DOI:
10.1039/C8FO01979G
(Paper)
Food Funct., 2019,
10, 99-111
Anti-melanogenesis properties of condensed tannins from Vigna angularis seeds with potent antioxidant and DNA damage protection activities
Received
10th October 2018
, Accepted 28th November 2018
First published on 29th November 2018
Abstract
Condensed tannins contained in food are known to have many beneficial impacts on human health. In this study, we attempt to evaluate the structural features, antityrosinase effects, anti-melanogenesis properties, antioxidant activity and DNA damage protection activity of condensed tannins purified from the seeds of Vigna angularis (Willd.) Ohwi et Ohashi. MALDI-TOF MS, ESI-Full-MS, and HPLC-ESI-MS demonstrated that condensed tannins are composed of procyanidins, prodelphinidins and their gallates, among which procyanidins are the dominant components. As reversible and mixed-type inhibitors of tyrosinase, condensed tannins from V. angularis strongly inhibited the monophenolase and odiphenolase activities with IC50 values of 130.0 ± 0.5 and 35.1 ± 2.0 μg mL−1, respectively. What's more, condensed tannins had a good inhibitory effect on cell proliferation, cellular tyrosinase activity, and melanogenesis of B16 mouse melanoma cells. Based on fluorescence quenching analyses, these compounds were determined to be effective quenchers of the enzyme and its substrates. According to molecular docking, the strong interaction between condensed tannins and tyrosinase was mainly driven by hydrogen bonding and hydrophobic force. In addition, condensed tannins showed a powerful antioxidant capacity and DNA damage protection activity. Therefore, condensed tannins from V. angularis have feasible applications in food, medicine, and the cosmetics industry.
1. Introduction
Tyrosinase (EC 1.14.18.1) is a multifunctional oxidase which possesses monophenolase and diphenolase activities simultaneously.1 It is responsible for melanin hyperpigmentation in humans, molting in insects, and undesirable browning in fruits and vegetables.2–4 Hence, the inhibition of tyrosinase activity is considered as a feasible approach to the therapy of melanoma and skin pigmentation disorders, the control of agricultural pests, and the improvement of food quality. Although a large number of tyrosinase inhibitors have been screened, few of them are applied today as many of them possess side effects or low effectiveness.5 Therefore, the evaluation of safe and effective tyrosinase inhibitors has become attractive because of their possible applications in the areas of pharmaceuticals, food, agriculture, and cosmetics.6,7 Anti-tyrosinase compounds derived from plants are generally considered to be free of harmful side effects.8 Therefore, condensed tannins from the seeds of Vigna angularis (Willd.) Ohwi et Ohashi are used as the source of tyrosinase inhibitors.
Condensed tannins are a class of polyphenols widely distributed in cereals and legume seeds. Owing to their diverse biological activities, these polymers have aroused enough interest in the areas of food, health, and nutrition.9–11 It was reported that these compounds have many physiological effects by acting as antioxidant, antiviral, anticarcinogen, antibacterial, and neuroprotective agents.12–14 However, it is universally acknowledged that the bioactive capacity of plant condensed tannins largely depends upon their structure.15 Therefore, it is necessary to study their structure. The structure of condensed tannins varies widely depending on the diversity of the monomer units, interflavan linkage, degree of polymerization (DP), and substituent of the 3-hydroxyl group.16–18 The characterization of condensed tannins remains difficult because of the complexity and diversity of their structure. Therefore, MALDI-TOF MS, ESI-Full-MS, and HPLC-ESI-MS were employed to characterize the structure of condensed tannins.
V. angularis is a well-known food and a folk medicine and is widely distributed in East Asia.19 The seed coat of this plant is rich in pigments including proanthocyanidins and anthocyanins.20,21 The extracts of this plant have been reported to show many bioactivities such as antioxidant, anti-inflammatory, anticarcinogen, and hepatoprotective activities.19,20 However, there have been no reports of the anti-tyrosinase activity, anti-melanogenesis properties, antioxidant activity and DNA damage protection activity of polymeric condensed tannins from the seeds of V. angularis. In this study, in order to make full use of this plant, polymeric condensed tannins were extracted and purified, and their structure, antityrosinase activity, anti-melanogenesis properties, antioxidant activity and DNA damage protection activity were studied to provide scientific evidence for the development of natural tyrosinase inhibitors and antioxidants.
2. Materials and methods
2.1. Reagents, chemicals, and standards
Sephadex LH-20, amberlite IRP-64 cation-exchange resin, gallic acid, cesium chloride, 2,5-dihydroxybenzoic acid, benzyl mercaptan, trifluoroacetic acid, HPLC standards, and chemicals used for enzyme analysis (L-tyrosine, 3,4-dihydroxyphenylalanine, mushroom tyrosinase, arbutin) were purchased from Sigma-Aldrich (St Louis, MO, USA). 2,2-Azinobis(3-ethylbenzothiazoline-6-sulfonic acid) diammonium salt (ABTS), 2,2-diphenyl-1-picrylhydrazyl (DPPH), 2,4,6-tripyridyl-S-triazine (TPTZ), Trolox, ascorbic acid (Vc), and butylated hydroxyanisole (BHA) were also obtained from Sigma-Aldrich. Trypsin-EDTA, penicillin, streptomycin, and RPMI-1640 (for cell tests) were purchased from Gibco (Germany). Diethyl pyrocarbonate (DEPC), 3-(4,5-dimethylthiazol-2-yl)-2,5-diphenyltetrazolium bromide (MTT), and dimethyl sulfoxide (DMSO) were the products of Amresco (USA). pET30 a(+) (Novagen, Madison, WI) was used for analyzing the protection of condensed tannins against DNA damage. All analytical-grade solvents, including acetone, petroleum ether, ethyl acetate, methanol, ferrous sulfate (FeSO4), and hydrogen peroxide (H2O2), were purchased from Sinopharm (Shanghai, China).
2.2. Preparation of sample powder
The seeds of V. angularis were supplied by a local farm (Guangfeng County, Shangrao City, Jiangxi Province of China) in July 2016. They were selected according to the uniformity of shape, weight, and colour. The sample powders used for extraction were prepared by the procedure as follows: the seeds of V. angularis were immediately washed and frozen for 24 hours at −20 °C in a refrigerator, and then they were freeze-dried in a freeze-dryer (Boyikang, Beijing, China). The dry sample was further ground and passed through a 40-mesh sieve to obtain the final sample powders.
2.3. Total phenolics and extractable condensed tannins assay
The total polyphenol content of V. angularis seed powders was measured according to a previous study.15 The absorbance was read at 725 nm by using a Beckman UD-800 spectrophotometer (Beckman coulter, Pasadena, California, USA). Gallic acid was used as a standard and the result was expressed in mg gallic acid equivalents per g dry weight. For the assay of extractable condensed tannin content in the sample powders, the butanol–HCl method was performed.22 Purified tannins obtained from our purification process were used as the standard and the result was expressed as mg purified tannin equivalents per g dry weight. All tests were repeated three times.
2.4. Extraction and purification of condensed tannins from the seeds of V. angularis
The flow schemes of extraction and purification were carried out according to our previous study.23 In brief, the powder (10 g) of V. angularis seed was ultrasonically extracted thrice with 70% acetone (3 × 200 mL, 0.5% acetic acid was included). Petroleum ether (3 × 150 mL) and ethyl acetate (3 × 150 mL) were selected to eliminate non-polar fractions, lipophilic compounds, and low molecular phenolics. The remaining fraction was then chromatographed on a Sephadex LH-20 column (Pharmacia Biotech, Uppsala, Sweden), which was eluted with 60% methanol and 70% acetone orderly. For the latter, acetone was removed and lyophilized to dryness to obtain purified condensed tannins (335 mg).
2.5. Structure analysis
A Bruker Reflex III (Germany) equipped with a delayed extraction ion source and a pulsed nitrogen laser was used for the MALDI-TOF MS analysis of condensed tannins from the seeds of V. angularis. The parameters for the measurement were as follows: 20 kV accelerating voltage, 23.0 kV reflectron voltage, 16.32 kV delayed extraction voltage, and 3 ns for the length of one laser pulse. 2,5-Dihydroxybenzoic acid was selected as the matrix and cesium chloride was used as the cationization reagent. Sample solution (10 mg mL−1), cesium chloride solution (1.52 mg mL−1), and matrix solution (10 mg mL−1) were mixed at a volumetric ratio of 1
:
1
:
3 before they were measured in a mass analyzer.24
ESI-Full-MS was performed by using a Xevo G2 QTof MS (Waters). The ionization mode was negative electrospray (ESI−). The source temperature was set at 120 °C, and the dissolving temperature was set at 400 °C. The capillary voltage and sampling cone voltage were 2000 V and 100 V, respectively. The mass spectrum was collected with a scan range from 500 to 2000 m/z. MS data and spectra were managed by MassLynx 4.0 (Waters).
The thiolysis and HPLC-ESI-MS assays of condensed tannins were executed according to previous studies.25,26 For the reversed-phase HPLC-ESI-MS assay, the chromatogram was collected from an Agilent 1200 system (Agilent, Palo Alto, CA, USA) with a 3200 QTRAP system (Applied Biosystems, Foster, USA). H2O (containing 0.5% trifluoroacetic acid) and acetonitrile were used as mobile phases. The linear gradient elution system was executed as follows: 0 to 45 min, 12% to 80% acetonitrile; 45 to 50 min, 80% to 12% acetonitrile. The flow rate was set at 1 mL min−1, and the chromatogram was recorded at 280 nm. For the normal-phase HPLC-ESI-MS assay, we used two mixed solvents as mobile phases: A = water/acetic acid/methanol/dichloromethane (1
:
1
:
7
:
41, v/v/v/v) and B = water/acetic acid/methanol/dichloromethane (1
:
1
:
5
:
43, v/v/v/v). The linear gradient for the elution was: 0 to 20 min, 0–13.5% B; 20 to 50 min, 13.5–29.2% B; 50 to 55 min, 29.2–100% B; 55 to 60 min, 100% B. Conditions for HPLC and MS analyses were the same as those for reversed-phase HPLC-ESI-MS analysis described previously.27
2.6. Enzyme assay
We executed enzymatic assays in compliance with a previous report.27L-Tyrosine and 3,4-dihydroxyphenylalanine (L-DOPA) were selected as the substrates of monophenolase and diphenolase activity assays, respectively. The reaction medium contained a substrate (2 mM L-tyrosine or 0.5 mM L-DOPA), sodium phosphate buffer (50 mM, pH 6.8), H2O, enzyme (0.2 mg mL−1), and an inhibitor with different concentrations. The reaction mixture was mixed and the final volume was 3 mL. The initial slope of the kinetic curve was recorded as an indicator of tyrosinase activity. Absorption at 475 nm was read on a Beckman UD-800 spectrophotometer. The inhibitory effects were evaluated with IC50 values. Inhibitory mechanism assay was carried out by changing the enzyme concentration in 3 mL of reaction medium. The inhibition type was executed according to the Lineweaver–Burk plot.
2.7. Cell culture and measurement of cell viability
In the current study, RPMI 1640 medium was selected to culture mouse melanoma cells (B16). Heat-inactivated fetal bovine serum (10%), penicillin (100 units per mL), and streptomycin (100 μg mL−1) were added to the medium to ensure the growth of the B16 cells. The cells were cultured under 5% CO2 at 37 °C.17 For measuring the activity of B16 mouse melanoma cells, the MTT assay was carried out.28
2.8. Detection of intracellular tyrosinase activity and melanin content in the B16 mouse melanoma cells
The effects of condensed tannins from V. angularis seeds on the intracellular tyrosinase activity and melanin content of B16 mouse melanoma cells were detected in line with previous reports.14,29 The cells were plated in 6-well plates at a density of 150 cells per mL. For the intracellular tyrosinase activity assay, the B16 cells were co-cultured with condensed tannins for 48 h first. Then, the cells were lysed by using lysis buffer. The cellular lysate was centrifuged at 10
000g at 4 °C for 20 min to obtain a supernatant. The protein contents of the supernatant were determined by using the BCA protein assay kit (Pierce, Rockford, IL). The supernatant (0.1 mL), L-DOPA (0.9 mL, 0.5 mM), and phosphate buffer (pH 6.8) were mixed well to measure tyrosinase activity in the absence and presence of condensed tannins from V. angularis seeds. The results were read on a Beckman DU-800 at 475 nm. For the melanin content assay, B16 cells were also co-cultured with the condensed tannins for 48 h. The cells were collected and centrifuged at 10
000g for 15 min. The protein contents were determined by using the BCA protein assay kit and the rest of the cells were lysed by using 0.5 mL of NaOH (5 M) at 100 °C for 1 h. Next, a part of crude cell extracts (0.2 mL) was moved into a 96-well plate. The melanin content was determined at 405 nm.
2.9. Interactions of condensed tannins and substrates (L-tyrosine and L-DOPA)/tyrosinase with fluorescence quenching
Fluorescence quenching of condensed tannins on substrates (L-tyrosine and L-DOPA) and tyrosinase was carried out by using a Varian Cary Eclipse fluorescence spectrophotometer. The reaction system (1 mL) was made up of mushroom tyrosinase solution (100 μL, 0.2 mg mL−1), sample solution (100 μL) with different concentrations, and sodium phosphate buffer (800 μL, 50 mM, pH 6.8). The excitation wavelength was set at 290 nm.24
In the present study, the fluorescence quenching constant of tyrosinase by condensed tannins from V. angularis seeds was calculated by using the Stern–Volmer equation.30
| F0/F = 1 + Kqτ0[Q] = 1 + KSV[Q] | (1) |
In the equation, F0 and F denote the fluorescence intensities in the absence and presence of the quencher, respectively. Kq means the bimolecular quenching constant of the enzyme and τ0 (10−8 s) is the lifetime of the fluorophore without the quencher. [Q] represents the concentration of the quencher, and KSV is the Stern–Volmer quenching constant.
Static and dynamic mechanisms are involved in fluorescence quenching. For the static mechanism, the values of KA (apparent binding constant) and n (the binding affinity) could be evaluated using the following equation.
| log[(F0 − F)/F] = log KA + n log[Q] | (2) |
2.10. Molecular docking
The X-ray structure of mushroom tyrosinase (2Y9W, RCSB Protein Data Bank) was used as the initial protein model for molecular docking. The water molecules of the enzyme were removed, whereas Gasteiger charges and polar hydrogen atoms were then added to the enzyme molecule by using AutoDock Tools. The 3D structures of epicatechin and catechin were obtained from ChemBioDraw Ultra 12.0. All the parameters were adopted according to the default settings. The optimal binding mode was obtained from the docked conformation with the lowest free energy.2
2.11. Determination of antioxidant activity
In this study, three antioxidant methods were used for determining the oxidation resistance of condensed tannins from V. angularis seeds.14 The reaction system of the DPPH radical scavenging activity assay included 0.1 mL of sample solution with different concentrations and 3 mL of DPPH solution (dissolved in methanol). The results were recorded at 517 nm by using a Beckman UD-800 spectrophotometer. For ABTS radical scavenging activity assay, ABTS solution (7 mM) and potassium persulfate solution (2.45 mM) were mixed and stored in the dark for 16 h to prepare ABTS+ stock solution. The stock solution was diluted with 80% ethanol to an absorbance of 0.700 ± 0.050 at 734 nm to acquire ABTS+ working solution before the moment of analysis. The reaction mixture contained 0.1 mL of sample solution and 3.9 mL of the ABTS+ working solution. The absorbance of the mixture was measured at 734 nm after incubation for 6 min. For FRAP assay, FRAP reagent (3 mL) was mixed well with the sample solution (0.1 mL). Then, the mixture was measured at 593 nm after incubation for 10 min. The FRAP reagent was generated from a mixture of 10 mM TPTZ, 20 mM ferric chloride, and 300 mM sodium acetate buffer with pH = 3.6 (1
:
1
:
10).
2.12. Protective effect of condensed tannins against DNA damage induced by hydrogen dioxide
Plasmid DNA pET30 a(+) was oxidised by hydroxyl radicals in the absence and presence of condensed tannins from V. angularis seeds and monitored by 0.8% agarose gel electrophoresis with a voltage of 120 V. In brief, the reaction system contained 1 μL of pET30 a(+) plasmid DNA, 10 μL of phosphate buffered potassium (pH 6.8), and 2 μL of sample solution with different concentrations (0, 5, 50, and 100 μg mL−1), 6 μL of FeSO4 (10 mM), and 6 μL of 15% H2O2. The total volume of the reaction was 25 μL. Gels were stained with ethidium bromide and photographed using a gel documentation system (Bio-Rad Laboratories, Inc., USA).
2.13. Statistical analysis
All the assays were repeated three times. The curves presented in the picture were plotted according to the average value. Statistical analyses of data were performed using SPSS 19.0 (SPSS, Chicago, IL, USA). One-way analysis of variance and post-hoc test were used, and the differences were significant when P < 0.05.
3. Results and discussion
3.1. Yield, purity, total phenolics and extractable condensed tannin content
In the current study, polymeric condensed tannins were purified from the seeds of V. angularis by solvent extraction and Sephadex LH-20 column chromatography. We finally obtained 335 mg purified condensed tannins from 10 g of dry seed powders, and the yield was evaluated to be 3.35% (Table 1). Polyphenol compounds have attracted more and more attention in recent years because of their various biological functions (e.g. antioxidant and anticancer).12,13 A high level of total phenolics (131.5 ± 1.7 mg gallic acid equivalents per g dry weight) and extractable condensed tannins (70.5 ± 0.1 mg purified tannin equivalents per g dry weight) (Table 1) in the seeds of V. angularis revealed that this material could be selected as a source of these valuable constituents. In addition, the UV/Vis spectrum proved the existence of purified condensed tannins in V. angularis seeds, since they showed a catechin-like and symmetrical peak near 280 nm. In addition, epicatechin was selected as a standard, and the purity was estimated to be 98.01%.
Table 1 Yield, the content of total phenolics and extractable condensed tannins
Sample |
Yield |
Total phenolics (g per kg dry weight) |
Extractable condensed tannins (g per kg dry weight) |
Condensed tannins |
3.35% |
131.5 ± 1.7 |
70.5 ± 0.1 |
3.2. Structural analysis
3.2.1. MALDI-TOF MS analysis.
The MALDI-TOF MS spectrum of condensed tannins from V. angularis seeds is shown in Fig. 1. The mass spectrum indicated clearly that condensed tannins exhibited a series of primary peaks which increased equably at a mass distance of 288 Da. It could be concluded that 288 Da derived from the addition of one (epi)catechin. For each cluster of peaks described above, the subsequent peak with 16 Da more was also detected when they were magnified (enlarged 3-mers in Fig. 1A). They were attributed to the presence of (epi)gallocatechin units. Additionally, A-type condensed tannins were also detected in the MS spectrum after magnifying tannin polymers (Fig. 1B).
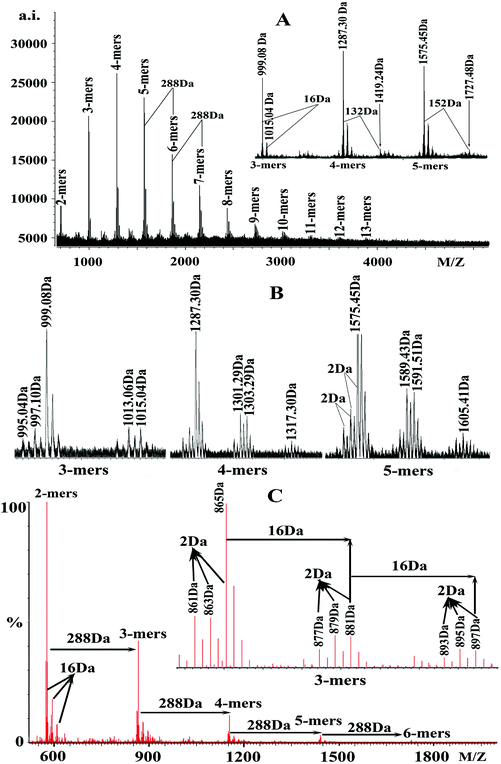 |
| Fig. 1 (A) MALDI-TOF positive-ion (Cs+) reflectron mode mass spectra of condensed tannins from the seeds of V. angulari. (B) The enlarged MALDI-TOF spectra of 3-mers, 4-mers, and 5-mers. (C) ESI-Full-MS analysis of condensed tannins from V. angulari in the negative mode. | |
These findings suggested that condensed tannins extracted from V. angularis seeds were composed of procyanidins and prodelphinidins, among which procyanidins were the main components. These compounds had structural heterogeneity due to the diversity of monomer units and interflavan linkage (A-type and B-type). In addition, the series of peaks with more 152 Da following the major set of peaks were also observed (enlarged 5-mers in Fig. 1A), which were in line with the addition of one galloyl group at the heterocyclic C-ring.31 Also, the series of peaks with more 132 Da following the major set of peaks were quasimolecular ions [M + 2Cs+ − H]+ that produced by simultaneous attachment of two Cs+ and the absence of a proton (see enlarged 4-mers in Fig. 1A).32 Furthermore, the degree of polymerization of condensed tannins extracted from V. angularis seeds ranged from 2-mers to 13-mers, with the highest intensity in 4-mers (Fig. 1A). In summary, polymeric condensed tannins from V. angularis seeds were complex mixtures of procyanidins, prodelphinidins, and their gallates with a DP up to 13-mers. Kawakami et al.21 employed the MALDI-TOF MS technique to detect proanthocyanidins from adzuki seed coat, recorded as Na+ adducts in the positive ion reflectron mode. Their results showed that the proanthocyanidins contained procyanidins (consisting of catechins/epicatechins) which ranged from 3-mers to 5-mers. Compared with the findings of Kawakami et al., we used Cs+ ionizing condensed tannins and our study provided a relatively comprehensive structure outline (including monomer units, interflavan linkage, DP, and substituent) of condensed tannins extracted from V. angularis seeds.
3.2.2. ESI-Full-MS analysis.
To better understand the structural information of condensed tannins from V. angularis seeds, ESI-Full-MS (negative mode) analysis was carried out. As shown in Fig. 1C, the result showed that the degree of polymerization of condensed tannins varied from 2-mers to 6-mers. It was clear that the mass spectra had a series of peaks which increased equably with a mass distance of 288 Da, which was in accordance with the addition of one (epi)catechin. It could be further concluded that the main constituent of V. angularis seed condensed tannins was procyanidins. For each tannin polymers, masses of 16 Da more following the major set of peaks were also detected in spectra when they were magnified (Fig. 1C). These masses were heteropolymers of repeating constitution units, which indicated the presence of (epi)gallocatechin. Therefore, it could be further concluded that V. angularis seed condensed tannins contained prodelphinidins. Furthermore, A-type (861, 863, 877, 879, 893, and 895 Da) condensed tannins were clearly found when polymers were enlarged (the enlarged mass spectrum of 3-mers in Fig. 1C). On the basis of the ESI-Full-MS results, it was found that this technique was sensitive to the detection of A-type condensed tannins. These results agreed well with the MALDI-TOF MS analysis.
3.2.3. HPLC-ESI-MS analysis of condensed tannins.
Normal-phase HPLC-ESI-MS analysis was executed to understand the structural profile of condensed tannins from V. angularis seeds (Fig. 2A). The result confirmed the presence of tannin polymers which ranged from monomers to 11-mers. From the chromatogram of normal-phase HPLC, it was found that monomers to 6-mers were precisely isolated. However, tannin polymers whose DP > 11 formed a single broad peak in the end of the chromatogram. Moreover, A-type condensed tannins were also detected, and peaks 2a (575 Da [M − H]−), 3a (863 Da, [M − H]−), 4a (1151 Da, [M − H]−), and 5a (1439 Da, [M − H]−) were 2-mers, 3-mers, 4-mers, and 5-mers with A-type linkage, respectively. Our results were similar to the findings of Gu et al.26 and a good supplement of MS.
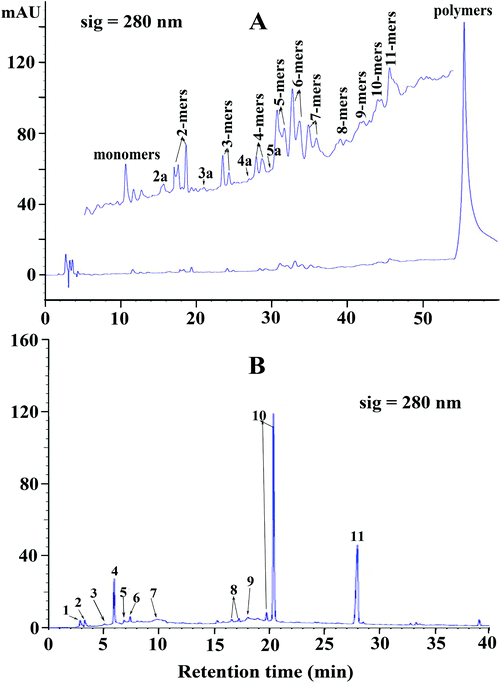 |
| Fig. 2 (A) Normal phase HPLC chromatogram of condensed tannins from V. angulari. (B) Reversed-phase HPLC chromatogram of condensed tannins from V. angulari after thiolytic degradation. Peaks 1, 2, 3, 4, 5, 6, 7, 8, 9, 10, and 11 were gallocatechin, epigallocatechin, catechin, epicatechin, catechin gallate, epicatechin gallate, A-type dimer, epi/gallocatechin benzylthioether, A-type trimer benzylthioether, epi/catechin benzylthioether, and benzyl mercaptan, respectively. | |
Reversed phase HPLC-ESI-MS coupled with thiolysis analysis was utilized to obtain more detailed information on the chemical composition of condensed tannins from V. angularis seeds. The chromatogram of thiolytic degraded condensed tannins is shown in Fig. 2B. The result indicated that the terminal unit was mainly epicatechin (peak 4) and the extension units were primarily (epi)catechin benzylthioethers (peaks 10). It was further revealed that procyanidins were the main constituents of V. angularis condensed tannins. Moreover, other terminal units, including gallocatechin (peak 1), epigallocatechin (peak 2), catechin (peak 3), catechin gallate (peak 5), and epicatechin gallate (peak 6), were detected. Other extension units were (epi)gallocatechin benzylthioethers (peaks 8). A-type dimer (peak 7) and A-type trimer benzylthioether (peak 9) were also found in the condensed tannins of V. angularis seeds. The above results illustrated the presence of prodelphinidins and A-type condensed tannins. In addition, peak 11 was benzyl mercaptan which was used for thiolysis. These findings were good supplements of MS and normal-phase HPLC-ESI-MS analysis. In summary, the structure of condensed tannins from V. angularis seeds was complex because of the diversity of the monomer units, interflavan linkage, DP, and the substituent of the 3-hydroxyl group.
3.3. Effect of condensed tannins from V. angularis seeds on the monophenolase activity of tyrosinase
Kinetic courses of L-tyrosine oxidation by mushroom tyrosinase were tested in the presence of condensed tannins with different concentrations (Fig. 3A). When L-tyrosine was used as the substrate, the monophenolase activity of tyrosinase was assayed in the absence of an inhibitor, and it was found that there was a remarkable lag time. The system reached a steady-state rate after the lag period (lag time).33 The steady-state rate of monophenolase activity can be negatively affected by the addition of condensed tannins (Fig. 3B-I). The steady-state rate decreased in a dose-dependent way following the increase of the inhibitor concentrations. When the final concentration of the condensed tannins in the system reached 166.67 μg mL−1, the steady-state rate decreased from 100% to 44.6%. The IC50 value was estimated to be 130 ± 0.5 μg mL−1 for monophenolase activity (Table 2).
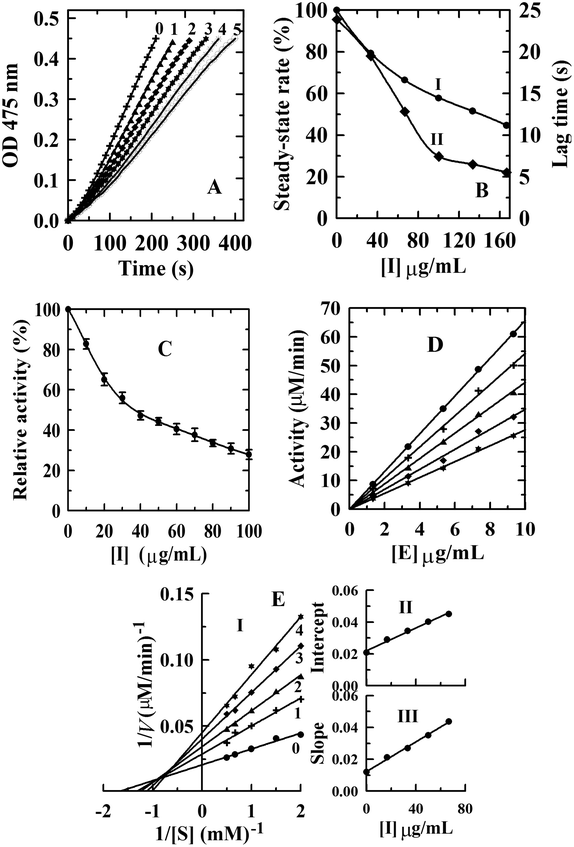 |
| Fig. 3 (A) Inhibition effect of condensed tannins from V. angulari on the monophenolase activity of mushroom tyosinase: progress curves for the oxidation of L-tyrosine by the enzyme. (B) The effect of inhibitor on the steady-state rate (I, filled circle) and on the lag time (II, solid square) for the oxidation of tyrosine. The concentrations of condensed tannins for curves 0–5 were 0, 33.33, 66.67, 100.00, 133.33, and 166.67 μg mL−1, respectively. Inhibition effect (C), inhibitory mechanism (D), and inhibitory type (E-I) and inhibitory constant (E-II, III) of condensed tannins from V. angulari on the diphenolase activity of mushroom tyrosinase. Concentrations of condensed tannins for curves 0–4 were 0, 25, 50, 75, and 100 μg mL−1, respectively. | |
Table 2 Effect of condensed tannins from V. angularis on the monophenolase and diphenolase activities of tyrosinase
Sample |
Monophenolase |
Diphenolase |
IC50 (μg mL−1) |
IC50 (μg mL−1) |
Inhibition |
Inhibition type |
Inhibition constants (μg mL−1) |
Condensed tannins |
130.0 ± 0.5 |
35.1 ± 2.0 |
Reversible |
Mixed |
K
IS = 61.2 ± 2.5 |
K
I = 26.4 ± 1.3 |
Compared with condensed tannins from the pericarp of Clausena lansium17 (IC50 = 23.6 ± 0.3 μg mL−1), the fruit of avocado24 (IC50 = 40 ± 1.2 μg mL−1), and the fruit stone of hawthorn25 (IC50 = 37 ± 0.5 μg mL−1), the inhibition activity of condensed tannins from V. angularis seeds towards monophenolase was weaker. This might arise from the structural characteristics of the condensed tannins illustrated in the MALDI-TOF MS analysis. Our previous studies showed that high polymer content had stronger inhibition on the tyrosinase than the oligomer.27 It should be emphasized that the inhibition activity of V. angularis seed condensed tannins towards the mushroom tyrosinase was still very strong. Structural similarity between condensed tannins and the substrate of tyrosinase provided the foundation for the strong inhibition.24 In addition, the corresponding lag time decreased from 23.9 s of the initial state to 5.1 s in the presence of 160 μg mL−1 condensed tannins (Fig. 3B-II). In summary, condensed tannins inhibited the monophenolase activity of tyrosinase by reducing its steady-state rate and extending the lag time.
3.4. Inhibitory effect, mechanism, and type of condensed tannins from V. angularis seeds on the diphenolase activity of tyrosinase
The concentration effect of the condensed tannins from V. angularis seeds on the diphenolase activity of tyrosinase was assayed. The result indicated that the diphenolase activity decreased with increasing concentrations of the condensed tannins (Fig. 3C). The IC50 value was determined to be 35.1 ± 2.0 μg mL−1 (Table 2). Compared with arbutin (IC50 = 1442.9 ± 2.8 μg mL−1),24 condensed tannins were much better diphenolase inhibitors. Similarly, it could be speculated that the effective inhibition of these compounds on the diphenolase activity derived from the structural similarity between condensed tannins and the substrate of tyrosinase. Because of the homogeneity (polyphenol compounds and multiple hydroxyl groups), the inhibition mechanism of the condensed tannins on the diphenolase activity of tyrosinase was studied. Fig. 3D shows that the enzyme activity against the concentration of enzyme gave a group of straight lines, all of which passed through the origin, indicating the reversible inhibition of the condensed tannins on tyrosinase (Table 2). Finally, the plot of 1/v against 1/[S] (the Lineweaver–Burk plot) was used to evaluate the inhibitory type (Fig. 3). Under the experimental conditions employed, the oxidation reaction catalyzed by the enzyme followed Michaelis–Menten kinetics. The results gave a family of straight lines which intersected at the second quadrant (Fig. 3E-I). Therefore, it could be concluded that condensed tannins were mixed-type inhibitors (Table 2). The equilibrium constants for the inhibitor binding (the free enzyme KI and enzyme substrate complex KIS) were obtained from the slope or the vertical intercept versus the inhibitor concentration, respectively. In this work, KI and KIS were estimated to be 26.4 ± 1.3 and 61.2 ± 2.5 μg mL−1, respectively (Fig. 3E-II, III and Table 2). The value of KI was smaller than that of KIS, which indicated that the affinity of the inhibitor for free enzyme was stronger than that for the enzyme–substrate complex.
3.5. Effect of condensed tannins from V. angularis seeds on cell proliferation, intracellular tyrosinase activity and melanogenesis of B16 mouse melanoma cells
In this study, the MTT method was employed to measure the inhibitory effect of condensed tannins from V. angularis seeds on the proliferation of B16 mouse melanoma cells. When the concentration of condensed tannins was kept at 50 μg mL−1, they slightly accelerated the growth of the melanoma cells (Fig. 4). However, these tannin polymers showed inhibition in the proliferation of the cells when their concentration came up to 100 μg mL−1 and displayed a dose-dependent manner after that. The cell-survival rate decreased to 45.7 ± 1.8% when the concentration of the condensed tannins reached 400 μg mL−1. Moreover, intracellular tyrosinase activity was evaluated by using L-DOPA as the substrate. As shown in Fig. 4, the condensed tannins inhibit the activity of cellular tyrosinase in a concentration-dependent manner after their concentration reached 100 μg mL−1. With the concentration of tannin polymers at 400 μg mL−1, the relative cellular tyrosinase activity of B16 mouse melanoma cells declined to 41.7 ± 1.2%. The intracellular melanin content was further measured in the presence of tannin polymers with different concentrations by using DMSO as a negative control. The result revealed that melanogenesis was gradually inhibited with the increasing concentrations of the condensed tannins. The melanin content decreased to 40.7 ± 1.3% when the concentration of these tannin polymers came up to 400 μg mL−1. In conclusion, the condensed tannins from V. angularis seeds showed strong inhibition of the cell proliferation, cellular tyrosinase activity, and melanogenesis of B16 mouse melanoma cells.
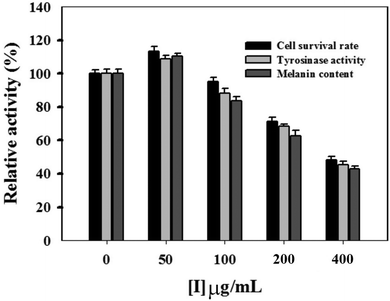 |
| Fig. 4 Effects of condensed tannins from V. angulari on cell activity, intracellular tyrosinase activity, and cellular melanin content of B16 cells. Cells were treated with compounds for 48 h at various concentrations. All the experiments were repeated three times. | |
3.6. Fluorescence quenching of mushroom tyrosinase and its substrate by condensed tannins with different concentrations
In this study, the interaction between condensed tannins from V. angularis seeds and mushroom tyrosinase and the conformational alteration of the enzyme were evaluated by fluorescence quenching. As shown in Fig. 5A, the fluorescence emission spectra of the enzyme were dramatically reduced with increasing concentrations of the condensed tannins. The maximum fluorescence intensity decreased from 101.1 ± 2.5 to 28.3 ± 1.6 when the condensed tannins concentration reached 100 μg mL−1.
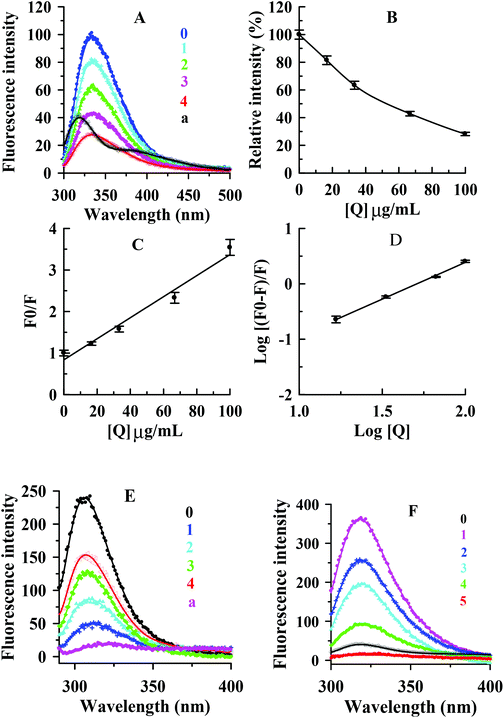 |
| Fig. 5 (A) Fluorescence emission spectra of mushroom tyrosinase in the presence of condensed tannins from V. angulari with different concentrations. (B) Maximum florescence intensity changes (%); (C) the Stern–Volmer plot for the fluorescence quenching of tyrosinase; (D) plot of log[(F0 − F)/F] against log[Q]. (E) Fluorescence emission spectra of tyrosine in the presence of inhibitor with different concentrations. (F) Fluorescence emission spectra of L-DOPA in the presence of condensed tannins with different concentrations. The proanthocyanidin concentrations for curves 0–4 were 0, 12.5, 25, 50, and 100 μg mL−1, respectively. Curve a is the fluorescence emission spectra of proanthocyanidins at 100 μg mL−1. | |
The concentration leading to 50% loss of maximum fluorescence intensity of tyrosinase was 52.5 ± 3.2 μg mL−1 (Fig. 5B). It could be concluded that the interaction between the condensed tannins and the enzyme was strong. No significant λem shift occurred with the accumulation of condensed tannins. The result indicated that the condensed tannins did not induce the enzyme conformation to change after binding to the enzyme molecule. According to the Stern–Volmer plot, the values of Kq and KSV (quenching constants) were calculated to be (2.5 ± 0.2) × 106 (μg mL−1 s−1)−1 and (2.5 ± 0.2) × 10−2 (μg mL−1)−1, respectively (Fig. 5C and Table 3). Considering the molecular weight (more than 288 g moL−1) of condensed tannins, the value of Kq could be greater than 8.68 × 1012 L mol−1 s−1, which was much greater than 2.0 × 1010 L mol−1 s−1.34 Therefore, it could be concluded that the inhibitor-enzyme complex has been formed, and the quenching mechanism of condensed tannins extracted from V. angularis seeds on the fluorescence of the enzyme was static. Fig. 5D shows that the values of KA and n were (5.4 ± 0.2) × 10−3 (μg mL−1)−1 and 1.3 ± 0.2, respectively (Table 3). The number of binding site (n) was approximately equal to 1, indicating only one binding site for the condensed tannins binding to the enzyme.
Table 3 Stern–Volmer equation for the interaction between quencher and tyrosinase
Quencher |
Type of quenching |
K
SV (μg mL−1)−1 |
K
q (μg mL−1 s−1)−1 |
K
A (μg mL−1)−1 |
n
|
Values were expressed as mean of triplicate determinations ± standard deviation. |
Condensed tannins |
Static |
(2.5 ± 0.2) × 10−2 |
(2.5 ± 0.2) × 106 |
(5.4 ± 0.2) × 10−3 |
1.3 ± 0.4 |
The interactions of the condensed tannins with substrates (L-tyrosine and L-DOPA) of tyrosinase were further investigated by using the fluorescence quenching method. Fig. 5E and F shows that the condensed tannins intensively diminished the fluorescence intensity of L-tyrosine and L-DOPA. The maximum fluorescence intensity reduced from 241.8 ± 8.3 to 151.2 ± 5.2 for L-tyrosine and from 359.8 ± 9.5 to 16.7 ± 1.3 for L-DOPA when the condensed tannins concentration reached 100 μg mL−1. There was no λem shift in both tests, indicating that the condensed tannins did not cause the change of substrate configuration. These results demonstrated that condensed tannins could inhibit the enzyme activity by binding both tyrosinase and its substrates. This offered a feasible mechanism to explain the efficient inhibition of condensed tannins on the tyrosinase catalytic reaction. Specifically, L-tyrosine and L-DOPA were proved to be hormone-like regulators of melanocyte functions,35 and this revealed the importance of the V. angularis condensed tannins in the regulation of melanocyte functions.
3.7. Molecular docking analysis
Molecular docking, a complementary application, has recently been widely employed to explore the probable interactive mechanism between inhibitor and tyrosinase.36,37 In this study, we selected catechin and epicatechin (the main constitutional unit) as ligands. The entire surfaces of protein for binding sites are shown in Fig. 6A, B, and C, D. As shown in Fig. 6E, F, G, and H, the internal forces were proved mainly to be hydrogen bonds and hydrophobic interactions. Hydrogen bonds were produced by catechin with B chain of amino residue Glu356, and epicatechin with A chain of amino residues Gln44 and Lys158, respectively (Fig. 6E, F and Table 4).
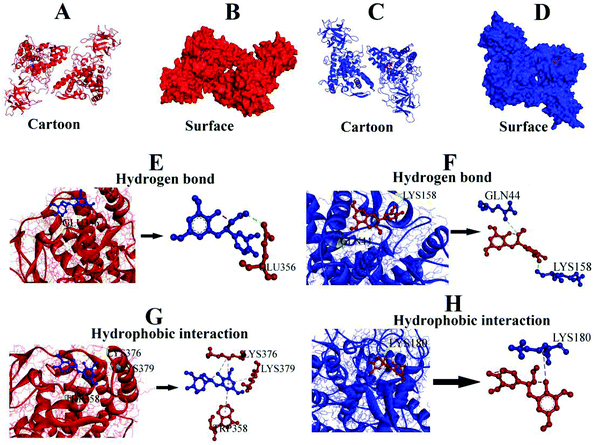 |
| Fig. 6 (A, C) Molecular docking result of the ligands (catechin and epicatechin) and tyrosinase. (B, D) Predicted binding mode of catechin/epicatechin docked with tyrosinase on the molecular surface. (E, F) Hydrogen bonds between catechin/epicatechin and amino residues. (G, H) Hydrophobic interactions of catechin/epicatechin with amino residues. | |
Table 4 Molecular docking results of the ligands with tyrosinase residues
Ligand |
Hydrogen bond |
Hydrophobic interaction |
A and B indicate chain of tyrosinase. |
Catechin |
Glu356 (B) |
Trp358, Lys376, Lys379 (B) |
Epicatechin |
Gln44, Lys158 (A) |
Lys180 (A) |
Besides, there were hydrophobic interactions between catechin and B chain of amino residues TRP358, LYS376, and LYS379, respectively (Fig. 6G, and Table 4). It was also found that hydrophobic interaction was generated between epicatechin and A chain of amino residue Lys180 (Fig. 6H and Table 4). The results obtained from molecular docking offered a feasible intrinsic mechanism to understand the efficient inhibition of condensed tannins from V. angularis seeds on the tyrosinase.
3.8. Antioxidant and DNA oxidative damage protection assays
Excessive free radicals in the body are regarded as harmful to human health, because they can lead to cell injury and death, and result in lots of diseases such as tumour, Alzheimer's disease, and arteriosclerosis.38 Uptake of food containing antioxidants from plant source could prevent the emergence of diseases.
In the current study, DPPH free-radical scavenging capacity, ABTS free-radical scavenging capacity, and FRAP (ferric reducing antioxidant power) assays were used in combination to assess the antioxidant capacity of condensed tannins from V. angularis seeds (Fig. 7). According to the DPPH and ABTS analyses, the condensed tannins showed a potent DPPH and ABTS radicals scavenging activities with the IC50 values of 60.6 ± 1.3 and 71.7 ± 0.4 μg mL−1, respectively (Fig. 7A, B and Table 5). The antioxidant activity of these compounds were significantly better than those used as standards (ascorbic acid, trolox and BHA). In addition, FRAP assay demonstrated that the condensed tannins possessed similar ferric ion reducing antioxidant power to trolox. The FRAP value was estimated to be 2.6 ± 0.1 mmol AAE g−1 (Table 5). Besides the above findings, the concentration-dependent effect was found in all three antioxidant methods. In sum, it could be concluded that condensed tannins from V. angularis seeds were good antioxidants.
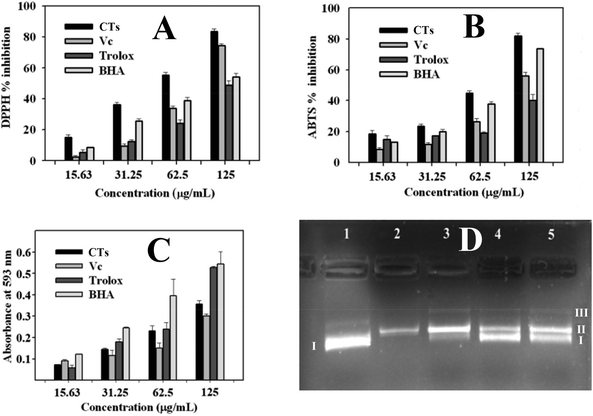 |
| Fig. 7 Antioxidant activities of the condensed tannins from V. angulari. (A) DPPH free-radical scavenging activity assay. (B) ABTS free-radical scavenging activity assay. (C) Ferric reducing antioxidant power (FRAP) assay. (D) Protective effect of condensed tannins against Pet-30a(+) plasmid DNA damage caused by hydroxyl radicals. Lane 1: plasmid DNA only; Lane 2: plasmid DNA + FeSO4 + H2O2; Lanes 3–5: plasmid DNA + FeSO4 + H2O2 + condensed tannins (5, 50, and 100 μg mL−1). Note: CTs, condensed tannins; Vc, ascorbic acid; BHA, butylated hydroxyanisole. I, supercoiled DNA; II, linear DNA; III, open circular DNA. | |
Table 5 Antioxidant activities of condensed tannins from V. angulari (DPPH free-radical scavenging assay, ABTS radical scavenging assay, and ferric reducing antioxidant power assay)
Sample |
Antioxidant activity |
IC50/DPPH (μg mL−1) |
IC50/ABTS (μg mL−1) |
FRAP (mmol AAE g−1) |
Values were expressed as the mean of triplicate determinations ± standard deviation; different letters (a,b,c,d) in the same column show significant differences from each other at P < 0.05 level. AAE: ascorbic acid equivalents. |
Condensed tannins |
60.6 ± 1.3a |
71.7 ± 0.4a |
2.6 ± 0.1b |
Ascorbic acid |
86.9 ± 3.6b |
112.8 ± 1.6c |
|
Trolox |
138.4 ± 2.0d |
159.8 ± 2.4d |
2.7 ± 0.1b |
BHA |
126.0 ± 2.9c |
83.4 ± 0.6b |
4.4 ± 0.2a |
Supercoiled plasmid DNA would be cleaved into open circular or linear DNA in the presence of hydroxyl radicals.39 The DNA damage protective activity of the condensed tannins from V. angularis seeds on plasmid DNA pET30 a(+) was evaluated by using agarose gel electrophoresis. As is shown in Fig. 7D, plasmid DNA was mainly of supercoiled form, including a small number of linear form as well (line 1). The supercoiled form was completely broken into the linear/open circular form with the addition of FeSO4 and H2O2 (line 2). In contrast, when the above reaction mixture was further added into condensed tannins with different concentrations simultaneously, the part of supercoiled plasmid DNA pET30 a(+) was protected (lines 3 to 5). In addition, the protective effect of the condensed tannins (5, 50, and 100 μg mL−1) on the plasmid DNA damage showed a concentration-dependent manner. Therefore, the condensed tannins from V. angularis seeds could be a great protective agent against DNA damage.
In summary, condensed tannins purified from the seeds of V. angularis were complex homo- and heteropolymers of procyanidins, prodelphinidins, and their derivatives. They exhibited structural heterogeneity in monomer units, interflavan linkage, DP, and substituent. They were further proved to be effective, reversible, and mixed-type inhibitors of tyrosinase. Moreover, the tannin polymers had a good inhibitory effect on cell proliferation, intracellular tyrosinase activity, and melanin production of B16 mouse melanoma cells. Interactions of these tannin polymers with the enzyme (hydrogen bonds and hydrophobic interaction) and its substrates (L-tyrosine and L-DOPA) were significant molecular mechanisms to explain their efficient inhibition in the enzyme activity. Simultaneously, the tannin polymers showed efficient antioxidant potential and DNA damage protection activity. Therefore, this study confirmed a new and efficient tyrosinase inhibitor and antioxidant, which laid a scientific foundation for the potential usage of V. angularis condensed tannins in the areas of pharmaceuticals, food, and cosmetics.
Conflicts of interest
There are no conflicts to declare.
Acknowledgements
The present investigation was supported by the Natural Science Foundation of China (No. 31501414), the Natural Science Fund of Jiangxi Province (No. 20171BAB214019), the Education Department Project of Jiangxi Province (No. GJJ150302) and the Open Project Program of Key Laboratory of Functional Small Organic Molecule, Ministry of Education, Jiangxi Normal University (No. KLFS-KF-201604).
References
- F. Garcia-Molina, J. L. Munoz, R. Varon, J. N. Rodriguez-Lopez, F. Garcia-Canovas and J. Tudela, J. Agric. Food Chem., 2007, 55, 9739–9749 CrossRef CAS PubMed.
- W. M. Chai, Q. Huang, M. Z. Lin, C. Ou-Yang, W. Y. Huang, Y. X. Wang, K. L. Xu and H. L. Feng, J. Agric. Food Chem., 2018, 66, 908–917 CrossRef CAS PubMed.
- Z. Z. Pan, H. L. Li, X. J. Yu, Q. X. Zuo, G. X. Zheng, Y. Shi, X. Liu, Y. M. Lin, G. Liang, Q. Wang and Q. X. Chen, J. Agric. Food Chem., 2011, 59, 6645–6649 CrossRef CAS PubMed.
- Y. F. Lin, Y. H. Hu, H. T. Lin, X. Liu, Y. H. Chen, S. Zhang and Q. X. Chen, J. Agric. Food Chem., 2013, 61, 2889–2895 CrossRef CAS PubMed.
- W. Yi, X. Q. Wu, R. H. Cao, H. C. Song and L. Ma, Food Chem., 2009, 117, 381–386 CrossRef CAS.
- Z. P. Zheng, Q. Zhu, C. L. Fan, H. Y. Tan and M. F. Wang, Food Funct., 2011, 2, 259–264 RSC.
- G. Zengin, C. Sarikurkcu, E. Gunes, A. Uysal, R. Ceylan, S. Uysal, H. Gungor and A. Aktumsek, Food Funct., 2015, 6, 2794–2802 RSC.
- M. E. Chiari, M. B. Joray, G. Ruiz, S. M. Palacios and M. C. Carpinella, Food Chem., 2010, 120, 10–14 CrossRef CAS.
- J. K. Hellström, A. R. Törrönen and P. H. Mattila, J. Agric. Food Chem., 2009, 57, 7899–7906 CrossRef PubMed.
- S. de Pascual-Teresa and M. N. Clifford, J. Agric. Food Chem., 2017, 65, 8093–8095 CrossRef.
- C. S. Malisch, J. P. Salminen, R. Kolliker, M. T. Engstrom, D. Suter, B. Studer and A. Luscher, J. Agric. Food Chem., 2016, 64, 9307–9316 CrossRef CAS PubMed.
- P. M. Aron and J. A. Kennedy, Mol. Nutr. Food Res., 2008, 52, 79–104 CrossRef CAS.
- A. Smeriglio, D. Barreca, E. Bellocco and D. Trombetta, Br. J. Pharmacol., 2017, 174, 1244–1262 CrossRef CAS.
- W. M. Chai, C. Ou Yang, Q. Huang, M. Z. Lin, Y. X. Wang, K. L. Xu, W. Y. Huang and D. D. Pang, Food Chem., 2018, 260, 27–36 CrossRef CAS.
- H. C. Zhou, Y. M. Lin, S. D. Wei and N. F. Y. Tam, Food Chem., 2011, 129, 1710–1720 CrossRef CAS.
- W. Hümmer and P. Schreier, Mol. Nutr. Food Res., 2008, 52, 1381–1398 CrossRef.
- W. M. Chai, M. Z. Lin, H. L. Feng, Z. R. Zou and Y. X. Wang, Food Funct., 2017, 8, 1043–1051 RSC.
- K. Fraser, V. Collette and K. R. Hancock, J. Agric. Food Chem., 2016, 64, 6676–6684 CrossRef CAS PubMed.
- T. Yu, H. M. Ahn, T. Shen, K. Yoon, H. J. Jang, Y. J. Lee, H. M. Yang, J. H. Kim, C. Kim, M. H. Han, S. H. Cha, T. W. Kim, S. Y. Kim, J. Lee and J. Y. Cho, J. Ethnopharmacol., 2011, 137, 1197–1206 CrossRef PubMed.
- K. H. Han, T. Kitano-Okada, J. M. Seo, S. J. Kim, K. Sasaki, K. Shimada and M. Fukushima, J. Funct. Foods, 2015, 14, 692–701 CrossRef CAS.
- W. Kawakami, A. Oshima and E. Yanase, Food Chem., 2018, 239, 1110–1116 CrossRef CAS PubMed.
- T. Terrill, A. Rowan, G. Douglas and T. Barry, J. Sci. Food Agric., 1992, 58, 321–329 CrossRef CAS.
- W. M. Chai, M. Z. Lin, Y. X. Wang, K. L. Xu, W. Y. Huang, D. D. Pan, Z. R. Zou and Y. Y. Peng, Food Res. Int., 2017, 100, 731–739 CrossRef CAS.
- W. M. Chai, M. K. Wei, R. Wang, R. G. Deng, Z. R. Zou and Y. Y. Peng, J. Agric. Food Chem., 2015, 63, 7381–7387 CrossRef CAS.
- W. M. Chai, C. M. Chen, Y. S. Gao, H. L. Feng, Y. M. Ding, Y. Shi, H. T. Zhou and Q. X. Chen, J. Agric. Food Chem., 2014, 62, 123–129 CrossRef CAS.
- L. W. Gu, M. Kelm, J. F. Hammerstone, G. Beecher, D. Cunningham, S. Vannozzi and R. L. Prior, J. Agric. Food Chem., 2002, 50, 4852–4860 CrossRef CAS.
- W. M. Chai, Y. Shi, H. L. Feng, L. Xu, Z. H. Xiang, Y. S. Gao and Q. X. Chen, J. Agric. Food Chem., 2014, 62, 6382–6389 CrossRef CAS PubMed.
- P. R. Twentyman and M. Luscombe, Br. J. Cancer, 1987, 56, 279 CrossRef CAS PubMed.
- L. H. Chen, Y. H. Hu, W. Song, K. K. Song, X. Liu, Y. L. Jia, J. X. Zhuang and Q. X. Chen, J. Agric. Food Chem., 2012, 60, 1542–1547 CrossRef CAS PubMed.
- M. S. Silva, I. Garcia-Estevez, E. Brandao, N. Mateus, V. de Freitas and S. Soares, J. Agric. Food Chem., 2017, 65, 6415–6424 CrossRef CAS PubMed.
- J. D. Reed, C. G. Krueger and M. M. Vestling, Phytochemistry, 2005, 66, 2248–2263 CrossRef CAS.
- P. Xiang, Y. Lin, P. Lin, C. Xiang, Z. Yang and Z. Lu, J. Appl. Polym. Sci., 2007, 105, 859–864 CrossRef CAS.
- M. M. Jiménez, S. Chazarra, J. Escribano, J. Cabanes and F. Garcia-Carmona, J. Agric. Food Chem., 2001, 49, 4060–4063 CrossRef.
- Y. Xie, W. Yang, X. Chen and J. Xiao, Food Funct., 2014, 5, 2582–2589 RSC.
- A. Slominski, M. A. Zmijewski and J. Pawelek, Pigm. Cell Melanoma Res., 2012, 25, 14–27 CrossRef CAS.
- W. M. Chai, M. Z. Lin, F. J. Song, Y. X. Wang, K. L. Xu, J. X. Huang, J. P. Fu and Y. Y. Peng, Int. J. Biol. Macromol., 2017, 102, 425–430 CrossRef CAS PubMed.
- R. Thomsen and M. H. Christensen, J. Med. Chem., 2006, 49, 3315–3321 CrossRef CAS PubMed.
- T. A. Siswoyo, E. Mardiana, K. O. Lee and K. Hoshokawa, J. Agric. Food Chem., 2011, 59, 5648–5656 CrossRef CAS PubMed.
- B. N. Singh, B. R. Singh, R. L. Singh, D. Prakash, R. Dhakarey, G. Upadhyay and H. B. Singh, Food Chem. Toxicol., 2009, 47, 1109–1116 CrossRef CAS.
|
This journal is © The Royal Society of Chemistry 2019 |