the positions in the azole ring remained unsubstituted. Given the remarkable significance of chiral indoles bearing a nitrogen in the α-position and hydroxyindoles (Fig. 1B), the development of new methodologies for the synthesis of chiral indolyl amines is the great interest for organic synthesis.
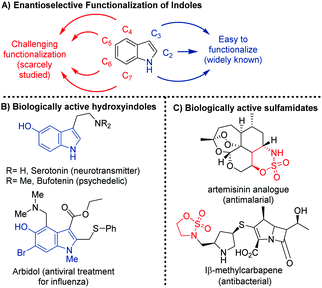 |
| Fig. 1 Enantioselective functionalization of indoles. Examples of biologically active hydroxyindoles and sulfamidates. | |
In this communication, to accomplish the enantioselective functionalization in the carbocyclic ring of indoles, we have chosen cyclic imines (benzoxathiazine 2,2-dioxides) as electrophiles. Very recently, benzoxathiazine 2,2-dioxides have attracted attention in asymmetric catalysis, because these compounds have been proved to be powerful building blocks for the synthesis of chiral benzosulfamidate heterocycles. In this context, several sulfamidates have shown important biological activities11 (Fig. 1C) and several examples of enantioselective reactions have been described using these cyclic imines as electrophiles.12 However, the number of enantioselective aza-Friedel–Crafts reactions using benzoxathiazine 2,2-dioxides is scarce.13,14 In 2017, Kim has described the organocatalytic enantioselective alkylation of indoles at the C-3 position with cyclic imines catalyzed by chiral Brønsted phosphoric acid (Scheme 1A).13a The corresponding 3-indolyl sulfamidate derivatives were obtained with excellent yields and enantioselectivities. Herein, we described a complementary methodology for obtaining chiral indolyl sulfamidates (Scheme 1B). By using a quinine-derived bifunctional organocatalyst, we achieve the functionalization of the carbocyclic ring of hydroxyindoles with cyclic imines, obtaining 4-indolyl, 5-indolyl and 7-indolyl sulfamidate derivatives (Scheme 1B).
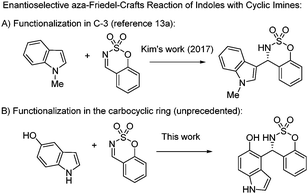 |
| Scheme 1 Enantioselective aza-Friedel–Crafts reaction of indoles with cyclic imines. | |
Results and discussion
We chose the aza-Friedel–Crafts reaction between benzoxathiazine 2,2-dioxides (1a) and 4-hydroxyindole (2) for the optimization studies. Different bifunctional organocatalysts derived from Cinchona alkaloids (Fig. 2) in CH2Cl2 at room temperature were screened (Table 1). When quinine (I) was tried as a catalyst, the regioselectivity was poor and we observed 3 compounds after 21 h of reaction (Table 1, entry 1). The major product was the corresponding hydroxyindole alkylated at the C-5 position (3a) in 48% yield but was a racemic mixture. Then, we isolated as an inseparable mixture after column chromatography, the product alkylated at the C-7 position (4a) and the double alkylated product at C-5 and C-7 positions (5a, a mixture of diasteroisomers in 1
:
1 ratio determined by 1H NMR). Different bifunctional organocatalysts such cupreines, thioureas and squaramides were tested,15 but we found that 9-O-benzylcupreine (II), derived from quinine, was the only one with a promising enantioselectivity (33% ee, entry 2). However, product 5a was still obtained with high quantity (28%). We tried several cupreine derivatives with a variety of substituents on the secondary hydroxyl group. The best catalyst in terms of enantioselectivity was catalyst XI, giving compound 3a in 48% yield, and 43% ee in 6 h (entry 11).
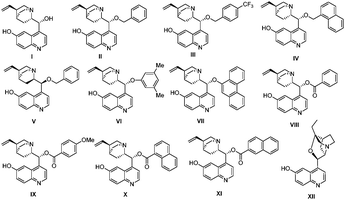 |
| Fig. 2 Bifunctional organocatalysts screened. | |
Table 1 Optimization of the reaction conditionsa
Next, we examined different solvents (Table 2), obtaining the best enantioselectivities with chlorinated solvents. In particular, when the reaction was run in 1,2-dichloroethane, compound 3a was obtained with 57% ee (entry 3, Table 2). Then, the effect of the reaction temperature was investigated. By lowering the reaction temperature to 4 or −20 °C, or increasing to 50 °C, the enantioselectivity was worse than that at room temperature. Afterward, different concentrations (entry 13 and 14) were tested without any improvement in the enantiomeric excess. Finally, different catalyst loadings were evaluated (entries 15–17), obtaining an enhancement of the enantiomeric excess to 67% ee and 46% yield, when 2 mol% of catalyst was used (entry 16). Our efforts to improve the enantiomeric excess of compound 3a were unsuccessful; therefore, we decided to study the scope and generality of the reaction under the conditions shown in entry 16, Table 2.
Table 2 Optimization of the reaction conditionsa
First we studied the effect of the substituents in the cyclic imines using 4-hydroxyindole as a nucleophile (Scheme 2). The presence of a strong electron-donating group (MeO) at the 6 position led to a nearly racemic mixture, while the presence of electron-withdrawing groups at the 6 position (Br) led to an improvement of the yield of product 3d to 89%16 maintaining the enantioselectivity (70% ee). Once that we studied the reaction with 4-hydroxyindole, we decided to apply our methodology for the functionalization of indoles in every position of the carbocyclic ring. So, we continued our research studying the reaction of 5-hydroxyindole (6) and differently substituted benzoxathiazine 2,2-dioxides. To our delight, the corresponding product 7a, regioselectively alkylated at the 4 position, was obtained with good yield (71%)17 and good enantiomeric excess (80% ee). The introduction of substituents in the aromatic ring of the cyclic imines revealed that both electron-donating and -withdrawing groups were well-tolerated at the 6 position on the ring (7b–7d, 89–98% yield and 84–85% ee). Moreover, cyclic imines (1e–1f) with two substituents that provide steric hindrance were suitable substrates for the aza-Friedel–Crafts reaction, affording good enantiomeric excess (86% ee) and good yields (99% and 73%). In addition, benzoxathiazine 2,2-dioxides bearing functional groups in the 8-position were also tolerated as substrates giving the reaction product 7g, with good yield (95%) and enantiomeric excess (81%). However, a naphthyl ring was not tolerated and the corresponding product was obtained with a moderate yield and enantioselectivity.18 Unfortunately, 6-hydroxyindole showed low reactivity under the optimized reaction conditions, the 7-alkylated product 9a was obtained with complete regioselectivity, but a moderate yield (51%) and low enantioselectivity (37% ee) after 3 days of reaction. Finally, 7-hydroxyindole was also tested under the optimized reaction conditions, but unfortunately the regioselectivity was low obtaining a ratio of 1
:
1 of alkylated products at C-6 and at C-4, and the enantiomeric excesses of these compounds were also very poor.15 We attribute these results to the interference between the NH of the indole group and the hydroxyl group.
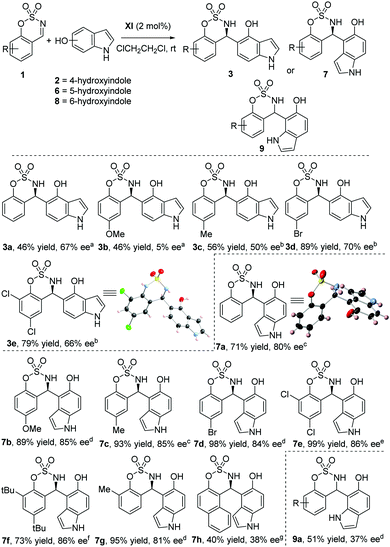 |
| Scheme 2 Scope of the enantioselective aza-Friedel–Crafts reaction of hydroxyindoles with cyclic imines. Reaction conditions: 1 (0.1 mmol), hydroxyindole (0.2 mmol) and XI (2 mol%) in 1 mL of ClCH2CH2Cl. Isolated yields after column chromatography. Determined by chiral HPLC. a 6 h reaction time. b 15 h reaction time. c 48 h reaction time. d 72 h reaction time. e 3 h reaction time. f 144 h reaction time. g 240 h reaction time. | |
The absolute configuration of compounds 3e and 7a was determined to be (R) by X-ray analysis19 (Scheme 2), and for the rest of the products 3 and 7 was assigned on the assumption of a uniform mechanistic pathway. The observed stereochemistry can be explained through a plausible transition state depicted in Scheme 3, where the catalyst activates both the nucleophile and the electrophile through hydrogen bonding. The hydrogen bonding between the quinuclidine tertiary amine and the hydroxyl group of indole can be ascertained due to the different reactivities of 5-methoxyindole (10). When 10 was used as a nucleophile under the optimized reaction conditions, the reaction took place at the C-3 position of the indole (the normal position for a Friedel–Crafts alkylation) and the corresponding product 11 was obtained with good yield (73%), but nearly racemic (6% ee).
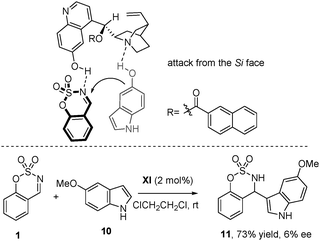 |
| Scheme 3 Elucidation of the bifunctional mode of activation. | |
Finally, we carried out the reduction of the sulfamidate moiety20 of compound 7a (Scheme 4), using LiAlH4 obtaining the corresponding chiral amine bearing phenol and hydroxyindole moieties, which was protected in situ as its Boc derivative 12, with good yield (59%) and preserving the enantiomeric excess of compound 7a (81% ee).
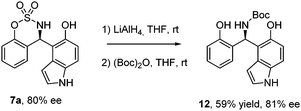 |
| Scheme 4 Synthetic transformation. | |
Conclusions
In summary, we have described an enantioselective functionalization in the carbocyclic ring of indoles through an aza-Friedel–Crafts alkylation reaction of hydroxyindoles with benzoxathiazine 2,2-dioxides as electrophiles using a quinine-derived bifunctional organocatalyst. The corresponding chiral sulfamidates were obtained with good yields and from moderate to good enantioselectivities. In general, the best yields and enantioselectivities were obtained when 5-hydroxyindole was used as a nucleophile, the reaction occurring at the C-4 position of the carbocyclic ring. This methodology represents the first Friedel–Crafts alkylation in the carbocyclic ring of indoles with cyclic imines.
Conflicts of interest
There are no conflicts to declare.
Acknowledgements
Financial support from the Agencia Estatal de Investigación (AEI, Spanish Government) and Fondo Europeo de Desarrollo Regional (FEDER, European Union) (CTQ2017-84900-P) is acknowledged. C. V. thanks the Spanish Government for the RyC contract (RYC-2016-20187). Access to NMR, MS and X-ray facilities from the Servei Central de Suport a la Investigació Experimental (SCSIE)-UV is also acknowledged.
Notes and references
-
(a)
Friedel–Crafts Chemistry, ed. G. A. Olah, Wiley, New York, 1973 Search PubMed;
(b)
Catalytic Asymmetric Friedel–Crafts Alkylations, ed. M. Bandini and A. Umani-Ronchi, Wiley-VCH, Weinheim, 2009 Search PubMed;
(c) T. B. Poulsen and K. A. Jorgensen, Chem. Rev., 2008, 108, 2903 CrossRef CAS PubMed;
(d) V. Terrasson, R. M. de Figueiredo and J. M. Campagne, Eur. J. Org. Chem., 2010, 2635 CrossRef CAS;
(e) M. Bandini and A. Eichholzer, Angew. Chem., Int. Ed., 2009, 48, 9608 CrossRef CAS PubMed;
(f) G. Bartoli, G. Bencivenni and R. Dalpozzo, Chem. Soc. Rev., 2010, 39, 4449 RSC;
(g) E. Marques-Lopez, A. Diez-Martinez, P. Merino and R. P. Herrera, Curr. Org. Chem., 2009, 13, 1585 CrossRef CAS;
(h) S.-L. You, Q. Cai and M. Zeng, Chem. Soc. Rev., 2009, 38, 2190 RSC;
(i) M. Montesinos-Magraner, C. Vila, G. Blay and J. R. Pedro, Synthesis, 2016, 2151 CAS.
-
Chiral Amine Synthesis: Methods, Developments and Applications, ed. T. C. Nugent, Wiley-VCH, Weinheim, 2010 Search PubMed.
- Y. C. Chen and Z. F. Xie, Chin. J. Org. Chem., 2012, 32, 462 CrossRef CAS.
- For selected examples of the asymmetric Friedel-Crafts reaction of indoles with imines, see:
(a) M. Hatano, T. Mochizuki, K. Nishikawa and K. Ishihara, ACS Catal., 2018, 8, 349 CrossRef CAS;
(b) Y.-Q. Wang, J. Song, R. Hong, H. Li and L. J. Deng, J. Am. Chem. Soc., 2006, 128, 8156 CrossRef CAS PubMed;
(c) Q. Kang, Z. A. Zhao and S.-L. You, J. Am. Chem. Soc., 2007, 129, 1484 CrossRef CAS PubMed;
(d) G. B. Rowland, E. B. Rowland, Y. Liang, J. A. Perman and J. C. Antilla, Org. Lett., 2007, 9, 2609 CrossRef CAS PubMed;
(e) G.-W. Zhang, L. Wang, J. Nie and J.-A. Ma, Adv. Synth. Catal., 2008, 350, 1457 CrossRef CAS;
(f) Y.-X. Jia, J.-H. Xie, H.-F. Duan, L.-X. Wang and Q.-L. Zhou, Org. Lett., 2006, 8, 1621 CrossRef CAS PubMed;
(g) T. Arai and J. Kakino, Angew. Chem., Int. Ed., 2016, 55, 15263 CrossRef CAS PubMed;
(h) K. Wu, Y.-J. Jiang, Y.-S. Fan, D. Sha and S. Zhang, Chem. – Eur. J., 2013, 19, 474 CrossRef CAS PubMed;
(i) F. Xu, D. Huang, C. Han, W. Shen, X. Lin and Y. Wang, J. Org. Chem., 2010, 5, 8677 CrossRef PubMed;
(j) Y. Qian, G. Ma, A. Lv, H.-L. Zhu, J. Zhao and V. H. Rawal, Chem. Commun., 2010, 46, 3004 RSC;
(k) Q. Kang, Z.-A. Zhao and S.-L. You, Tetrahedron, 2009, 65, 1603 CrossRef CAS;
(l) M. Johannsen, Chem. Commun., 1999, 2233 RSC;
(m) G.-W. Zhang, L. Wang, J. Nie and J.-A. Ma, Adv. Synth. Catal., 2008, 350, 1457 CrossRef CAS;
(n) R. Husmann, E. Sugiono, S. Mersmann, G. Raabe, M. Rueping and C. Bolm, Org. Lett., 2011, 13, 1044 CrossRef CAS PubMed;
(o) J. Feng, W. Yan, D. Wang, P. Li, Q. Sun and R. Wang, Chem. Commun., 2012, 48, 8003 RSC;
(p) P. Yu, J. He and C. Guo, Chem. Commun., 2008, 2355 RSC;
(q) L. Osorio-Planes, C. Rodríguez-Escrich and M. A. Pericàs, Chem. – Eur. J., 2014, 20, 2367 CrossRef CAS PubMed;
(r) L.-Y. Chen, H. He, W.-H. Chan and A. W. M. Lee, J. Org. Chem., 2011, 76, 7141 CrossRef CAS PubMed;
(s) Y. Wang, L. Jiang, L. Li, J. Dai, D. Xiong and Z. Shao, Angew. Chem., Int. Ed., 2016, 55, 15142 CrossRef CAS PubMed;
(t) X.-W. Wang, Y.-Z. Hua and M.-C. Wang, J. Org. Chem., 2016, 81, 9227 CrossRef CAS PubMed;
(u) X. Zhang, J. Zhang, L. Lin, H. Zheng, W. Wu, X. Liu and X. Feng, Adv. Synth. Catal., 2016, 358, 3021 CrossRef CAS.
-
(a)
R. J. Sundberg, Indoles, Academic Press, San Diego, 1996 Search PubMed;
(b)
S. M. Bronner, G. Y. J. Im and N. K. Garg, Heterocycles in Natural Product Synthesis, Wiley-VCH, Weinhem, 2011, p. 221 Search PubMed.
-
(a) Q. Kang, X. J. Zheng and S.-L. You, Chem. – Eur. J., 2008, 14, 3539 CrossRef CAS PubMed;
(b) B.-B. Huang, L. Wu, R.-R. Liu, L.-L. Xing, R.-X. Liang and Y.-X. Jia, Org. Chem. Front., 2018, 5, 929 RSC.
- For reviews in the non-enantioselective functionalization of indoles in the carbocyclic ring, see:
(a) A. H. Sandtorv, Adv. Synth. Catal., 2015, 357, 2403 CrossRef CAS;
(b) J. A. Leitch, Y. Bhonoah and C. G. Frost, ACS Catal., 2017, 7, 5618 CrossRef CAS.
-
(a) M. Montesinos-Magraner, C. Vila, A. Rendón-Patiño, G. Blay, I. Fernández, M. C. Muñoz and J. R. Pedro, ACS Catal., 2016, 6, 2689 CrossRef CAS;
(b) M. Montesinos-Magraner, C. Vila, G. Blay, I. Fernández, M. C. Muñoz and J. R. Pedro, Org. Lett., 2017, 19, 1546 CrossRef CAS PubMed;
(c) C. Vila, J. Rostoll-Berenguer, R. Sánchez-García, G. Blay, I. Fernández, M. C. Muñoz and J. R. Pedro, J. Org. Chem., 2018, 83, 6397 CrossRef CAS PubMed.
- Jørgensen and co-workers have described just one example of the Friedel–Crafts/oxa-Michael reaction of 4-hydroxyindole occurring at the C-5 position of the carbocyclic ring:
(a) P. H. Poulsen, K. S. Feu, B. M. Paz, F. Jensen and K. A. Jørgensen, Angew. Chem., Int. Ed., 2015, 54, 8203 CrossRef CAS PubMed For other recent examples, see: ;
(b) M. Xiao, D. Xu, W. Liang, W. Wu, A. S. C. Chan and J. Zhao, Adv. Synth. Catal., 2018, 360, 917 CrossRef CAS;
(c) W. Xun, B. Xu, B. Chen, S. Meng, A. S. C. Chan, F. G. Qiu and J. Zhao, Org. Lett., 2018, 20, 590 CrossRef CAS PubMed;
(d) J.-Y. Liu, X.-C. Yang, H. Lu, Y.-C. Gu and P.-F. Xu, Org. Lett., 2018, 20, 2190 CrossRef CAS PubMed;
(e) Z.-T. Yang, W.-L. Yang, L. Chen, H. Sun and W.-P. Deng, Adv. Synth. Catal., 2018, 360, 2049 CrossRef CAS.
- For the earlier non-enantioselective examples of the activating/directing ability of the OH group in hydroxyindoles, see:
(a) S. A. Monti, W. O. Johnson and D. H. White, Tetrahedron Lett., 1966, 7, 4459 CrossRef;
(b) F. Troxler, G. Bormann and F. Seemann, Helv. Chim. Acta, 1968, 51, 1203 CrossRef CAS.
-
(a) S. J. Williams, Expert Opin. Ther. Pat., 2013, 23, 79 CrossRef CAS PubMed;
(b) L. W. L. Woo, A. Purohit and B. V. L. Potter, Mol. Cell. Endocrinol., 2011, 340, 175 CrossRef CAS PubMed;
(c) J.-Y. Winum, A. Scozzafava, J.-L. Montero and C. T. Supuran, Med. Res. Rev., 2005, 25, 186 CrossRef CAS PubMed;
(d) S. J. Kim, M.-H. Jung, K. H. Yoo, J.-H. Cho and C.-H. Oh, Bioorg. Med. Chem. Lett., 2008, 5815 CrossRef CAS PubMed;
(e) S. J. Kim, H. B. Park, J. S. Lee, N. H. Jo, K. H. Yoo, D. Baek, B.-W. Kang, J.-H. Cho and C.-H. Oh, Eur. J. Med. Chem., 2007, 1176 CrossRef PubMed;
(f) S. R. Hanson, L. J. Whalen and C.-H. Wong, Bioorg. Med. Chem., 2006, 8386 CrossRef CAS PubMed.
-
L. De Munck, C. Vila and J. R. Pedro, in Targets in Heterocyclic Chemistry, ed. O. A. Attanasi, P. Merino and D. Spinelli, Società Chimica Italiana, 2017, p. 137 Search PubMed.
-
(a) S. G. Lee and S.-G. Kim, RSC Adv., 2017, 7, 34283 RSC;
(b) M. Montesinos-Magraner, R. Cantón, C. Vila, G. Blay, I. Fernández, M. C. Muñoz and J. R. Pedro, RSC Adv., 2015, 75, 60101 RSC.
- For other asymmetric aza-Friedel-Crafts reactions with other cyclic imines, see:
(a) Z. Yan, X. Gao and Y.-G. Zhou, Chin. J. Catal., 2017, 38, 784 CrossRef CAS;
(b) M. Rueping, S. Raja and A. Nuñez, Adv. Synth. Catal., 2011, 353, 563 CrossRef CAS;
(c) D. Zhou, Z. Huang, X. Yu, Y. Wang, J. Li, W. Wang and H. Xie, Org. Lett., 2015, 17, 5554 CrossRef CAS PubMed;
(d) L. Wu, R.-R. Liu, G. Zhang, D.-J. Wang, H. Wu, J. Gao and Y.-X. Jia, Adv. Synth. Catal., 2015, 357, 709 CrossRef CAS;
(e) K.-F. Zhang, J. Nie, R. Guo, Y. Zheng and J.-A. Ma, Adv. Synth. Catal., 2013, 355, 3497 CrossRef CAS;
(f) T. Kano, R. Takechi, R. Kobayashi and K. Maruoka, Org. Biomol. Chem., 2014, 12, 724 RSC;
(g) E. Xie, A. Rahman and X. Lin, Org. Chem. Front., 2017, 4, 1407 RSC.
- See the ESI† for further details.
- We did not observe the formation of the 7-alkylated product 4d or the 5,7-dialkylated product 5d.
- We only observed the product alkylated at the C-4 position and we did not observe in the crude 1H NMR any other alkylated product.
- Cyclic ketimines such as 4-methylbenzo[e][1,2,3]oxathiazine 2,2-dioxide or 3-methylbenzo[d]isothiazole 1,1-dioxide were not reactive under the optimized reaction conditions.
- CCDC 1875758 (3e) and CCDC 1846586 (7a) contains the supplementary crystallographic data for this paper.
- L. De Munck, A. Monleón, C. Vila and J. R. Pedro, Adv. Synth. Catal., 2017, 359, 1582 CrossRef CAS.
Footnote |
† Electronic supplementary information (ESI) available. CCDC 1875758 and 1846586. For ESI and crystallographic data in CIF or other electronic format see DOI: 10.1039/c8nj05577g |
|
This journal is © The Royal Society of Chemistry and the Centre National de la Recherche Scientifique 2019 |