DOI:
10.1039/C8FO02052C
(Paper)
Food Funct., 2019,
10, 235-243
Housefly (Musca domestica) larvae powder, preventing oxidative stress injury via regulation of UCP4 and CyclinD1 and modulation of JNK and P38 signaling in APP/PS1 mice
Received
20th October 2018
, Accepted 19th November 2018
First published on 12th December 2018
Abstract
Housefly (Musca domestica) Larvae powder (HL) is rich in antioxidants. As oxidative stress is considered as one of the main pathogenesis in Alzheimer's Disease (AD), this study was designed to explore the protective effects of HL as an antioxidant on APP/PS1 mice. 2-Month-old APP/PS1 mice were divided into a model control (MC) group, a Donepezil group and a HL group, and C57BL/6 mice were used as the normal control (NC) group. After 180 days of treatment, the memory ability was measured by Morris Water Maze (MWM). The presence of Aβ and the expression of Uncoupling Protein 4 (UCP4) and CyclinD1 were detected by immunohistochemistry. The expressions of Superoxide Dismutase 1 (SOD1), Catalase (CAT) and Mitogen-activated Protein Kinase (MAPK) signal pathways were measured by western blotting. Compared with untreated APP/PS1 mice, the memory abilities of the HL-treated mice were significantly improved. Furthermore, the HL treatment not only down-regulated the deposition of Aβ and the expression of CylinD1, but also increased both the mRNA and protein levels of SOD, CAT, and UCP4, and enhanced the phosphorylation of JNK and P38 MAPK activation. In conclusion, these results suggest that HL may have a protective effect against memory impairment and prevent oxidative stress-induced injury via the regulation of UCP4 and CyclinD1 and the modulation of JNK and P38 MAPK signaling in AD.
1. Introduction
Alzheimer's disease (AD) is a degenerative disease of the central nervous system, which is characterized by progressive dementia and cognitive impairments. The main pathological features are senile plaques composed of extracellular amyloid-β (Aβ) peptide, intracellular neurofibrillary tangles (NFTs) composed of hyper-phosphorylated tau, and loss of neurons.1,2 Since the exact pathogenesis of AD is unclear currently, there are no effective medications or supplements for it.
Oxidative stress is one of the most important factors contributing to the underlying pathology of AD.3,4 Some studies have shown that oxidative stress impairs neurons in the early stages of AD.5 Uncoupling proteins (UCPs) are a family of mitochondrial inner membrane proteins which are transporters, including UCP1 to UCP5. Particularly, UCP4 is detected mainly in the central nervous system.6,7 UCP4 can maintain the function of mitochondria in neural tissue, enhance neuronal survival8 and relieve oxidative stress.9,10
Adult neurons are believed to be in a quiescent state after they are differentiated. Cell cycle reactivation (CCR) means mature neurons re-enter various phases of the cell cycle, resulting from the abnormal activation of cell cycle regulators, such as Cyclin.11 The expression of Cyclin D and Cyclin B in the affected neurons of patients suffering from AD was shown to be abnormally higher than those of healthy people at the same age.12 In addition, some studies have suggested that oxidative stress induces neuronal injury via CCR,13 and antioxidants decreased the expression of Cyclin D1 to prevent cell cycle disorder.14 Therefore, CCR may be associated with AD and oxidative stress, however the molecular mechanism has not yet been demonstrated.
Housefly (Musca domestica) is a common insect in nature and its larvae (HL) are rich in nutrition. HL has been shown to exhibit antiviral and15,16 antifungal activities,16 anti-atherosclerosis pro-inflammatory responses17 and antitumor18 effects. Furthermore, some studies have shown that HL is rich in antioxidants19 and is able to scavenge oxidative radicals.20,21 Our previous study has shown that HL contains several anti-oxidative proteins, including cuticle proteins, catalase proteins, AhpC/TSA family proteins, antibacterial peptide, heat shock proteins etc., which contribute to the anti-oxidative protection of HL.19 However, there are limited studies available on the antioxidant protection of HL in AD. Therefore, this study aimed to investigate the neuro-protective effect of HL on APP/PS1 mice, and explored the possible molecular mechanism involved in it. We also compared the effects of HL on Donepezil, which was included as a positive control.
2. Materials and methods
2.1 Drugs and reagents
Donepezil was obtained from Eisai Co., Ltd (China). SOD1 and CAT antibodies were from OriGene Technologies Co., Ltd (USA). UCP4 and CyclinD1 antibodies were from Novus Biologicals Co., Ltd (USA). Horseradish peroxide-labeled goat-anti-rabbit and goat-anti-mouse IgG were obtained from Cell Signaling Technology Co., Ltd (USA). DAB staining solution was obtained from Sangon Biotech Co., Ltd (China). Other reagents were obtained from Beyotime Biotechnology Co., Ltd (China) or Tianjin Baishi Chemical Industry Co., Ltd (China).
2.2 Preparation of HL powder
HL was purchased from Yaodetang (China) and cleaned with distilled water and disinfected with 70% ethanol. Physiological saline was added to a mass-to-volume ratio of 1
:
1. HL was turned into a mixture by using a grinder. To obtain HL powder, the mixture was defatted by the Soxhlet extraction method,22 and was filtered through a 100-mesh gauze, and the filtered liquid was vacuum-dried. A moderate amount of HL powder was dissolved in physiological saline before using for daily gastric gavage. According to previous research, HL has no oral toxicity in mice23–25 and 200 mg kg−1 of HL was effective in delaying aging in vivo;26 we chose 200 mg kg−1 as the dosage of HL in our study.
2.3 Animals and drug treatment
Thirty 2-month-old APPSwe/PS1dE9 mice were provided by Nanjing Biomedical Research Institute of Nanjing University. These mice were randomly divided into three groups: a model control (MC) group, a Housefly Larvae (HL) group and a Donepezil group (the positive control). Ten 2-month-old C57BL/6 mice were purchased from Guangdong Medical Laboratory Animal Center, and were considered as the normal control (NC) group. Drug treatment started from 2-month-old to 8-month-old for a total period of 180 days. Drugs (HL: 200 mg kg−1; Donepezil: 5.7 mg kg−1) were administered intra-gastrically. The other groups were given the same volume of saline. The mice were housed at a specific-pathogen-free facility at Guangdong Pharmaceutical University, and were maintained on a 12 h light/dark cycle, with free access to food and water. All the study protocols involving animals in this study were approved by the Guangdong Pharmaceutical University Animal Care and Use Committee (NO. gdpulac20150157).
2.4 Morris water maze (MWM)
The MWM was used to evaluate the learning memory abilities in mice.27,28 Briefly, a circular pool was filled with a 24 ± 1 °C opaque water–milk mixture. A video camera with a tracking system (Chengdu Techman Software, China) was automatically used to record data. Mice were trained to find the platform for five days in a water maze every day. A maximum time of 90 s was provided to each mouse. If they failed to find the platform in 90 s, the mice would be guided to the platform and kept on the platform for 10 s. The system recorded the time needed for each mouse to reach the platform (latency). The platform was removed on the sixth day. The times covered in the previous platform position by each mouse was recorded to assess the memory ability.
2.5 Immunohistochemical (IHC) staining
Paraffin-embedded brain tissues were prepared and the sections were incubated with primary antibodies (dilution 1
:
400 for Aβ, 1
:
400 for CyclinD1 and 1
:
100 for UCP4) at 4 °C overnight, followed by incubation with horseradish peroxide-labeled goat-anti-mouse (for Aβ and CyclinD1) and goat-anti-rabbit (for UCP4) IgG working solution. Then sections were stained in DAB and counter-stained with hematoxylin. Finally, each cover-slipped section was examined under a microscope at 100× magnification.
2.6 Western blotting
The proteins were extracted from hippocampus tissue. The protein concentration was determined using a Bradford assay (Sangon, Shanghai, China). The protein extract (10 μg) was resolved by SDS-PAGE according to standard protocols and transferred to a nitrocellulose membrane. The membranes were blocked in fat-free milk. Primary antibodies including SOD1 (1
:
1000, Origene, USA), CAT (1
:
200, Origene, USA), Tubulin (1
:
2000, Seebio, China) and GAPDH (1
:
500, Xianzhi, China) were incubated at 4 °C overnight. Secondary antibodies were goat anti-mouse or goat anti-rabbit IgG (1
:
2000, Cell Signaling Technology, USA).
2.7 Real time reverse transcription-polymerase chain reaction (RT-PCR)
RT-PCR was used to analyze the levels of CAT, SOD1, UCP4 and CyclinD1 mRNA. The brains were placed on ice. A general RNA extraction kit (Dongsheng Biotech) was used for the preparation of total RNA in the hippocampus tissue. A HiScript II Q RT SuperMix Kit (Vazyme Biotech, China) was used to prepare cDNA from 1 μg RNA. The corresponding primer sequences of each detected protein are shown in Table 1.
Table 1 Primer pairs used in RT-PCR
Gene |
Primers |
Sequences |
Amplicon (bp) |
UCP4 |
Forward |
5′-TCG AGA CAA ACA AGG AAG GGG-3′ |
132 |
Reverse |
5′-GAC CAA GGG GTC ATT CTC AGC-3′ |
CAT |
Forward |
5′-CCC CTA TTG CCG TTC GAT TCT-3′ |
211 |
Reverse |
5′-TTC AGG TGA GTC TGT GGG TTT-3′ |
SOD1 |
Forward |
5′-GGG TTC CAC GTC CAT CAG TA-3′ |
132 |
Reverse |
5′-AGT CAC ATT GCC CAG GTC TC-3′ |
CyclinD1 |
Forward |
5′-TCC TGC TAC CGC ACA ACG-3′ |
175 |
Reverse |
5′-GAC CAG CCT CTT CCT CCA C-3′ |
β-Actin |
Forward |
5′-AGA GGG AAA TCG TGC GTG AC-3′ |
138 |
Reverse |
5′-CAA TAG TGA TGA CCT GGC CGT-3′ |
2.8 Statistical analysis
All data were analyzed using Prism 5 software and expressed as mean ± SD. Statistical analysis was performed by one-way ANOVA followed by Tukey's tests for multiple comparisons. Differences with P < 0.05 were considered statistically significant.
3. Results
3.1 Effects of HL on escape latency of APP/PS1 mice in the MWM test
APP/PS1 mice were trained in the MWM to test their spatial learning and memory abilities. As shown in Fig. 1a, the escape latency (the time to find the hidden platform) was not significantly different between groups on the first day. After 3 days of training, the escape latency was significantly shortened in the NC, HL and Donepezil groups compared to that of the MC group (P < 0.05) (Fig. 1b). The results showed that the administration of HL or Donepezil caused a significant reduction in escape latency compared with the MC group (P < 0.05). In addition, the escape latency in the HL group was significantly less than that of the Donepezil group (P < 0.05).
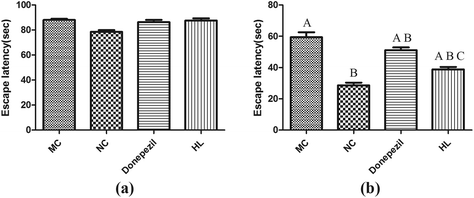 |
| Fig. 1 The mean of latencies to find a hidden platform in the MWM. (a) Escape latency on the first day; (b) escape latency on the third day after training. Data are presented as mean ± S.E.M. (A: P < 0.05 vs. NC; B: P < 0.05 vs. MC; C: P < 0.05 vs. Donepezil). | |
3.2 Effect of HL on the deposition of Aβ in the hippocampus
The photomicrograph of IHC results showed that there was no deposition of Aβ in the NC group. By contrast, the MC group had a massive deposition of Aβ in the hippocampus (Fig. 2). In the groups treated with Donepezil and HL, the deposition of Aβ was notably decreased compared with that of the MC group (P < 0.05).
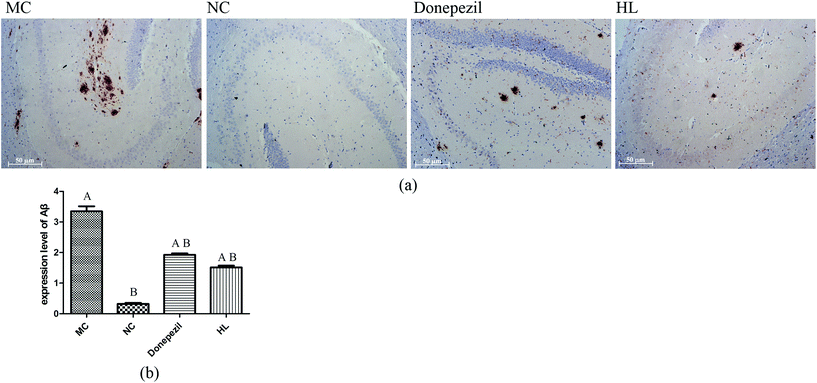 |
| Fig. 2 IHC staining of Aβ deposition in hippocampus tissue of mice in the experimental groups. (a) Photomicrograph of Aβ deposition in all groups (scale bar = 50 μm). (b) The analysis data of Aβ deposition in the hippocampus of all groups by Image J. Data are presented as mean ± S.E.M. (A: P < 0.05 vs. NC; B: P < 0.05 vs. MC; magnification 100×). | |
3.3 Effect of HL on UCP4 and CyclinD1 expression
As illustrated in Fig. 3(a, c and e), the percentage of immuno-positive neurons of UCP4 in the hippocampus was remarkably lower in the MC group (P < 0.05) than that in the NC group. After treating with HL, the expression of UCP4 was significantly enhanced compared with that of the MC group (P < 0.05) while the expression of UCP4 was not statistically different between the Donepezil group and the MC group. Moreover, as shown in Fig. 3(b, d and f), the percentage of immuno-positive neurons of CyclinD1 in the hippocampus was dramatically higher in the MC group than that in the NC group (P < 0.05). After treating with HL and Donepezil, the expression of CyclinD1 was significantly decreased compared with that of the MC group (P < 0.05). The above-mentioned results were all verified from the levels of both protein and mRNA.
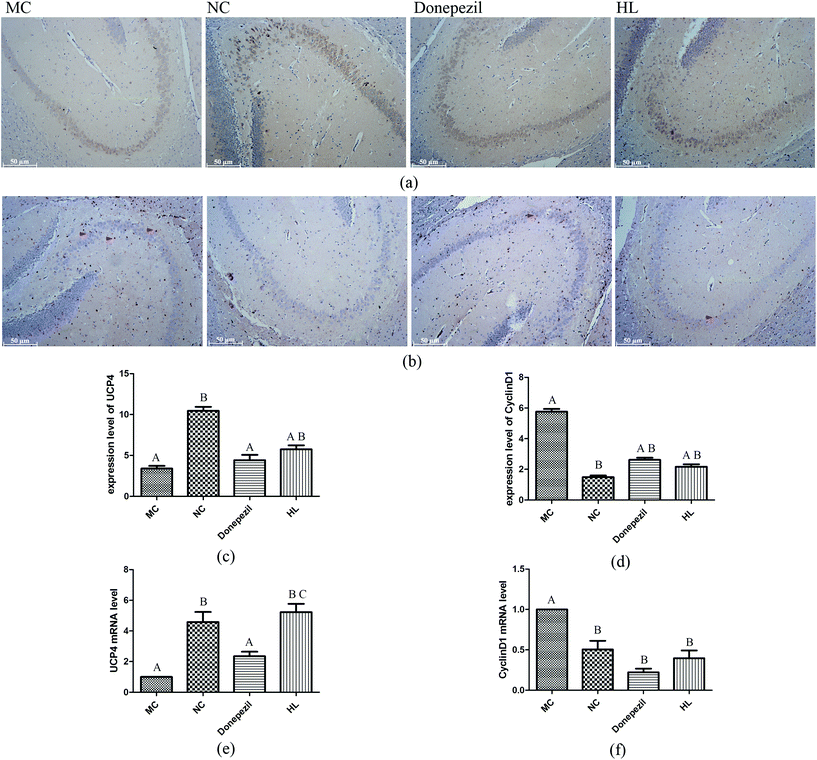 |
| Fig. 3 IHC staining of UCP4 and CyclinD1 in hippocampus tissue of mice in the experimental groups. (a and b) Photomicrograph of UCP4 and CyclinD1 in the hippocampus (scale bar = 50 μm). (c and d) The analysis data of UCP4 and CyclinD1 protein levels in the hippocampus by Image J. (e and f) The UCP4 and CyclinD1 mRNA levels in the hippocampus (A: P < 0.05 vs. NC; B: P < 0.05 vs. MC; magnification 100×). | |
3.4 Effect of HL on CAT and SOD1 levels
As shown in Fig. 4(c–f), the levels of CAT and SOD1 in the MC group were remarkably lower than those in the NC group (P < 0.05). In addition, both levels of CAT and SOD1 in the HL group were significantly increased compared with those in the MC group (P < 0.05), while the level of SOD1, not CAT, in the Donepezil group was significantly higher than that in the MC group (P < 0.05). Besides, the level of CAT in the HL group was significantly higher than that in the Donepezil group (P < 0.05).
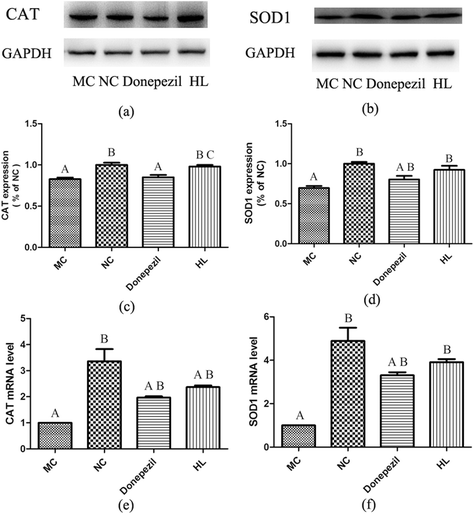 |
| Fig. 4 Expression of SOD1 and CAT in the hippocampus of mice in the experimental groups. (a and b) Western blotting results of the expression of CAT and SOD1. (c and d) The relative density-analysis of western blotting using a Tanon Gel Imaging system. (e and f) The analysis of CAT and SOD1 mRNA levels. (A: P < 0.05 vs. NC; B: P < 0.05 vs. MC; C: P < 0.05 vs. Donepezil). | |
3.5 Effect of HL on the expression of p-JNK and p-P38
As shown in Fig. 5(c and d), the expression levels of p-JNK and p-P38 in the MC groups were significantly lower than those in the NC group (P < 0.05). Compared with the MC group, the levels of p-JNK and p-P38 in the HL group were significantly increased (P < 0.05). However, Donepezil-treatment only improved JNK (P < 0.05), but not P38 activation, which suggests that HL enhanced JNK and P38 MAPK activation in APP/PS1 mice, while Donepezil only enhanced JNK activation. Otherwise, the degree of activation in JNK and P38 MAPK signaling in the HL group was significantly higher than that in the Donepezil group (P < 0.05).
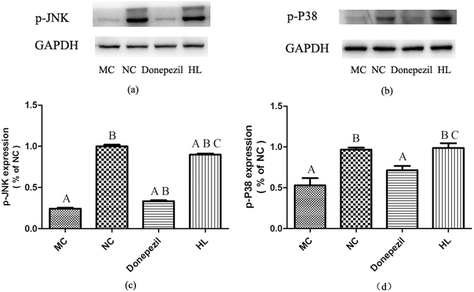 |
| Fig. 5 Expression of p-JNK and p-P38 in the hippocampus of mice in the experimental groups. (a and b) Western blotting results of the expression of p-JNK and p-P38. (c and d) The relative density-analysis of western blotting using a Tanon Gel Imaging system. (A: P < 0.05 vs. NC; B: P < 0.05 vs. MC; C: P < 0.05 vs. Donepezil). | |
4. Discussion
MWM is widely used to evaluate the memory and learning abilities in mouse models of AD.27 A short escape latency could be associated with the good memory of mice.29 In the present study, we observed a significantly longer escape latency in the MC group than that in the NC group after training. Donepezil and HL significantly shortened the escape latency. The results revealed that HL was protective against learning and memory impairment, which was as effective as Donepezil.
The aggregation of beta-amyloid peptide (Aβ) is characteristic of AD, which leads to senile plaques.30 The deposition of Aβ in the hippocampal region can induce oxidative stress, contributing to neuronal death.31 Many studies have demonstrated that Aβ promotes reactive oxygen species (ROS) production, and oxidative damage also induces the aggregation of Aβ in neuronal cells.32–34 In the present study, we found that HL and Donepezil significantly decreased the accumulation of Aβ in the hippocampus. Furthermore, HL, an antioxidant,19 inhibited Aβ-induced oxidative damage, and oxidative stress induced Aβ deposition through decreasing the oxidative stress level in hippocampal neurons.
Adult neurons are terminally differentiated and remain in the G0 phase of the cell cycle. Cell cycle-related proteins participate in cell cycle regulation, such as Cyclin, CDK, etc. However, it has been reported that the expression of cell cycle-related proteins was identified in the AD brain.35 It was reported that Aβ induced neuronal damage and CDK5 over-activation.36 Aβ promoted ROS production and CDK5 activation through disrupting the antioxidant system, leading to cell death.37 Cyclin D is a member of the cyclin protein family regulating cell cycle progression, including CyclinD1, CyclinD2, and CyclinD3. Among the proteins, Cyclin D1 is a crucial factor, which provokes neurons to leave the G0 phase and enter into the G1 phase. Some studies indicated that CyclinD1 can be detected in the affected neurons from the AD brain.35 In the present study, we found that the level of CyclinD1 was significantly higher in the MC group than that in the NC group. HL and Donepezil treatment significantly down-regulated the CyclinD1 level, alleviating neuronal damage. However, there are no significant differences between HL and Donepezil groups. HL is a natural antioxidant with non-toxicity23,25 and can be taken as a precaution against AD, which is better than Donepezil in animal models.
Uncoupling proteins (UCPs) are the family of mitochondrial anion transporters, including UCP1 to UCP5. UCP2 and 4 mainly express in the brain tissue of mammals. Both of them play an important role in the function of the central nervous system. UCPs can be neuro-protective agents in neurodegenerative diseases through reducing the production of ROS.38–40 Indeed, it has been observed that the mRNA level of UCP4 was remarkably decreased in the brain of AD patients.41 It is suggested that the function of UCP4 may be associated with preventing oxidative injury in AD. The current study demonstrated that the down-regulation of UCP4 in MC mice might aggravate neuron damage by inducing oxidative stress. The oxidative stress further induced the aggregation of Aβ, and led to neuronal death. In the HL group, the expression of UCP4 was remarkably up-regulated, which could decrease ROS production, and protect neurons against oxidative injury in the hippocampus of APP/PS1 mice. HL-treatment may be responsible for enhancing the expression of UCP4 and therefore it alleviates neuronal oxidative damage. However, Donepezil doesn't have an obvious effect on the expressional level of UCP4. Hence, Donepezil doesn't protect against AD via the regulation of UCP4.
Superoxide dismutase (SOD1) is one of the antioxidant enzymes, converting harmful superoxide radicals to hydrogen peroxide and molecular oxygen.42 Catalase (CAT) is another antioxidant enzyme that catalyzes the breakdown of hydrogen peroxide to oxygen and water.43 Both SOD1 and CAT are antioxidant defense systems against oxidative stress in vivo. The expression of SOD1 is decreased in the AD patients rather than in individuals without AD.44 Furthermore, a decrease in CAT level is seen in the cognitive deficits of rat models.45 In the present study, we found that the expressions of SOD1 and CAT in the hippocampus of MC mice were significantly decreased compared to NC mice, suggesting an impaired antioxidant capacity in AD mice. However, the expressions of SOD1 and CAT were significantly increased following HL-treatment and Donepezil-treatment. In particular, the expression of CAT in HL mice was significantly higher than that in Donepezil mice. These results indicated that HL may be more remarkable than Donepezil in relieving the oxidative stress in APP/PS1 mice.
Mitogen-Activated Protein Kinase (MAPK) signaling includes JNK and P38 signal pathways. Each one contributes to the physiologic function of cells, including the cell cycle, cell differentiation and cell survival.46,47 A neuro-protective agent has been observed to affect neurodegenerative rats by the activation of JNK.48 Antioxidant agents protect PC12 cells against tunicamycin mediated damage via enhancing JNK and P38 signal pathways.49 The reported results are similar to the results in our present study. The expression levels of p-JNK and p-P38 in the MC group were significantly lower compared with the NC group. HL-treatment significantly enhanced the activation of MAPK signaling, including JNK and P38 pathways. The results suggest that the activation of MAPK signaling helped neurons combat oxidative stress injury. Therefore, HL may have potential therapeutic effects in AD, which are associated with the activation of P38 and JNK signaling. However, we observed that the activation of P38 wasn't dramatically changed after Donepezil-treatment. Hence, Donepezil might protect against AD through only the JNK signal pathway, but not the P38 pathway. Additionally, we found the lower activation of JNK and P38 signal pathways accompanied by lower expression of UCP4 in APP/PS1 mice. Meanwhile, there is significantly enhanced activation of JNK and P38 signal pathways and the significant up-regulation of UCP4 after HL-treatment. These results suggest that JNK and P38 signal pathways may be involved in the regulation of UCP4 expression following HL-treatment. However, the relationship among UCP4, JNK, and P38 signaling in AD needs to be further confirmed.
According to our recently on-line published data, HL is a mixture rich in antioxidant proteins, including catalase proteins, AhpC/TSA family protein, antibacterial peptide and so on.19 Though the above results in this paper showed the antioxidant protection of HL in AD, it is not known which protein is the exact one that works. In our next work, we plan to use the identified pure anti-oxidative protein of HL, such as the antibacterial peptide, AhpC/TSA family protein, etc. to test their protective activities in AD individually.
5. Conclusion
In summary, HL has a protective effect on AD. The molecular mechanism may be the up-regulation of UCP4, SOD1, and CAT, and the down-regulation of CyclinD1 via the activation of P38 and JNK MAPK signal pathways, and a reduction of oxidative injury (Fig. 6). Hence, HL might become a promising food adjuvant for the treatment of AD and further investigation is warranted.
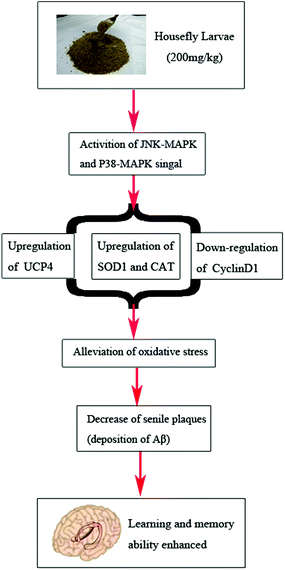 |
| Fig. 6 Potential neuro-protective mechanism of HL against oxidative damage in APP/PS1 mice derived from the above results. | |
Conflicts of interest
The authors declared no conflicts of interest.
Acknowledgements
The present study was supported by grants from the National Natural Science Foundation of China (81401150 and 81403129). The authors thank Associate Professor Shailendra Anoopkumar-Dukie, who provided guidance and critical help in manuscript drafts.
References
- J. A. Hardy and G. A. Higgins, Alzheimer's disease: the amyloid cascade hypothesis, Science, 1992, 256, 184–185 CrossRef CAS PubMed.
- R. Hollister, H. West, S. Mui, J. H. Growdon, R. C. Petersen, J. E. Parisi and B. T. Hyman, Neuronal loss correlates with but exceeds neurofibrillary tangles in Alzheimer's disease, Ann. Neurol., 2010, 41, 17–24 Search PubMed.
- W. R. Markesbery, Oxidative stress hypothesis in Alzheimer's disease, Free Radicals Biol. Med., 1997, 23, 134–147 CrossRef CAS.
- T. Kanamaru, N. Kamimura, T. Yokota, K. Iuchi, K. Nishimaki, S. Takami, H. Akashiba, Y. Shitaka, K. I. Katsura and K. Kimura, Oxidative stress accelerates amyloid deposition and memory impairment in a double-transgenic mouse model of Alzheimer's disease, Neurosci. Lett., 2015, 587, 126–131 CrossRef CAS.
- S. I. Mota, O. C. Rui, I. L. Ferreira, I. Santana, G. L. Caldeira, C. Padovano, A. C. Fonseca, I. Baldeiras, C. Cunha and L. Letra, Oxidative stress involving changes in Nrf2 and ER stress in early stages of Alzheimer's disease, Biochim. Biophys. Acta, 2015, 1852, 1428–1441 CrossRef CAS PubMed.
- W. Mao, X. X. Yu, A. Zhong, W. Li, J. Brush, S. W. Sherwood, S. H. Adams and G. Pan, UCP4, a novel brain-specific mitochondrial protein that reduces membrane potential in mammalian cells, FEBS Lett., 1999, 449, 326–330 CrossRef.
- A. Smorodchenko, A. Rupprecht, I. Sarilova, O. Ninnemann, A. U. Bräuer, K. Franke, S. Schumacher, S. Techritz, R. Nitsch and M. Schuelke, Comparative analysis of uncoupling protein 4 distribution in various tissues under physiological conditions and during development, Biochim. Biophys. Acta, Biomembr., 2009, 1788, 2309–2319 CrossRef CAS.
- L. H. Perreten, M. Zenger, G. Azarias, J. Y. Chatton, P. J. Magistretti and S. Lengacher, Control of Mitochondrial pH by Uncoupling Protein 4 in Astrocytes Promotes Neuronal Survival, J. Biol. Chem., 2014, 289, 31014–31028 CrossRef PubMed.
- D. Liu, S. L. Chan, N. C. D. Souzapinto, J. R. S. Jr, R. P. Wersto, M. Zhan, K. Mustafa, R. D. Cabo and M. P. Mattson, Mitochondrial UCP4 mediates an adaptive shift in energy metabolism and increases the resistance of neurons to metabolic and oxidative stress, NeuroMol. Med., 2006, 8, 389–413 CrossRef CAS.
- S. Cardoso, S. Correia, C. Carvalho, E. Candeias, A. I. Plácido, A. I. Duarte, R. M. Seiça and P. I. Moreira, Perspectives on mitochondrial uncoupling proteins-mediated neuroprotection, J. Bioenerg. Biomembr., 2015, 47, 119–131 CrossRef CAS.
- J. M. Frade and M. C. Ovejerobenito, Neuronal cell cycle: the neuron itself and its circumstances, Cell Cycle, 2015, 14, 712–720 CrossRef CAS.
- Y. Yang, E. J. Mufson and K. Herrup, Neuronal Cell Death Is Preceded by Cell Cycle Events at All Stages of Alzheimer's Disease, J. Neurosci., 2003, 23, 2557–2563 CrossRef CAS PubMed.
- J. A. Klein and S. L. Ackerman, Oxidative stress, cell cycle, and neurodegeneration, J. Clin. Invest., 2003, 111, 785–793 CrossRef CAS.
-
Z. Y. J. Zheng, The transport removals and regulation of Tenuigenin on Beta-Amyloid-induced PC12 cells function and cell cycle, Master's Degree, Guangdong Pharmaceutical University, 2016 Search PubMed.
- X. Lu, X. Jin, J. Wang, F. Chu and J. Zhu, Antihepatitis B Virus Activity of a Protein-Enriched Fraction from Housefly (Musca domestica) in a Stable HBV-Producing Cell Line, Sci. World J., 2014, 2014, 389560 Search PubMed.
- J. Zhou, L. Kong, N. Fang, B. Mao and H. Ai, Synthesis and
Functional Characterization of MAF-1A Peptide Derived From the Larvae of Housefly, Musca domestica (Diptera: Muscidae), J. Med. Entomol., 2016, 53, 1467–1472 CrossRef CAS.
- F. J. Chu, X. B. Jin and J. Y. Zhu, Housefly maggots (Musca domestica) protein-enriched fraction/extracts (PE) inhibit lipopolysaccharide-induced atherosclerosis pro-inflammatory responses, J. Atheroscler. Thromb., 2011, 18, 282 CrossRef CAS.
- L. Hou, Y. Shi, P. Zhai and G. Le, Antibacterial activity and in vitro anti-tumor activity of the extract of the larvae of the housefly (Musca domestica), J. Ethnopharmacol., 2007, 111, 227–231 CrossRef PubMed.
- H. F. Mei, J. Xu, Y. He, X. Yang, W. Liu, W. Tian, Yu Zeng and J. Zhu, Protein-rich extract of Musca domestica larvae alleviated metabolic disorder in STZ-induced type 2 diabetic rat model via hepatoprotective and pancreatic β-cell protective activities, J. Biosci., 2018, 43(5), 969–983 CrossRef CAS.
- L. Zhu, P. Wang, Q. L. Qin, H. Zhang and Y. J. Wu, Protective effect of polypeptides from larva of housefly (Musca domestica) on hydrogen peroxide-induced oxidative damage in HepG2 cells, Food Chem. Toxicol., 2013, 60, 385–390 CrossRef CAS.
- H. Ai, F. Wang, N. Zhang, L. Zhang and C. Lei, Antiviral, Immunomodulatory, and Free Radical Scavenging Activities of a Protein-Enriched Fraction from the Larvae of the Housefly, Musca domestica, J. Insect Sci., 2014, 13, 112 Search PubMed.
-
L. M. Harwood and C. Moody, Experimental organic chemistry: principles and practice, 1989 Search PubMed.
- Y. X. Li, X. B. Jin, F. J. Chu, M. Y. Liu, D. Y. Shi and J. Y. Zhu, Scientific evaluation of the subchronic toxicity of musca domestica larvae extracts in Sprague Dawley rats, Food Chem. Toxicol., 2013, 59, 464–469 CrossRef CAS PubMed.
-
Li Yunxi, Safety Evaluation of Musca domestica Larvae Powder, Doctoral dissertation, Guangdong Pharmaceutical University, 2010 Search PubMed.
- Li Yunxi, Z. Jiayong, J. Xiaobao, Z. Aihua and C. Fujiang, Acute oral toxicity and mutagenicity of powder extracted from larvae of Musca domestica in mice, China J. Public Health, 2010, 26, 1416–1417 Search PubMed.
- F. Panpan, Lu Xuemei, J. Xiaobao and Z. Jiayong, Anti-aging effect of luoxianzi extract on D–gal-induced aging mice, J. Guangzhou Pharm. Univ., 2015, 31, 479–482 Search PubMed.
- R. D'Hooge and P. P. De Deyn, Applications of the Morris water maze in the study of learning and memory, Brain Res. Rev., 2001, 36, 60–90 CrossRef.
- L. Wang, H. C. Chiang, W. Wu, B. Liang, Z. Xie, X. Yao, W. Ma, S. Du and Y. Zhong, Epidermal growth factor receptor is a preferred target for treating amyloid-β-induced memory loss, Proc. Natl. Acad. Sci. U. S. A., 2012, 109, 16743–16748 CrossRef CAS PubMed.
- D. H. Malin, M. K. Crothers, J. R. Lake, P. Goyarzu, R. E. Plotner, S. A. Garcia, S. H. Spell, B. J. Tomsic, T. Giordano and N. W. Kowall, Hippocampal Injections of Amyloid β-Peptide 1-40 Impair Subsequent One-Trial/Day Reward Learning, Neurobiol. Learn. Mem., 2001, 76, 125–137 CrossRef CAS PubMed.
- P. Cras, M. Kawai, D. Lowery, P. Gonzalez-Dewhitt, B. Greenberg and G. Perry, Senile Plaque Neurites in Alzheimer Disease Accumulate Amyloid Precursor Protein, Proc. Natl. Acad. Sci. U. S. A., 1991, 88, 7552–7556 CrossRef CAS.
- C. Chen, B. Li, G. Cheng, X. Yang, N. Zhao and R. Shi, Amentoflavone Ameliorates Aβ 1–42 -Induced Memory Deficits and Oxidative Stress in Cellular and Rat Model, Neurochem. Res., 2018, 1–12 Search PubMed.
- K. O. Chay, K. Y. N. Koong, S. Hwang, J. K. Kim and C. S. Bae, NADPH Oxidase Mediates β-Amyloid Peptide-Induced Neuronal Death in Mouse Cortical Cultures, Chonnam Med. J., 2017, 53, 196–202 CrossRef PubMed.
- Q. Q. Fu, L. Wei, J. Sierra, J. Z. Cheng, M. T. Moreno-Flores, H. You and H. R. Yu, Olfactory Ensheathing Cell-Conditioned Medium Reverts Aβ 25–35 -Induced Oxidative Damage in SH-SY5Y Cells by Modulating the Mitochondria-Mediated Apoptotic Pathway, Cell. Mol. Neurobiol., 2016, 37, 1043–1054 CrossRef PubMed.
- S. Jiang, Y. Zhao, T. Zhang, J. Lan, J. Yang, L. Yuan, Q. Zhang, K. Pan and K. Zhang, Galantamine inhibits β-amyloid-induced cytostatic autophagy in PC12 cells through decreasing ROS production, Cell Proliferation, 2018, 51, 12427 CrossRef PubMed.
- L. A. G. V. Leeuwen and J. J. M. Hoozemans, Physiological and pathophysiological functions of cell cycle proteins in post-mitotic neurons: implications for Alzheimer's disease, Acta Neuropathol., 2015, 129, 511–525 CrossRef PubMed.
- A. Wilkaniec, M. Gąssowska-Dobrowolska, M. Strawski, A. Adamczyk and G. A. Czapski, Inhibition of cyclin-dependent kinase 5 affects early neuroinflammatory signalling in murine model of amyloid beta toxicity, J. Neuroinflammation, 2018, 15, 1 CrossRef.
- J. Park, H. Choi, J. I. Min, B. Kim, S. A. Lee, J. W. Yun, M. O. Choi, K. A. Chang and D. E. Lee, Loss of mitofusin 2 links beta-amyloid-mediated mitochondrial fragmentation and Cdk5-inducedoxidative stress in neuron cells, J. Neurochem., 2015, 132, 687–702 CrossRef CAS.
- S. Xu, X. Yang, Y. Qian and Q. Xiao, Parkinson disease-related DJ-1 modulates the expression of uncoupling protein 4 against oxidative stress, J. Neurochem., 2018, 145, 312–322 CrossRef CAS PubMed.
- P. W. Ho, J. W. Ho, H. F. Liu, D. H. So, Z. H. Tse, K. H. Chan, D. B. Ramsden and S. L. Ho, Mitochondrial neuronal uncoupling proteins: a target for potential disease-modification in Parkinson's disease, Transl. Neurodegener., 2012, 1, 3 CrossRef CAS PubMed.
- M. Lu, X. L. Sun, C. Qiao, Y. Liu, J. H. Ding and G. Hu, Uncoupling protein 2 deficiency aggravates astrocytic endoplasmic reticulum stress and nod-like receptor protein 3 inflammasome activation, Neurobiol. Aging, 2014, 35, 421–430 CrossRef CAS PubMed.
- D. L. M. Sm and J. R. Wands, Molecular indices of oxidative stress and mitochondrial dysfunction occur early and often progress with severity of Alzheimer's disease, J. Alzheimer's Dis., 2006, 9, 167–181 Search PubMed.
- J. M. McCord and I. Fridovich, Superoxide dismutase. An enzymic function for erythrocuprein (hemocuprein), J. Biol. Chem., 1969, 244, 6049–6055 CAS.
- P. Chelikani, I. Fita and P. C. Loewen, Diversity of structures and properties among catalases, Cell. Mol. Life Sci., 2004, 61, 192–208 CrossRef CAS.
- K. Murakami, N. Murata, Y. Noda, S. Tahara, T. Kaneko, N. Kinoshita, H. Hatsuta, S. Murayama, K. J. Barnham and K. Irie, SOD1 (Copper/Zinc Superoxide Dismutase) Deficiency Drives Amyloid β Protein Oligomerization and Memory Loss in Mouse Model of Alzheimer Disease, J. Biol. Chem., 2011, 286, 44557–44568 CrossRef CAS PubMed.
- S. M. Pacheco, S. Msp, J. M. Gutierres, G. Mfb, F. B. Carvalho, J. H. Azambuja, S. Mrc, F. M. Stefanello and R. M. Spanevello, Anthocyanins as a potential pharmacological agent to manage memory deficit, oxidative stress and alterations in ion pump activity induced by experimental sporadic dementia of Alzheimer's type, J. Nutr. Biochem., 2018, 56, 193 CrossRef CAS.
- G. L. Johnson and R. Lapadat, Mitogen-activated protein kinase pathways mediated by ERK, JNK, and p38 protein kinases, Science, 2002, 298, 1911–1912 CrossRef CAS PubMed.
- K. Ono and J. Han, The p38 signal transduction pathway: activation and function, Cell. Signalling, 2000, 12, 1–13 CrossRef CAS.
- T. Dalli, M. Beker, S. Terziogluusak, F. Akbas and B. Elibol, Thymoquinone activates MAPK pathway in hippocampus of streptozotocin-treated rat model, Biomed. Pharmacother., 2018, 99, 391 CrossRef CAS PubMed.
- J. H. Yen, P. S. Wu, S. F. Chen and M. J. Wu, Fisetin Protects PC12 Cells from Tunicamycin-Mediated Cell Death via Reactive Oxygen Species Scavenging and Modulation of Nrf2-Driven Gene Expression, SIRT1 and MAPK Signaling in PC12 Cells, Int. J. Mol. Sci., 2017, 18, 852 CrossRef.
Footnote |
† These authors contributed equally to this work. |
|
This journal is © The Royal Society of Chemistry 2019 |
Click here to see how this site uses Cookies. View our privacy policy here.