DOI:
10.1039/C8FO01863D
(Paper)
Food Funct., 2019,
10, 296-303
Effect of conjugated linoleic acid overproducing Lactobacillus with berry pomace phenolic extracts on Campylobacter jejuni pathogenesis
Received
25th September 2018
, Accepted 28th November 2018
First published on 29th November 2018
Abstract
Campylobacter jejuni (CJ) is one of the predominant causative agents of acute gastroenteritis in the US and other developed countries through the handling of raw chicken or the consumption of undercooked poultry and poultry products. Probiotics and their metabolites such as conjugated linoleic acids (CLAs) play a crucial role in improving host health and act as antimicrobials against enteric pathogens. Furthermore, prebiotics or prebiotic-like components such as bioactive phenolics from berry pomace can stimulate the growth of beneficial microbes including Lactobacillus casei (LC) and its metabolites, and competitively inhibit the growth of enteric bacterial pathogens. In this study, we aimed at enhancing the efficiency of antimicrobial/beneficial activities of LC and the extent of production of bioactive compounds by combining berry pomace phenolic extract (BPPE) and overproducing CLA in L. casei (LC-CLA). Under mixed culture conditions, LC-CLA in the presence of BPPE reduced the growth of CJ by more than 3 log CFU ml−1 within 48 h. The cell-free culture supernatant (CFCS) of LC-CLA in the presence of BPPE also reduced significantly the growth of CJ >3.2 log CFU ml−1 at 24 h. The interactions of CJ with cultured chicken fibroblast cells (DF-1), chicken macrophage (HD-11), and human epithelial cells (HeLa) were altered significantly. Treatments with BPPE and/or CFCS also altered the injured cell number, auto-aggregation capacity and cell surface hydrophobicity of CJ, significantly. Furthermore, combined treatments with BPPE and CFCSs of LC-CLA altered the expression of multiple virulence genes such as ciaB, cdtB, cadF, flaA, and flaB of CJ from 0.45 fold to 6.85 fold. Overall, BPPE enhanced the effect of LC-CLA in the reduction of CJ growth, survival ability, host cell–CJ interactions, and virulence gene expression. This finding indicates that a combination of BPPE and LC-CLA may be able to prevent the colonization of CJ in poultry, reduce the cross-contamination of poultry products and control poultry-borne campylobacteriosis in humans.
1. Introduction
According to the data from the most recent outbreaks caused by multi-drug resistant bacterial isolates in the US, Campylobacter has become one of the major concerns for health professionals and food producing industries.1 From 2010 to 2015, there were 209 foodborne Campylobacter outbreaks that led to 2234 cases of illnesses in the US.2 Furthermore, this huge number of campylobacteriosis cases increased the risk of post-infection complexity specifically Guillain Barre Syndrome, irritable bowel syndrome, reactive arthritis and immunoproliferative small intestinal diseases.3,4 The most commonly identified causative agent of human campylobacteriosis is Campylobacter jejuni (CJ) and it commonly occurred due to the handling or consumption of raw or partially cooked poultry products, and unpasteurized milk or milk products,4–6 as chickens and other warm blooded farm animals naturally harbor Campylobacter in their gastrointestinal (GI) tracts.5 In addition, organic or pasture practicing farmers cannot use any antibiotics or chemicals; so with an increased chance of cross-contamination with foodborne pathogens especially CJ in organic animal food products,5,7–11 alternative strategies for the reduction of the colonization of Campylobacter in the farm animal gut to limit the cross-contamination of animal food products and environment are urgently required.
Probiotics are known to play important roles in modulating the gut microbial ecosystem particularly reducing the colonization of bacterial pathogens in the gut and improving host gut health.12–14 Although the molecular basis of this modulation has not been fully explored, various mechanisms including the ability of probiotics to use quorum sensing to control the virulence genes of pathogens,15 disruption of the specific protein synthesis and secretion, and limitation in motility, flagellar assembly and other pathogenicity associated traits16 have been proposed. The effectiveness of probiotics against different enteric bacterial pathogens generally depends on the number of probiotics and the amount of bioactive products, specifically polyunsaturated fatty acids i.e., conjugated linoleic acid (CLA), produced by them.17Lactobacillus casei (LC) has already been accepted as an effective probiotic in relieving gastrointestinal pathogenic bacterial infections both ex vivo and in vivo with no pathogenic traits in humans and animals.18–20 Furthermore, the antimicrobial properties of LC and its metabolites, specifically CLA, showed effectiveness in the presence of prebiotics or prebiotic-like components, e.g., peanut fractions, cocoa, olive, and various plant extracts.20–23
Various plant byproducts, especially blackberry (Rubus fruticosus) and blueberry (Vaccinium corymbosum) byproducts/pomace, contain bioactive phenolics along with various other compounds, which have shown antimicrobial effects against different enteric pathogens including Campylobacter.24–27 Furthermore, previous studies in our laboratory have provided evidence that berry juice also stimulates the growth of probiotics.28
In this study, we aim at evaluating the combined effect of genetically modified LC with the over-expression of the linoleate isomerase gene and berry pomace phenolic extract (BPPE) against the survival ability of one of the major zoonotic pathogens, CJ, and its interaction with host cells. In addition, physicochemical properties and expression levels of pathogenic virulence mediatory genes were also investigated to determine the underlying mechanisms of action.
2. Materials and methods
2.1. Bacterial strains and growth conditions
The over-expression of the linoleate isomerase gene in Lactobacillus casei (LC-CLA) (ATCC 334) was used in this study and grown on de Man–Rogosa–Sharpe (MRS) agar (EMD Chemicals Inc., USA) overnight at 37 °C in a CO2 (5%) enriched environment (Thermo Fisher Scientific Inc., USA). Campylobacter jejuni RM1221 (CJ) (ATCC BAA-1062) was grown on Karmali agar (EMD Chemicals Inc., USA) overnight at 37 °C, under microaerophilic conditions (10% CO2, 5% O2 and 85% N2) (Thermo Fisher Scientific Inc., USA).23
2.2. Preparation of pomace extracts
Commercial blackberry and blueberry pomace samples (donated by Milne Fruit Products Inc., USA) were stored at −20 °C and used to extract phenolic compounds according to the protocol previously described by Salaheen et al. and Yang et al.26,28 A spectrophotometric method was used for the determination of total phenolic contents in blackberry and blueberry pomaces and expressed as the Gallic Acid Equivalent (GAE).29 Berry pomace phenolic extract (BPPE) was composed of blackberry and blueberry pomace extracts at a 1
:
1 (v/v) ratio.
2.3. Cell lines and culture conditions
Chicken fibroblast, DF-1 (ATCC®CRL-12203™), chicken macrophage, HD-11 (kindly provided by Dr Uma S. Babu, Immunobiology Branch, Food and Drug Administration, Laurel, MD, USA) and human epithelial, HeLa (ATCC® CCL2™) cells were cultured following the standard method (5% CO2) in Dulbecco's Modified Eagle's Medium (DMEM) (Corning cellgro, USA) supplemented with 10% heat-inactivated Fetal Bovine Serum (FBS) (Corning cellgro, USA) and 50 μg ml−1 of gentamycin (Lonza, USA) at 37 °C. 24-Well culture plates (Greiner bio-one Inc., USA) were used for seeding DF-1, HD-11 and HeLa cells at 2 × 105 cells per ml and then they were grown under the standard conditions as mentioned above up to 90% or more confluence. The confluent cultures were then washed with phosphate-buffered saline (PBS) three times and immersed in DMEM (antibiotic free) supplemented with 10% heat-inactivated FBS for the cell adhesion and invasion assay.25
2.4. Growth of LC-CLA in the absence or presence of BPPE
LC-CLA was grown on MRS agar plates overnight according to the procedure described above. A bacterial suspension (400 μl) containing 107 colony forming unit (CFU) per ml in the absence or presence of BPPE of 0.1 mg ml−1 GAE concentration was grown in MRS broth (EMD Chemicals Inc., USA) to a final volume of 4 ml at 37 °C in a CO2 (5%) enriched environment for a time period of 72 h. At 24, 48, and 72 h time points of incubation, serial dilutions were performed in phosphate buffered saline (PBS), followed by plating on MRS agar.
2.5. Growth inhibition assay
LC-CLA and CJ were grown on their respective selective agar plates overnight according to the procedure described above. BPPE of 0.1 mg ml−1 GAE concentration and LC-CLA bacterial suspension containing 107 CFU ml−1 in the presence of BPPE (total 400 μl) were co-cultured with 400 μl of CJ bacterial suspension containing 106 CFU ml−1 in 3.2 ml of Bolton broth (HiMedia, India) supplemented with 10% heat-inactivated FBS at 37 °C under microaerophilic conditions. At 24, 48, and 72 h incubation time periods, serial dilutions were performed in phosphate buffered saline (PBS), followed by plating on Karmali agar for CJ.
Overnight liquid cultures of LC-CLA were centrifuged at 4000g for 20 min (Thermo Fisher Scientific Inc., USA) and cell free culture supernatants (CFCSs) were collected and filtered through a 0.2 μm sterile syringe filter (VWR Inc., USA).30 CFCSs collected from LC-CLA (CFCS) were stored at 4 °C. A CJ bacterial cell suspension containing 106 CFU ml−1 of 400 μl was inoculated with 10% of CFCS with BPPE (0.1 mg ml−1 GAE) in Bolton broth with 10% FBS, respectively, and incubated at 37 °C under microaerophilic conditions. At 24, 48, and 72 h time points of incubation, serial dilutions were performed in phosphate buffered saline (PBS), followed by plating on Karmali agar for CJ.
2.6. Cell adhesion and invasion assay
The DF-1, HD-11, and HeLa cell monolayers were grown in the wells of a 24-well plate with 800 μl DMEM containing 10% FBS and pre-treated with 100 μl DMEM (control), BPPE of 0.1 mg ml−1 GAE concentration or LC-CLA and CFCS in the presence of BPPE, respectively, for 1 h with each treatment in triplicate. After pre-treatment, 100 μl of the CJ bacterial suspension with a multiplicity of infection (MOI) of 10 (2 × 106 CFU ml−1) were inoculated into each well, incubated further for 2 h, and after incubation, the cell monolayers were washed with DMEM with 10% FBS three times. Then the cells were lysed by treating with 0.1% Triton X-100 for 15 min, serially diluted and plated on Karmali agar plates for the determination of the adhesive bacterial cells. Cell invasion activity was determined by further 1 h incubation of the washed monolayers in DMEM containing 10% FBS and 100 μg ml−1 gentamicin followed by washing three times, lysis with a Triton X-100, serial dilution and plating on Karmali agar plates.21
2.7. Physicochemical properties of CJ treated with the CFCS
Physicochemical properties (injured bacterial cell ratio, auto-aggregation activity, and cell surface hydrophobicity) were evaluated following the methodologies previously described by Ahn et al.31 with slight modifications. In brief, bacterial cells were grown in the presence of BPPE (0.1 mg ml−1 GAE) or CFCS with BPPE (0.1 mg ml−1 GAE) or in the absence of any treatments (control) at 37 °C for 18 h. The CJ cells were then collected by centrifuging at 3000g for 20 min followed by serial dilution and plating on Karmali agar and LB agar containing 5% FBS at 37 °C, under the conditions described above for the injured cell rate assay, and auto-aggregation and hydrophobicity assays were carried out.
2.8. Quantitative RT-PCR assay
The CJ bacterial cells were grown in the absence or presence of BPPE (0.1 mg ml−1 GAE) or/and the CFCS and RNA was extracted from the treated or untreated cells according to the protocol of a ZR Bacterial RNA MiniPrep kit (Zymo Research Corp., USA). The synthesis of cDNA was performed by using a qScript cDNA SuperMix (Quanta Biosciences, USA). The custom-synthesized oligonucleotide primers (Eurofins MWG Operon, USA) for ciaB (Campylobacter invasion antigen), cdtB (Cytolethal distending toxin), cadF (Campylobacter adhesion fibronectin-binding protein), flaA (Flagellin A subunit synthesis), and flaB (Flagellin B subunit synthesis) of CJ were used for the qPCR assay following the methodology previously described by Tabashsum et al.18 The relative transcription levels of target genes were estimated by the comparative log fold change. The Cycle Threshold (CT) values of target genes in treated bacterial cells were compared to the ones from untreated bacterial cells and normalized to the housekeeping gene (16s).
2.9. Statistical analysis
The Statistical Analysis System Software (SAS Institute Inc., USA) was used for data analysis. The one-way analysis of variance (ANOVA) for each single time point was used followed by Tukey's test to evaluate the treatments and determine the significant differences between control and treatments based on the significant level of 0.05.
3. Results
3.1. Effect of BPPE on the growth of LC-CLA
The growth of LC-CLA in the absence or presence of BPPE (0.1 mg ml−1 GAE) was evaluated for 72 h to assess the effect of BPPE on the growth of LC-CLA (Fig. 1). In the presence of 0.1 mg/ ml−1 GAE of BPPE, the growth of LC-CLA was stimulated throughout 72 h. At 24 h, the growth of LC-CLA was increased only numerically in the presence of BPPE. At 48 h and 72 h, the growth of LC-CLA was increased in the presence of BPPE significantly by 0.71 and 0.19 log CFU ml−1, respectively (Fig. 1).
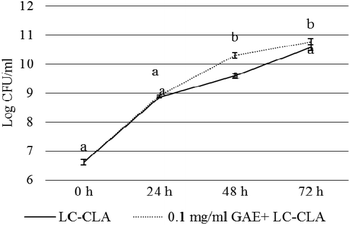 |
| Fig. 1 Growth of LC-CLA in the absence or presence of BPPE (0.1 mg ml−1 GAE) at 24, 48, and 72 h. Error bars indicate the standard deviation from 3 or more parallel trials. Different letters at each time point indicate the significant difference in growth at p < 0.05. | |
3.2. Effects of the probiotic strain and/or BPPE on the growth and survival of CJ
Pathogen (CJ) and probiotic (LC-CLA) strains were co-cultured in the presence of BPPE (0.1 mg ml−1 GAE) to determine the combined effect of the probiotic strain in combination with the prebiotic-like component, BPPE, on the growth and survival of CJ at various time points. The growth inhibition pattern of CJ under the abovementioned co-culture conditions is shown in Fig. 2. The growth of CJ was reduced >1.8 logs during the first 24 h in the presence of 0.1 mg ml−1 GAE of BPPE alone. We also observed that the probiotic strain, LC-CLA, in the presence of BPPE (0.1 mg ml−1 GAE) reduced the growth of CJ in a similar pattern in a time-dependent manner (Fig. 2). Overall, LC-CLA in the presence of BPPE showed a higher inhibitory effect than the BPPE alone.
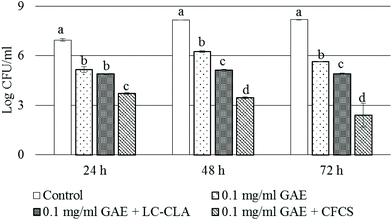 |
| Fig. 2 Growth and survival of CJ at 24, 48, and 72 h. The sign ‘+’ indicates the combination of the treatments. Error bars indicate the standard deviation from 3 or more parallel trials. Different letters (a to d) at each time point indicate the significance of growth reduction when compared with single culture as a control and the treatments at p < 0.05. | |
In a similar experiment, the CFCS, collected from the overnight culture of LC-CLA, in the presence of BPPE, showed strong inhibitory effects on the growth of CJ (Fig. 2). During the first 24 h, the growth of CJ was reduced >3.2 log CFU ml−1 in the presence of BPPE at a concentration of 0.1 mg ml−1 GAE with the CFCS. After 48 h of incubation, the growth of CJ was reduced >4.5 logs in the presence of BPPE of 0.1 mg ml−1 GAE concentration with the CFCS. The most effective growth inhibition of CJ was observed by the treatment of BPPE at a concentration of 0.1 mg ml−1 GAE with the CFCS after 72 h of incubation (Fig. 2).
3.3. Alteration of adhesion and invasion of CJ to cultured cells
Adhesion to and invasion into pre-treated cultured cells in the presence of BPPE and/or the probiotic strain or its CFCS were assessed to evaluate their role in the alteration of CJ–host cell interactions. In the presence of BPPE alone, probiotic strain LC-CLA or its CFCS reduced the adhesion and invasion of CJ to DF-1, HD-11, and HeLa cells, significantly (Fig. 3). It was observed that when pre-treated with BPPE of 0.1 mg ml−1 GAE or probiotic strains LC-CLA and BPPE, the adhesion of CJ to DF-1 cells significantly reduced from a range of 1.25 log CFU ml−1 to 2.22 log CFU ml−1 (Fig. 3A). It was also detected that the CFCS in the presence of BPPE significantly reduced (2.56 log CFU ml−1) the adhesion of CJ to DF-1 cells. In the same assay, BPPE (0.1 mg ml−1 GAE) either with live probiotic cells LC-CLA or its CFCS reduced the invasion of CJ into DF-1 cells from a range of 0.8 log CFU ml−1 to 1.95 log CFU ml−1 (Fig. 3A).
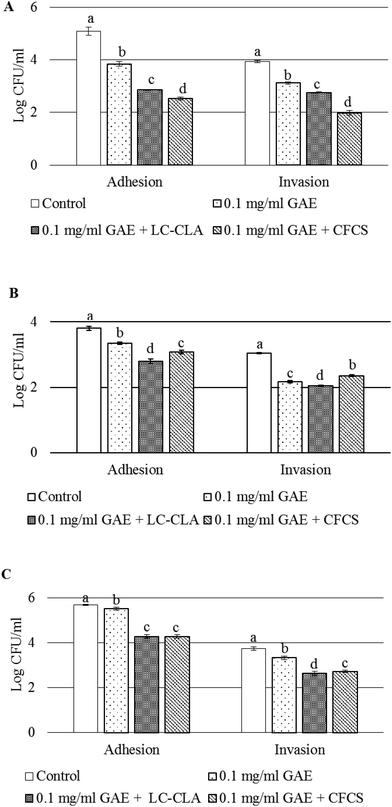 |
| Fig. 3 Alteration of interactions between CJ and cultured cells. Adherence and invasion of CJ to DF-1 cells treated with BPPE (0.1 mg ml−1 GAE) or live probiotic strain LC-CLA or the CFCS collected from the overnight culture of LC-CLA (A), adhesion and invasion levels of CJ to HD-11 cells with BPPE (0.1 mg ml−1 GAE) or live probiotic strain LC-CLA or the CFCS collected from the overnight culture of LC-CLA (B), and cell adhesion and invasion levels of CJ to HeLa cells with BPPE (0.1 mg ml−1 GAE) or live probiotic strain LC-CLA or the CFCS collected from the overnight culture of LC-CLA (C). The sign ‘+’ indicates the combination of the treatments. Error bars indicate the standard deviation from 3 or more parallel trials. Bars with different letters (a to d) are used to indicate significant differences when compared with the control and the treatments at p < 0.05. | |
In a similar experiment, the adhesion of CJ to HD-11 cells was also significantly reduced from a range of 0.46 log CFU ml−1 to 1.0 log CFU ml−1 when pre-treated with BPPE or the probiotic strain or its cell free culture supernatant with BPPE (Fig. 3B). Similarly, the invasion of CJ into HD-11 cells was also significantly reduced from a range of 0.69 log CFU ml−1 to 1.0 log CFU ml−1 in the presence of BPPE (0.1 mg ml−1 GAE) or BPPE with the probiotic cell or the CFCS collected from the respective overnight culture of the probiotic strain (Fig. 3B).
When the adhesion of CJ to HeLa cells was assessed, BPPE of 0.1 mg ml−1 GAE concentration reduced the adhesion by 0.18 log CFU ml−1. The probiotic strain LC-CLA or its cell free culture supernatants in the presence of BPPE (0.1 mg ml−1 GAE) significantly reduced the adhesion of CJ by 1.4 log CFU ml−1 or more (Fig. 3C). The invasion of CJ into HeLa cells was reduced by 0.41 log CFU ml−1 by BPPE of 0.1 mg ml−1 GAE. Other pre-treatments (LC-CLA or its CFCS in the presence of BPPE) reduced the CJ invasion by more than 1.0 log CFU ml−1 into cultured HeLa cells (Fig. 3C).
3.4. Alteration of the physicochemical properties of CJ in the presence of CFCS and/or BPPE
The physicochemical properties of CJ, specifically a ratio of injured cells or auto-aggregation activity and cell surface hydrophobicity, were altered when pre-treated with BPPE or/and CFCS obtained from the overnight culture of LC-CLA (Table 1). For example, we observed a significantly increased percentage of injured CJ cells, when compared to the control. The CFCS with BPPE of 0.1 mg ml−1 GAE concentration was the most effective pre-treatment resulting in 32.32% injured CJ cells (Table 1). The auto-aggregation capacity of CJ was significantly decreased by the pre-treatments, while BPPE (0.1 mg ml−1 GAE) in the presence of CFCS was more effective (56.13% decrease) and BPPE of 0.1 mg ml−1 GAE concentration was less effective (22.13% decrease) (Table 1). We also found that the cell surface hydrophobicity of CJ was reduced significantly by the CFCS in the presence of BPPE (0.1 mg ml−1 GAE) but BPPE (0.1 mg ml−1 GAE) alone could reduce the cell surface hydrophobicity of CJ only numerically when compared to the control group (Table 1).
Table 1 Physicochemical properties of CJ treated with the CFCS and/or BPPE
Treatment |
Injured cell (%) |
Auto-aggregation (%) |
Hydrophobicity (%) |
†Values indicate mean ± standard deviation and means with different letters (a to c) within the same column are different when compared with the control and the treatments at p < 0.05. The sign ‘+’ indicates the combine effects of BPPE and the CFCS of LC-CLA. CFSC indicates the cell free culture supernatant collected from the overnight culture of LC-CLA. |
Control |
19.71 ± 1.07†,a |
49.28 ± 2.36a |
14.50 ± 2.98a |
0.1 mg ml−1 GAE |
24.04 ± 0.87b |
38.37 ± 6.7b |
10.90 ± 1.36ab |
0.1 mg ml−1 GAE + CFCS |
32.32 ± 1.73c |
21.62 ± 1.41c |
6.92 ± 1.41b |
3.5. Effects of BPPE or/and the CFCS of the probiotic on CJ virulence gene expression
In the presence of BPPE with or without CFCS, we evaluated the expression level of several virulence genes of CJ including ciaB, cdtB, cadF, flaA and flaB based on qPCR analysis (Fig. 4). These genes were chosen as ciaB, cadF, flaA and flaB, and are commonly known for their critical role in CJ colonization to host cells and cdtB is imperious in CJ toxin production in host cells.32 The relative expression level of the ciaB gene was significantly down-regulated >1.5 fold by BPPE of 0.1 mg ml−1 GAE in the presence of the CFCS. The relative expression level of the cdtB gene was also significantly down-regulated more than 1.65 fold by BPPE of 0.1 mg ml−1 GAE in the presence of CFCS. The relative expression levels of flaA and flaB genes were up-regulated significantly in the presence of BPPE with or without CFCS from a range of 3.4 fold to 6.85 fold. All other relative expression levels of the abovementioned genes were insignificant (Fig. 4).
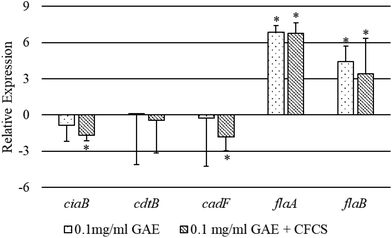 |
| Fig. 4 Relative expression of different virulence genes of CJ treated with BPPE (0.1 mg ml−1 GAE) or the CFCS from LC-CLA in the presence of BPPEs. The sign ‘+’ indicates the combination of the treatments. Error bars indicate the standard deviation from 3 or more parallel trials. Bars with asterisk (*) are significantly different at p < 0.05. | |
4. Discussion
In this study, we investigated the combined role of the genetically modified, LC-CLA containing mcra gene, and BPPE extracted from blackberry and blueberry pomaces/byproducts in the growth, survival and metabolic activities of CJ, as well as their effect on the interactions between host (DF-1, HD-11, and HeLa) cells and CJ. The effectiveness of metabolites alone, in the absence of live LC-CLA, and the culture supernatants produced by the L. casei mutant strain (LC-CLA) was tested for their role in the inhibition of CJ growth and survival as well as in the alteration of CJ pathogenesis in the presence of a sub-lethal concentration of BPPE.
Previously, we reported that the CLA overproducing L. casei strain LC-CLA or its cell free culture supernatant or BPPE alone can inhibit the growth and survival of CJ.18,26 In this study, we aimed to combine the effects of LC-CLA and BPPE for intensifying their role in inhibiting the growth of CJ under co-culture conditions. Our finding indicates that the combination of BPPE and LC-CLA was able to inhibit growth, and reduce the adherence and invasion abilities of CJ in chicken and human origin cultured cells more aggressively.
The possible reasons behind this inhibitory effect of LC-CLA against CJ growth under co-culture conditions may include the production or over-production of anti-pathogenic metabolites particularly CLA with other PUFAs and SCFAs and nutritional competition.17,22 The mechanism behind growth inhibition by the BPPE possibly involves phenolics damaging the bacterial cell membrane, and its role in inhibiting extracellular microbial enzyme secretion, obstruction on microbial metabolism and also increased growth of the probiotic strain in the presence of phenolic compounds may out-compete CJ in the growth media.24,26,28 The combined effects of LC-CLA and BPPE were more pronounced against CJ pathogenesis; one of the most obvious reasons could be the increased growth of LC-CLA in the presence of BPPE. The BPPE in the presence of CFCS from the overnight culture of the probiotic strain was also able to reduce the growth of CJ and the explanation behind this inhibition may include acidic conditions generated by the metabolites from the probiotic strain,33,34 and hydrogen peroxide and antimicrobial polypeptides produced by the probiotic strain.35–37 Anti-pathogenic traits of phenolic compounds and CLA along with other metabolites produced by the probiotic strain have enhanced efficacy when combined, as the combined effect shows better performance than previous individual effect studies in our laboratory.18,20,22–26,28
Adhesion is one of the prerequisites for bacterial colonization to host cells followed by invasiveness and is considered to be an important virulence property.38,39 In this study, the adhesion efficacy of CJ was reduced significantly in cultured chicken fibroblast (DF-1), chicken macrophage (HD-11), and human origin HeLa cells under co-culture conditions or by the metabolites obtained from the overnight culture with BPPE or only BPPE without the probiotic strain/its metabolites when compared to the control group (growth media without any supplements). This finding indicates that in the presence of this genetically modified probiotic strain with a feed or water supplement with BPPE may be able to reduce the colonization of CJ in poultry. Previously, it was reported that similar carbohydrate-binding specific proteins are displayed on the Lactobacillus spp. surface which may be involved in decreasing the adhesion and invasiveness of enteric bacterial pathogens by pre-occupying the surface binding receptors on host cells.40,41 Furthermore, researchers also reported that CFCSs, collected from various strains of Lactobacillus spp., also restricted the adhesion to and invasion into host cells of different enteric bacterial pathogens in agreement with our findings.12,42
According to the injured cell ratio assay, a higher percentage of injured CJ cells (viable) was observed in the presence of BPPE and CFCS, collected from LC-CLA. This amplification of the effectiveness from BPPE or higher concentrations of CLA in the CFCS produced by the LC-CLA strain also supported their synergistic effects. This finding was also supported by several previous reports.18,24–26 Bacterial cell surface hydrophobicity and auto-aggregation capability are believed to be positively correlated with its host cell association activities43–45 and the auto-aggregation capacity serves as a virulence marker of many Gram-negative enteric bacterial pathogens.46,47 Treatments such as that with the CFCS from LC-CLA with BPPE result in the alteration of mechanical and physicochemical properties (decreased hydrophobicity and auto-aggregation) and may have impact on the reduction of invasiveness in CJ, also supported by previous studies.26,28
We further investigated the relative expression levels of several virulence associated genes of CJ when cultured with BPPE in the presence or absence of CFCS/metabolites obtained from the probiotic strain. The expression of several virulence genes was altered; particularly ciaB, cdtB, and cadF were down-regulated and flaA and flaB were upregulated in the presence of the treatments in agreement with the findings of different previous studies.18,26,48,49 The mechanism behind the decreased or increased expression levels of virulence genes of CJ is still partially understood, though the genes involved in colonization and toxin production are confirmed.
5. Conclusion
In conclusion, a probiotic strain, specifically LC-CLA, in the presence of a sub-lethal and poultry growth promoting concentration of BPPE exhibited distinctive effects on CJ pathogenesis; the combination of bioactive probiotics and anti-oxidative and anti-inflammatory natural components complemented each other's ability to eliminate or alter pathogenic characteristics with elevated bidirectional activities in both the accelerating growth of the Lactobacillus strain and the exclusion/inhibition of CJ. This study also confirms the promising functions of the combination of the genetically engineered probiotic LC-CLA and bioactive BPPE in alteration of physicochemical properties, disruption of pathogen–cell interactions, and virulence gene suppression. These traits might be applied in farm animals to reduce the cross-contamination of zoonotic pathogens and to improve the quality of poultry products and public health.
Conflicts of interest
The authors declare no conflict of interest.
Acknowledgements
This research project was supported by the UMD-TIER-1 (2-945060) grant.
References
-
CDC. National Center for Emerging and Zoonotic Infectious Diseases, GA, USA, 2017. https://www.cdc.gov/campylobacter/outbreaks/outbreaks.html/Accessed 05.31.2018 Search PubMed.
-
CDC. National Center for Emerging and Zoonotic Infectious Diseases, GA, USA, 2018. https://www.cdc.gov/campylobacter/outbreaks/puppies-9-17/index.html/Accessed 05.31.2018 Search PubMed.
- X. Chen, G. W. Naren, C. M. Wu, Y. Wang, L. Dai and L. N. Xia,
et al., Prevalence and antimicrobial resistance of Campylobacter isolates in broilers from China, Vet. Microbiol., 2010, 144(1–2), 133–139 CrossRef CAS.
- B. Garin, M. Gouali, M. Wouafo, A. M. Perchec, P. M. Thu and N. Ravaonindrina,
et al., Prevalence, quantification and antimicrobial resistance of Campylobacter spp. on chicken neck-skins at points of slaughter in 5 major cities located on 4 continents, Int. J. Food Microbiol., 2012, 157(1), 102–107 CrossRef CAS.
- S. Salaheen, M. Peng and D. Biswas, Ecological dynamics of Campylobacter in integrated mixed crop–livestock farms and its prevalence and survival ability in post-harvest products, Zoonoses Public Health, 2016, 63(8), 641–650 CrossRef CAS.
- A. Wingstrand, J. Neimann, J. Engberg, E. M. Nielsen, P. Gerner-Smidt and H. C. Wegener,
et al., Fresh chicken as main risk factor for campylobacteriosis, Denmark, Emerging Infect. Dis., 2006, 12(2), 280–284 CrossRef.
- M. Peng, S. Salaheen, J. A. Almario, B. Tesfaye, R. Buchanan and D. Biswas, Prevalence and antibiotic resistance pattern of Salmonella serovars in integrated crop-livestock farms and their products sold in local markets, Environ. Microbiol., 2016, 18(5), 1654–1665 CrossRef CAS.
- M. Peng, S. Salaheen, R. L. Buchanan and D. Biswas, Alterations of Salmonella Typhimurium antibiotic resistance under environmental pressure, Appl. Environ. Microbiol., 2018, 84(19), e01173-18 CrossRef.
- S. Salaheen, M. Peng, J. Joo, H. Teramoto and D. Biswas, Eradication and sensitization of methicillin resistant Staphylococcus aureus to methicillin with bioactive extracts of berry pomace, Front. Microbiol., 2017, 8, 1–10 Search PubMed.
-
M. Peng, S. Salaheen, D. Biswas and C. Park, in Encyclopedia of Agriculture and Food Systems, ed. N. K. Van Alfen, Elsevier Ltd., 2014, vol. 1, pp. 346–357 Search PubMed.
-
S. Salaheen, M. Peng and D. Biswas, in Microbial Food Safety and Preservation Techniques, ed. V. R. Rai, J. A. Bai, CRC Press, 2015, pp. 305–327 Search PubMed.
- R. Campana, S. Federici, E. Ciandrini and W. Baffone, Antagonistic activity of Lactobacillus acidophilus ATCC 4356 on the growth and adhesion/invasion characteristics of Human Campylobacter jejuni, Curr. Microbiol., 2012, 64(4), 371–378 CrossRef CAS.
- C. Maldonado Galdeano and G. Perdigón, The probiotic bacterium Lactobacillus casei induces activation of the gut mucosal immune system through innate immunity, Clin. Vaccine Immunol., 2006, 13(2), 219–226 CrossRef.
- A. L. Servin and M. H. Coconnier, Adhesion of probiotic strains to the intestinal mucosa and interaction with pathogens, Baillieres Best Pract. Res. Clin. Gastroenterol., 2003, 17, 741–754 CrossRef CAS.
- M. J. Medellin-Peña, H. Wang, R. Johnson, S. Anand and M. W. Griffiths, Probiotics affect virulence-related gene expression in Escherichia coli O157:H7, Appl. Environ. Microbiol., 2007, 73(13), 4259–4267 CrossRef PubMed.
- V. Sperandio, C. C. Li and J. B. Kaper, Quorum-sensing Escherichia coli regulator A: A regulator of the LysR family involved in the regulation of the locus of enterocyte effacement pathogenicity island in enterohemorrhagic E. coli, Infect. Immun., 2002, 70(6), 3085–3093 CrossRef CAS.
- M. Peng and D. Biswas, Short chain and polyunsaturated fatty acids in host gut health and foodborne bacterial pathogen inhibition, Crit. Rev. Food Sci. Nutr., 2017, 57(18), 3987–4002 CrossRef CAS PubMed.
- Z. Tabashsum, M. Peng, S. Salaheen, C. Comis and D. Biswas, Competitive elimination and virulence property alteration of Campylobacter jejuni by genetically engineered Lactobacillus casei, Food Control, 2018, 85, 284–291 CrossRef.
- L. H. Shi, K. Balakrishnan, K. Thiagarajah, N. I. M. Ismail and O. S. Yin, Beneficial properties of probiotics, Trop. Life Sci. Res., 2016, 27(2), 73–90 CrossRef PubMed.
- M. Peng, U. Aryal, B. Cooper and D. Biswas, Metabolites produced during the growth of probiotics in cocoa supplementation and the limited role of cocoa in host-enteric bacterial pathogen interactions, Food Control, 2015, 53, 124–133 CrossRef CAS.
- M. Peng, X. Zhao and D. Biswas, Polyphenols and tri-terpenoids from Olea europaea L. in alleviation of enteric pathogen infections through limiting bacterial virulence and attenuating inflammation, J. Funct. Foods, 2017, 36, 132–143 CrossRef CAS.
- M. Peng, E. Bitsko and D. Biswas, Functional properties of peanut fractions on the growth of probiotics and foodborne bacterial pathogens, J. Food Sci., 2015, 80(3), M635–M641 CrossRef CAS PubMed.
- S. Salaheen, B. White, B. J. Bequette and D. Biswas, Peanut fractions boost the growth of Lactobacillus casei that alters the interactions between Campylobacter jejuni, and host epithelial cells, Food Res. Int., 2014, 62, 1141–1146 CrossRef CAS.
- S. Salaheen, J. A. Almario and D. Biswas, Inhibition of growth and alteration of host cell interactions of Pasteurella multocida with natural byproducts, Poult. Sci., 2014, 93(6), 1375–1382 CrossRef CAS.
- S. Salaheen, E. Jaiswal, J. Joo, M. Peng, R. Ho, D. OConnor and D. Biswas, Bioactive extracts from berry byproducts on the pathogenicity of Salmonella Typhimurium, Int. J. Food Microbiol., 2016, 237, 128–135 CrossRef CAS.
- S. Salaheen, C. Nguyen, D. Hewes and D. Biswas, Cheap extraction of antibacterial compounds of berry pomace and their mode of action against the pathogen Campylobacter jejuni, Food Control, 2014, 46, 174–181 CrossRef CAS.
- R. Puupponen-Pimiä, L. Nohynek, H. L. Alakomi and K. M. Oksman-Caldentey, Bioactive berry compounds - Novel tools against human pathogens, Appl. Microbiol. Biotechnol., 2005, 67, 8–18 CrossRef.
- H. Yang, D. Hewes, S. Salaheen, C. Federman and D. Biswas, Effects of blackberry juice on growth inhibition of foodborne pathogens and growth promotion of Lactobacillus, Food Control, 2014, 37(1), 15–20 CrossRef CAS.
- V. L. Singleton, R. Orthofer and R. M. Lamuela-Raventos, Analysis of total phenols and other oxidation substrates and antioxidants by means of Folin Ciocalteau reagent, Methods Enzymol., 1999, 299, 152–178 CAS.
- M. Peng, G. Reichmann and D. Biswas, Lactobacillus casei and its byproducts alter the virulence factors of foodborne bacterial pathogens, J. Funct. Foods, 2015, 15, 418–428 CrossRef CAS.
- J. Ahn and D. Biswas, Influence of bacteriophage P22 on the inflammatory mediator gene expression in chicken macrophage HD-11 cells infected with Salmonella Typhimurium, FEMS Microbiol. Lett., 2014, 352, 11–17 CrossRef CAS PubMed.
- D. E. Fouts, E. F. Mongodin, R. E. Mandrell, W. G. Miller, D. A. Rasko and J. Ravel,
et al., Major structural differences and novel potential virulence mechanisms from the genomes of multiple Campylobacter species, PLoS Biol., 2005, 3(1), 15 CrossRef.
- R. Gyawali and S. A. Ibrahim, Synergistic effect of copper and lactic acid against Salmonella and Escherichia coli, O157:H7:A review, Emir. J. Food Agric., 2012, 24(1), 1–11 Search PubMed.
- M. R. Adams and C. J. Hall, Growth inhibition of food-borne pathogens by lactic and acetic acids and their mixtures, Int. J. Food Sci. Technol., 1988, 23(3), 287–292 CrossRef CAS.
- P. Wannun, S. Piwat and R. Teanpaisan, Purification and characterization of bacteriocin produced by oral Lactobacillus paracasei, SD1, Anaerobe, 2014, 27, 17–21 CrossRef CAS PubMed.
- F. Atassi and A. L. Servin, Individual and co-operative roles of lactic acid and hydrogen peroxide in the killing activity of enteric strain Lactobacillus johnsonii
NCC933 and vaginal strain Lactobacillus gasseri KS120.1 against enteric, uropathogenic and vaginosis-associated pathogen, FEMS Microbiol. Lett., 2010, 304(1), 29–38 CrossRef CAS PubMed.
- H. Y. Xu, W. H. Tian, C. X. Wan, L. J. Jia, L. Y. Wang and J. Yuan,
et al., Antagonistic potential against pathogenic microorganisms and hydrogen peroxide production of indigenous Lactobacilli isolated from vagina of Chinese pregnant women, Biomed. Environ. Sci., 2008, 21(5), 365–371 CrossRef.
- R. A. Edwards and J. L. Puente, Fimbrial expression in enteric bacteria: A critical step in intestinal pathogenesis, Trends Microbiol., 1998, 6, 282–287 CrossRef CAS PubMed.
- R. A. Adegbola, Bacterial adhesion and pathogenicity, Afr. J. Med. Sci., 1988, 17(2), 63–69 CAS.
- S. Salaheen, Z. Tabashsum, S. Gaspard, A. Dattilio, T. H. Tran and D. Biswas, Reduced Campylobacter jejuni colonization in poultry gut with bioactive phenolics, Food Control, 2018, 84, 1–7 CrossRef CAS.
- J. R. Neeser, D. Granato, M. Rouvet, A. Servin, S. Teneberg and K. A. Karlsson, Lactobacillus johnsonii La1 shares carbohydrate-binding specificities with several enteropathogenic bacteria, Glycobiology, 2000, 10(11), 1193–1199 CrossRef CAS PubMed.
- F. Bendali, M. Hebraud and D. Sadoun, Anti-bacterial and anti-adherence activities of a probiotic strain of Lactobacillus paracasei against Listeria monocytogenes, IJAMBR, 2014, 2, 52–63 Search PubMed.
- F. M. Oliveira, E. M. Dos Santos, A. C. Alves, M. A. Campana-Pereira, G. A. Ramaldes and V. N. Cardoso,
et al., Digestion, absorption and tissue distribution of ovalbumin and palmitoyl-ovalbumin: Impact on immune responses triggered by orally administered antigens, Scand. J. Immunol., 2007, 65(2), 139–147 CrossRef CAS PubMed.
- G. S. Lorite, R. Janissen, J. H. Clerici, C. M. Rodrigues, J. P. Tomaz and B. Mizaikoff,
et al., Surface Physicochemical Properties at the Micro and Nano Length Scales: Role on bacterial adhesion and Xylella fastidiosa biofilm development, PLoS One, 2013, 8(9), e75247 CrossRef CAS PubMed.
- S. Saran, M. Bisht, K. Singh and U. Teotia, Comparing adhesion attributes of two isolates of Lactobacillus acidophilus for assessment of prebiotics, honey and inulin, Int. J. Sci. Res. Publ., 2012, 2(1), 2250–3153 Search PubMed.
- S. L. Chiang, R. K. Taylor, M. Koomey and J. J. Mekalanos, Single amino acid substitutions in the N-terminus of Vibrio cholerae TcpA affect colonization, autoagglutination, and serum resistance, Mol. Microbiol., 1995, 17(6), 1133–1142 CrossRef CAS PubMed.
- F. D. Menozzi, P. E. Boucher, G. Riveau, C. Gantiez and C. Locht, Surface-associated filamentous hemagglutinin induces autoagglutination of Bordetella pertussis, Infect. Immun., 1994, 62(10), 4261–4269 CAS.
- A. Mundi, V. Delcenserie, M. Amiri-Jami, S. Moorhead and M. W. Griffiths, Cell-free preparations of Lactobacillus acidophilus strain La-5 and Bifidobacterium longum strain NCC2705 affect virulence gene expression in Campylobacter jejuni, J. Food Prot., 2013, 76(10), 1740–1746 CrossRef CAS PubMed.
- W. Ding, H. Wang and M. W. Griffiths, Probiotics down-regulate flaA sigma28 promoter in Campylobacter jejuni, J. Food Prot., 2005, 68(11), 2295–2300 CrossRef CAS PubMed.
|
This journal is © The Royal Society of Chemistry 2019 |
Click here to see how this site uses Cookies. View our privacy policy here.