DOI:
10.1039/C8FO01991F
(Paper)
Food Funct., 2019,
10, 304-314
Immunomodulatory activity of a water-soluble polysaccharide obtained from highland barley on immunosuppressive mice models
Received
12th October 2018
, Accepted 9th December 2018
First published on 12th December 2018
Abstract
A water-soluble polysaccharide (BP-1) was extracted and purified from highland barley (Hordeum vulgare L.) and its average molecular weight was about 6.7 × 104 Da. In this study, the immunomodulatory activity of BP-1 on the immunosuppressive BALB/c mice model and its molecular mechanism were elucidated. It was found that the weight indexes of spleen and thymus were significantly increased by BP-1 (80 mg kg−1 and 160 mg kg−1) treatment in the immunosuppressive mice model. The results showed that BP-1 (80 mg kg−1 and 160 mg kg−1) could significantly increase the number of bone marrow cells (BMC) and peripheral blood white blood cells (WBC) in the immunosuppressive mice model. In addition, the result further confirmed that BP-1 could increase the serum levels of IL-2, TNF-α and IFN-γ, so as to improve the immune function of immunosuppressive mice. The results showed that BP-1 (80 mg kg−1 and 160 mg kg−1) could promote the proliferation of spleen cells and the natural killer (NK) cell activity in vivo. The quantitative real-time polymerase chain reaction (qRT-PCR) and ELISA results revealed that BP-1 (80 mg kg−1 and 160 mg kg−1) could enhance the production of IL-2, TNF-α, IFN-γ, IgG and IgM in the spleen of immunosuppressive mice. The HE (hematoxylin and eosin) stained histopathological images showed that BP-1 (80 mg kg−1 and 160 mg kg−1) could repair the damage induced by CTX in the spleen cells of immunosuppressive mice. The result of macrophages showed that BP-1 (80 mg kg−1 and 160 mg kg−1) could promote the proliferation and phagocytosis activity of macrophages in immunosuppressive mice. Furthermore, BP-1 could activate macrophages by the TLR-4, TRAF6, TAK1 and nuclear factor κB (NF-κB) p65 pathways in vivo. These results suggested that BP-1 has a remarkable immunomodulatory activity on the immunosuppressive mice model.
1. Introduction
Barley (Hordeum vulgare L.) is a member of the Poaceae family and ranks fourth in the worldwide cereal crop production.1 It is generally classified as hulled or covered barley with husk, and hulless or naked barley without husk.2 Highland barley, called Qing Ke in China, is a cultivar of hulless barley. Highland barley is a principal food crop in the Qinghai–Tibet Plateau of China because it is the only crop that can be cultivated at high altitudes of 4200–4500 m above the sea level.3 With an increasing focus on dietary fibre and its health benefits, highland barley, with its high contents of these constituents, has gained increased attention over the last decade and has the potential to become more attractive for human consumption.4,5
The immune system is the body's defense against microbes and foreign antigens.6 The increasing proliferation of splenocytes in tumor bearing mice could activate the immune system and modulate the immune activity.7,8 Natural killer (NK) cells combat viruses and tumor cells as the body's first immune response to such stimuli. NK cells can exert direct cytotoxic activity against tumor cells (innate system), and also stimulate the production of cytokines (adaptive system).9 One of the most important non-specific immune activities in situ is phagocytosis performed by macrophages (as well as by other types of leukocytes). Phagocytosis is accompanied by the release of lysosomal enzymes like acid phosphatase (AP) used for the killing and digestion of microbial pathogens.10 Macrophage phagocytosis is also assigned by the liberation of free radicals such as nitric oxide (NO) and cytokines (IL-1β, IL-6, TNF-α, and IFN-γ), involved in pathogen killing.11 Therefore, there is considerable interest in discovering immunomodulatory agents that can enhance the body's immunity to a variety of diseases.
Toll-like receptor-4 (TLR-4) is a membrane protein receptor for the recognition of lipopolysaccharides (LPS) from Gram-negative bacteria, and participates in the body's immune response by promoting the synthesis and release of cellular immune factors and so on.12,13 Taking macrophages as an example, after external stimulation of TLR on the surface of the cell membrane, the pathogen-related analysis mode is activated.14,15 Then the signal is transferred to the intracellular pathway through a transmembrane structure, activating the downstream NF-κB pathway and promoting the translocation of NF-κB p65 into the nucleus, thereby promoting the synthesis and release of relevant immune factors such as various interleukins, TNF-α and IFN-γ.16
In a previous study, it has been reported that a highland barley polysaccharide of grains has potential inhibition effects on colon cancer.17 So far, there has been no published data about the beneficial effect of a highland barley polysaccharide on an immunosuppressive mice model. Thus, this study was designed to assess the effect of BP-1 on the immune system, using BALB/c mice as the animal model. The effects of BP-1 on the weight indexes of spleen and thymus in vivo were investigated for the first time. The number of BMC and peripheral blood WBC and the serum levels of IL-2, TNF-α, and IFN-γ in BALB/c mice were measured. Furthermore, the proliferation of spleen cells and the NK cell activity were investigated in vivo. ELISA was used to investigate the productions of IL-2, TNF-α, IFN-γ, IgG and IgM in the spleen of immunosuppressive mice. The histopathological image of the spleen was observed by HE (hematoxylin and eosin) staining to explore the immunomodulatory activity of BP-1 in vivo. In addition, the effects of BP-1 on the proliferation and phagocytosis activity of macrophages were investigated. The protein expressions of TLR-4, TRAF6, TAK1 and NF-κB p65 were measured by western blot in macrophages in vivo. Moreover, the mechanisms for the underlying immunomodulatory activities were assessed, focusing on the effects on immunosuppression. It was anticipated that the results generated from the present study would help develop novel functional foods in the future.
2. Materials and methods
2.1. Materials
Highland barley (Hordeum vulgare L. var. nudum Hook. f., Zangqing 25, Tibet, China) was obtained from the Academy of State Administration of Grain (Beijing, PR China). The barley samples were milled into whole grain flour using a 60-mesh size screen, thoroughly mixed and then stored at −20 °C. A water-soluble polysaccharide (BP-1) was extracted from highland barley (Hordeum vulgare L.) by using hot water and purified using Sepharose column chromatography. BP-1 contains 94.6% of total sugar as measured by the phenol–sulfuric acid method. No absorption was detected by the UV spectrum at 280 and 260 nm. It had a negative response to the Bradford method, indicating the inexistence of nucleic acid and protein in BP-1. It had an average molecular weight of about 6.7 × 104 Da and was composed of glucose (Glc), xylose (Xyl), arabinose (Ara) and rhamnose (Rha) with a relative molar ratio of 8.82
:
1.92
:
1.50
:
1.00.17
Penicillin–streptomycin solution, trypsin, phosphate buffer saline (PBS) and dimethyl sulfoxide side (DMSO) were purchased from Thermo (Beijing, China). 3-(4,5-Dimethylthiazol-2-yl)-2,5-diphenyltetrazolium bromide (MTT), lipopolysaccharide (LPS) and cytoxan (CTX) were purchased from Sigma (St. Louis, MO, USA). Fetal bovine serum, RPMI-1640 and DMEM medium were purchased from Gibco BRL (Grand Island, NY, USA). NF-κB p65, β-actin, TLR-4, TRAF6, TAK1 and horseradish peroxidase-conjugated secondary antibodies were purchased from Cell Signaling Technology (Danvers, MA, USA). The HE staining kit was purchased from Beyotime (Jiangsu, China). All other chemicals were of the analytically purest grade available.
2.2. Establishment of the immunosuppressive BALB/c mice model and treatments
Female BALB/c mice (six-week old), weighing 18 ± 2 g, were provided by the Experimental Animal Center of China Academy of Chinese Medical Sciences, Beijing, China. All animal procedures were performed in accordance with the Guidelines for Care and Use of Laboratory Animals of “Tianjin University of Science and Technology” University and approved by the Animal Ethics Committee of “Animal Ethical and Welfare Committee (AEWC)”. One hundred BALB/c mice were maintained in a pathogen-free environment (23 ± 2 °C, 55% ± 5% humidity) on a 12 h light or 12 h dark cycle, with free access to a standard pellet diet and water. 8 mg mL−1 BP-1 solution: 8 mg of BP-1 was dissolved in 1 mL of distilled water. 16 mg mL−1 BP-1 solution: 16 mg of BP-1 was dissolved in 1 mL of distilled water. 32 mg mL−1 BP-1 solution: 32 mg of BP-1 was dissolved in 1 mL of distilled water.
Cytoxan (CTX) was used for inducing the immunosuppressive models. The animals were randomly divided into 5 groups (10 BALB/c mice per treatment group) for various treatments (Table 1). These were the no CTX group (normal), the immunosuppressive model group (CTX), the 40 mg kg−1 BP-1 prevention group, the 80 mg kg−1 BP-1 prevention group, and the 160 mg kg−1 BP-1 prevention group. Except for the normal group, 100 mg kg−1 CTX was injected only on days 7–9 to establish the immunosuppressive mice model in other groups. In the BP-1 treatment groups, firstly, the mice were intragastrically administered with different doses of BP-1 for 14 days. On days 7–9 of the intragastric BP-1, the mice were injected with 100 mg kg−1 CTX (0.1 mL d−1) to investigate the protective effect of BP-1 on the immunosuppressive mice by CTX.
Table 1 Groups of BALB/c mouse with different treatments in vivo
Group |
Treatment |
Duration |
Normal |
Normal saline (0.1 mL d−1, gavage) |
14 straight days |
CTX |
100 mg kg−1 CTX (0.1 mL d−1, inject) |
Days 7–9 |
CTX + 40 mg kg−1 BP-1 |
40 mg kg−1 BP-1 (0.1 mL d−1, gavage) |
Days 1–14 |
CTX + 80 mg kg−1 BP-1 |
80 mg kg−1 BP-1 (0.1 mL d−1, gavage) |
Days 1–14 |
CTX + 160 mg kg−1 BP-1 |
160 mg kg−1 BP-1 (0.1 mL d−1, gavage) |
Days 1–14 |
2.3. Determination of thymus and spleen indexes
On the 14th day after various treatments, BALB/c mice in each group were killed through a cervical dislocation method. The spleen and thymus were dissected and weighed. The organ index was calculated as follows:
2.4. Assay of peripheral blood white cell count and the serum levels of IL-2, TNF-α, and IFN-γ
On the 14th day after various treatments, blood was collected from the eyes of mice and white blood cells were counted by using a blood cell analyzer (XT-2000iV, Japan, Sysmex). The blood samples were collected from the eyes of mice and the serum was separated by centrifugation at 3000 rpm for 10 min; then the upper layer was collected. The levels of IL-2, TNF-α and IFN-γ in the serum were evaluated by using commercial ELISA kits (R&D Systems, Minneapolis, MN) according to the manufacturer's instructions. The absorbance was measured in an ELISA reader at 450 nm. The concentrations of cytokines were calculated according to the standard curve using each of the recombinant cytokines in the ELISA kits.
2.5. Assay of bone marrow cell count
On the 14th day after various treatments, BALB/c mice in each group were killed through a cervical dislocation method. The intact femur was taken from one side of the mice and the soft tissue was removed. All bone marrow was rinsed using the centrifuge tube with PBS, and the volume was constant to 5 mL. The absolute number of bone marrow cells was counted by a count plate.
2.6. Preparation of splenocytes
On the 14th day after various treatments, BALB/c mice in each group were killed through a cervical dislocation method. After the mice were killed, they were soaked in 75% ethanol for 3 min for disinfection. The mouse spleen was taken out in a sterile environment for grinding and the splenocyte suspension was collected. The cells were treated with lysis buffer (Sorlabio Co., Beijing, China), and were centrifuged at 1000 rpm for 5 min to remove red blood cells. To remove adherent cells such as macrophages, the cells were incubated in a 6-well plate for 3 h. The collection of the unattached cell suspension was the splenocytes.
2.7. Assay of splenocyte proliferation
Splenocyte proliferation was assessed by using the MTT assay. Briefly, an aliquot of 0.1 mL of splenocytes (1 × 105 mL−1) was seeded in a 96-well flat-bottom microtiter plate, and then concanavalin A (Con A: Solarbio Co., Beijing, China) and a lipopolysaccharide (LPS: Solarbio Co., Beijing, China) (final concentration of 5 μg mL−1) were added, giving a final volume of 0.2 mL. After incubation at 37 °C in an incubator with 5% CO2 for 40 h, 20 μL of MTT solution (5 mg mL−1) was added to each well and incubated for another 4 h. The plate was centrifuged at 10
000g for 5 min and the supernatant was discarded, and 150 μL of DMSO was then added to each well. The absorbance at 570 nm was measured on a microplate reader (Thermo, USA).
2.8. Analysis of NK cell activity
The activity of natural killer (NK) cells was measured as previously described with some modifications. Briefly, NK-sensitive YAC-1 mouse lymphoma cells (purchased from Shanghai Institute of Cell Biology, Chinese Academy of Sciences, Shanghai, China), used as target cells, were seeded in 96-well plates at a density of 2 × 105 cells per well in DMEM. Splenocytes, used as the effector cells, were added at 1 × 107 cells per well to obtain effector/target cells at a ratio of 10
:
1. After incubation for 20 h, the cells were subjected to the MTT assay.
The NK cell activity was calculated as follows:
where OD
T is the optical density value of the control target cells, OD
S is the optical density value of the test samples and OD
E is the optical density value of the control effector cells.
2.9. The detection of the secretion levels of cytokines and Igs of the spleen in BALB/c mice by ELISA
After the mice were killed, they were soaked in 75% ethanol for 3 min for disinfection. The mouse spleen was taken out in a sterile environment and washed with sterile Hank's buffer twice. 100 mg of the spleen tissue was accurately weighed and cut, which was put into a homogenate tube. And then 2 mL normal saline was added into the homogenate tube to ice bath homogenate. After being centrifuged at 3500 rpm for 15 min, the upper layer was collected. The levels of IL-2, TNF-α, IFN-γ, IgG and IgM in the spleen were evaluated by using commercial ELISA kits (R&D Systems, Minneapolis, MN) according to the manufacturer's instructions. The absorbance was measured in an ELISA reader at 450 nm. The concentrations of cytokines were calculated according to the standard curve using each of the recombinant cytokines and Igs in the ELISA kits.
2.10. HE staining of spleen tissues
The spleen tissue blocks (3–5 μm thick) were cut with a freezing microtome and then used for HE staining using assay kits (Beyotime, Jiangsu, China), according to the manufacturer's protocols. Briefly, the samples were fixed in 10% (v/v) neutral buffered formaldehyde. Then the samples were rinsed thoroughly in PBS and stained with hematoxylin and eosin (H&E) stain. Finally, the sections were scanned by laser scanning confocal microscopy and all images were acquired using the same intensity and photodetector gain.
2.11. Assay of proliferation and phagocytic activity of macrophages
Macrophages were extracted from the abdominal cavity in mice and then examined by using Trypan Blue to ensure a 95% survival rate for the assay. The proliferation of macrophages was determined by the MTT assay. After the cells were cultured with RPMI-1640 medium supplemented with 10% fetal bovine serum for 48 h, the cells were incubated with 20 μL MTT solution (5 mg mL−1) in the medium for 4 hours at 37 °C. Viable cells convert the MTT into formazan, which generates a blue-purple color after dissolving in 150 μL DMSO. The absorbance at 570 nm was measured with a microplate reader (Thermo, USA). All tests were carried out in six independent experiments.
The phagocytic ability of macrophages was measured by 0.1% neutral red uptake. After the cells were cultured in RPMI-1640 medium supplemented with 10% fetal bovine serum for 48 h, 100 μL of neutral red solutions dissolved in 10 mmol L−1 of phosphate buffer solution (PBS) was added and incubated for 2 h. The supernatant was discarded and the cells in 96-well plates were washed with PBS twice to remove the neutral red that was not phagocytized by macrophages. Then cell lysis buffer (ethanol and 0.01% acetic acid at a ratio of 1
:
1 (100 μL per well)) was added to lyse the cells. After the cells were incubated overnight at room temperature, the absorbance at 540 nm was measured with a microplate reader (Thermo, USA).
2.12. Western blot analysis
To investigate the potential immunomodulatory mechanism, the expression of characteristic proteins in macrophages was measured by western blot. Macrophages were seeded onto 6-well culture plates at 1 × 105 cells per mL and incubated for 48 h. After incubation, the macrophages in each group was used for the preparation of protein extracts. Protein concentrations were quantified with the BCA protein assay kit using bovine serum albumin as a standard. All the primary antibodies were diluted with PBS 1000 times (Cell Signaling Technology, Danvers, MA, USA). In brief, cell lysates were subjected to SDS-PAGE and transferred to nitrocellulose membranes. The nitrocellulose membranes were then blocked in a TBST (Tris = 20 mM, pH 7.6, NaCl = 150 mM and Tween20 = 0.1%) containing 5% non-fat dry milk powder (BD. Co.) and incubated with the indicated antibodies overnight at room temperature. The membranes were subsequently washed with TBST and incubated for 1 h at room temperature with the appropriate secondary anti-bodies conjugated with horseradish peroxidase (diluted with PBS 100 times, Amersham Pharmacia Biotech, Piscataway, NJ, USA). The immune-reactive bands were detected using an enhanced chemiluminescence (ECL) kit (Millipore Co., Billerica, MA, USA). In SDS-PAGE, we used 5% concentrated gel and 12% separation gel for western blotting analysis. Each test was performed in six independent experiments.
2.13. Statistical analysis
The data are presented as means ± SD, and each experiment was repeated at least three times. Statistical analysis was performed using the SPSS software (version 18.0) to determine the significant differences. All values were analysed by one-way analysis of variance (ANOVA) followed by post hoc Tukey's test for multiple comparisons. The values of P < 0.05 were considered as statistically significant. The expression or phosphorylation of proteins was quantified by pixel intensity of Image J software to test the variability and statistical significance.
3. Results
3.1. Effects of BP-1 on thymus and spleen indexes in BALB/c mice
The effects of BP-1 on thymus and spleen indexes in BALB/c mice are shown in Table 2. The thymus and spleen indexes could reflect the animal immune function to some extent. When compared with the CTX group, the BP-1-treated groups at different concentrations exhibited a stronger effect on the thymus index (P < 0.01), especially in the 80 mg kg−1 BP-1 and 160 mg kg−1 BP-1 groups. In the 80 mg kg−1 BP-1 group, the thymus index was 9.87 ± 0.18 and reached the maximum (P < 0.01). Compared with the CTX group, the spleen indexes in the 80 mg kg−1 and 160 mg kg−1 BP-1 groups were significantly increased (P < 0.05). In the 80 mg kg−1 BP-1 group, the spleen index was 27.03 ± 0.94 and reached the maximum (P < 0.05). Meanwhile, BP-1 also increased the thymus and spleen indexes to some extent. These results suggested that BP-1 might affect immune organs in enhancing immunity.
Table 2 Effect of BP-1 on the immune organ index of immunosuppressive mice induced by CTX
Group |
BP-1 (mg kg−1 day−1) |
Initial weight (g) |
Final weight (g) |
Thymus index (mg 10 g−1) |
Spleen index (mg 10 g−1) |
Note: #P < 0.05, ##P < 0.01 vs. the normal group, *P < 0.05, **P < 0.01 vs. the CTX group, respectively. The data are expressed as means ± SD, n = 10. |
Normal |
— |
19.44 ± 0.63 |
21.42 ± 1.83 |
12.24 ± 0.13 |
28.18 ± 0.37 |
CTX |
— |
19.37 ± 0.33 |
18.47 ± 1.21# |
7.91 ± 0.14## |
22.84 ± 0.25## |
CTX + 40 mg kg−1 BP-1 |
40 |
19.45 ± 0.42 |
20.43 ± 0.93 |
8.54 ± 0.47* |
25.34 ± 0.66 |
CTX + 80 mg kg−1 BP-1 |
80 |
19.62 ± 0.85 |
21.22 ± 1.13* |
9.87 ± 0.18** |
27.03 ± 0.94* |
CTX + 160 mg kg−1 BP-1 |
160 |
19.24 ± 0.53 |
20.82 ± 0.74 |
9.16 ± 0.65** |
26.93 ± 0.76* |
3.2. Effects of BP-1 on the number of BMC and peripheral blood WBC and the serum levels of IL-2, TNF-α, and IFN-γ in BALB/c mice
As shown in Table 3, compared with the normal group, the number of BMC and peripheral blood WBC was significantly decreased in the CTX group. When compared with the CTX group, the number of BMC and peripheral blood WBC was significantly increased in the BP-1-treated groups, especially in the 80 mg kg−1 and 160 mg kg−1 BP-1 groups (P < 0.01). These results showed that BP-1 could significantly increase the number of BMC and peripheral blood WBC in the immunosuppressive mice model.
Table 3 Effects of BP-1 on peripheral blood and bone marrow of immunosuppressive mice induced by CTX
Group |
BMC/107 L−1 |
WBC/109 L−1 |
IL-2/ng L−1 |
TNF-α/ng L−1 |
IFN-γ/ng L−1 |
Note: #P < 0.05, ##P < 0.01 vs. the normal group, *P < 0.05, **P < 0.01 vs. the CTX group, respectively. The data are expressed as means ± SD, n = 10. |
Normal |
8.24 ± 0.13 |
5.74 ± 1.13 |
73.65 ± 11.15 |
253.58 ± 23.57 |
18.43 ± 0.53 |
CTX |
5.76 ± 0.93## |
1.75 ± 0.33## |
42.63 ± 9.36## |
132.79 ± 18.38## |
13.91 ± 0.74## |
CTX + 40 mg kg−1 BP-1 |
6.58 ± 0.63* |
2.98 ± 0.67* |
54.66 ± 10.65* |
194.66 ± 21.98* |
15.44 ± 0.97* |
CTX + 80 mg kg−1 BP-1 |
7.82 ± 0.85** |
4.32 ± 1.05** |
64.62 ± 8.68** |
234.74 ± 20.69** |
16.97 ± 0.88** |
CTX + 160 mg kg−1 BP-1 |
7.53 ± 0.69** |
3.94 ± 0.93** |
61.35 ± 9.45** |
214.98 ± 18.45** |
16.56 ± 0.64** |
The potential for BP-1 to regulate the serum levels of IL-2, TNF-α and IFN-γ in immunosuppressive mice was examined by ELISA. As shown in Table 3, the addition of BP-1 resulted in an increase in the serum levels of IL-2, TNF-α and IFN-γ in immunosuppressive mice. In the meantime, the protein expression levels of IL-2, TNF-α and IFN-γ were significantly increased (P < 0.01) in the 80 mg kg−1 and 160 mg kg−1 BP-1 groups. These results further confirmed that BP-1 could increase the serum levels of IL-2, TNF-α and IFN-γ, so as to improve the immune function of immunosuppressive mice.
3.3. Effects of BP-1 on the spleen in BALB/c mice
3.3.1. Effects of BP-1 on splenocyte proliferation and NK cell activity in BALB/c mice.
As shown in Fig. 1A, the CTX group was found to significantly decrease (P < 0.01) the proliferation of splenocytes, compared with the normal group. Combining with concanavalin (Con A) or a lipopolysaccharide (LPS), BP-1 enhanced the proliferation of the splenocyte immunosuppressive mice model. The 80 mg kg−1 and 160 mg kg−1 BP-1 groups showed a significant promotion in the proliferation of splenocytes with Con A and LPS (P < 0.01). BP-1 did not act as an independent mitogen similarly to T cell response mitogen (Con A) and B cell response mitogen (LPS). The result showed that BP-1 could improve the immune-competence of splenocytes in the immunosuppressive mice model.
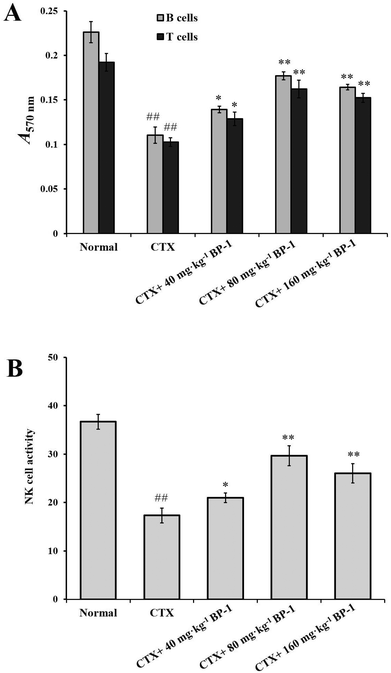 |
| Fig. 1 (A) Effects of BP-1 on the proliferation of splenocytes in BALB/c mice. (B) Effect of BP-1 on splenic NK cytotoxic activity in BALB/c mice. #P < 0.05, ##P < 0.01 vs. the normal group, *P < 0.05, **P < 0.01 vs. the CTX group, respectively. The data are expressed as means ± SD, n = 10. | |
In addition, NK cells play an important role in immunomodulatory activity in vivo. As shown in Fig. 1B, compared with the CTX group, the 80 mg kg−1 and 160 mg kg−1 BP-1 groups showed a significant promotion in the proliferation of NK cells (P < 0.01). In the 80 mg kg−1 group, the NK cell activity was 29.66 ± 2.36% and reached the maximum (P < 0.01). The result revealed that BP-1 could enhance the immune-competence of NK cells in the immunosuppressive mice model.
3.3.2. Effects of BP-1 on secretion levels of cytokines and Igs of splenocytes in BALB/c mice.
To address whether BP-1 elicits innate immune responses in splenocytes, ELISA was performed to examine the secretion levels of cytokines and Igs, respectively. As shown in Fig. 2, BP-1 shows the effects on the secretion levels of cytokines and Igs in splenocytes. The secretion levels of IL-2, TNF-α, IFN-γ, IgG and IgM in the CTX treated group were lower than those in the normal group. The groups treated with BP-1 showed significant elevation of the secretion levels of these cytokines and Igs. In the BP-1 treatment groups, the 80 mg kg−1 and 160 mg kg−1 BP-1 groups showed significant promotion the secretion levels of IL-2, TNF-α, IFN-γ, IgG and IgM. These results suggested that BP-1 could reverse the down-regulation of IL-2, TNF-α, IFN-γ, IgG and IgM expressions induced by CTX, but the dose-dependence was not linear.
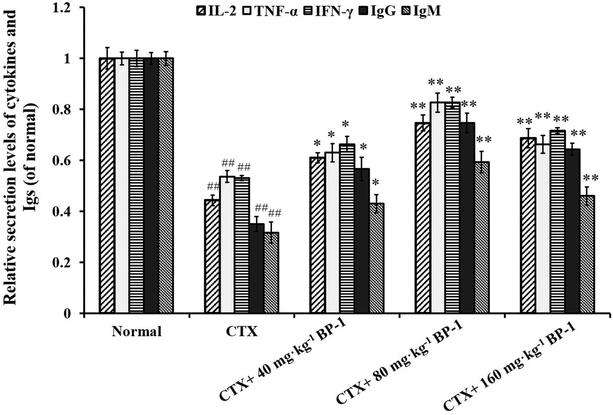 |
| Fig. 2 ELISA analysis of the secretion levels of IL-2, TNF-α, IFN-γ, IgG and IgM in the spleen. #P < 0.05, ##P < 0.01 vs. the normal group, *P < 0.05, **P < 0.01 vs. the CTX group, respectively. The data are expressed as means ± SD, n = 10. | |
3.3.3. Effects of BP-1 on the spleen in histological examinations of BALB/c mice.
In the present study, the HE (hematoxylin and eosin) stained histopathological images are shown in Fig. 3. In the normal group, the spleen cells were tight and arranged in very good order with a clear nucleus. In the CTX group, the spleen cells were sparse and arranged in disorder with the nucleus pyknotic. All the spleen cells differed in size and shape. Furthermore, there were necrotic areas with no cell structures in the HE stained histopathological images. While in the 80 mg kg−1 and 160 mg kg−1 BP-1 groups, the spleen cells were tight and arranged in good order with a clear nucleolus and less interstitial space, which was the closest with the normal group. The results indicated that BP-1 could repair the damage by CTX in spleen cells.
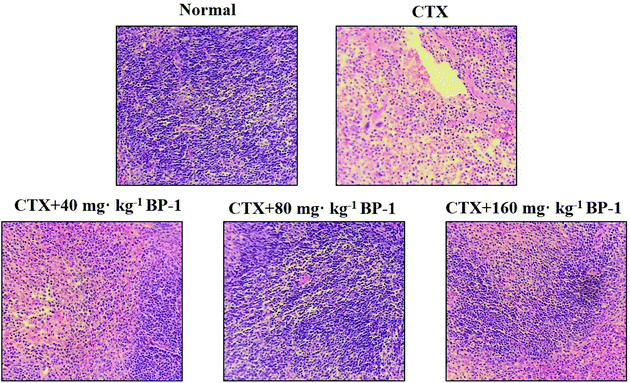 |
| Fig. 3 Effects of BP-1 on the spleen tissues in the HE stained histopathological images (scale bar = 100 μm, objective: 20×). | |
3.4. Effects of BP-1 on peritoneal macrophages in BALB/c mice
3.4.1. Effects of BP-1 on proliferation and phagocytic activity of macrophages in BALB/c mice.
Macrophages are monocyte-derived phagocytic cells that play crucial roles in adaptive and innate immunities. To investigate the immunomodulatory effect of BP-1 in vivo, the MTT assay was used to evaluate macrophage proliferation. As shown in Fig. 4, the cell proliferation activity was significantly increased in the 80 mg kg−1 and 160 mg kg−1 BP-1 groups (P < 0.01, P < 0.05), relative to the CTX group. The results suggested that BP-1 could promote the proliferation of macrophages in the immunosuppressive mice model. As shown in Fig. 4, the cell phagocytic activity was significantly increased by the 80 mg kg−1 BP-1 and 160 mg kg−1 BP-1 groups (P < 0.01, P < 0.05), relative to the CTX group. These results revealed that BP-1 overcame the immunosuppressive action induced by CTX in vivo.
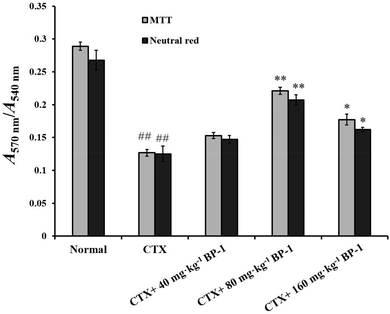 |
| Fig. 4 Effects of BP-1 on the proliferation and phagocytic activity of macrophages in BALB/c mouse. #P < 0.05, ##P < 0.01 vs. the normal group, *P < 0.05, **P < 0.01 vs. the CTX group, respectively. The data are expressed as means ± SD, n = 10. | |
3.4.2. Effects of BP-1 on protein expressions of macrophages in BALB/c mice.
As shown in Fig. 5, a marked increase in the TLR-4 protein level was detected in the macrophages of immunosuppressive mice after treatment with 80 mg kg−1 and 160 mg kg−1 BP-1, relative to the CTX group. This indicates that BP-1 could activate the receptor protein TLR-4 of the macrophages in immunosuppressive mice. In the meantime, the results showed that the protein expressions of TRAF6 and TAK1 were up-regulated by 80 mg kg−1 and 160 mg kg−1 BP-1 treatment, compared with the CTX group. In addition, the results also showed that the protein expression of the nucleus NF-κB p65 was increased by 80 mg kg−1 and 160 mg kg−1 BP-1 treatment, compared with the CTX group. These results suggested that BP-1 could up-regulate the protein expressions of TRAF6 and TAK1 and promote the translocation of NF-κB p65 into the nucleus in peritoneal macrophages.
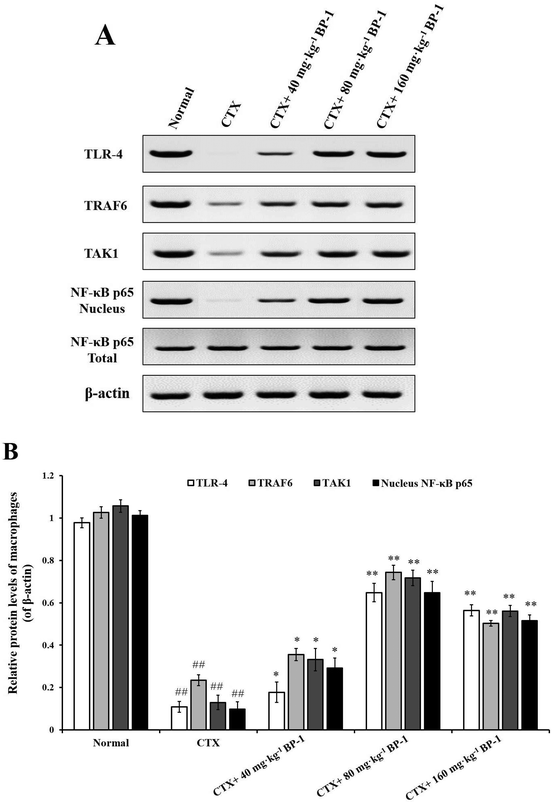 |
| Fig. 5 Measurement of TLR-4, TRAF6, TAK1 and NF-κB p65 in macrophages by western blot. β-Actin was used as an equal loading control. #P < 0.05, ##P < 0.01 vs. the normal group, *P < 0.05, **P < 0.01 vs. the CTX group, respectively. The data are expressed as means ± SD, n = 10. | |
4. Discussion
Barley (Hordeum vulgare L.), a member of the Poaceae family, is one of the first and traditionally domesticated crops among wheat and pea dating from about 10
000 years ago in the Fertile Crescent of the Middle East.18 Highland barley is a cultivar of hulless barley and used as a staple food in the Qinghai–Tibet Plateau of China, and generally used as animal fodder and ingredients of various foods such as baked products and beverages.19 It has been suggested that the health benefits of plant-based foods are attributed to the additive and synergistic effects of bioactive phytochemicals.20 The polysaccharides extracted from plants and fungi have recently drawn more attention of researchers and consumers, due to their antitumor activities and relatively low toxicity. Therefore, the discovery and evaluation of polysaccharides with antitumor and immuno-stimulating properties have emerged as one of the important research fields in chemistry and biology. A water-soluble polysaccharide (BP-1) was extracted and purified from highland barley (Hordeum vulgare L.) and its average molecular weight was about 6.7 × 104 Da.17 Cytoxan is one of the most commonly used chemotherapy drugs, which can lead to immunosuppression in mice.21
In this study, the immunomodulatory effects of BP-1 in BABL/c mice with CTX-induced immunosuppression were successfully evaluated. The immunosuppression induced by the high dose of CTX was confirmed by declines in the body weight and the thymus and spleen indexes,22,23 which were all strongly relieved by 2 weeks of BP-1 administration.
Bone marrow is a hematopoietic organ and the central immune organ of humans and mammals, and bone marrow suppression is one of the most obvious side effects of chemotherapy drugs.24 Peripheral white blood cells are an important part of an organism to resist external microorganisms, whose lifetime is short and need bone marrow stem cells to differentiate continuously complement.25 In this study, it was observed that the number of BMC and peripheral blood WBC was decreased by CTX in the immunosuppressive mice model and BP-1 (80 mg kg−1 and 160 mg kg−1) could significantly increase the number of BMC and peripheral blood WBC in the immunosuppressive mice model. These results indicated that BP-1 could antagonize the reduction of BMC and WBC caused by CTX and enhance the immune function of immunosuppressive mice. The serum levels of immune factors reflect the body's ability to respond to antigens.26
IL-2 shows therapeutic benefit in the alleviation of chronic hepatitis C virus-mediated vasculitis by increasing Treg cell counts and augmenting NK cells.27 TNF-α, which is produced by almost all immune system cells and many other cell types, plays a pivotal role in the host defense system and can induce the expression of a number of other immuno-regulatory and inflammatory mediators to eliminate the tumor cell.28 IFN-γ, released after viral infection, regulates the immune response in a sophisticated manner, including the regulation of the differentiation and growth of macrophages, NK cells, and T and B cells.29 Due to its antiviral effects, IFN-γ improves macrophage phagocytic action and modifies the functions of NK cells.30 Therefore, CTX inhibits the levels of IL-2, TNF-α and IFN-γ, which may result in profound depression of immunocyte-mediated action. Comparatively, BP-1 displays immune-supporting activities via up-regulation of IL-2, TNF-α and IFN-γ levels.
The spleen is one of the most important immune organs and is the source of lymphocytes.31 Splenocyte proliferation is a crucial event in the activation cascade of both cellular and humoral immune responses.32,33 T lymphocytes are mainly responsible for cellular immunity and B lymphocytes are the only cells capable of producing humoral immune responses.34–36 Splenic NK cells are large granular lymphocytes that are recognized by their distinctive capacity to lyse target cells without the need for prior exposure.37 In CTX-injected mice, BP-1 markedly increased the viability of NK cells and the proliferation of splenocytes. BP-1 did not act as an independent mitogen similarly to T cell response mitogen (Con A) and B cell response mitogen (LPS). The result showed that BP-1 could improve the immune-competence of splenocytes and the proliferation of NK cells in the immunosuppressive mice model.
Cytokines are important in immune responses and play a pivotal role in fighting against tumor growth.38,39 An outstanding feature of natural antibodies (IgG, IgM and so on) is their usual poly-reactivity, which provides animals with pre-existing broad antibody re-activities that allow them to rapidly recognize and protect against pathogens that have not been encountered previously. Protective roles for natural antibodies have been described in numerous viral, bacterial, fungal, and parasitic infections.40 To assess the secretion levels of cytokines and Igs associated with the immunity in immunosuppressive BABL/c mice treated with BP-1, the secretion levels of IL-2, TNF-α, IFN-γ, IgG and IgM in the spleen were measured. All the data indicated that BP-1 (80 mg kg−1 and 160 mg kg−1) could enhance the immune function of the spleen by promoting the secretion levels of IL-2, TNF-α, IFN-γ, IgG and IgM in CTX-induced immunocompromised mice. Among existing diagnostic technologies, the histopathological image of a biopsy or surgical specimen is the routine diagnostic method, and hematoxylin–eosin (HE) staining remains the gold criterion for diagnosis and grading.41 Now HE staining is the routine tissue staining method and HE images are easily available. These phenomena also lead to the extrinsic pathway of proliferation of spleen cells. In the present study, the HE stained histopathological images are shown in Fig. 3. The result of HE staining in the spleen showed that BP-1 (80 mg kg−1 and 160 mg kg−1) could repair the damage by CTX in spleen cells. This result is consistent with the determination of other indicators in the spleen.
Macrophages are key players in various host protective processes ranging from protection against pathogen infections to clearance of cellular debris and tissue repair and regeneration, with different macrophage subsets being associated with the propagation or resolution of inflammation.42–44 In this study, the results showed that BP-1 (80 mg kg−1 and 160 mg kg−1) could significantly enhance the proliferation and phagocytic activity in the macrophages of immunosuppressive mice. To investigate the mechanism of macrophages by BP-1 treatment, the protein expressions of TLR-4, TRAF6, TAK1 and NF-κB p65 in peritoneal macrophages were measured by western blot. TLR4 is a membrane protein receptor for the recognition of lipopolysaccharides (LPS) from Gram-negative bacteria, and participates in the body's immune response.14 TRAF6 is a member of the tumor necrosis factor receptor related factor (TRAF) family, which can mediate the TLR signaling pathway.45,46 Transforming growth factor to activate kinase 1 (TAK1) is a member of the MAP3K family, which can be activated by a variety of cytokines.47 Recent studies have shown that TAK1 mediates the activation of mitogen activated protein kinase (MAPK) and nuclear transcription factor (NF-κB) signaling pathways, which in turn play an important role in inflammatory reactions, apoptosis and immune responses.48,49 In CTX-injected mice, BP-1 (80 mg kg−1 and 160 mg kg−1) could up-regulate the protein expressions of TRAF6 and TAK1 and promote the translocation of NF-κB p65 into the nucleus in peritoneal macrophages, which indicates that BP-1 could activate macrophages by the TLR-4, TRAF6, TAK1 and NF-κB p65 pathways in vivo and taking an appropriate amount of BP-1 could promote the immune function of macrophages.
5. Conclusion
In conclusion, immune responses in immunosuppressive mice models treated with BP-1 were assessed in the present study. A speculated schematic diagram of BP-1 improving the immunomodulatory activity is depicted in Fig. 6. The immunomodulatory effects of BP-1 were associated with the weight index of spleen and thymus, peripheral blood, bone marrow, spleen and macrophages in immunosuppressive mice models. The results showed that BP-1 could antagonize the reduction of BMC and WBC caused by CTX and increase the serum levels of IL-2, TNF-α and IFN-γ in immunosuppressive mice. The results of the spleen showed that BP-1 could promote the proliferation activity of spleen cells and natural killer (NK) cells. A significant increment in the spleen cytokine and Ig level, and the repair of the damage of the spleen tissue by BP-1 treatment were found. BP-1 could activate macrophages by the TLR-4, TRAF6, TAK1 and NF-κB p65 pathways in vivo. These results suggested that BP-1 (80 mg kg−1) has a remarkable response of the host immune system in the immunosuppressive mice model. In the next study, the digestive pathways of BP-1 in the body will be tracked to explore the immune-stimulatory activity of BP-1 in vivo.
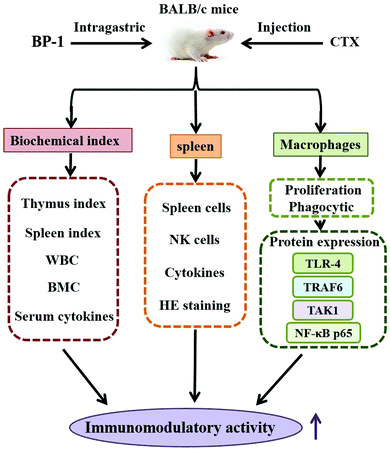 |
| Fig. 6 A tentative scheme indicating the pathways involved in the immunomodulatory effect of BP-1. Abbreviations: BP-1, a water-soluble polysaccharide obtained from highland barley; CTX: cytoxan; WBC: white blood cells; BMC, bone marrow cells; TLR-4, toll-like receptor 4; TRAF6, TNF receptor-associated factor 6; TAK1, TGF-activated kinase; and NF-κB p65, nuclear factor κB p65. | |
Abbreviations
BP-1 | A water-soluble polysaccharide obtained from highland barley |
CTX | Cytoxan |
TLR-4 | Toll-like receptor 4 |
TNF-α | Tumor necrosis alpha |
TRAF6 | TNF receptor-associated factor 6 |
TAK1 | TGF-activated kinase |
IL-2 | Interleukin 2 |
WBC | White blood cells |
BMC | Bone marrow cells |
NF-κB p65 | Transcription factor nuclear factor-kappa B |
IFN-γ | Interferon-γ |
IgG | Immunoglobulin G |
IgM | Immunoglobulin M |
Con A | Concanavalin |
LPS | Lipopolysaccharide |
NK cells | Natural killer cells |
HE | Hematoxylin–eosin |
qRT-PCR | Quantitative real-time polymerase chain reaction |
MTT | 3-(4,5-Dimethylthiazol-2-yl)-2,5-diphenyltetrazolium bromide |
PBS | Phosphate buffered saline |
DMSO | Dimethyl sulfoxide |
Author contributions
Chunling Wang and Lirong Han conceived and designed the experiments; Lirong Han performed the experiments; Meng Meng and Mingzhu Guo analyzed the data; Meng Meng and Dai Cheng contributed reagents/materials/analysis tools; Lirong Han wrote the paper; and Xu Wang and Lei Shi revised the paper. All authors read and approved the final manuscript.
Funding
This study was supported by National “13th Five-Year” Plan for Science & Technology (grant number 2016YFNC010104; 2016YFD0400505); the Innovative Research Team of Tianjin Municipal Education Commission (grant number TD13-5013); National “12th Five-Year” Plan for Science & Technology (grant number 2012BAD33B04); State Key Laboratory of Food Nutrition and Safety, Tianjin University of Science & Technology (grant number 17YDLJNC00130; 17PTSYJC00080; 18ZYPTJC00020); the Project program of Key Laboratory of Food Nutrition and Safety, Ministry of Education, China (No. 2018013); State Key Laboratory of Food Nutrition and Safety, Tianjin University of Science and Technology, Tianjin, 300457, P.R. China (grant number SKLFNS-KF-201811).
Conflicts of interest
The authors declare that they have no competing interest.
References
- F. Kebebew, Z. Gebeyaw, Y. Tsehaye, J. R. Stepp, F. S. Wyndham and R. K. Zarger, Int. J. Cancer, 2002, 97, 72–81 CrossRef PubMed.
- D. G. Caldwell, N. Mccallum, P. Shaw, G. J. Muehlbauer, D. F. Marshall and R. Waugh, Plant J., 2004, 40, 143–150 CrossRef CAS PubMed.
- Z. F. Pan, G. B. Deng, X. G. Zhai, F. Wu and M. Q. Yu, Genet. Resour. Crop Evol., 2007, 54, 1691–1699 CrossRef CAS.
- K. Chwialkowska, U. Nowakowska, A. Mroziewicz, I. Szarejko and M. Kwasniewski, J. Exp. Bot., 2016, 67, 1109–1121 CrossRef CAS PubMed.
- M. C. Shelden, D. A. Dias, N. S. Jayasinghe, A. Bacic and U. Roessner, J. Exp. Bot., 2016, 67, 3731–3745 CrossRef CAS PubMed.
- K. M. Ko and H. Y. Leung, Chin. Med., 2007, 2, 3 CrossRef PubMed.
- K. Matsunaga, T. W. Klein, H. Friedman and Y. Yamamoto, Clin. Vaccine Immunol., 2002, 9, 864–871 CrossRef CAS.
- R. Lopez-Posadas, I. Ballester, A. C. Abadia-Molina, M. D. Suarez, A. Zarzuelo, O. Martinez-Augustin and F. Sanchez de Medina, Biochem. Pharmacol., 2008, 76, 495–506 CrossRef CAS PubMed.
- S. Panthong, S. Ruangnoo, P. Thongdeeying, B. Sriwanthana and A. Itharat, BMC Complementary Altern. Med., 2014, 14, 1–7 CrossRef PubMed.
- C. Tümer, H. M. Bilgin, B. D. Obay, H. Diken, M. Atmaca and M. Kelle, Cell Biol. Int., 2007, 31, 565–569 CrossRef PubMed.
- J. Boubaker, F. Chaabane, A. Bedoui, R. Aloui, B. B. Ahmed, K. Ghedira and L. Chekir-Ghedira, Cancer Cell Int., 2015, 15, 82 CrossRef PubMed.
- J. L. Lai, Y. H. Liu, C. Liu, M. P. Qi, R. N. Liu, X. F. Zhu, Q. G. Zhou, Y. Y. Chen, A. Z. Guo and C. M. Hu, Inflammation, 2017, 40, 1–12 CrossRef CAS PubMed.
- A. M. Didierlaurent, S. Morel, L. Lockman, S. L. Giannini, M. Bisteau, H. Carlsen, A. Kielland, O. Vosters, N. Vanderheyde and F. Schiavetti, J. Immunol., 2009, 183, 6186 CrossRef CAS PubMed.
- G. Fan, X. Jiang, X. Wu, P. A. Fordjour, L. Miao, H. Zhang, Y. Zhu and X. Gao, Inflammation, 2016, 39, 1–10 CrossRef PubMed.
- C. D. Pietro, P. Zhang, T. K. O'Rourke, T. S. Murray, L. Wang, C. J. Britto, J. L. Koff, D. S. Krause, M. E. Egan and E. M. Bruscia, Sci. Rep., 2017, 7, 10882 CrossRef PubMed.
- Y. Li, X. Wang, J. Ren, X. Lan, J. Li, J. Yi, L. Liu, Y. Han, S. Zhang, D. Li and S. Lu, BMC Pharmacol. Toxicol., 2017, 18, 5 CrossRef PubMed.
- D. Cheng, X. Zhang, M. Meng, L. Han, C. Li, L. Hou, W. Qi and C. Wang, Int. J. Biol. Macromol., 2016, 92, 88–95 CrossRef CAS PubMed.
- J. Jacobsen, I. Venables, M. B. Wang, P. Matthews, M. Ayliffe and F. Gubler, Methods Mol. Biol., 2006, 343, 171–183 Search PubMed.
- F. Zhu, B. Du and B. Xu, J. Cereal Sci., 2015, 65, 43–47 CrossRef CAS.
- R. H. Liu, J. Cereal Sci., 2007, 46, 207–219 CrossRef CAS.
-
Y. U. Chuncheng, Y. Zhang, S. Zhai, H. Zheng and M. Zhu, Med. Plant, 2017, 8, 37–39 Search PubMed.
- S. Nafees, S. Rashid, N. Ali, S. K. Hasan and S. Sultana, Chem.-Biol. Interact., 2015, 231, 98–107 CrossRef CAS PubMed.
- S. Salva, G. Marranzino, J. Villena, G. Agüero and S. Alvarez, Int. Immunopharmacol., 2014, 22, 209–221 CrossRef CAS PubMed.
- W. Deng, Q. Han, W. Ge, S. You, C. Li, W. Zhang, H. Deng and C. Zhao, Chin. J. Immunol., 2004, 20, 431 Search PubMed.
- S. Arslan, E. Ozyurek and C. Gunduz-Demir, Cytometry, Part A, 2014, 85, 480–490 CrossRef PubMed.
- A. S. Jahromi, A. Zar, F. Ahmadi, P. Krustrup, K. Ebrahim, F. Hovanloo and D. Amani, Immune Network, 2014, 14, 255 CrossRef PubMed.
- R. S. Waters, J. S. Perry, S. Han, B. Bielekova and T. Gedeon, Math. Med. Biol., 2017, 35, 79–119 CrossRef PubMed.
- D. Qiao, X. He, C. Wei, L. Xia and L. Bao, J. Food Sci., 2016, 81, H1288–H1294 CrossRef CAS PubMed.
- H. Almughamsi and M. M. Whalen, Arch. Toxicol., 2016, 90, 1695–1707 CrossRef CAS PubMed.
- Z. A. Sayiner, A. Eraydın, T. Metin and M. Özkaya, BMJ Case Rep., 2017 DOI:10.1136/bcr-2017-221228.
- A. I. Gasisova, A. B. Atkenova, N. B. Ahmetzhanova, L. M. Murzabekova and A. C. Bekenova, Anat., Histol., Embryol., 2016, 46, 132–142 CrossRef PubMed.
- J. Han, J. W. Shui, X. Zhang, B. Zheng, S. Han and T. H. Tan, Mol. Cell. Biol., 2005, 25, 6869–6878 CrossRef CAS PubMed.
- F. H. Liao, W. Y. Hsiao, Y. C. Lin, Y. C. Chan and C. Y. Huang, Cell Cycle, 2016, 15, 1073–1083 CrossRef CAS PubMed.
- L. Fan, S. Ding, L. Ai and K. Deng, Carbohydr. Polym., 2012, 90, 870–874 CrossRef CAS PubMed.
- G. Méndez-Lagares, D. Lu, C. Chen, N. Terrault, M. R. Segal, M. Khalili, A. Monto, H. Shen, M. M. Manos and L. L. Lanier, J. Immunol., 2017, 200, ji1701364 Search PubMed.
- C. P. Rui, D. Martinelli, R. Cancedda, C. Gentili and A. Poggi, Front. Immunol., 2016, 7, 415 Search PubMed.
- A. B. H. Bakker, J. Wu, J. H. Phillips and L. L. Lanier, Hum. Immunol., 2000, 61, 18–27 CrossRef CAS PubMed.
- W. C. Zeng, Z. Zhang, H. Gao, L. R. Jia and W. Y. Chen, Carbohydr. Polym., 2012, 89, 694–700 CrossRef CAS PubMed.
- S. A. Saenz, B. C. Taylor and D. Artis, Immunol. Rev., 2008, 226, 172–190 CrossRef CAS PubMed.
- G. Mourglia-Ettlin, M. Cucher, P. Arbildi, M. Rosenzvit and S. Dematteis, Immunobiology, 2016, 221, 103–115 CrossRef CAS PubMed.
- A. Qu, J. Chen, L. Wang, J. Yuan, F. Yang, Q. Xiang, N. Maskey, G. Yang, J. Liu and Y. Li, Sci. China Inform. Sci., 2015, 58, 1–13 CrossRef.
- S. Gordon and A. Mantovani, Eur. J. Immunol., 2011, 41, 2470–2472 CrossRef CAS PubMed.
- E. L. Gautier, T. Shay, J. Miller, M. Greter, C. Jakubzick, S. Ivanov, J. Helft, A. Chow, K. G. Elpek, S. Gordonov, A. R. Mazloom, A. Ma'ayan, W. J. Chua, T. H. Hansen, S. J. Turley, M. Merad, G. J. Randolph and C. Immunological Genome, Nat. Immunol., 2012, 13, 1118–1128 CrossRef CAS PubMed.
- C. Z. Han, I. J. Juncadella, J. M. Kinchen, M. W. Buckley, A. L. Klibanov, K. Dryden, S. Onengut-Gumuscu, U. Erdbrügger, S. D. Turner and Y. M. Shim, Nature, 2016, 539, 570 CrossRef CAS PubMed.
- S. E. Keating, G. M. Maloney, E. M. Moran and A. G. Bowie, J. Biol. Chem., 2007, 282, 33435–33443 CrossRef CAS PubMed.
- K. Hoshino, O. Takeuchi, T. Kawai, H. Sanjo, T. Ogawa, Y. Takeda, K. Takeda and S. Akira, J. Immunol., 1999, 162, 3749–3752 CAS.
-
H. Matsui, Q. Zhang and K. Sasaki, Two MAP3Ks, TAK1 and ASK1 are activated via different molecular mechanism in response to mechanical stress, 2014 Search PubMed.
- M. Tomomura, R. Suzuki, Y. Shirataki, H. Sakagami, N. Tamura and A. Tomomura, PLoS One, 2015, 10, e0130174 CrossRef PubMed.
- F. T. Huang, J. F. Peng, W. J. Cheng, Y. Y. Zhuang, L. Y. Wang, C. Q. Li, J. Tang, W. Y. Chen, Y. H. Li and S. N. Zhang, Dig. Dis. Sci., 2017, 62, 1–14 CrossRef PubMed.
|
This journal is © The Royal Society of Chemistry 2019 |
Click here to see how this site uses Cookies. View our privacy policy here.