DOI:
10.1039/C8FO02142B
(Paper)
Food Funct., 2019,
10, 289-295
Interactions of food matrix and dietary components on neonicotinoid bioaccessibility in raw fruit and vegetables
Received
31st October 2018
, Accepted 3rd December 2018
First published on 4th December 2018
Abstract
Humans are frequently exposed to the residues of various neonicotinoids, highlighting the need to understand human exposure through oral ingestion of contaminated foods. In this study, the effects of different food matrices (tomato, cucumber, and carrot) and their interaction with dietary component additives, including proteins and dietary fiber, was investigated. The results showed that the presence of a food matrix had a significant effect on the bioaccessibility of neonicotinoids (imidacloprid, thiamethoxam, acetamiprid, and thiacloprid) in both the gastric and intestinal environments. Neonicotinoids in tomato presented relatively low bioaccessibility, indicating that the daily intake of the tomato can be regarded as being relatively safer. Moreover, the addition of protein or dietary fiber to fruit and vegetables had a marked influence on neonicotinoid bioaccessibility and the effects varied between the different matrices. In particular, the addition of 2.0% dietary fiber significantly reduced the bioaccessibility (18.38–67.91%). Therefore, we recommend that consuming an increased intake of dietary fiber could improve the safety of fruit and vegetables in daily life. The present results can support the identification of suitable food intake conditions for the significant reduction of pesticide residue levels.
Introduction
Food safety has become a major concern over the world. Pesticide residues are a main contributory factor to this concern, posing a dietary intake risk to human health. The hazardous effects of pesticide residues include endocrine disruption, infertility and immune suppression, and carcinogenic and teratogenic effects.1 In most cases, a diet of fruit and vegetables, on average constituting over 30% of the consumer's diet, is the main source of exposure to pesticide residues for the general population.2 Moreover, in comparison to cooked food, the ingestion of fruit and vegetables could result in higher exposure to pesticide residues because of the method of consumption3,4 (that is, raw or semi-processed). Thus, studies on pesticide residues in fruit and vegetables represent one of the “worst” cases with respect to pesticide control in general, especially for raw or semi-processed fruit and vegetables.
Previously, traditional risk assessment used the total chemical concentration (CTotal) for calculation of exposure;5 however, this does not represent the actual degree of the contaminants being ingested by humans. The concept of employing “bioaccessibility” to assess risks associated with contaminants is well acknowledged.6 Bioaccessibility is defined as the fraction of chemicals that is soluble in the gastrointestinal tract of humans and available for absorption.7 Studies of bioaccessibility suggest that the amount of compound absorbed may be less than the level of a contaminant in the soil or food. Thus, bioaccessibility is a critical variable for understanding the risk of pesticides via oral ingestion. However, to date, the bioaccessibility of pesticide residues in food for human consumption is poorly understood.
In an oral ingestion scenario, in vitro gastrointestinal methods were applied to estimate the bioaccessibility of heavy metals,8 persistent organic pollutants (POPs),9,10 and mycotoxins11,12 in various food sources and soils. The bioaccessibility of a chemical or nutrient compound can be affected by molecular interactions between the potential physicochemical conditions and the food matrix.13 Included here are dietary fibers which might be added deliberately for their flavor or health aspects. The influence of digestive conditions, such as gastrointestinal pH, time, and the solid–liquid (S/L) ratio, on the bioaccessibility of certain contaminants has been reported, including pesticides. Our previous investigation on the release of pesticides during simulated human digestion using a static model showed a significant effect of the physicochemical conditions.14,15 It was deduced that the presence of food may influence the release of pesticides from the food matrix. However, so far, we have limited knowledge about how variations in this parameter affect the bioaccessibility of pesticides during simulated human digestion.
In this study, the human gastric and intestinal digestion (SHIME) method (gastric and small intestinal phases) was used to thoroughly evaluate the effect of a range of food (tomato (Lycopersicon esculentum Mill.), cucumber (Cucumis sativus L.), and carrot (Daucus carota L. var. sativa Hoffm.)) matrices on the rate and extent of neonicotinoid (imidacloprid, thiamethoxam, acetamiprid, and thiacloprid) bioaccessibility. In addition, protein and dietary fiber addition was used to investigate the interaction between dietary components and the food matrix.
Materials and methods
Chemicals and pesticide-contaminated samples
Pesticide standards, including imidacloprid (96%), thiamethoxam (97%), acetamiprid (97%), and thiacloprid (98%) were purchased from the ANPEL (ANPEL Laboratory Technologies, China). Standard stock solutions of the neonicotinoids were prepared in methanol. Subsequently, matrix-matched standard solutions were prepared by adding the appropriate stock solution using blank extracts of fruit and vegetable samples.
QuEChERS bulk sodium chloride along with dispersive solid-phase extraction (SPE) salt and sorbent [anhydrous MgSO4, C18 and primary-secondary amine (PSA)] were obtained from Agilent Technologies (USA). Solvents (methanol and acetonitrile, ≥99.9% purity) were obtained from Tedia Company, Inc. (Ohio, USA). Simulated gastrointestinal juice reagents, including arabinogalactan, peptone, xylan, pectin, soluble starch, mucin, cysteine, glucose yeast, pepsin, bile extract, and pancreatin, used for the SHIME model, were obtained from Sigma-Aldrich Company Ltd (Dorset, UK). Solutions of enzymes were all freshly prepared just before use.
In this study, three fruits and vegetables, tomato, cucumber, and carrot, collected from local markets in the city of Hefei (China), were used to evaluate bioaccessibility, with four neonicotinoid (imidacloprid, thiamethoxam, acetamiprid, and thiacloprid) concentrations of 5.0 mg kg−1. Before sample spiking, samples were homogenized and lyophilized with an FD5-4 freeze-dryer (GOLD-SIM, USA) at −50 °C. In addition, another portion was subjected to gastrointestinal digestion, and then the gastrointestinal juices were fortified with three spiked levels (0.5, 1.0, and 5.0 mg kg−1) for method validation.
An in vitro digestion model based on human SHIME
The SHIME in vitro digestion model was selected for this study because this model involves fast and simple steps compared to other in vitro digestion models.8 The human SHIME protocol was used as described previously with some modifications.15 Briefly, 1L of the nutrient solution containing 3.0 g yeast extract, 3.0 g starch, 2.0 g pectin, 1.0 g arabinogalactan, 1.0 g xylan, 1.0 g peptone, 1.0 g mucin, 0.5 g cysteine, and 0.4 g glucose, was autoclaved at 121 °C for 15 min before use. The in vitro digestion model consisted of two compartments simulating the stomach and small intestine. For gastric juice, the chemicals used were 200 mL nutrient solution and 25 mL gastric acid (0.09 g of pepsin per liter and 0.1 M HCl). On the other hand, the chemicals used for intestinal juice were 12.5 g NaHCO3, 6.0 g bile powder, and 0.9 g pancreatin per liter of nutrient solution.
During peptic digestion, 0.5 g of the freeze-dried samples (in triplicate) were added to 20 mL of gastric juice and incubated at 37 °C for 1 h followed by pH adjustment to 3.0 ± 0.1 with HCl (0.1 M) to stop peptic digestion. Afterwards, 10 mL of intestinal juice was added into the residuum, and the pH was adjusted to 7.2 ± 0.1 with NaHCO3 (0.1 M) for incubation at 37 °C for 4 h.
Parameters of the human digestive process
Based on the physiological conditions of the human gastrointestinal system, variations in the following parameters that related to human gastrointestinal digestion after ingestion were modeled. Generally, the normal gastric pH value of fasting gastric and intestinal juice is approximately 2.0 and 7.5, respectively.16 Moreover, bile salts and the combination of enzymes were shown to facilitate the hydrolysis of triacylglycerols or cholesteryl esters.17,18 Thus, buffer solutions with different pH values (pH2.0 and pH7.5) without any digestive enzymes and juices were investigated over 2 h of incubation. The pesticides were reacted with the two types of pH buffers, respectively.
The evaluation of the influence of dietary components was performed using protein and dietary fiber (0.1, 0.2, 0.5, 1.0, and 2.0%) separately in fresh samples (in triplicate). The mixture was then homogenized at 25
000g using a homogenizer (G50, Coyote Bioscience Co., Ltd, Beijing, China). Subsequently, samples were lyophilized and stored at −50 °C until the application of the simulated digestion. Dietary components were added and simulated according to Dietary Guidelines for Chinese Residents (2016).19
Sampling and analysis
Extraction was carried out following the QuEChERS multiresidue method with modifications.20 Briefly, simulated gastrointestinal digestion was accomplished by centrifuging the samples for 10 min at 2820g using the HC-3514 centrifuge (Anhui Zhongke Zhongjia Science Instrument Co. Ltd, Anhui, China). Subsequently, the extraction and purification procedures were as follows: 10 mL of the supernatant was transferred and extracted with 20 mL acetonitrile, followed by shaking, addition of 5 g of NaCl, centrifugation for 5 min at 1504g, clean-up with 0.75 g of MgSO4, 0.25 g of PSA, 0.25 g of C18, and then 2 mL of the supernatant was evaporated to dryness before reconstitution in 2 mL of methanol/water (v/v, 1/1) for HPLC analysis.
HPLC was performed on an Agilent 1260 HPLC system (Agilent Technologies Inc., California, USA) equipped with UV detection (HPLC-UV) and a ZORBAX SB-C18 column (4.6 mm × 250 mm, 5 μm). The mobile phase was acetonitrile
:
water (v/v = 25
:
75) at a flow rate of 1.0 mL min−1 with a column oven temperature of 30 °C. The UV detection wavelength was 254 nm, and the injection volume was 10 μL.
Statistical analysis
All tests were performed with at least three independent replicates. The results are presented as the mean ± standard deviation (SD). Statistical significance (p < 0.05) was determined using one-way analysis of variance (ANOVA) followed by Tukey's test.21 Figures were drawn using the software GraphPad Prism 7 (GraphPad Software, Inc., USA). Bioaccessibility of the samples (gastric and intestinal) was calculated using the following formula:
where C1 is the concentration of the compound of interest in the intestinal or gastric juice (mg kg−1), V is the volume of intestinal or gastric juice (mL), C2 is the concentration of the compound of interest in the sample (mg kg−1), and M is the weight of the sample (g).
Results and discussion
Method validation
Method validation was carried out with respect to selectivity, linearity, accuracy, and the limits of quantification (LOQ). The matrix-matched calibration solutions were used for quantification, and no interfering peaks were observed in the control samples. Satisfactory linearity was obtained, with the squared correlation coefficients (r2) > 0.999 for the four neonicotinoids analyzed by HPLC that ranged from 0.1 to 5.0 mg kg−1. The sensitivity of the method was expressed in terms of the limits of detection (LOQs), which were 0.05 mg kg−1 for the four neonicotinoids. Accuracy and repeatability were evaluated through analysis of three different concentration levels of the target compounds: 0.5, 1.0, and 5.0 mg kg−1. For all matrices, the recoveries of the added standards were in the range of 78.08–106.50% at the low spiked level (Fig. 1a), 73.90–117.15% at the medium spiked level (Fig. 1b), and 85.25–103.74% at the high spiked level (Fig. 1c). Good precision was obtained with relative standard deviations (RSDs) < 10% for all pesticides for the three levels of spiking. The reproducibility of the recovery results, as indicated by relative standard deviations (RSDs), confirmed that the method was sufficiently reliable for pesticide analysis in this study.
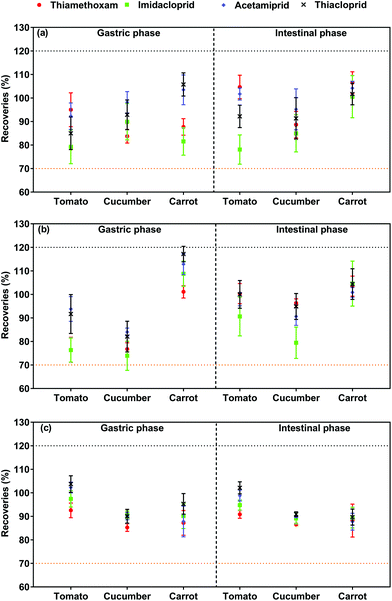 |
| Fig. 1 Recoveries of four neonicotinoids at three different concentration levels in tomato, cucumber, and carrot, at low level (a; 0.5 mg kg−1), medium level (b; 1.0 mg kg−1), and high level (c; 5.0 mg kg−1). Error bars represent the relative standard deviations (RSDs) of five replicates. | |
Variability of neonicotinoid bioaccessibility between matrices
The different sorption to chemicals in matrices may lead to varying solubility and release of certain contaminants. Fig. 2 compared neonicotinoid bioaccessibility among the matrices. Significant differences (p < 0.05) were observed in the tomato, cucumber and carrot regarding neonicotinoid bioaccessibility, indicating that the matrix is a major factor affecting bioaccessibility. The release of neonicotinoids and their bioaccessibility from the matrix was in the order of carrot > cucumber > tomato, while there was no difference in the bioaccessibility of thiamethoxam in the cucumber and tomato. Sugar in foods significantly affects the bioaccessibility,22,23 and tomato contains relatively higher levels of sugar in comparison with carrot and cucumber.24 The results suggested that sugar enhanced the overall stability of neonicotinoids in the fruit and vegetable powder, resulting in a relative reduction in the fraction of pesticide release. Regarding the bioaccessibility of pesticides, acetamiprid (55.27–90.92%) showed relatively high bioaccessibility, whereas the bioaccessibility of thiamethoxam (39.44–55.75%) was comparatively lower. The bioaccessibility of the neonicotinoids was significantly higher than that of pyrethroids (4.42–35.63%) as reported in our previous study.14 A similar phenomenon was observed for pesticides in tea infusions, which may be explained by systemic pesticides with a relatively low log octanol–water partitioning coefficient (log
Kow) having strong systemic properties, resulting in easier leaching.25
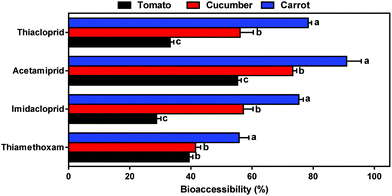 |
| Fig. 2 Food matrix effects on neonicotinoid bioaccessibility via tomato, cucumber, and carrot ingestion at 5.0 mg kg−1. Bars with different lowercase letters are significantly different (one-way ANOVA followed by Tukey's multiple comparison test; p < 0.05). | |
On the other hand, no significant pesticide release occurred in the gastric and intestinal juice after 2 h of incubation. In contrast, statistically significant (p < 0.05) variation between gastric and intestinal juice was only seen with thiamethoxam. For the tomato matrix, the bioaccessibility of thiamethoxam in the gastric juice (81.73%) was significantly higher than that in the intestinal juice (44.66%), whereas a contrary trend was observed for cucumber and carrot (Fig. 3a–c). pH has been identified as a factor affecting the solubility and release of certain contaminants from the food matrix.26 Moreover, the presence of food would affect the pH in the gastric or intestinal juice and subsequently influence the release of contaminants from the matrix.27 The results showed that the pH value was likely to be one of the main factors affecting bioaccessibility during the gastric and intestinal phases.
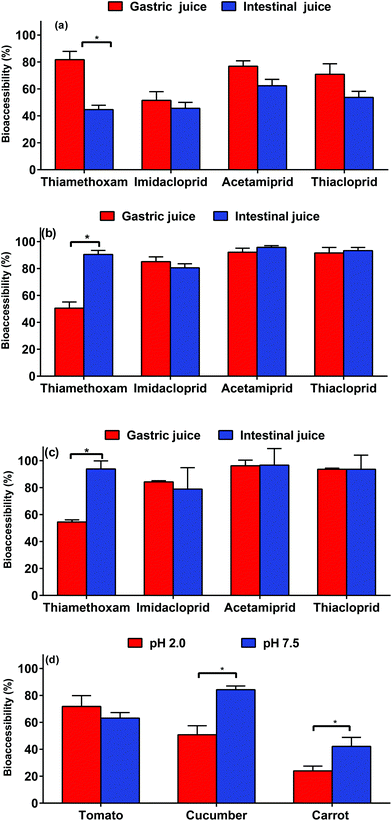 |
| Fig. 3 Variability in neonicotinoid bioaccessibility in tomato (a), cucumber (b) and carrot (c) between gastric and intestinal juices. Effect of the pH without enzymes on the bioaccessibility of thiamethoxam (d). Error bars represent the standard deviation of three replicates. Bars marked with an asterisk indicate significant differences with respect to the bioaccessibility (p < 0.05). | |
For further investigation of the effect of pH, the bioaccessibility of thiamethoxam at different pH values (pH2.0 and pH7.5) was evaluated. As shown in Fig. 3d, a similar phenomenon was observed for thiamethoxam, indicating that pH had a marked effect on the bioaccessibility of thiamethoxam. Moreover, a marked reduction in its bioaccessibility was observed at low pH. Exceptions included the thiamethoxam in tomato, where a slight enhancement was seen. It is probable that the pH played double roles in the bioaccessibility assay. On the one hand, thiamethoxam was stable in weak acid to alkaline solutions but aqueous hydrolysis occurred at low pH and thus, likely reduced the overall fraction of pesticide in solution.28 Karmakar et al. (2009) also showed that thiamethoxam can be hydrolyzed to clothianidin in acidic buffer with <pH 4.29 On the other hand, the release of pesticides from the fruit and vegetable samples occurred as a result of dissolution processes which are dependent on digestive juice chyme.30 In this case, a greater activation in the presence of alkalinity resulted in the enhancement of the release from the carrot and cucumber. This needs further investigation. Overall, these results confirmed that the co-existence of pH and matrix affect the bioaccessibility of neonicotinoids during human digestion in vitro.
Effects of dietary component addition on neonicotinoid bioaccessibility in different matrices
In general, a diet rich in dietary components provides certain benefits because of their positive effects on human health. However, the literature has shown that the degree of release of contaminants by digestive fluids may closely depend on the co-occurring food material in the system.31 In our study, the addition of protein and dietary fiber was effective in changing the bioaccessibility of neonicotinoids.
In the presence of protein (Fig. 4), a higher percentage release of neonicotinoids was observed in carrot, and the increases were not statistically significant (p < 0.05) except for thiamethoxam (26.92% increase). Moreover, a significant change in the bioaccessibility of the neonicotinoids was observed in cucumber, with percentage protein of 0.1–2.0%, whereas moderate changes were detected in the tomato. Exceptions included the bioaccessibility for acetamiprid, with a 15.36% decrease. It has been reported that the protein component could increase the apparent solubility and desorption of hydrophobic organic compounds (HOCs) such as pyrethroids7 and polychlorinated biphenyls (PCB)32 and thus increase the potential bioaccessibility. In most cases, our results are in agreement with previous findings. However, >11.50% decrease in bioaccessibility was also observed for some neonicotinoids with 2.0% protein addition, suggesting possible protein interaction with the bioactivities of food, subsequently preventing their release from the food matrix. As we know, the presence of some dietary components, known as antinutritional factors, such as tannin and phytate, may diminish or hinder the absorption of some compounds, due to the formation of insoluble complexes.33 This factor needs further investigation.
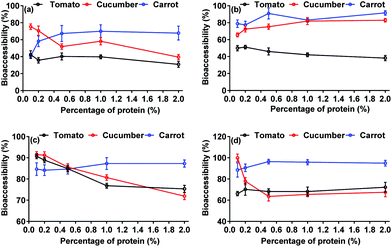 |
| Fig. 4 Effects of protein addition on bioaccessibility of thiamethoxam (a), imidacloprid (b), acetamiprid (c), and thiacloprid (d) in different matrices. | |
In the presence of dietary fiber, our study has also highlighted that with an increase in dietary fiber content, there is a concomitant decrease in the in vitro bioaccessibility of neonicotinoids (Fig. 5). The addition of 2.0% dietary fiber reduced thiamethoxam by 19.70–67.91%, imidacloprid by 25.29–60.31%, acetamiprid by 18.38–36.21%, and thiacloprid by 32.34–37.88%. It is apparent the addition of dietary fiber had a marked influence on neonicotinoid bioaccessibility. Tomas et al. (2018)34 and Sun-Waterhouse et al. (2007)35 proposed that dietary fiber could play a positive role in decreasing bioaccessibility of chemicals, and the enhancement was attributed to the fact that dietary fiber was capable of capturing antinutritional factors, such as polyphenols, into their structure, and then preventing their release. In addition, the percentage decrease in thiamethoxam, imidacloprid, and thiacloprid in carrot was comparatively greater, whereas it was relatively lower for acetamiprid, possibly due to the bioactivity of food content and the physicochemical properties of pesticides.
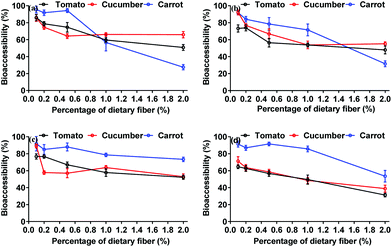 |
| Fig. 5 Effects of dietary fiber addition on bioaccessibility of thiamethoxam (a), imidacloprid (b), acetamiprid (c), and thiacloprid (d) in different matrices. | |
Conclusions
This study clearly demonstrated that the food matrix plays a key role in the bioaccessibility of pesticides, and that interactions may take place between food antinutritional factors and dietary components including proteins and dietary fiber. The results pointed out that the dietary components had a marked influence on neonicotinoid bioaccessibility and the effects varied among the different matrices. These findings provide empirical evidence that increased intake of dietary fiber could improve the safety of fruit and vegetables in daily life, and shed light on a novel direction for investigating pesticide bioaccessibility.
Conflicts of interest
There are no conflicts to declare.
Acknowledgements
This work was supported by the National Natural Science Foundation of China (31601663).
Notes and references
- T. Fernández-Cruz, E. Martínez-Carballo and J. Simal-Gándara, Optimization of selective pressurized liquid extraction of organic pollutants in placenta to evaluate prenatal exposure, J. Chromatogr. A, 2017, 1495, 1–11 CrossRef.
- C. Chen, Y. Qian, Q. Chen, C. Tao, C. Li and Y. Li, Evaluation of pesticide residues in fruits and vegetables from Xiamen, China, Food Control, 2011, 22, 1114–1120 CrossRef CAS.
- U. Bajwa and K. S. Sandhu, Effect of handling and processing on pesticide residues in food-a review, J. Food Sci. Technol., 2014, 51, 201–220 CrossRef CAS PubMed.
- Z. Huan, Z. Xu, W. Jiang, Z. Chen and J. Luo, Effect of Chinese traditional cooking on eight pesticides residue during cowpea processing, Food Chem., 2015, 170, 118–122 CrossRef CAS PubMed.
- S. A. G. Alla, N. M. Loutfy, A. H. Shendy and M. T. Ahmed, Hazard index, a tool for a long term risk assessment of pesticide residues in some commodities, a pilot study, Regul. Toxicol. Pharmacol., 2015, 73, 985–991 CrossRef PubMed.
- H.-H. Li, L.-J. Chen, L. Yu, Z.-B. Guo, C.-Q. Shan, J.-Q. Lin, Y.-G. Gu, Z.-B. Yang, Y.-X. Yang, J.-R. Shao, X.-M. Zhu and Z. Cheng, Pollution characteristics and risk assessment of human exposure to oral bioaccessibility of heavy metals via urban street dusts from different functional areas in Chengdu, China, Sci. Total Environ., 2017, 586, 1076–1084 CrossRef CAS PubMed.
- J. Wang, K. Lin, A. Taylor and J. Gan, In vitro assessment of pyrethroid bioaccessibility via particle ingestion, Environ. Int., 2018, 119, 125–132 CrossRef CAS.
- H. Y. Yu, B. Wu, X. X. Zhang, S. Liu, J. Yu, S. P. Cheng, H. Q. Ren and L. Ye, Arsenic Metabolism and Toxicity Influenced by Ferric Iron in Simulated Gastrointestinal Tract and the Roles of Gut Microbiota, Environ. Sci. Technol., 2016, 50, 7189–7197 CrossRef CAS PubMed.
- I. Yebra-Pimentel, R. Fernandez-Gonzalez, E. Martinez-Carballo and J. Simal-Gandara, A Critical Review about the Health Risk Assessment of PAHs and Their Metabolites in Foods, Crit. Rev. Food Sci. Nutr., 2015, 55, 1383–1405 CrossRef CAS PubMed.
- I. Nybom, G. Waissi-Leinonen, K. Maenpaa, M. T. Leppanen, J. V. K. Kukkonen, D. Werner and J. Akkanen, Effects of activated carbon ageing in three PCB contaminated sediments: Sorption efficiency and secondary effects on Lumbriculus variegatus, Water Res., 2015, 85, 413–421 CrossRef CAS PubMed.
- B. Warth, M. Sulyok and R. Krska, LC-MS/MS-based multibiomarker approaches for the assessment of human exposure to mycotoxins, Anal. Bioanal. Chem., 2013, 405, 5687–5695 CrossRef CAS PubMed.
- B. Kabak and F. Ozbey, Aflatoxin M-1 in UHT milk consumed in Turkey and first assessment of its bioaccessibility using an in vitro digestion model, Food Control, 2012, 28, 338–344 CrossRef CAS.
- G. Mandalari, M. Vardakou, R. Faulks, C. Bisignano, M. Martorana, A. Smeriglio and D. Trombetta, Food Matrix Effects of Polyphenol Bioaccessibility from Almond Skin during Simulated Human Digestion, Nutrients, 2016, 8, 568 CrossRef PubMed.
- Y. Shi, J. Xiao, R. Feng, Y. Liu, M. Liao, X. Wu, R. Hua and H. Cao,
In vitro bioaccessibility of five pyrethroids after human ingestion and the corresponding gastrointestinal digestion parameters: A contribution for human exposure assessments, Chemosphere, 2017, 182, 517–524 CrossRef CAS PubMed.
- Y.-H. Shi, J.-J. Xiao, R.-P. Feng, Y.-Y. Liu, M. Liao, X.-W. Wu, R.-M. Hua and H.-Q. Cao, Factors Affecting the Bioaccessibility and Intestinal Transport of Difenoconazole, Hexaconazole, and Spirodiclofen in Human Caco-2 Cells Following in Vitro Digestion, J. Agric. Food Chem., 2017, 65, 9139–9146 CrossRef CAS PubMed.
- W. Guo, W. Lu, Y. Xu, L. Wang, Q. Wei and Q. Zhao, Relationship between adverse gastric reactions and the timing of enteric-coated aspirin administration, Clin. Drug Invest., 2017, 37, 187–193 CrossRef CAS PubMed.
- D. Y. Hui and P. N. Howles, Carboxyl ester lipase structure-function relationship and physiological role in lipoprotein metabolism and atherosclerosis, J. Lipid Res., 2002, 43, 2017–2030 CrossRef CAS.
- J. Maldonado-Valderrama, P. Wilde, A. Macierzanka and A. Mackie, The role of bile salts in digestion, Adv. Colloid Interface Sci., 2011, 165, 36–46 CrossRef CAS PubMed.
-
T. C. N. Society, The Food Guide Pagoda for Chinese Residents, http://dg.cnsoc.org/upload/images/source/20160519163856103.jpg.
- A. Zachara, D. Galkowska and L. Juszczak, Contamination of Tea and Tea Infusion with Polycyclic Aromatic Hydrocarbons, Int. J. Environ. Res. Public Health, 2018, 15, 45 CrossRef PubMed.
- M. N. Ghosh and D. Sharma, Power of Tukey's Test for Non-Additivity, J. R. Stat. Soc. Series B: Stat. Methodol., 1963, 25, 213–219 Search PubMed.
- M. H. Choi, G. H. Kim, K. H. Park, Y. S. Kim and S. M. Shim, Bioaccessibility of Total Sugars in Carbonated Beverages and Fermented Milks, J. Korean Soc. Appl. Biol. Chem., 2011, 54, 778–782 CrossRef CAS.
- M. Intawongse and J. R. Dean,
In vitro testing for assessing oral bioaccessibility of trace metals in soil and food samples, TrAC, Trends Anal. Chem., 2006, 25, 876–886 CrossRef CAS.
- I. Molnárperl and M. A. Morvai, Rapid method for the simultaneous GC quantitation of acids and sugars in fruits and vegetables, Food Addit. Contam., 1992, 9, 505–514 CrossRef PubMed.
- K. Matsuda, S. D. Buckingham, D. Kleier, J. J. Rauh, M. Grauso and D. B. Sattelle, Neonicotinoids: insecticides acting on insect nicotinic acetylcholine receptors, Trends Pharmacol. Sci., 2001, 22, 573–580 CrossRef CAS PubMed.
- R. A. Rocha, B. de la Fuente, M. J. Clemente, A. Ruiz, D. Velez and V. Devesa, Factors affecting the bioaccessibility of fluoride from seafood products, Food Chem. Toxicol., 2013, 59, 104–110 CrossRef CAS PubMed.
- Y. Kang, W. J. Pan, S. Y. Liang, N. Li, L. X. Zeng, Q. Y. Zhang and J. W. Luo, Assessment of relative bioavailability of heavy metals in soil using in vivo mouse model and its implication for risk assessment compared with bioaccessibility using in vitro assay, Environ. Geochem. Health, 2016, 38, 1183–1191 CrossRef CAS PubMed.
- J. Meijide, J. Gómez, M. Pazos and M. A. Sanromán, Degradation of thiamethoxam by the synergetic effect between anodic oxidation and Fenton reactions, J. Hazard. Mater., 2016, 319, 43–50 CrossRef CAS PubMed.
-
R. Karmakar, S. B. Singh, G. K. J. J. o. E. Science and P. B. Health, Kinetics and mechanism of the hydrolysis of thiamethoxam, 2009, vol. 44, pp. 435–441 Search PubMed.
- C. Bucking and C. Wood, The effect of postprandial changes in pH along the gastrointestinal tract on the distribution of ions between the solid and fluid phases of chyme in rainbow trout, Aquacult. Nutr., 2009, 15, 282–296 CrossRef CAS.
- N. Stanisavljević, J. Samardžić, T. Janković, Š. K. M. Mojsin, V. Topalović and M. J. F. C. Stevanović, Antioxidant and antiproliferative activity of chokeberry juice phenolics during in vitro simulated digestion in the presence of food matrix, Food Chem., 2015, 175, 516–522 CrossRef PubMed.
- H. Ertl and W. Butte, Bioaccessibility of pesticides and polychlorinated biphenyls from house dust: in vitro methods and human exposure assessment, J. Exposure Sci. Environ. Epidemiol., 2012, 22, 574–583 CrossRef CAS PubMed.
- K. Shilpa and A. Jyothi Lakshmi, Comparison of enhancement in bioaccessible iron and zinc in native and fortified high-phytate oilseed and cereal composites by activating endogenous phytase, Int. J. Food Sci. Technol., 2012, 47, 1613–1619 CrossRef CAS.
- M. Tomas, J. Beekwilder, R. D. Hall, C. D. Simon, O. Sagdic and E. Capanoglu, Effect of dietary fiber (inulin) addition
on phenolics and in vitro bioaccessibility of tomato sauce, Food Res. Int., 2018, 106, 129–135 CrossRef CAS PubMed.
- D. Sun-Waterhouse, L. D. Melton, C. J. O'Connor, P. A. Kilmartin and B. G. Smith, Effect of apple cell walls and their extracts on the activity of dietary antioxidants, J. Agric. Food Chem., 2007, 56, 289–295 CrossRef PubMed.
Footnote |
† These authors contributed equally to this work. |
|
This journal is © The Royal Society of Chemistry 2019 |