DOI:
10.1039/C8MD00328A
(Research Article)
Med. Chem. Commun., 2019,
10, 80-88
Evaluation of novel N′-(3-hydroxybenzoyl)-2-oxo-2H-chromene-3-carbohydrazide derivatives as potential HIV-1 integrase inhibitors†
Received
3rd July 2018
, Accepted 1st November 2018
First published on 10th December 2018
Abstract
In an attempt to identify potential new agents that are active against HIV-1 IN, a series of novel coumarin-3-carbohydrazide derivatives were designed and synthesised. The toxicity profiles of these compounds showed that they were non-toxic to human cells and they exhibited promising anti-HIV-1 IN activities with IC50 values in nM range. Also, an accompanying molecular modeling study showed that the compounds bind to the active pocket of the enzyme.
Introduction
Human immunodeficiency virus type 1 (HIV-1) is the causative agent for acquired immune deficiency syndrome (AIDS).1,2 Although improvement in the management of HIV/AIDS has been achieved through the use of antiretroviral drugs, it remains one of the world's most serious health problems.3 Despite the progress made in development of a number of drugs for treating people living with HIV/AIDS, the usefulness of many of these drugs is limited by toxicity, side effects and the development of HIV resistance.4–6 In developing countries, where the disease burden is highest, drugs targeting protease and reverse transcriptase are most commonly used as components of highly active anti-retroviral therapy (HAART),7 while integrase inhibitors are typically only introduced as a third-line treatment. There is an ongoing need for further development of anti-HIV drugs with reduced toxicity, which can be introduced in first-line therapy.
HIV-1 IN has been demonstrated as an attractive target for the development of new drugs since homologous enzymes are lacking in the human host, and the relevant enzyme is essential for a stable infection.8 Presently, the approved drugs for inhibition of HIV-1 IN, namely Raltegravir (RAL) 1,9 elvitegravir10 (EVG), dolutegravir (DTG), and Bictegravir (BIC)11,12 are all strand transfer inhibitors. Using Yan's classification of scaffolds, these successful inhibitors belong to the most well studied classes, and the two metal binding motif is conserved in each case.13 None of these are used as components of HAART in developing countries, or even as second line drugs, due to cost of manufacture.7
Inspired by the “scaffold-hopping” approach employed by Fan and co-workers,14 and rather than building on the well-studied but complex pyrimidine and quinolone scaffolds, we decided to combine two of the less established scaffolds, coumarin derivatives combined with the hydrazide moiety. Compound 2 was synthesised by Burke et al.15 and found to be active in inhibiting 3′ processing rather than the strand transfer step of IN. Compound 3 is an analogue of bis-salicyl hydrazide,15 which has been described to inhibit IN through metal chelation, but is only active in assays using Mn2+ and not Mg2+, and lacked antiviral activity in cell-based assays. Wild-type IN has been shown to use either Mn2+ or Mg2+.9 Structure activity relationship studies of salicylhydrazide inhibitors have showed that the salicyl moiety is essential for activity.15,16 Therefore, we decided to incorporate the hydrazide group16,17 on the coumarin moiety18 to achieve a synergistic effect in the anti-HIV-IN activity with possibility of reduced toxicity relative to the salicylhydrazides, and improved synthetic efficiency relative to Raltegravir.19 In this study, we designed 2-oxo-2H-chromene-3-carbohydrazide derivatives, producing relatively simple potential lead compounds as HIV-1 IN inhibitors.
We envisioned that 3-acyl-coumarin could mimic the diketo-acid moiety of Raltegravir 1 (Fig. 1), and there was the additional possibility of hydrogen bonding through the interaction of the oxygen in the pyran ring, as well as the other hydroxy, alkoxy and halogens with the amino acid residues within the catalytic site of HIV-1 IN.18 The unique structure of hydrazides has been proposed to inhibit cell proliferation through hydrophobic interaction and hydrogen bonding with the target protein.17
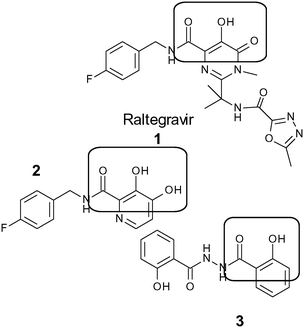 |
| Fig. 1 Raltegravir, its analogue (2) and hydrazide 3 showing the diketo moiety. | |
Results and discussion
Derivatives of N′-[2-hydroxybenzoyl]-2-oxo-2H-chromene-3-carbohydrazide, compounds 8a–t were synthesized (Scheme 1) by reacting 2-oxo-2H-chromene-3-carbonyl chlorides 4a–e with 2-hydroxybenzohydrazides 7a–e under basic conditions. The acid chloride precursors 4a–e were successfully synthesized using previously reported Knoevenagel condensation20 of salicylic aldehydes 1a–e with diethyl malonate to afford ethyl 2-oxo-2H-chromene-3-carboxylate derivatives 2a–e in good yields (73–92%). This was followed by alkaline hydrolysis using NaOH to give 3a–e (75–89%), which were reacted with oxalyl chloride to afford the key intermediates 4a–e which were used without further purification. Esterification of substituted salicylic acids 5a–d using sulfuric acid in methanol afforded the corresponding 2-hydroxy benzoate derivatives 6a–d, hydrazinolysis of which afforded substituted 2-hydroxy benzohydrazides 7a–d. Finally, the target compounds, N′-[2-hydroxybenzoyl]-2-oxo-2H-chromene-3-carbohydrazide derivatives 8a–t were obtained by stirring 7a–d with 4a–e in the presence of saturated Na2CO3 overnight.
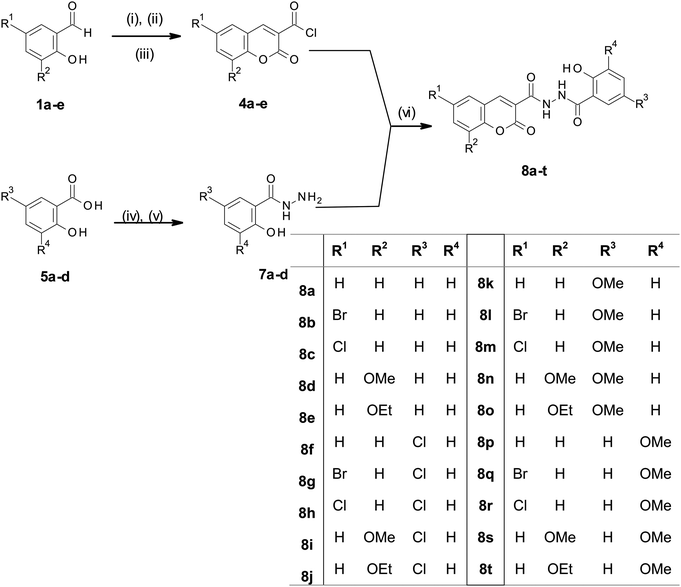 |
| Scheme 1 Reagents and conditions (i) diethyl malonate, piperidine, CH3COOH, ethanol, 80 °C, 12 h (ii) NaOH, ethanol, reflux (iii) oxalyl chloride, reflux, 8 h. (iv) Conc. H2SO4, CH3OH, reflux, 24 h (v) 65% N2H4·H2O, 80–90 °C, overnight. (vi) Na2CO3, room temperature, overnight. | |
Simple transformations of readily available starting materials were employed for the short, convergent synthesis of compounds 8a–t from substituted salicylaldehydes and salicylic acids. The methodology was, apparently, quite robust, facilitating the preparation of a fairly diverse library of compounds. The yield of the final coupling step was variable, depending on the substitution patterns on the two ring systems. Although the alkoxy substituents gave the best yields (56–83%), the most efficient syntheses were between electron rich salicyl hydrazides and coumarins bearing halides or hydrogen (8k–m and p–r). The simplicity of the method (six steps, medium temperatures, ethanol as solvent) was considered advantageous.
Compounds 8a–t (Table 1) were evaluated for their in vitro HIV-1 IN inhibition activity relative to chicoric acid (CA) as a standard, according to the method described by McColl et al.21 Both chicoric acid and Raltegravir are strand transfer inhibitors, which are effective in enzyme inhibition assays.13 CA is simply the cheaper of the two. This method uses HIV-1 subtype C IN bound to donor DNA immobilized in 96 well plates. Activity is detected using absorbance of SureBlue Reserve at 620 nm, following oxidation. Entries 16–20 showed minimal inhibition (less than 55%), and they were not examined further. It is notable that all of these compounds bear a methoxy group at position 3 on the C-ring. By contrast, entries 1–5 (Table 1), with no additional substitution on the C-ring, all showed inhibition >85%. This agrees with the result found by Burke and coworkers that the intact salicyl grouping is important for activity.15 Compounds 8a–o, which showed at least 70% inhibition of extracellular HIV-1 IN, were examined further to determine their IC50 values (calculated from dose response curves, included in the ESI†). Among these compounds, two were particularly noteworthy: 8b (IC50 = 14 nM), and 8c (IC50 = 13 nM), both of which were found to be comparable to the standard, chicoric acid (IC50 = 10 nM). These could thus serve as potential leads to develop effective IN inhibitors.
Table 1 Inhibitory potencies of coumarin hydrazides as measured in an HIV-I integrase assay
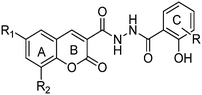
|
Entry |
Compound |
A |
C |
% inhibition |
HIV-integrase IC50/nM (SE)a |
Chicoric acid (+ve control) |
101.5 |
10. (1) |
IC50 values were determined from single replicates and are quoted with errors determined from the graphs (SE).
|
1 |
8a
|
H |
H |
90 |
70 (14) |
2 |
8b
|
6-Br |
H |
94 |
14 (29) |
3 |
8c
|
6-Cl |
H |
95 |
13 (12) |
4 |
8d
|
8-OMe |
H |
89 |
47 (11) |
5 |
8e
|
8-OEt |
H |
85 |
104 (29) |
6 |
8f
|
H |
5′Cl |
91 |
33 (29) |
7 |
8g
|
6-Br |
5′Cl |
70 |
175 (70) |
8 |
8h
|
6-Cl |
5′Cl |
79 |
41 (34) |
9 |
8i
|
8-OMe |
5′Cl |
86 |
23 (30) |
10 |
8j
|
8-OEt |
5′Cl |
88 |
31 (42) |
11 |
8k
|
H |
5′OMe |
83 |
5800 (1700) |
12 |
8l
|
6-Br |
5′OMe |
88 |
163 (49) |
13 |
8m
|
6-Cl |
5′OMe |
86 |
605 (85) |
14 |
8n
|
8-OMe |
5′OMe |
70 |
901 (143) |
15 |
8o
|
8-OEt |
5′OMe |
60 |
592 (277) |
16 |
8p
|
H |
3′OMe |
41 |
— |
17 |
8q
|
6-Br |
3′OMe |
54 |
— |
18 |
8r
|
6-Cl |
3′OMe |
44 |
— |
19 |
8s
|
8-OMe |
3′OMe |
32 |
— |
20 |
8t
|
8-OEt |
3′OMe |
24 |
— |
From the table it is possible to discern three groups according to IC50. The first group (8a–f, and h–j) show the highest levels of inhibition, and IC50 values near or below 100 nM. Within this group the compounds with the highest activity bore chloro- or bromo- substituents on ring A and only a hydroxy on ring C. These compounds both have IC50 values comparable to CA. The lowest activity was observed for those compounds bearing the methoxy groups on the C-ring (8k–o and p–t). The compounds bearing chloro-substituents on ring C showed some potential, particularly where this was coupled with an electron rich coumaryl substituent (8i and j).
In general, it was observed that the presence of chlorine atom on either ring C (salicyl moiety) or the coumarin system results in good anti-HIV-1 IN activity. The greatest impact is the substitution on ring C, suggesting that this is most likely to be the end of the molecule which binds to the metal ions in the active site.
Previous reports on the salicylhydrazide class of IN inhibitors have shown that the majority of compounds in this class are severely cytotoxic in cell-based assays, thus limiting their therapeutic application.22 In order to investigate the therapeutic index of our compounds, the cytotoxicity of all 20 compounds were measured against HeLa cells (Fig. 2). All the compounds showed low levels of cytotoxicity with cell viability remaining above 70% in most cases.
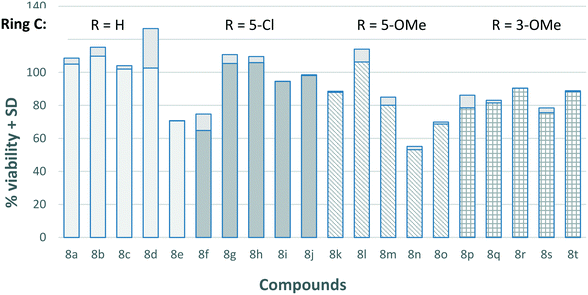 |
| Fig. 2 Cell toxicity assay (at 20 μM) of substituted N′-[2-hydroxybenzoyl]-2-oxo-2H-chromene-3-carbohydrazide derivatives (SD indicated with shading on each bar). Shading of bars correlates with substitution on C-ring. | |
The four best inhibitors in this series were 8b and c, and 8i and j, showing low or negligible cytocoxicity and excellent enzyme inhibition. We chose the best of each pair to represent the different substitution on ring C. Thus, in order to explore the binding modes of compounds 8c (IC50 = 13 nM) and 8i (IC50 = 23 nM) with the active site of the IN enzyme, molecular modelling was employed. The 1QS4 crystal structure of HIV-1 IN was selected from the protein data bank. Chicoric acid (L-CA, Fig. 3) was docked into the active site of 1QS4 because it binds to the same binding pocket as the diketo acids (DKAs),23 including Raltegravir.24 However, chicoric acid is larger than the DKAs, and thus has the potential to make significantly more contact with residues within the active site. Therefore, L-CA represents an effective probe of IN catalytic activity.25 In addition, several point mutations of IN lead to resistance to DKAs, while reduced inhibition by L-CA is only observed with one.26 This suggests that binding of L-CA might be more robust to mutations than various DKAs, the option of several interactions with the active site making up for the losses of one or two.
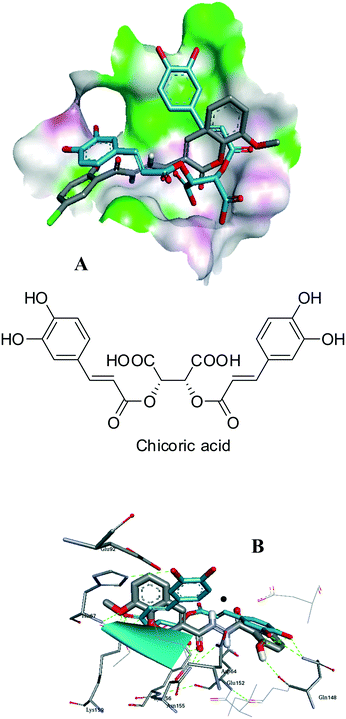 |
| Fig. 3 Molecular docking and ligand interaction of 8i (A and B) superimposed on chicoric acid (blue) and Mg2+ (black) in the active site of 1QS4, showing similarities among active site interactions. | |
In our modeling studies it was observed that chicoric acid docked near the binding site of the Mg2+, and hydrogen bonding interactions with Glu152, His67 and Gln148 were observed. The lowest energy binding mode of 8i overlaid on chicoric acid (blue) in the active site of 1QS4 (Fig. 4) revealed that inhibitors 8i and chicoric acid fill the binding pocket of 1QS4 in similar ways, with the coumaryl end interacting with His67 and the salicyl end H-bonding to Gln148.
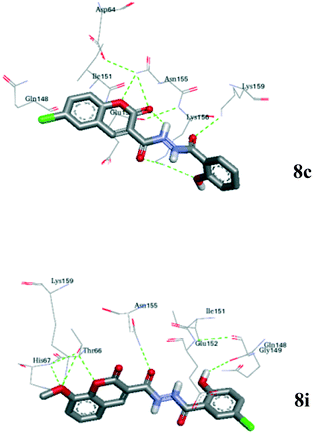 |
| Fig. 4 The binding mode of 8i and 8c (coloured by atom) in the active site of 1QS4. Hydrogen bonds are shown as dashed lines. | |
The docking of the representative carbohydrazide inhibitors in this series (8i and 8c) to the active site of the monomeric subunit A of 1QS4 was performed using the Auto dock 4.2 programme.27 Compounds 8i and 8c bound well in the active site of 1QS4 with hydrogen bonding through the interaction of the oxygen in the pyran ring, the methoxyl oxygen, and the carbonyl oxygen with proximate active amino acid residues (Glu152, Gln148, Gly149 Asn155, Thr66, His67, Lys159 and Asp64) in the 1QS4 active site (Fig. 4). The close proximity of the docked compounds to the binding site of chicoric acid in the active site of the enzyme could explain the inhibitory potency of these compounds when compared to chicoric acid.
Fig. 4 highlighted an interesting comparison between the two hydrazides chosen: the two ligands (8c and 8i) bind in slightly different orientations, depending on the location of the chloro substituent. In each case the ligand was centred close to Asn155, with the ring bearing the chloro group closest to Gln148. The result is that the particular binding mode relative to the metal ions and the hydrophobic pocket changes depending on the substituents.
Experimental
Materials and methods
All solvents were chemically pure or pre-purified and freshly distilled before use. Solvents were dried by standard procedures prescribed in Vogel.20 The reagents were of analytical grade as supplied by Sigma Aldrich or Fluka. Reactions were monitored by thin layer chromatography (TLC) on Merck pre-coated silica GF254 plates. Melting points (uncorrected) were determined using hot stage apparatus. 1H and 13C-NMR spectra were recorded on a Bruker Avance 600 MHz spectrometer at 298 K, and were calibrated using the solvent signals; chemical shifts are reported in ppm, and coupling constants are given in Hertz (Hz). High-resolution mass spectra (HRMS): electrospray ionisation accurate mass measurements were recorded in positive mode (or negative mode where specified) on a Waters API Q-TOF Ultima spectrometer with an ESI† source.
Synthetic procedures
General procedure for synthesis of coumarin hydrazides 8a–8t28.
To a stirred solution of the appropriate 2-hydroxybenzohydrazide (1.25 mmol) in 4 mL of water, was added the appropriate solution of 2-oxo-2H-chromene-3-carbonyl chlorides 4a (1.25 mmol) in 6 mL of toluene. Thereafter, 6 mL of a saturated aqueous solution of Na2CO3 was added to maintain pH ∼8, and the mixture was stirred for 4 h and left overnight. After the completion of the reaction as monitored by TLC, the precipitate was filtered off, washed with water and air-dried. The resultant crude material was recrystallized from ethanol to afford the corresponding coumarin hydrazides derivatives 8a–8t.
N′-(2-Hydroxybenzoyl)-2-oxo-2H-chromene-3-carbohydrazide 8a28.
Yellow solid. Yield (0.280 g, 69%), m.p. >300 °C; [HRMS: m/z calculated for C17H13N2O5 (MH+) 325.0824. Found 325.0819]; νmax/cm−1 3391 and 3280 (2 × NH), 2700–3570 (br, OH), 1696 and 1608 (C
O); δH (300 MHz, DMSO-d6) 11.91 (1H, s, NH), 8.88 (1H, s, Ar–H), 8.00 (1H, dd, J = 7.8 Hz, 1.4 Hz, Ar–H), 7.80–7.69 (2H, m, Ar–H), 7.52 (1H, d, J = 8.3 Hz, Ar–H), 7.44 (1H, t, J = 7.5 Hz, Ar–H), 7.14–7.08 (1H, m, Ar–H), 6.70–6.64 (2H, overlapping m, Ar–H); δC (75 MHz, DMSO-d6) 164.4, 160.4 and 160.2 (C
O), 153.6 (Ar–C), 153.4 (Ar–C), 145.9 (Ar–C), 137.8 (Ar–C), 133.5 (Ar–C), 130.0 (Ar–C), 129.9 (Ar–C), 128.2 (Ar–C), 125.1 (Ar–C), 120.8 (Ar–C), 119.5 (Ar–C), 118.9 (Ar–C), 116.5 (Ar–C) and 116.2 (Ar–C).
6-Bromo-N′-(2-hydroxybenzoyl)-2-oxo-2H-chromene-3-carbohydrazide 8b.
Yellow solid (0.220 g, 44%), m.p. >300 °C; [HRMS (negative mode): m/z calculated for C17H10N2O5Br (M − H) 400.9773. Found 400.9774]; νmax/cm−1 3038 and 3244 (2 × NH), 2835–3306 (br, OH), 1685 and 1545 (C
O); δH (600 MHz, DMSO-d6) 15.15 (1H, s, NH), 11.91 (1H, s, NH), 8.83 (1H, s, Ar–H), 8.25 (1H, s, Ar–H), 7.85–7.76 (2H, overlapping m, Ar–H), 7.48 (1H, d, J = 8.1 Hz, Ar–H), 7.09 (1H, s, Ar–H), 6.66–6.67 (2H, overlapping m, Ar–H); δC (150 MHz, DMSO-d6) 164.6, 160.2 and 160.0 (C
O), 153.0 (Ar–C), 152.7 (Ar–C), 144.6 (Ar–C), 135.6 (Ar–C), 131.8 (Ar–C), 129.8 (Ar–C), 128.2 (Ar–C), 121.0 (Ar–C), 121.0 (Ar–C), 120.6, (Ar–C), 118.4 (Ar–C), 116.6 (Ar–C), 116.4 (Ar–C) and 116.1 (Ar–C).
6-Chloro-N′-(2-hydroxybenzoyl)-2-oxo-2H-chromene-3-carbohydrazide 8c.
Yellow solid (0.210 g, 48%), m.p. >300 °C; [HRMS (negative mode): m/z calculated for C17H10N2O5Cl (M − H) 357.0278. Found 357.0268]; νmax/cm−1 3250 and 3040 (2 × NH), 3300–2820 (br, OH) and 1685, 1545 (C
O); δH (600 MHz, DMSO-d6) 15.20 (1H, s, NH), 11.93 (1H, s, NH), 8.85 (1H, s, Ar–H), 8.15 (1H, d, J = 2.5 Hz, Ar–H), 7.75 (2H, t, J = 9.0 Hz, Ar–H), 7.55 (1H, d, J = 8.8 Hz, Ar–H), 7.08 (1H, t, J = 7.6 Hz, Ar–H), 6.67–6.64 (2H, overlapping m, Ar–H); δC (150 MHz, DMSO-d6) 164.5, 160.1 and 160.0 (C
O), 152.8 (Ar–C), 152.2 (Ar–C), 144.6 (Ar–C), 132.8 (Ar–C), 129.7 (Ar–C), 128.8 (Ar–C), 128.7 (Ar–C), 128.1 (Ar–C), 121.0 (Ar–C), 120.7 (Ar–C), 120.4 (Ar–C), 118.1 (Ar–C), 116.5 (Ar–C) and 116.1 (Ar–C).
N′-(2-Hydroxybenzoyl)-8-methoxy-2-oxo-2H-chromene-3-carbohydrazide 8d.
Yellow solid (0.24 g, 57%), m.p. >300 °C; [HRMS: m/z calculated for C18H15N2O6 (MH+) 355.0930. Found 355.0915]; νmax/cm−1 3290 (overlapping OH and NH), 3620–2740 (br, OH), and 1709 and 1608 (C
O); δH (600 MHz, DMSO-d6) 11.94 (1H, s, NH), 8.81 (1H, s, Ar–H), 7.79 (1H, d, J = 7.4 Hz, Ar–H), 7.48 (1H, d, J = 7.1 Hz, Ar–H), 7.40–7.31 (2H, m, Ar–H), 7.12 (1H, t, J = 7.0 Hz, Ar–H), 6.73–6.67 (2H, overlapping m, Ar–H), 3.93 (3H, s, OCH3); δC (150 MHz, DMSO-d6) 164.7, 160.4 and 160.3 (C
O), 153.8 (Ar–C), 146.5 (Ar–C), 146.4 (Ar–C), 143.0 (Ar–C), 130.2 (Ar–C), 128.4 (Ar–C), 125.3 (Ar–C), 121.1 (Ar–C), 120.7 (Ar–C), 119.5 (2 × Ar–C), 116.8 (Ar–C), 116.5 (Ar–C), 115.7 (Ar–C) and 56.4 (OCH3).
N′-(2-Hydroxybenzoyl)-8-ethoxy-2-oxo-2H-chromene-3-carbohydrazide 8e.
Yellow solid (0.260 g, 60%), m.p. >300 °C; [HRMS: m/z calculated for C19H17N2O6 (MH+) 369.1087. Found 369.1073]; νmax/cm−1 3471 and 3276 (2 × NH), 3610–2770 (br, OH) and 1709 and 1608 (C
O); δH (600 MHz, DMSO-d6) 8.79 (1H, s, Ar–H), 7.80 (1H, d, J = 7.3 Hz, Ar–H), 7.46 (1H, d, J = 6.9 Hz, Ar–H), 7.37–7.29 (2H, m, Ar–H), 7.15 (1H, t, J = 7.2 Hz, Ar–H), 6.78–6.70 (2H, overlapping m, Ar–H), 4.17 (2H, q, J = 6.6 Hz, –OCH2CH3), 1.41 (3H, t, J = 6.6 Hz,–OCH2CH3); δC (150 MHz, DMSO-d6) 164.5, 160.4 and 160.3 (C
O), 154.4 (Ar–C), 146.9 (Ar–C), 145.8 (Ar–C), 143.2 (Ar–C), 130.8 (Ar–C), 128.7 (Ar–C), 125.5 (Ar–C), 121.2 (Ar–C), 120.2 (Ar–C), 119.6 (Ar–C), 119.3 (Ar–C), 117.2 (Ar–C), 116.7 (Ar–C) and 116.7 (Ar–C), 64.9 (OCH2) and 15.0 (CH3).
N′-(5-Chloro-2-hydroxybenzoyl)-2-oxo-2H-chromene-3-carbohydrazide 8f.
Yellow solid (0.194 g, 43%), m.p. >300 °C; [HRMS: (negative mode) m/z calculated for C17H10N2O5Cl (M-H) 357.0278. Found 357.0287]; νmax/cm−1 3392 and 3278 (2 × NH), 3610–2700 (br, OH), 1704 and 1612 (C
O); δH (600 MHz, DMSO-d6) 11.82 (1H, s, NH), 8.88 (1H, s, Ar–H), 7.99 (1H, d, J = 7.5 Hz, Ar–H), 7.77–7.70 (2H, m, Ar–H), 7.51 (1H, d, J = 8.3 Hz, Ar–H), 7.44 (1H, t, J = 7.4 Hz, Ar–H), 7.12 (1H, d, J = 10.8 Hz, Ar–H), 6.72 (1H, d, J = 8.6 Hz, Ar–H); δC (150 MHz, DMSO-d6) 162.9, 160.5 and 159.5 (C
O), 154.0 (Ar–C), 153.8 (Ar–C), 146.5 (Ar–C), 133.8 (Ar–C), 130.2 (Ar–C), 129.8 (Ar–C), 127.4 (Ar–C), 125.3 (Ar–C), 121.9 (Ar–C), 120.0 (Ar–C), 119.1 (Ar–C), 118.9 (Ar–C), 118.5 (Ar–C) and 116.3 (Ar–C).
6-Bromo-N′-(5-chloro-2-hydroxybenzoyl)-2-oxo-2H-chromene-3-carbohydrazide 8g.
Yellow solid (0.210 g, 38%), m.p. >300 °C; [HRMS: (negative mode) m/z calculated for C17H9N2O5BrCl (M − H) 434.9383. Found 434.9381]; νmax/cm−1 3316 and 3043 (2 × NH), 3370–2990 (br, OH) and 1698 and 1547 (C
O); δH (600 MHz, DMSO-d6) 15.40 (1H, s, NH), 11.83 (1H, s, NH), 8.83 (1H, s, Ar–H), 8.26 (1H, s, Ar–H), 7.85 (1H, d, J = 7.9 Hz, Ar–H), 7.70 (1H, s, Ar–H), 7.48 (1H, d, J = 8.7 Hz Ar–H), 7.09 (1H, d, J = 8.3 Hz, Ar–H), 6.69 (1H, J = 8.5 Hz, Ar–H); δC (150 MHz, DMSO-d6) 163.1, 160.0, 159.5 (C
O), 153.3 (Ar–C), 152.7 (Ar–C), 144.9 (Ar–C), 135.8 (Ar–C), 131.9 (Ar–C), 129.4 (Ar–C), 127.3 (Ar–C), 122.2 (Ar–C), 120.8 (Ar–C), 120.4 (Ar–C), 119.8 (Ar–C), 118.4 (Ar–C), 118.2 (Ar–C) and 116.7 (Ar–C).
6-Chloro-N′-(5-chloro-2-hydroxybenzoyl)-2-oxo-2H-chromene-3-carbohydrazide 8h.
Yellow solid (0.244 g, 50%), m.p. >300 °C; [HRMS: (negative mode) m/z calculated C17H9N2O5Cl2 for (M − H) 390.9689. Found 390.9691]; νmax/cm−1 3291 and 3046 (2 × NH), 3600–2825 (br, OH), and 1709 and 1550 (C
O); δH (600 MHz, DMSO-d6) 11.85 (1H, s, N–H), 8.84 (1H, s, Ar–H), 8.13 (1H, s, Ar–H), 7.76–7.68 (2H, m, Ar–H), 7.55 (1H, d, J = 8.8 Hz, Ar–H), 7.10 (1H, d, J = 8.5 Hz, Ar–H), 6.69 (1H, d, J = 8.6 Hz, Ar–H); δC (150 MHz, DMSO-d6) 163.1, 160.1 and 159.5 (C
O), 153.3 (Ar–C), 152.4 (Ar–C), 145.0 (Ar–C), 133.1 (Ar–C), 129.5 (Ar–C), 130.0 (Ar–C), 128.9 (Ar–C), 127.3 (Ar–C), 122.3 (Ar–C), 120.4 (Ar–C), 120.4 (Ar–C), 119.9 (Ar–C), 118.3 (Ar–C) and 118.3 (Ar–C).
N′-(5-Chloro-2-hydroxybenzoyl)-8-methoxy-2-oxo-2H-chromene-3-carbohydrazide 8i.
Yellow solid (0.324 g, 67%), m.p. >300 °C; [HRMS: (negative mode) m/z calculated for C18H12N2O6Cl (M − H) 387.0384. Found 387.0377]; νmax/cm−1 3565 and 3230 (2 × NH), 3600–2825 (br, OH) and 1693 (ester C
O), 1608 and 1574 (amide C
O); δH (300 MHz, DMSO-d6) 11.88 (1H, s, N–H), 8.84 (1H, s, Ar–H), 7.71 (1H, s, Ar–H), 7.52 (1H, d, J = 6.6 Hz, Ar–H), 7.38 (2H, t, J = 8.5 Hz, Ar–H), 7.10 (1H, d, J = 10.3 Hz, Ar–H), 6.70 (1H, d, J = 8.5 Hz, Ar–H), 3.94 (3H, s, OCH3); δC (75 MHz, DMSO-d6) 163.0, 160.2 and 159.6 (C
O), 153.6 (Ar–C), 146.4 (Ar–C), 146.36 (Ar–C), 142.3 (Ar–C), 129.4 (Ar–C), 127.2 (Ar–C), 125.1 (Ar–C), 122.2 (Ar–C), 121.0 (Ar–C), 119.8 (Ar–C), 119.4 (Ar–C), 119.3 (Ar–C), 118.3 (Ar–C), 115.6 (Ar–C) and 56.3 (OCH3).
N′-(5-Chloro-2-hydroxybenzoyl)-8-ethoxy-2-oxo-2H-chromene-3-carbohydrazide 8j.
Yellow solid (0.29 g, 58%), m.p. >300 °C; [HRMS: m/z calculated for C19H16N2O6Cl (MH+) 403.0697. Found 403.0700]; νmax/cm−1 3443 and 3261 (2 × NH), 3610–2700 (br, OH) and 1694 (ester C
O), 1606, 1575 (amide C
O); δH (600 MHz, DMSO-d6) 15.51 (1H, s, NH), 11.87 (1H, s, NH), 8.84 (1H, s, Ar–H), 7.70 (1H, s, Ar–H), 7.51 (1H, d, J = 6.8 Hz, Ar–H), 7.38 (1H, d, J = 7.5 Hz, Ar–H), 7.34 (1H, d, J = 7.7 Hz, Ar–H), 7.09 (1H, d, J = 7.7 Hz, Ar–H), 6.69 (1H, d, J = 8.20 Hz, Ar–H), 4.20 (2H, q, J = 6.8 Hz, OCH2), 1.43 (3H, t, J = 6.9 Hz, CH3); δC (150 MHz, DMSO-d6) 162.9, 160.2, and 159.6 (C
O), 153.5 (Ar–C), 146.4 (Ar–C), 145.6 (Ar–C), 143.0 (Ar–C), 129.3 (Ar–C), 127.2 (Ar–C), 125.1 (Ar–C), 122.3 (Ar–C), 120.9 (Ar–C), 119.7 (Ar–C), 119.5 (Ar–C), 119.3 (Ar–C), 118.2 (Ar–C), 116.3 (Ar–C), 64.6 (OCH2) and 14.70 (CH3).
N′-(2-Hydroxy-5-methoxybenzoyl)-2-oxo-2H-chromene-3-carbohydrazide 8k.
Yellow solid (0.35 g, 79%), m.p. >300 °C; [HRMS: m/z calculated for C18H15N2O6 (MH+) 355.0930. Found 355.0927]; νmax/cm−1 3550–2700 (overlapping NH and OH) and 1704 (ester C
O), 1609 and 1567 (amide C
O) δH (300 MHz, DMSO-d6) 11.97 (1H, s, NH), 8.79 (1H, s, Ar–H), 7.89 (1H, s, Ar–H), 7.70 (1H, s, Ar–H), 7.47–7.36 (3H, overlapping m, Ar–H), 6.75–6.67 (2H, overlapping m, Ar–H), 3.66 (3H, s, OCH3); δC (75 MHz, DMSO-d6) 165.0, 161.3 and 155.0 (C
O), 154.2 (Ar–C), 154.0 (Ar–C), 151.2 (Ar–C), 147.2 (Ar–C), 133.6 (Ar–C), 130.7 (Ar–C), 126.2 (Ar–C), 121.0 (Ar–C), 119.4 (Ar–C), 119.2.(Ar–C), 117.8 (Ar–C), 117.6 (Ar–C), 116.9 (Ar–C), 112.8 (Ar–C) and 56.2 (OCH3).
6-Bromo-N′-(2-hydroxy-5-methoxybenzoyl)-2-oxo-2H-chromene-3-carbohydrazide 8l.
Yellow solid (0.41, 76%), m.p. >300 °C [HRMS: (negative mode) m/z calculated for C18H12N2O6Br (M − H) 430.9879. Found 430.9870]; νmax/cm−1 3550–2700 (overlapping OH and NH) and 1703 (ester C
O), 1611 (amide C
O); δH (600 MHz, DMSO-d6) 11.87 (1H, s, NH), 8.82 (1H, s, Ar–H), 8.26 (1H, s, Ar–H), 7.85 (1H, s, Ar–H), 7.48 (1H, s, Ar–H), 7.36 (1H, s, Ar–H), 6.74 (1H, s, Ar–H), 6.65 (1H, s, Ar–H), 3.68 (3H, s, OCH3); δC (150 MHz, DMSO-d6) 164.3, 160.0 and 153.6 (C
O), 153.4 (Ar–C), 152.7 (Ar–C), 150.8 (Ar–C), 144.8 (Ar–C), 135.8 (Ar–C), 131.9 (Ar–C), 120.9 (Ar–C), 120.8 (Ar–C), 120.6 (Ar–C), 118.5 (Ar–C), 116.9 (Ar–C), 116.7 (Ar–C), 116.6 (Ar–C), 112.1 (Ar–C) and 55.5 (OCH3).
6-Chloro-N′-(2-hydroxy-5-methoxybenzoyl)-2-oxo-2H-chromene-3-carbohydrazide 8m.
Yellow solid (0.40, 83%), m.p. >300 °C; [HRMS: m/z calculated for C18H14N2O6Cl (MH+) 389.0540 found 389.0539]; νmax/cm−1 3510–2850 (overlapping OH and NH), 1703 and 1609 (C
O); δH (600 MHz, DMSO-d6) 11.91 (1H, s, N–H), 8.81 (1H, s, Ar–H), 8.10 (1H, s, Ar–H), 7.73 (1H, s, Ar–H), 7.57 (1H, s, Ar–H), 7.53 (1H, s, Ar–H), 6.73 (1H, s, Ar–H), 6.64 (1H, s, Ar–H) and 4.10 (3H, s, OCH3); δC (150 MHz, DMSO-d6) 164.6, 160.3 and 153.8 (C
O), 153.5 (Ar–C), 152.50 (Ar–C), 150.9 (Ar–C), 145.1.(Ar–C), 133.2 (Ar–C), 129.1 (Ar–C), 121.2 (Ar–C), 121.0 (Ar–C), 120.7 (Ar–C), 120.5 (Ar–C), 118.4 (Ar–C), 111.0 (Ar–C), 116.8 (Ar–C), 112.3 (Ar–C) and 55.6 (OCH3).
N′-(2-hydroxy-5-methoxybenzoyl)-8-methoxy-2-oxo-2H-chromene-3-carbohydrazide 8n.
Yellow solid (0.299, 47%), m.p. >300 °C; [HRMS: m/z calculated for C19H17N2O7 (MH+) 385.1036. Found 385.1040]; νmax/cm−1 3560–2770 (overlapping OH and NH), 1701 (ester C
O), 1609 and 1561 (amide C
O); δH (600 MHz, DMSO-d6) 11.81 (1H, s, N–H), 8.83 (1H, s, Ar–H), 7.52 (1H, m, Ar–H), 7.34–7.41 (3H, m, Ar–H), 6.77 (1H, dd, J = 8.7, 3.3 Hz, Ar–H), 6.67 (1H, d, J = 8.7 Hz, Ar–H), 3.94 (3H, s, OCH3), 3.69 (3H, s, OCH3). δC (150 MHz, DMSO-d6) 164.1, 160.0 and 154.1 (C
O), 153.5 (Ar–C), 150.8 (Ar–C), 146.4 (Ar–C), 143.0 (Ar–C), 125.1 (Ar–C), 121.0 (Ar–C), 120.2 (2 × Ar–C), 119.4 (Ar–C), 117.1 (Ar–C), 116.7 (Ar–C), 115.6 (Ar–C), 112.1 (2 × Ar–C), 56.3 (OCH3) and 55.5 (OCH3).
8-Ethoxy-N′-(2-hydroxy-5-methoxybenzoyl)-2-oxo-2H-chromene-3-carbohydrazide 8o.
Yellow solid (0.253 g, 51%), m.p. >300 °C [HRMS: m/z calculated for C20H19N2O7 (MH+) 399.1184. Found 399.1144]; νmax/cm−1 3468 and 3259 (2 × NH), 3600–2780 (br, OH), 1688 (ester C
O), 1607 and 1582 (amide C
O); δH (600 MHz, DMSO-d6) 11.84 (1H, s, NH), 8.82 (1H, s, Ar–H), 7.49 (1H, d, J = 7.4 Hz, Ar–H), 7.39–7.37 (2H, m, Ar–H), 7.33 (1H, t, J = 7.9 Hz, Ar–H), 6.76 (1H, dd, J = 8.7, 3.2 Hz, Ar–H), 6.66 (1H, d, J = 8.7 Hz, Ar–H), 4.20 (2H, q, J = 6.9 Hz, OCH2), 3.69 (3H, s, OCH3), 1.43 (3H, t, J = 6.9 Hz, CH3); δC (150 MHz, DMSO-d6) 164.1, 160.1 and 153.9 (C
O), 153.6 (Ar–C), 150.7 (Ar–C), 146.3 (Ar–C), 145.6 (Ar–C), 143.0 (Ar–C), 125.1 (Ar–C), 120.9 (Ar–C), 120.5, (Ar–C), 119.5 (Ar–C), 119.4 (Ar–C), 116.9 (Ar–C), 116.6 (Ar–C), 116.4 (Ar–C), 112.1 (Ar–C), 64.6 (OCH2), 55.4 (OCH3) and 14.7 (CH3).
N′-(2-Hydroxy-3-methoxybenzoyl)-2-oxo-2H-chromene-3-carbohydrazide 8p.
Yellow solid (0.35 g, 79%), m.p. >300 °C, [HRMS: m/z calculated for C18H15N2O6 (MH+) 355.0930. Found 355.0931]; νmax/cm−1 3270–3053 (2 × NH), 3570–2840 (br, OH) and 1700 (ester C
O), 1609 and 1567 (amide C
O); δH (600 MHz, DMSO-d6) 15.50 (1H, s, OH), 11.88 (1H, s, N–H), 8.89 (1H, s, Ar–H), 8.00 (1H, s, Ar–H), 7.73 (1H, s, Ar–H), 7.52 (1H, s, Ar–H), 7.44–7.39 (2H, overlapping, Ar–H), 6.79 (1H, s, Ar–H), 6.53 (1H, s, Ar–H), 3.73 (3H, s, OCH3); δC (150 MHz, DMSO-d6) 164.6, 160.4 and 153.6 (C
O), 153.5 (Ar–C), 152.1 (Ar–C), 148.6 (Ar–C), 146.0 (Ar–C), 133.6 (Ar–C), 130.0 (Ar–C), 125.1 (Ar–C), 120.4 (Ar–C), 120.2 (Ar–C), 119.3 (Ar–C), 118.9 (Ar–C), 116.1 (Ar–C), 114.5 (Ar–C), 112.7 (Ar–C) and 55.7 (OCH3).
6-Bromo-N′-(2-hydroxy-3-methoxybenzoyl)-2-oxo-2H-chromene-3-carbohydrazide 8q.
Yellow solid (0.41, 76%), m.p. >300 °C; [HRMS: m/z for C18H14N2O6Br (MH+) 433.2100. Found 433.0196]; νmax/cm−1 3464 and 3282 (2 × NH) and 3625–3625 (br, OH), 1703 (ester C
O), 1616 and 1562 (amide C
O); δH (600 MHz, DMSO-d6) 15.53 (1H, s, OH), 11.89 (1H, s, N–H), 8.85 (1H, s, Ar–H), 8.28 (1H, s, Ar–H), 7.86 (1H, d, J = 7.9 Hz, Ar–H), 7.49 (1H, d, J = 8.0 Hz, Ar–H), 7.39 (1H, d, J = 6.8 Hz, Ar–H), 6.79 (1H, d, J = 6.3 Hz, Ar–H), 6.53 (1H, s, Ar–H), 3.72 (3H, s, CH3); δC (150 MHz, DMSO-d6) 164.7, 160.0 and 153.0 (C
O), 152.6 (Ar–C), 151.7 (Ar–C), 148.4 (Ar–C), 144.6 (Ar–C), 135.6 (Ar–C), 131.8 (Ar–C), 129.0 (Ar–C), 128.3 (Ar–C), 120.8 (Ar–C), 120.5 (Ar–C), 118.4 (Ar–C), 116.6 (Ar–C), 114.7 (Ar–C), 112.8 (Ar–C) and 55.7 (OCH3).
6-Chloro-N′-(2-hydroxy-3-methoxybenzoyl)-2-oxo-2H-chromene-3-carbohydrazide 8r.
Yellow solid (0.40, 83%), m.p. >300 °C; [HRMS: m/z calculated C18H14N2O6Cl for 389.0540 (MH+). Found 389.0537]; νmax/cm−1 3461, 3306 (2 × NH), 3600–2830 (br, OH) and 1710 (ester C
O), 1613 and 1561 (amide C
O); δH (600 MHz, DMSO-d6) 15.53 (1H, s, OH), 11.90 (1H, s, NH), 8.85 (1H, s, Ar–H), 8.15 (1H, s, Ar–H), 7.74 (1H, s, Ar–H), 7.55 (1H, d, J = 6.6 Hz, Ar–H), 7.39 (1H, d, J = 5.0 Hz, Ar–H), 6.79 (1H, s, Ar–H), 6.53 (1H, s, Ar–H), 3.72 (3H, s, OCH3); δC (150 MHz, DMSO-d6) 164.7, 160.0 and 153.0 (C
O), 152.2 (Ar–C), 151.8 (Ar–C), 148.54 (Ar–C), 148.47 (Ar–C), 144.7 (Ar–C), 132.9 (Ar–C), 128.84 (Ar–C), 128.76 (Ar–C), 120.5 (Ar–C), 120.4 (Ar–C), 120.3 (Ar–C), 118.1 (Ar–C), 114.7 (Ar–C), 112.7 (Ar–C) and 55.7 (OCH3).
N′-(2-Hydroxy-3-methoxybenzoyl)-8-methoxy-2-oxo-2H-chromene-3-carbohydrazide 8s.
Yellow solid (0.299 g, 47%), m.p. >300 °C; [HRMS: m/z calculated for C19H17N2O7 (MH+) 385.1036. Found 385.1036]; νmax/cm−1 3390 and 3289 (2 × NH), 3625–2780 (br, OH) and 1701 (ester C
O), 1609 and 1575 (amide C
O); δH (600 MHz, DMSO-d6) 15.59 (1H, s, OH), 11.89 (1H, s, NH), 8.84 (1H, s, Ar–H), 7.52 (1H, s, Ar–H), 7.38 (3H, m, Ar–H), 6.80 (1H, s, Ar–H), 6.53 (1H, s, Ar–H), 3.94 (3H, s, OCH3), 3.72 (3H, s, OCH3); δC (150 MHz, DMSO-d6) 164.6, 160.1 and 153.4 (C
O), 152.1 (Ar–C), 148.6 (Ar–C), 146.3 (Ar–C), 146.2 (Ar–C), 142.9 (Ar–C), 125.1 (Ar–C), 121.0 (Ar–C), 120.4 (Ar–C), 120.3 (Ar–C), 119.4 (2 × Ar–C), 115.5 (Ar–C), 114.5 (Ar–C), 112.7 (Ar–C), 56.2 (OCH3), 55.7 (OCH3).
8-Ethoxy-N′-(2-hydroxy-3-methoxybenzoyl)-2-oxo-2H-chromene-3-carbohydrazide 8t.
Yellow solid (0.253 g, 51%), m.p. >300 °C; [HRMS: m/z calculated for C20H19N2O7 (MH+) 399.1192. Found 399.1189]; νmax/cm−1 3615 and 3284 (2 × NH), 3620–2830 (br, OH), 1699 (ester C
O), 1610 and 1577 (amide C
O); δH (600 MHz, DMSO-d6) 15.59 (1H, s, OH), 11.89 (1H, s, NH), 8.84 (1H, s, Ar–H), 7.51 (1H, s, Ar–H), 7.36 (3H, m, Ar–H), 6.79 (1H, s, Ar–H), 6.53 (1H, s, Ar–H), 4.20 (2H, s, OCH2), 3.72 (3H, s, OCH3), 1.43 (3H, s, OCH2CH3); δC (150 MHz, DMSO-d6) 164.6, 160.2 and 153.3 (C
O), 151.9 (Ar–C), 148.5 (Ar–C), 146.2 (Ar–C), 145.6 (Ar–C), 143.0 (Ar–C), 125.0 (Ar–C), 120.9 (Ar–C), 120.5 (Ar–C), 120.4 (Ar–C), 119.5 (Ar–C), 119.4 (Ar–C), 116.2 (Ar–C), 114.5 (Ar–C), 112.7 (Ar–C), 64.5 (OCH2), 55.7 (OCH3) and 14.7 (CH3).
Biological assays
In vitro IN assays.
The HIV-1 IN strand transfer inhibition assay was adapted from previously described methods.8 Briefly, 20 nM double-stranded biotinylated donor DNA (5′-5biotin-TEG/ACCCTTTTAGTCAGTGTGGAAAATCTCTAGCA-3′ annealed to 5′-ACTGCTAGAGATTTTCCACACTGACTAAAAG-3′) was immobilized in wells of streptavidin-coated 96-well microtiter plates (R&D Systems, USA). Following incubation at room temperature for 40 minutes and a stringent wash step, 5 μg ml−1 purified recombinant HIV-1 subtype C IN in IN buffer 1 (50 mM NaCl, 25 mM Hepes, 25 mM MnCl2, 5 mM β-mercaptoethanol, 50 μg ml−1 BSA, pH 7.5) was added to individual wells. Test compounds and Raltegravir were added to individual wells to a final concentration of 20 μM. Recombinant HIV-1 subtype C IN was assembled onto the pre-processed donor DNA through incubation for 45 minutes at room temperature. Strand transfer reaction was initiated through the addition of 10 nM (final concentration) double-stranded FITC-labelled target DNA (5′-TGACCAAGGGCTAATTCACT/36-FAM/-3′ annealed to 5′-AGTGAATTAGCCCTTGGTCA-/36-FAM/-3′) in IN buffer 2 (same as buffer 1, except 25 mM MnCl2 replaced with 2.5 mM MgCl2). After an incubation period of 60 minutes at 37 °C, the plates were washed using PBS containing 0.05% Tween 20 and 0.01% BSA, followed by the addition of peroxidase-conjugated sheep anti-FITC antibody (Thermo Scientific, USA) diluted 1:1000 in the same PBS buffer. Finally, the plates were washed and peroxidase substrate (SureBlue Reserve™, KPL, USA) was added to allow for detection at 620 nm using a Synergy MX (BioTek®) plate reader. Absorbance values were converted to% enzyme activity relative to the readings obtained from control wells (enzyme without inhibitor).
Cell toxicity assays.
To determine the activity of the test compounds against human cervix adenocarcinoma (HeLa) cells, the cells were incubated with the compounds at a final concentration of 25 μM for a period of 48 hours in 96-well plates. The cells were seeded at 6.7 × 104 cells per well in DMEM supplemented with 10% foetal bovine serum and penicillin/streptomycin/amphotericin B and incubated at 37 °C in a 5% CO2 incubator for 24 hours, followed by compound addition and an additional 48 hour incubation. The numbers of cells surviving compound exposure were determined by adding 20 μL per well resazurin toxicology reagent (Sigma-Aldrich) and measuring fluorescence (Exc 560 nm, Em 590 nm) in a Spectramax M3 plate reader after an additional 2–4 hour incubation. Percentage cell viability in wells exposed to test compounds was calculated relative to the average fluorescence readings in control wells containing no compound.
Molecular Modelling
The structure and the molecular geometry of the synthesized compounds were constructed using the ChemBio3D Ultra Version 14 molecular modelling program (CambridgeSoft Corporation, Cambridge, MA, USA). The X-ray crystal structure of the HIV IN enzymes was obtained from Protein Data Bank (PDB code: 1QS4). The Discovery Studio Visualizer 3.5 (ref. 29) was used to prepare the protein for docking. All the bound water molecules and the original ligand were removed from the 1QS4 and polar hydrogen was added and non-polar hydrogen was merged followed by the addition of Gasteiger charges and assignment of AD4 atoms. Autodock 4.2 software27 were employed in the preparation of the pdb and pdbqt files of the ligand and the protein. The active site of the 1QS4 was centered with the grid box dimensions −19.422 × 32.402 × 66.325 Å and a grid spacing of 0.5 Å. Mapping of the protein active site and the surrounding residues were done using Autogrid. The procedure for docking was performed using the Lamarckian Genetic Algorithm, and the geometry of the docked complex was view and analyzed using Discovery Studio 4.0.22
Conclusions
The replacement of one salicylic moiety with a coumaryl moiety has been shown here to significantly improve the cytotoxicity of the resulting hydrazides. In addition, inhibition of HIV-1 IN has been demonstrated, with nanomolar efficiency for compounds with either hydrogen or chlorine on the salicyl moiety and halogens or alkoxy groups on the coumarin. A docking study suggests that the orientation within the active site positions the acyl hydrazide at the metal centres and one of the aromatic rings directed toward the hydrophobic pocket.
In summary, we have successfully designed, synthesised and evaluated a series of carbohydrazide derivatives as novel HIV-1 inhibitors. However, the structure of these compounds could be optimised in the development of new drug candidates for IN inhibition. Such optimization may include the incorporation of fluoro groups (common to other approved inhibitors), as well as the exploration of specific modes of toxicity.
Conflicts of interest
There are no conflicts to declare.
Acknowledgements
This research project was funded by the South African Medical Research Council (MRC) with funds from the National Treasury under its Economic Competitiveness and Support Package. O. J. Jesumoroti is supported by the Organization for Women in Science in the Developing World.
Notes and references
- J. d'Angelo, J. F. Mouscadet, D. Desmaële, F. Zouhiri and H. Leh, Pathol. Biol., 2001, 49, 237–246 CrossRef.
- F. Barre-Sinoussi, J. C. Chermann, F. Rey, M. T. Nugeyre, S. Chamaret, J. Gruest, C. Dauguet, C. Axler-Blin, F. Vezinet-Brun, C. Rouzioux, W. Rozenbaum and L. Montagnier, Science, 1983, 220, 868–871 CrossRef CAS.
- A. Pani, A. G. Loi, M. Mura, T. Marceddu, P. La Colla and M. E. Marongiu, Curr. Drug Targets: Infect. Disord., 2002, 2, 17–32 CrossRef CAS.
- G. Pais, X. Zhang, C. Marchand, N. Neamati, K. Cowansage, E. S. Svarovskaia, V. K. Pathak, Y. Tang, M. Nicklaus, Y. Pommier and T. J. Burke, J. Med. Chem., 2002, 45, 3184–3194 CrossRef CAS PubMed.
- Y. Mehellou and E. De Clercq, J. Med. Chem., 2010, 53, 521 CrossRef CAS PubMed.
- K. B. Ingale and M. S. Bhatia, Antiviral Chem. Chemother., 2011, 22, 95–105 CrossRef CAS PubMed.
- G. Meintjes, M. A. Moorhouse, S. Carmona, N. Davies, S. Dlamini, C. Van Vuuren, T. Manzini, M. Mathe, Y. Moosa, J. Nash, J. Nel, Y. Pakade, J. Woods, G. Van Zyl, F. Conradie and F. Venter, South. Afr. J. HIV Med., 2017, 18, e24 Search PubMed.
- Y. Goldgur, F. Dyda, A. B. Hickman, T. M. Jenkins, R. Craigie and D. R. Davies, Proc. Natl. Acad. Sci. U. S. A., 1998, 95, 9150–9154 CrossRef CAS.
- J. A. Grobler, K. Stillmock, B. Hu, M. Witmer, P. Felock, A. S. Espeseth, A. Wolfe, M. Egbertson, M. Bourgeois, J. Melamed, J. S. Wai, S. Young, J. Vacca and D. J. Hazuda, Proc. Natl. Acad. Sci. U. S. A., 2002, 99, 6661–6666 CrossRef CAS PubMed.
- B. J. Dong and J. Cocohoba, Clin. Ther., 2008, 30, 1747–1765 CrossRef PubMed.
- M. Tsiang, G. S. Jones, J. Goldsmith, A. Mulato, D. Hansen, E. Kan, L. Tsai, R. A. Bam, G. Stepan, K. M. Stray, A. Niedziela-Majka, S. R. Yant, H. Yu, G. Kukolj, T. Cihlar, S. E. Lazerwith, K. L. White and H. Jin, Antimicrob. Agents Chemother., 2016, 60, 7086 CAS.
-
K. Rosa and M. Hoffman, FDA Approves Bictegravir/FTC/TAF Combination HIV Therapy, 2018 Search PubMed.
-
Y. Li, S. Xuan, Y. Feng and A. Yan, Targeting HIV-1 integrase with strand transfer inhibitors, 2015, pp. 435–449 Search PubMed.
-
X. Fan, F. Zhang, R. I. Al-Safi, L. Zeng, Y. Shabaik, B. Debnath, T. W. Sanchez, S. Odde, N. Neamati and Y. LongDesign of HIV-1 integrase inhibitors targeting the catalytic domain as well as its interaction with LEDGF/p75: A scaffold hopping approach using salicylate and catechol groups, 2011, pp. 4935–4952 Search PubMed.
- H. Zhao, N. Neamati, S. Sunder, H. Hong, S. Wang, G. Milne, Y. Pommier and T. J. Burke, J. Med. Chem., 1997, 40, 937–941 CrossRef CAS PubMed.
- N. Neamati, S. Sunder and Y. Pommier, Drug Discovery Today, 1997, 2, 487–498 CrossRef CAS.
- F. Tian, F. Jiang, X. Han, C. Xiang, Y. Ge, J. Li, Y. Zhang, R. Li, X. Ding and Y. Liu, J. Phys. Chem. B, 2010, 114, 14842 CrossRef CAS.
- J. H. Park, S. U. Lee, S. H. Kim, S. Y. Shin, J. Y. Lee, C. Shin, K. H. Yoo and Y. S. Lee, Arch. Pharmacal Res., 2008, 31, 1–5 CrossRef CAS.
- V. Summa, A. Petrocchi, F. Bonelli, B. Crescenzi, M. Donghi, M. Ferrara, F. Fiore, C. Gardelli, O. Gonzalez Paz, D. J. Hazuda, P. Jones, O. Kinzel, R. Laufer, E. Monteagudo, E. Muraglia, E. Nizi, F. Orvieto, P. Pace, G. Pescatore, R. Scarpelli, K. Stillmock, M. V. Witmer and M. Rowley, J. Med. Chem., 2008, 51, 5843–5855 CrossRef CAS PubMed.
- D. E. Polyansky and D. C. Neckers, J. Phys. Chem. A, 2005, 109, 2793–2800 CrossRef CAS PubMed.
- D. J. McColl and X. Chen, Antiviral Res., 2010, 85, 101–118 CrossRef CAS PubMed.
- N. Neamati, H. Hong, J. M. Owen, S. Sunder, H. E. Winslow, J. L. Christensen, H. Zhao, T. J. Burke, G. Milne and Y. Pommier, J. Med. Chem., 1998, 41, 3202–3209 CrossRef CAS PubMed.
-
M. C. Lee, J. Deng, J. M. Briggs and Y. DuanLarge-Scale Conformational Dynamics of the HIV-1 Integrase Core Domain and Its Catalytic Loop Mutants, 2005, pp. 3133–3146 Search PubMed.
- A. L. Perryman, S. Forli, G. M. Morris, C. Burt, Y. Cheng, M. J. Palmer, K. Whitby, J. A. McCammon, C. Phillips and A. J. Olson, J. Mol. Biol., 2010, 397, 600–615 CrossRef CAS PubMed.
- E. F. Healy, J. Sanders, P. J. King and W. E. Robinson, J. Mol. Graphics Modell., 2009, 27, 584–589 CrossRef CAS.
- T. T. Charvat, D. J. Lee, W. E. Robinson and A. R. Chamberlin, Bioorg. Med. Chem., 2006, 14, 4552–4567 CrossRef CAS.
- O. Trott and A. J. Olson, J. Comput. Chem., 2010, 31, 455 CAS.
- A. D. Beldovskaya, G. A. Dushenko, N. I. Vikrishchuk, L. D. Popov, Y. V. Revinskii, I. E. Mikhailov and V. I. Minkin, Russ. J. Org. Chem., 2013, 49, 1861 CrossRef CAS.
-
B. Dassault Systèmes, Discovery Studio Modeling Environment, Release 3.5, 2016, Dassault Systèmes, San Diego, 2015 Search PubMed.
Footnote |
† Electronic supplementary information (ESI) available: NMR and IR spectra and mass spectral data. See DOI: 10.1039/c8md00328a |
|
This journal is © The Royal Society of Chemistry 2019 |
Click here to see how this site uses Cookies. View our privacy policy here.