DOI:
10.1039/C8NJ03040E
(Paper)
New J. Chem., 2019,
43, 338-347
Pharmacokinetic/pharmacodynamic modelling of effective components of Fangji Huangqi Tang for its treatment of nephrotic syndrome
Received
26th June 2018
, Accepted 16th November 2018
First published on 17th November 2018
Abstract
This study was designed to evaluate the potential effective components of Fangji Huangqi Tang for the treatment of nephrotic syndrome based on a pharmacokinetic/pharmacodynamic model. To quantify the relationships between the pharmacokinetics and pharmacodynamics of fangchinoline and tetrandrine, the pharmacokinetic/pharmacodynamic model was set up by combining pharmacokinetic parameters and the effective indexes in rats. Compared with a model group, the effective indexes including serum urea nitrogen, cysteine protease inhibitors C, total cholesterol and triglycerides in the FHT group became significantly lower with a dosing time extension. The pharmacokinetic/pharmacodynamic modeling results showed that there were good linear relationships between the areas under the concentration-time curves and these effective indexes. Finally, fangchinoline and tetrandrine were proved to be the main effective chemical materials of FHT to cure nephrotic syndrome in rats.
1. Introduction
Traditional Chinese Medicine (TCM) was applied to provide material support and an objective vector for the dialectical treatment under the guidance of traditional Chinese medical theory. TCM has a clinical application history of more than one thousand years in China, and has made a great contribution to the local people's health. The application of TCM in clinics emphasizes the compatibility of sovereign, subject, assistant and beadle. It aims to work through the synergy of the drugs. For a long time, the international development of TCM has been restricted, although the curative effects of TCM have been widely and fully recognized.1,2 The main reason for this restriction was that the material basis of TCM was still a mystery, as well as the conversion and interference of the complex compositions generated during the preparation processes.3–5 All these factors made the research of the effective material basis of TCM quite difficult. Because of this, the effective material basis research of TCM became a hot topic in modern Chinese medicine research field. At present, the research on the effective material basis of TCM is developing towards more pluralism, systematization and objectification. It was embodied in: ① from focusing on isolation to obtain a single component to focus more on effective sites and effective chemical composition groups;6,7 ② the mechanism of action of the components in vitro was further investigated at a molecular level;8,9 ③ from a single study on the chemical composition of TCM to reveal an inherent relationship between the components and the effects on biological bodies.10,11 As for the modern research methods to study TCM effective material basis, a series of related practical techniques emerged successively, including dismantlement on prescriptions, pharmaceutical chemistry, serum pharmacology, fingerprint profiling, pharmacokinetics of chemical components, spectrum-effect correlation, the pharmacokinetic/pharmacodynamic model (PK-PD model) and so on. Among all these methods, the PK-PD model is a dynamic process with changes of pharmacokinetic and pharmacodynamic indexes quantification in vivo.12–16 It is essentially the conversion process of drug quantity and its effect. This kind of study could provide a comprehensive understanding of some specific activities of TCM.17,18
Fangji Huangqi Tang (FHT) was a classical and practical formula that was first recorded in “Jin-Gui-Yao-Lue” by a Chinese physician, Zhongjing Zhang, which was widely used for treating the injuries of nephrotic syndrome, chronic glomerular nephritis, heart, edema, as well as rheumatoid arthritis. It was composed of four herbs including Astragalus membranaceus (Fisch.) Bge. var. mongholicus (Bge.) Hsiao, Glycyrrhiza uralensis Fisch., Stephania tetrandra S. Moore and Atractylodes macrocephala Koidz. Modern scholars have carried out some research work on the compatibility theory of FHT. The achievement of these studies laid a good foundation for the application of modern science and technology to explain the rationality of the compatibility theory of FHT. However, the depth of the study was limited to the chemical identification or determination of the main active components in vitro. The effective material basis of FHT was still unclear.19–21
Stephania tetrandra S. Moore is the primary ingredient in FHT, and is believed to be the monarch drug in this prescription according to the theory of TCM. Tetrandrine (TET) and fangchinoline (FAN) are the characteristic bisbenzylisoquinoline alkaloids isolated from Stephania tetrandra S. Moore. So, a hypothesis was given that there would be some underlying relationships between these two compounds and the FHT anti-nephrotic syndrome bioactivities. The hypothesis was tested by using a PK-PD model in this paper. For this purpose, the methods employed included analytical chemistry, pharmacology, molecular biology and mathematical statistics. The anti-nephrotic syndrome biological effects evaluation of FHT in this study included a systemic monitoring of urinary protein excretion, total cholesterol (TC), total triglyceride (TG), blood urea nitrogen (BUN) and cystatin C (Cys C). It was hoped that this study would give a reasonable chemical material foundation of FHT for the treatment of nephrotic syndrome. Also, it might provide a new thinking and reference mode for the modern research of the effective material basis of TCM.
2. Materials and methods
2.1 Chemicals and reagents
Astragalus membranaceus (Fisch.) Bge. and Glycyrrhiza uralensis Fisch. were obtained from the Nanjing Haichang Chinese Medicine Group Corporation (Nanjing, China). Stephania tetrandra S. Moore and Atractylodes macrocephala Koidz. were obtained from the Hebei Chinese Medicine Group Corporation (Hebei, China). These species were first identified by Prof. Jianwei Chen (College of Pharmacy, Nanjing University of Chinese Medicine) for their botanical origin before use. Acetonitrile and water were of LC-MS grade from Merck (Darmstadt, Germany). Tetrandrine, fangchinoline and isopsoralen (the internal standard, IS) with purities of 99% or higher were purchased from the National Institute for the Control of Pharmaceutical and Biological Products (Beijing, China). Their chemical structures are shown in Fig. 1. HPLC grade methanol was purchased from ANPEL Scientific Instrument Co., Ltd (Shanghai, China), and HPLC grade formic acid with a purity of 99% was purchased from Anaqua Chemicals Supply (USA). All the other reagents were of analytical grade and obtained from Nanjing Chemical Reagent Company (Nanjing, China).
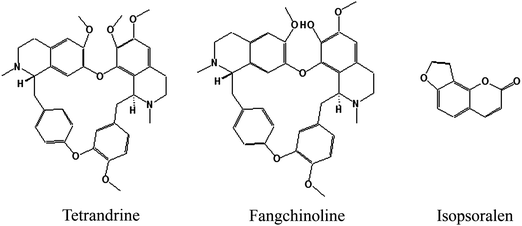 |
| Fig. 1 Chemical structures of tetrandrine, fangchinoline and isopsoralen (internal standard). | |
2.2 FHT preparation
According to the original composition and preparation process recorded in “Jin-Gui-Yao-Lue”, FHT was obtained following these procedures: pieces of Stephania tetrandra S. Moore, Astragalus membranaceus (Fisch.) Bge., Atractylodes macrocephala Koidz. and Glycyrrhiza uralensis Fisch. were mixed together in a ratio 4
:
5
:
3
:
2 (w/w/w/w) and macerated in deionized water for 30 min before being decocted twice with boiling water (1
:
10, w/v and 1
:
8, w/v, respectively), for 20 min each time. Then the solution was filtered through a two-layer mesh, and the filtrate was combined and concentrated to a density of 1 g mL−1.
2.3 Animals
Male Sprague-Dawley rats (n = 18), weighing 200–220 g, were supplied by the Slaccas Experiment Animal Company (Shanghai, China). The temperature and humidity conditions of the animal feeding environments were kept constant, with food and water provided ad libitum. All the rats were acclimated in the laboratory for at least one week prior to the experiment. Before testing, the animals were fasted overnight with free access to water. All the animal experiments were carried out according to the Guidelines for the Care and Use of Laboratory Animals, and were approved by the Animal Ethics Committee of Nanjing University of Chinese Medicine.
2.4 Modeling and sample collection
The rats were randomly divided into a normal group (Group A, n = 6) and a model group (n = 12). The nephrotic syndrome model was prepared by giving rats an injection of adriamycin (4 mg kg−1, single dose) via the tail vein on the 1st and 14th day. The normal group rats were injected with the same volume of saline instead.
On the 22nd day after the first adriamycin injection, all twelve nephropathic rats were further divided randomly into an untreated model group (n = 6) and a test group (n = 6). The rats in the test group were administered with FHT at a dose of 14 g kg−1 once a day for five weeks; meanwhile, the rats in the normal and model group were given saline instead. Both FHT and saline were given to the rats by gavage only once within one day. Then, blood samples (no more than 0.5 mL) of all three groups of rats were collected from the orbital vein into tubes without heparinization on the 5th, 12th, 19th, 22nd, 23rd, 24th, 25th, 36th, 44th, 49th, 54th and 57th day.
On the 0th (before modeling), 3rd, 10th, 17th, 28th, 45th and 52nd days, after the adriamycin injection, urine samples within 24 h from each rat in the normal, model and test groups were collected by using metabolic cages. The success of nephropathy modeling was confirmed by the detection of massive proteinuria in the urine samples from the model group rats.
2.5 Serum sample preparation
The blood samples were kept for 30 min and then centrifuged to get a good separation at 4000 rpm for 5 min. The supernatant was collected and stored at −20 °C until analysis. 0.1 mL of serum was spiked into centrifuge tubes and then mixed with 500 μL of methanol by vortex mixing for 30 s. The mixture was separated by centrifugation at 12
000 rpm for 5 min. The upper layer was then transferred into another tube and evaporated to dryness under nitrogen at 40 °C. The residue was reconstituted with 100 μL of chromatographic methanol, vortexed for 30 s, and centrifuged at 12
000 rpm for 3 min. A volume of 2 μL of the supernatant was injected for UHPLC-ESI-QqQ-MS analysis.
2.6 HE staining
Kidney samples from each group of rats were sliced by using a 10% formalin solution. After HE staining, the following indicators were observed: (1) hypertrophy of the glomerulus; (2) degeneration of renal tubular epithelial cells; (3) expansion of the renal tubule; (4) fibrosis in the renal interstitium; (5) inflammatory cell infiltration; (6) transparent tube type in renal tubules. The lesion was marked from light to heavy by 0.5 (slight), 1 (mild), 2 (moderate), 3 (severe), and 0 (basically normal), and then all the scores were added together. The average score of each animal was calculated and obtained (Mean ± SD).
2.7 Anti-nephrotic syndrome biological effects evaluation
Urinary protein excretion was determined by the benzethonium chloride turbidimetric method using the urine samples collected within a period of 24 h at certain time points after drug administration by using metabolic cages. TC, TG, BUN and Cys C levels were measured by using commercially available kits based on colorimetric assays (Jian Cheng Biological Technology Co., LTD, Nanjing, China).
2.8 UHPLC-ESI-QqQ-MS condition
The UHPLC system (Shimadzu, Kyoto, Japan) consisted of a LC-30AD binary pump, an autosampler (Model SIL-30SD), an on-line degasser (DGU-20A5R) and a column temperature controller compartment (CTO-30A). An Extend C18 column (Agilent, USA, 2.1 mm × 100 mm, 1.8 μm) at a temperature of 30 °C was used for separation. Mass spectrometric detection was performed using a triple quadrupole 5500 instrument (AB Sciex, Concord, Ontario, Canada) equipped with an electrospray ionization (ESI) source.
The separation was achieved by a linear gradient elution using a mobile phase consisting of 0.1% (v/v) aqueous formic acid (A) and acetonitrile (B). The gradient program was as follows: 0.1–0.5 min, 5–20% B; 0.5–3.5 min, 20–80% B; 3.5–5.5 min, 80–95% B; 5.5–6.5 min, 95% B; 6.5–7 min, 95–5% B; 7–7.5 min, 5% B.
The flow rate was maintained at 0.3 mL min−1. The samples were placed in an autosampler at 4 °C prior to injection, and the injection volume was 2 μL. Positive ionization mass spectrometry was conducted due to the physicochemical properties of the analytes. The scan mode was set to multiple reactions monitoring (MRM) mode, with the selected monitor ion pairs of m/z 623.2/174.1 for TET, m/z 609.3/367.1 for FAN and m/z 187.2/115.0 for I.S. The source condition parameters were as follows: the curtain-gas was set at 35; the ion source temperature was adjusted to 500 °C; the ion source gas 1 (GS 1) and ion source gas 2 (GS 2) were both set at 55.
2.9 The PK-PD model
The method used for drug concentration determination was developed and validated based on a previous study.22 The drug concentration data in the rats’ blood measured in this experiment were modeled by R software. The pharmacokinetic models of TET and FAN in rats were established. In this paper, the optimal model was selected based on fitting residuals. In this way, the pharmacokinetic characteristics of TET and FAN in rats were obtained. Also, the pharmacokinetic parameters were calculated, and the drug concentration-time curves were drawn. Coupled with the pharmacodynamic results, a PK-PD model of FHT was established for the treatment of nephrotic syndrome.
3. Results and discussion
3.1 Pharmacodynamics of FHT
3.1.1 Renal pathological section results.
After the calculation of the lesion score, the results showed that the scores for the normal group, model group and test group were 0, 9.81, and 5.25, respectively. The lesion of the renal tissue caused by the nephrotic syndrome model in rats in this study was mainly manifested in a coexistence of glomerular atrophy and glomerular hypertrophy. Also, the tubular vacuolization and dilation in the model group were more significant. Renal interstitial fibrosis, inflammatory cell infiltration and a large number of transparent tubes in the dilated lumen of the renal tubule were all visible. In all, compared with the normal group, these pathological changes mentioned above indicated that the nephrotic syndrome model was replicated successfully. FHT showed an effect of relieving relative kidney injuries and corrected them towards normal. Typical kidney histopathological sections of rats in each group are shown in Fig. 2.
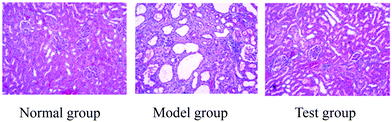 |
| Fig. 2 Kidney histopathological sections of rats in normal, model and test groups (HE, ×100). | |
3.1.2 Results of the biochemical indexes determination.
The urine protein content after 24 h in rats from the three groups was measured. If the urine protein content after 24 h was higher than 100 mg, it meant successful modeling was achieved. As shown in Fig. 3, the urine protein contents after 24 h were all higher than 100 mg, 10 days after modeling. Compared with the normal group, there were significant increments in the 24 h urinary protein content in the model group on the 3rd, 10th, 17th, 28th, 45th and 52nd days (P < 0.001). Meanwhile, compared with the model group, the urinary protein contents after 24 h in the test group were reduced, and significant differences were found on the 45th and 52nd days. A protective effect of FHT on the damage of the glomerular filtration membrane was indicated.
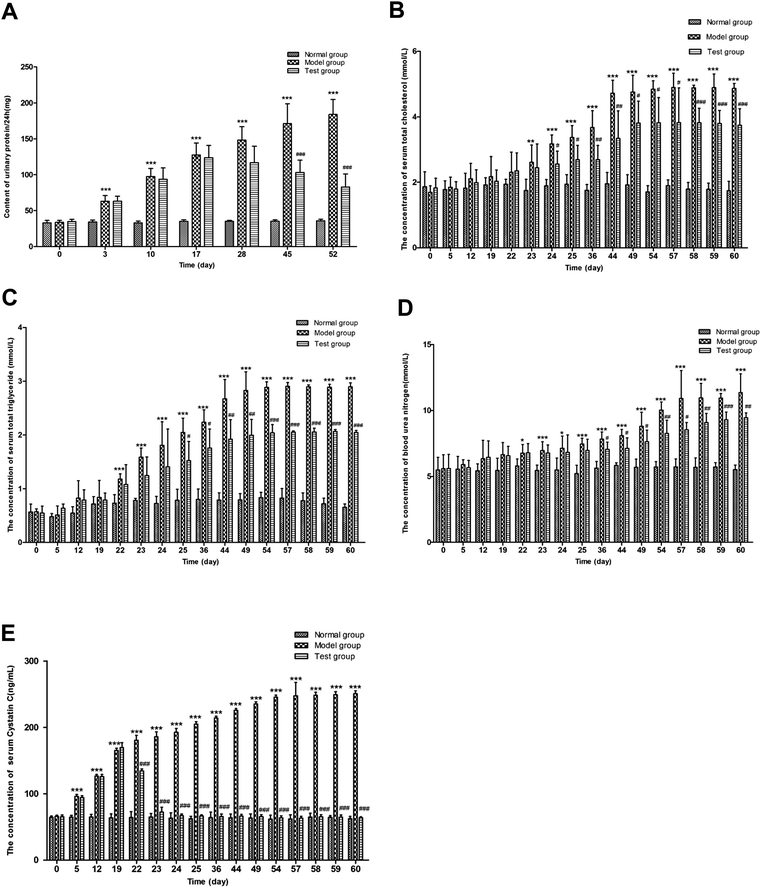 |
| Fig. 3 Urine protein of 24 h (A), serum total cholesterol (B), serum triglyceride (C), serum urea nitrogen (D) and cystatin C (E) contents of rats in normal, model and test groups. | |
Compared with the normal group, the serum TC levels of the model group on the 24th, 25th, 36th, 44th, 49th, 54th, 57th, 58th, 59th and 60th days were significantly increased (P < 0.001). Meanwhile, the serum total cholesterol levels of the test group on the 24th, 25th, 36th, 44th, 49th, 54th, 57th, 58th, 59th and 60th days were statistically lower in comparison with the model group. The results of the serum TC assay in the normal, model and test groups at different time points are shown in Fig. 3.
As shown in Fig. 3, the serum TG of the model group showed a significant increase on the 22nd, 23rd, 24th, 25th, 36th, 44th, 49th, 54th, 57th, 58th, 59th and 60th days (P < 0.001), compared with the normal group. After drug administration, under the effect of FHT, the serum TG levels in the test group on the 25th and 36th days showed a significant decrease (P < 0.05). As time went on, the trend became more evident on the 44th, 49th, 54th, 57th, 58th, 59th and 60th days (P < 0.01) compared with the model group.
Compared with the normal group, the BUN in the model group showed a significant increase on the 23rd, 24th, 25th, 36th, 44th, 49th, 54th, 57th, 58th, 59th and 60th days (P < 0.001). Compared with the model group, the contents of BUN in the test group were significantly reduced on the 36th, 44th, 49th, 54th, 57th, 58th, 59th and 60th days. The contents of BUN in each group are shown in Fig. 3.
In accordance with the biochemical indexes mentioned above, the contents of Cys C in the model group were significantly increased on the 5th, 12th, 19th, 22nd, 23rd, 24th, 25th, 36th, 44th, 49th, 54th, 57th, 58th, 59th and 60th days (P < 0.001), compared with the normal group. Also, after drug administration, the contents of Cys C in the test group showed a downward trend on the 22nd, 23rd, 24th, 25th, 36th, 44th, 49th, 54th, 57th, 58th, 59th and 60th days (P < 0.001) in comparison with the model group (Fig. 3).
3.2 Pharmacokinetic study of the main components in FHT
3.2.1 Method validation.
3.2.1.1 Specificity.
The representative chromatograms of a blank, a blank plasma spiked with standard solutions and a real plasma sample after oral administration of FHT are shown in Fig. 4. The retention times for FAN, TET and I.S. were 3.25, 3.36 and 4.04 min, respectively. Under the established chromatographic condition, no endogenous interference from the plasma was observed, and all the analytes, as well as I.S., did not interfere with each other.
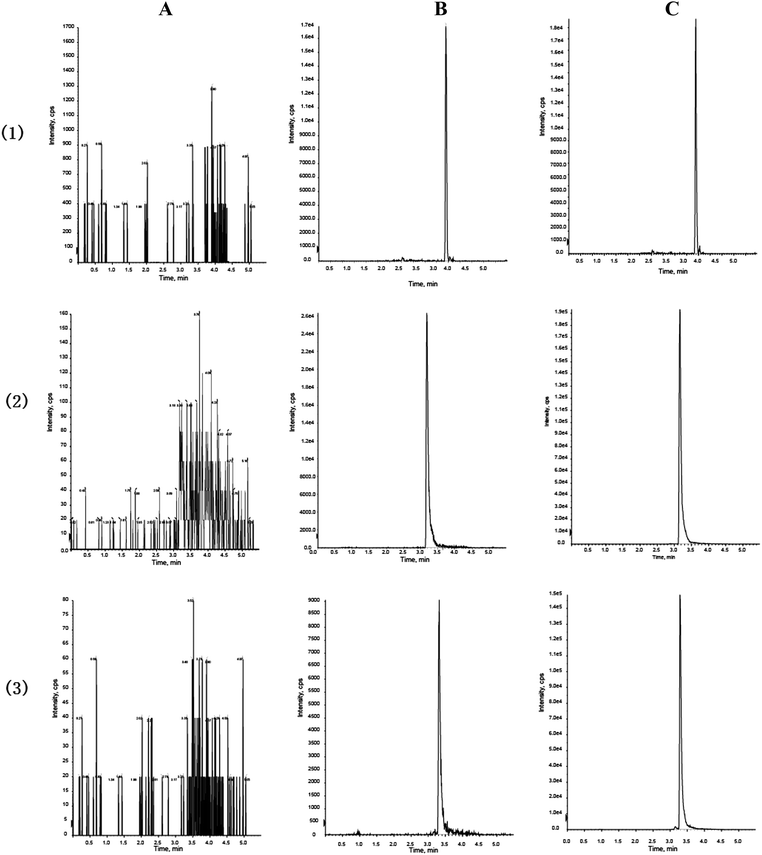 |
| Fig. 4 Representative MRM chromatograms of IS (1), fangchinoline (2) and tetrandrine (3) in plasma: (A) blank plasma; (B) blank plasma spiked with IS, fangchinoline and tetrandrine at LLOQ, respectively; (C) a real plasma sample obtained after oral administration of Fangji Huangqi Tang. | |
3.2.1.2 Calibration curves and LLOQs.
Typical equations for the calibration curves and correlation coefficients (r) were y = 0.67134x − 0.01541 (r = 0.9914) for FAN, and y = 0.16068x + 0.00050 (r = 0.9922) for TET. The calibration curves of the two analytes were linear within the ranges of 11.5–736 ng mL−1 and 15.0–960 ng mL−1, respectively. The lower limits of quantification (LLOQs) of the two analytes were 11.5 ng mL−1 and 15.0 ng mL−1, respectively.
3.2.1.3 Precision and accuracy.
The intra- and inter-day precision and accuracy were determined by measuring six replicates of QC samples at three different concentration levels. The results are shown in Table 1. The intra- and inter-day precision ranged within 1.7–9.7% for TET and 1.5–10.7% for FAN, respectively; while the intra- and inter-day accuracy ranged within 4.7–10.2% for TET and 5.8–11.2% for FAN, respectively.
Table 1 Precision, accuracy, and extraction recovery for the determination of tetrandrine and fangchinoline in rat plasma (n = 6)
Components |
Concentration (ng mL−1) |
Precision (RSD, %) |
Accuracy (RE, %) |
Extraction recovery |
Intra-day |
Inter-day |
Intra-day |
Inter-day |
Mean (%) |
RSD (%) |
Tetrandrine |
30.0 |
4.9 |
3.7 |
9.2 |
−6.5 |
98.1 |
9.4 |
120 |
9.7 |
6.4 |
10.2 |
7.5 |
102.4 |
9.3 |
768 |
1.7 |
4.3 |
4.7 |
−9.5 |
90.0 |
3.5 |
Fangchinoline |
23.0 |
3.6 |
4.3 |
6.8 |
−6.5 |
101.5 |
5.4 |
92 |
10.7 |
4.8 |
11.2 |
5.8 |
102.3 |
10.4 |
589 |
1.5 |
3.9 |
7.2 |
−9.7 |
89.0 |
2.8 |
3.2.1.4 Extraction recovery and matrix effect.
The extraction recoveries from the plasma samples at the three concentration levels were 98.1% (RSD, 9.4%), 102.4% (RSD, 9.3%) and 90.0% (RSD, 3.5%) for TET; and 101.5% (RSD, 5.4%), 102.3% (RSD, 10.4%) and 89.0% (RSD, 2.8%) for FAN as shown in Table 1.
As for a matrix effect, all the calculated values were between 91.6% and 101.6%, indicating that no significant matrix effect was observed.
3.2.1.5 Sample stability.
The mean relative differences of the concentrations of TET and FAN measured in the stability tests were within 1.1 to 12.0%, indicating that the analytes in the rat plasma were stable for 4 h at room temperature, for 30 days at −20 °C and after three freeze–thaw cycles (Table 2).
Table 2 Stability of tetrandrine and fangchinoline in rat plasma samples (n = 3)
Spiked concentration (ng mL−1) |
After 3 freeze and thaw cycles |
Room temperature for 24 h |
Cold storage for 30 days |
Mean ± SD |
RE (%) |
Mean ± SD |
RE (%) |
Mean ± SD |
RE (%) |
Tetrandrine |
30.0 |
30.9 ± 1.0 |
2.9 |
29.4 ± 0.9 |
−2.1 |
30.3 ± 0.7 |
1.1 |
120 |
111.2 ± 4.6 |
−7.4 |
110.6 ± 2.6 |
−7.9 |
107.2 ± 1.0 |
−10.7 |
768 |
844.0 ± 62.4 |
9.9 |
829.1 ± 9.5 |
−8.0 |
815.5 ± 34.3 |
6.2 |
|
Fangchinoline |
23.0 |
21.9 ± 0.8 |
−4.6 |
21.6 ± 1.1 |
−5.9 |
22.4 ± 0.3 |
−2.5 |
92 |
85.2 ± 2.5 |
−7.4 |
84.5 ± 4.3 |
−8.2 |
83.4 ± 1.7 |
−9.4 |
589 |
645.1 ± 15.6 |
9.6 |
659.2 ± 7.1 |
12.0 |
647.1 ± 22.5 |
9.9 |
3.2.2 Application to pharmacokinetic study.
3.2.2.1 Concentration determination of TET and FAN in rat plasma.
After validation, the experimental method was successfully used to analyze the samples collected from the pharmacokinetic study. The mean concentration–time curves of TET and FAN in rats after FHT administration are shown in Fig. 5.
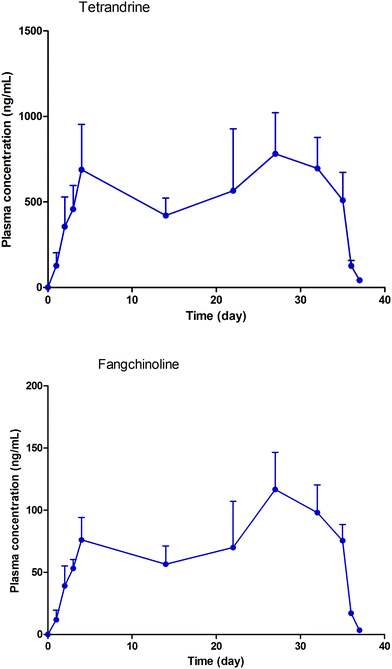 |
| Fig. 5 Mean plasma concentration–time curves of tetrandrine and fangchinoline after multiple doses of Fangji Huangqi Tang. | |
3.2.2.2 Pharmacokinetic study.
The pharmacokinetic study of FHT under multiple doses could be seen as a superposition of pharmacokinetic model of FHT with multiple single doses. Without considering the experiment under the premise of individual physiological parameters changing over time, it could be superimposed by calculating pharmacokinetic models with multiple single doses. The model was fitted with residuals minimized. The time–concentration curves of TET and FAN in the model are shown in Fig. 5. The main pharmacokinetic parameters are shown in Table 3.
Table 3 The pharmacokinetic parameters of tetrandrine and fangchinoline in rats after multiple doses of FHT
Parameters |
K
a (h−1) |
K
e (h−1) |
V
d (L kg−1) |
CL (L kg−1 h−1) |
Tetrandrine |
0.00018216 |
0.04677 |
0.08193 |
0.003831866 |
Fangchinoline |
0.00016442 |
0.05259 |
0.56618 |
0.029775406 |
In this experiment, the concentrations of TET and FAN in rats were investigated. The fitting model was established based on the minimum residual error. It was found that the real concentration–time curves of the two components in rats were consistent with those predicted by the model. According to the experimental design, drug administration began on the 22nd day and finished on the 57th day. On the 24th day, the concentrations of TET and FAN in rats reached the plateau stage, and stayed at this stable level until the 57th day. When the drug administration was finished, their concentrations began to decline from the 58th day to the 59th day. Within the time period of drug administration and detection, the results given by the fitting model were consistent with the actual conditions. According to a previous study, both TET and FAN have a relatively long half-life of 30 h in rats.22 Therefore, two days after stopping FHT administration, TET and FAN could still be detected in rats (Fig. 6).
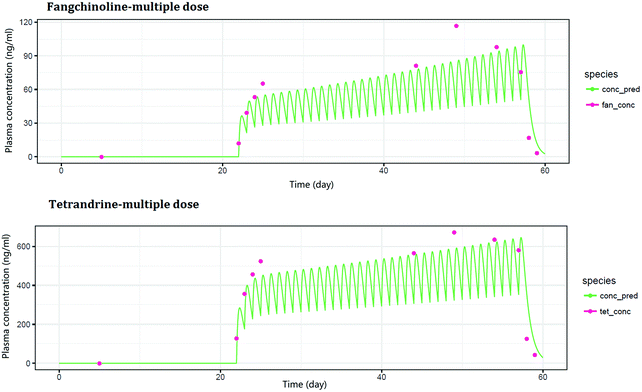 |
| Fig. 6 The observed and predicted concentration–time course of fangchinoline and tetrandrine in rats some days after multiple doses of Fangji Huangqi Tang. | |
3.3 Study on the PK-PD model
As a result of multiple dosing, the administered drug usually had a long-term accumulation effect in the experimental animals. In this situation, the AUC (area under the concentration–time curve) instead of the drug concentration was used as the independent variable of the PK-PD model for the description of the drug cumulative effect. In this way, the cumulative effect of drugs in the body would also be highlighted. The method of linear regression was used to analyze the correlation between the AUC values of TET and FAN and the efficacy indexes including serum total cholesterol, serum triglycerides and serum urea nitrogen. The results of the linear regression showed that the AUC values of TET and FAN had good linear relationships with FHT efficacy for nephrotic syndrome treatment (Fig. 7).
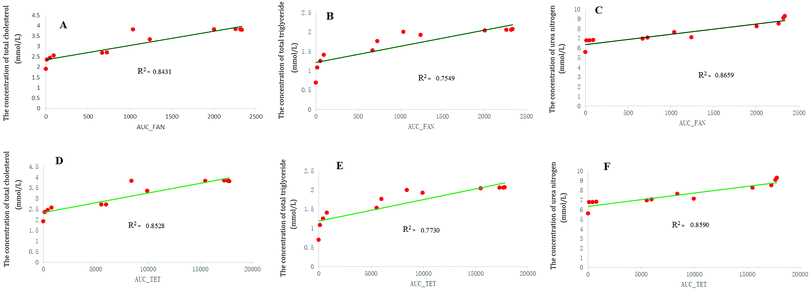 |
| Fig. 7 The linear regression curves: between the AUC value of fangchinoline and the contents of serum total cholesterol (A), serum triglycerides and (B) and serum urea nitrogen (C); between the AUC value of tetrandrine and the contents of serum triglycerides and (E), serum total cholesterol (D) and serum urea nitrogen (F) in rats. | |
4. Concluding remarks
In clinics, proteinuria is not only the most common clinical manifestation of renal disease, but also an important symptom of nephrotic syndrome. It is an independent risk factor for accelerating the progress of renal disease. Any treatment that reduces urinary protein may be beneficial to inhibiting the development of renal disease.23 In this study, the urine protein content after 24 h in the model group increased significantly, compared with the normal group. After FHT administration, in comparison with the model group, the urinary protein content after 24 h in the test group was beginning to decrease significantly on the 28th day. This phenomenon indicated that the function of glomerular filtration was improved in rats after a week of continuous FHT administration. Furthermore, serum urea nitrogen and cysteine protease inhibitor C are also two important indicators for evaluating glomerular filtration. The results of this paper showed that the levels of serum urea nitrogen and cysteine protease inhibitor C were significantly higher in the model group compared with the normal group. Then, after drug administration, compared with the model group, the test group showed a significant decrease in the levels of serum urea nitrogen and cysteine protease inhibitor C, indicating that FHT had a protective effect on glomerular injury.
Hyperlipidemia is an important clinical manifestation of nephrotic syndrome.24 The main reason is that lipid metabolism requires the transport and release of lipoproteins. The destruction of the glomerular filtration function will lead to a loss of large amounts of protein in blood, including a substantial reduction in apolipoprotein, which will then cause excessive synthesis and an increase in the secretion of lipoproteins in the liver, and will finally result in a disorder of lipid metabolism. The most important feature of hyperlipidemia is that the contents of serum total cholesterol and total triglyceride will increase.25 Actually, lipid metabolism disorder is a clinical manifestation of chronic renal disease and is involved in the occurrence and development of chronic renal disease. It was reported that lipids entering would damage the glomerular filtration membrane mesangial area, and cause mesangial cell proliferation and matrix increase. The lipids were filtered and then reabsorbed by the renal tubular, which would cause renal fibrosis. Lipid metabolism disorder could aggravate renal injury and promote glomerular sclerosis.26 In this study, compared with the normal group, the total serum total cholesterol and total triglyceride levels in the model group increased significantly. Meanwhile, compared with the model group, the contents of serum total cholesterol and triglycerides in the test group were significantly reduced. The results suggested that FHT could improve a lipid metabolism disorder caused by nephrotic syndrome in rats.
The area under the curve is an objective indicator to evaluate the extent of a drug entering the body.27 In this study, the AUCs of TET and FAN were selected as the independent variable of PK-PD modeling. The relationship between the efficacy indexes and AUCs of these two components were simulated. A good correlation between the PK input and the pharmacodynamic indexes was found. TET and FAN, as the main chemical compositions of FHT, which played an important role during the FHT pharmacodynamic effect, were successfully evaluated. They are alkaloids derived from Stephania tetrandra S. Moore—the monarch drug in this prescription. Moreover, these two components of alkaloids were also used as pharmacodynamic substances to fully embody the synergistic effect of components in complex traditional Chinese medicine systems in a multi-target way. Additionally, TET was found to be an herbal ingredient showing promise in the treatment for refractory nephrotic syndrome.28,29 Recently, FAN was reported to be able to protect against renal injury in diabetic nephropathy by modulating the MAPK signaling pathway.30 All these literatures together demonstrated that the conclusions of this paper were of high credibility.
List of abbreviations
K
a
| Absorption rate constant |
V
d
| Apparent volume of distribution |
AUC | Area under the concentration–time curve |
BUN | Blood urea nitrogen |
CL | Clearance |
Cys C | Cysteine protease inhibitors C |
ESI | Electrospray ionization |
K
e
| Elimination rate constant |
FAN | Fangchinoline |
FHT | Fangji Huangqi Tang |
HPLC | High-pressure liquid chromatography |
IS | Internal standard |
LC-MS | Liquid chromatography-mass spectrometer |
LLOQs | Lower limits of quantification |
MRM | Multiple reactions monitoring |
PK-PD model | Pharmacokinetic/pharmacodynamic model |
TET | Tetrandrine |
TC | Total cholesterol |
TG | Total triglyceride |
TCM | Traditional Chinese medicine |
UHPLC-ESI-QqQ-MS | Ultra-high-pressure liquid chromatography coupled electrospray ionization source triple-quadrupole mass spectrometer |
Conflicts of interest
None.
Acknowledgements
This study was financially supported by National Natural Science Foundation of China (No. 81503207), Major Projects of Natural Science Foundation of Jiangsu Higher Education Institutions (No. 17KJA360006) and Jiangsu Province “333” project.
References
- X. Y. Liu, H. Y. Wang, X. Q. Li, J. J. Wu, B. Y. Yu and J. H. Liu, Biomed. Pharmacother., 2017, 94, 794–803 CrossRef PubMed . [PubMed: 28802232].
- X. F. Tang, H. L. Nian, X. X. Li, Y. Yang, X. J. Wang, L. P. Xu, H. T. Shi, X. W. Yang and R. H. Liu, BMC Complementary Altern. Med., 2017, 17, 380 CrossRef PubMed . [PubMed: 28764781].
- M. Wang, D. Q. Chen, L. Chen, H. Zhao, D. Liu, Z. H. Zhang, N. D. Vaziri, Y. Guo, Y. Y. Zhao and G. Cao, J. Agric. Food Chem., 2018, 66, 1828–1842 CrossRef CAS PubMed . [PubMed: 29383936].
- M. Y. Zhang, H. W. Wu, F. F. Guo, Y. Y. Yu, J. Y. Wei, Y. Geng, S. F. Wang, S. Y. Li and H. J. Yang, Mol. BioSyst., 2017, 13, 1469–1480 RSC . [PubMed: 28604846].
- B. Cheng, H. Zheng, F. Wu, J. X. Wu, X. W. Liu, C. L. Tang, S. Y. Lu, Z. N. Chen, F. M. Song, J. X. Ruan, H. Y. Zhang, H. Song, W. Liu, H. W. Guo, Y. H. Liang and Z. H. Su, J. Chromatogr. B: Anal. Technol. Biomed. Life Sci., 2017, 1061, 282–291 CrossRef PubMed . [PubMed: 28763759].
- X. Zhang, Q. M. Du, Y. Yang, J. N. Wang, S. Dou, C. Liu and J. G. Duan, Biomed. Pharmacother., 2017, 91, 1042–1052 CrossRef CAS PubMed . [PubMed: 28525945].
- D. N. Cui, X. Wang, J. Q. Chen, B. Lv, P. Zhang, W. Zhang, Z. J. Zhang and F. G. Xu, Front. Pharmacol., 2017, 8, 211 CrossRef PubMed . [PubMed: 28484391].
- H. Q. Pang, S. J. Yue, Y. P. Tang, Y. Y. Chen, Y. J. Tan, Y. J. Cao, X. Q. Shi, G. S. Zhou, A. Kang, S. L. Huang, Y. J. Shi, J. Sun, Z. S. Tang and J. A. Duan, Front. Pharmacol., 2018, 9, 165 CrossRef PubMed . [PMID: 29551975].
- T. C. Zhao, L. Y. Chang, B. Y. Zhang, M. Lu, X. Y. Wang, J. O. Orgah, Y. F. Wang, X. X. Tian, J. Yang, G. W. Fan, B. L. Zhang and Y. Zhu, Front. Pharmacol., 2017, 8, 361 CrossRef PubMed . [PMID: 28659797].
- X. H. Zhang, W. Zheng, T. R. Wang, P. Ren, F. S. Wang, X. L. Ma, J. Wang and X. Huang, Front. Pharmacol., 2017, 8, 452 CrossRef PubMed . [PMID: 28769792].
- C. Lv, X. T. Wu, X. Wang, J. Su, H. W. Zeng, J. Zhao, S. Lin, R. H. Liu, H. L. Li, X. Li and W. D. Zhang, Sci. Rep., 2017, 7, 352 CrossRef PubMed . [PMID: 28336967].
- R. Tanaka, Y. Sato, K. Goto, N. Yasuda, Y. Ohchi, Y. Suzuki, T. Ueno, K. Ito, T. Kaneko, S. Kurogi, S. Nonoshita and H. Itoh, Biol. Pharm. Bull., 2017, 40, 1226–1231 CrossRef CAS PubMed . [PMID: 28769004].
- Y. Samukawa, M. Mutoh, S. Chen and N. Mizui, Biol. Pharm. Bull., 2017, 40, 1207–1218 CrossRef CAS PubMed . [PMID: 28769002].
- P. Lees, T. Potter, L. Pelligand and P. L. Toutain, J. Vet. Pharmacol. Ther., 2017, 41, 1–11 Search PubMed . [PMID: 28736817].
- Q. Y. Zhou, G. J. Zhang, Q. Wang, W. G. Liu, Y. Huang, P. L. Yu, Y. Q. Li, H. Z. Ding and B. H. Fang, Front. Pharmacol., 2017, 8, 392 CrossRef PubMed . [PMID: 28701951].
- S. Iwasaki, T. Hamada, I. Chisaki, T. Andou, N. Sano, A. Furuta and N. Amano, J. Pharmacol. Exp. Ther., 2017, 362, 441–449 CrossRef CAS PubMed . [PMID: 28698254].
- W. Ren, R. Zuo, Y. N. Wang, H. J. Wang, J. Yang, S. K. Xin, L. Y. Han, H. Y. Zhao, S. Y. Han, B. Gao, H. Hu, Y. J. Hu, B. L. Bian and N. Si, PLoS One, 2016, 11, e0156256 CrossRef PubMed . [PMID: 27280291].
- J. Song, J. Li, Y. Jin, H. Wang, S. R. Zheng and J. Gao, Eur. J. Drug Metab. Pharmacokinet., 2014, 39, 103–110 CrossRef CAS PubMed . [PMID: 24346849].
- D. Y. Yu, Y. C. Yang and Y. Lin, Chinese Journal of Integrated Traditional and Western Nephrology, 2009, 10, 295–298 Search PubMed.
- H. Y. Chen, L. Q. Jie and Y. J. Wang, Chinese Journal of Integrated Traditional and Western Nephrology, 2011, 12, 58–962 Search PubMed.
- G. L. Chen, H. P. Liu and F. Cheng, J. Tradit. Chin. Med., 2013, 33, 349–354 CrossRef PubMed . [PMID: 24024331].
- X. L. Wang, X. Liu, H. Cai, K. Pei, G. Cao, X. Y. Xu, F. Shi and B. C. Cai, J. Sep. Sci., 2015, 38, 1286–1293 CrossRef CAS PubMed . [PMID: 25645647].
- M. Maanaoui, C. Saint-Jacques, V. Gnemmi, M. Frimat, A. Lionet, M. Hazzan, C. Noël and F. Provot, Medicine, 2017, 96, e7196 CrossRef PubMed . [PMID: 28640105].
- V. P. Menéndez, V. T. Arrobas, J. A. Bermúdez, M. D. Romero Pérez, R. F. Fabiani and R. C. González, Clin. Investig. Arterioscler., 2016, 28, 227–229 Search PubMed . [PMID: 27623226].
- N. D. Vaziri, Kidney Int., 2016, 90, 41–52 CrossRef CAS PubMed . [PMID: 27165836].
- V. P. Patil, A. B. Patil, V. S. Patil and D. G. Ingleshwar, J. Clin. Diagn. Res., 2016, 10, 17–20 Search PubMed . [PMID: 27134858].
- S. Choi, D. S. Oh and U. M. Jerng, PLoS One, 2017, 12, e0182794 CrossRef PubMed . [PMID: 28797065].
- X. Q. Wang, L. Wang, Y. C. Tu and Y. C. Zhang, J. Evidence-Based Complementary Altern. Med., 2018, 2018, 8746349 Search PubMed . [PMID: 29507594].
- N. Bhagya and K. R. Chandrashekar, Phytochemistry, 2016, 125, 5–13 CrossRef CAS PubMed . [PMID: 26899361].
- Y. S. Jiang, J. G. Liu, Z. M. Zhou, K. Liu and C. Liu, Exp. Clin. Endocrinol. Diabetes, 2018 DOI:10.1055/a-0636-3883 [Epub ahead of print].
|
This journal is © The Royal Society of Chemistry and the Centre National de la Recherche Scientifique 2019 |