DOI:
10.1039/C8FO01725E
(Paper)
Food Funct., 2019,
10, 180-190
Physicochemical characterization of organogels prepared from menhaden oil or structured lipid with phytosterol blend or sucrose stearate/ascorbyl palmitate blend
Received
31st August 2018
, Accepted 28th November 2018
First published on 30th November 2018
Abstract
The purpose of this research was to produce organogels using two different organogelator blends and two different oil substrates and compare their physicochemical properties. A medium-long-medium (MLM)-type structured lipid (SL) containing 37.44 mol% MLM-type triacylglycerols (TAG) was produced by enzymatic modification of menhaden oil with ethyl caprate. Menhaden oil and SL were used as oil substrates to form organogels. One organogel was formed using a phytosterol blend of β-sitosterol and γ-oryzanol (molar ratios of 2
:
1, 1
:
1, and 1
:
2 at 4, 6, and 8% (w/w) in oil), and another was formed with a blend of sucrose stearate (HLB value: 2) and ascorbyl palmitate (SSAP) (molar ratios of 3
:
1, 1
:
1, and 1
:
3 at 8, 10, and 12% (w/w) in oil). Organogels were formed for all ratios except 2
:
1 and 3
:
1 for the phytosterol and SSAP blend, respectively. For both organogels, the 1
:
1 molar ratio was optimal. This ratio produced organogels with higher melting completion temperatures than menhaden oil and SL (13.6 and 14.3 °C). The SSAP blend had higher melting completion temperatures (72.3 and 72.4 °C) than the phytosterol blend (69.2 and 68.4 °C) for organogels formed using menhaden oil and SL, respectively. All 1
:
1 molar ratio blends exhibited β′ polymorphic form with short spacing peaks at 4.20, 3.97, and 3.71 Å. All organogels improved the oxidative stability of the menhaden oil and SL. These organogels have the potential for use as nutraceuticals or health beneficial low saturated fat alternatives to saturated and/or trans-fats.
Introduction
It has been reported that consuming significant amounts of saturated fats will raise LDL cholesterol in the body, which increases the risk of heart disease and stroke.1 It is necessary to reduce the consumption of highly saturated fats by consuming more monounsaturated fatty acids (MUFA) or polyunsaturated fatty acids (PUFA). Many fish oils and plant oils contain significant amounts of MUFA or PUFA.2 However, fats and oils do not have similar applications in food products.3 So, there is a need to produce a low saturated, zero trans-fat that behaves similarly to highly saturated fats in terms of their physicochemical properties.
Organogels (also known as oleogels for edible purposes) are a promising alternative for high saturated and trans-fats. Most oils contain saturated fat naturally, but in significantly lower amounts than in highly saturated fats.4 Organogels, a lipid gel, have the potential for many applications within and outside the food industry. Studies have shown that these organogels may inhibit oil migration in chocolate and control the release of health beneficial sensitive compounds such as antioxidants, bioactive compounds, and PUFA.4 Organogels have been shown to increase oxidative stability of the oil by trapping the oil in a gel network so there is decreased exposure to oxygen.5 Certain celluloses, waxes, and phytosterols such as a blend of β-sitosterol and γ-oryzanol have been shown to be good organogelators for food applications.4,6
A blend of sucrose stearate/ascorbyl palmitate (SSAP) is proposed in this research to form an organogel. This blend is significantly cheaper than the phytosterol blend and the gelators are food-grade as well. Sucrose stearate is a sucrose fatty acid ester of stearic acid and sucrose and is a known food emulsifier.7 Sucrose stearate has different hydrophile-lipophile balance (HLB) values based on the number of stearic acids that are esterified to the free hydroxyl groups of sucrose. The more stearic acid that is esterified, the lower the HLB number and the more lipophilic the molecule is. Ascorbyl palmitate is a fatty acid ester of ascorbic acid and palmitic acid with known antioxidant capability.8 Both sucrose stearate and ascorbyl palmitate have long chain fatty acid tails that are ester linked to cyclic polar compounds. There are currently no publications on the use of this blend to form an organogel. There may likely be intermolecular interactions between the long hydrocarbon chains, polar functional groups, and oil phase.9 It is of interest to investigate the type of structure and physicochemical properties that this blend will have since literature has shown that several different structures may form based on the organogelator(s) and the oil phase.4
The objective of this study was to form different organogels with menhaden oil or a structured lipid (SL) as the oil phase. Oils such as menhaden oil that are lower in saturated fat and contain significant amounts of health beneficial PUFA, could be used to form organogels with additional nutritional benefits such as promoting cardiovascular health, reducing blood triacylglycerol (TAG) levels, inflammation, and risk of neurodegenerative diseases.1 To the best of our knowledge, there is no research on the formation of an organogel using a SL as the oil phase. Specifically, medium-long-medium (MLM)-type SL are of interest for use in forming organogels because of the desirable nutritional benefits.10 The medium chain fatty acids (FA) in an MLM-type SL are metabolized quickly in the body and provide quick energy. The long chain FA, in 2-monoacylglycerol form, is more readily absorbed. These organogels may also improve the oxidative stability and alter the physicochemical properties of the menhaden oil and SL, allowing for their use in a wider range of applications.
Materials and methods
Materials
Menhaden oil, obtained from Omega Protein Inc. (Reedville, VA, USA), and the acyl donor ethyl caprate (≥98% purity), purchased from Sigma-Aldrich Chemical Co. (St Louis, MO, USA), were used as substrates in an interesterification reaction to produce an MLM-type SL. The SL was prepared according to the method outlined previously.11 Briefly, menhaden oil and ethyl caprate at a 1
:
3 substrate molar ratio were mixed with Lipozyme® 435 lipase (Novozymes North America, Inc., Franklinton, NC, USA) at 10% (w/w) of total substrates. The reaction took place in a 1 L batch reactor at 60 °C, with stirring at 250 rpm using a SL 2400 StedFast stirrer (Fisher Scientific Co., Fair Lawn, NJ, USA) for 16 h. Table 1 shows the relative fatty acid composition of the menhaden oil and SL. The SL contained 29.93 ± 0.26 mol% capric acid at the sn-1,3 positions, and 12.95 ± 0.36 mol% EPA and 19.53 ± 0.45 mol% DHA at the sn-2 position. The SL contained 37.44 mol% MLM-type TAG. The detailed fatty acid and TAG composition, oxidative stability, and thermal behavior of the SL have been discussed in further detail elsewhere.11 Ryoto Sugar Ester S-270 (melting point 61 °C), a sucrose fatty acid ester, was obtained from Mitsubishi-Kagaku Foods Corporation (Tokyo, Japan). The “S” corresponds to stearic acid as the primary fatty acid esterified to sucrose. The designation of “270” corresponds to the HLB value, ester composition, and purity of the stearic acid. The “2” indicates the product has an HLB value of 2, and the “70” indicates that the purity of stearic acid is 70%. Per manufacturer details, the Ryoto Sugar Ester S-270 contains 10% sucrose monostearate and 90% sucrose di-, tri-, and poly-stearate. Ascorbyl palmitate (melting point 115 °C) and β-sitosterol (melting point 136 °C) were obtained from Sigma Aldrich Chemical Co. (St Louis, MO, USA). The γ-oryzanol (melting point 151 °C) was obtained from TCI America (Portland, OR, USA). All other reagents and solvents were of analytical or HPLC grade and were purchased from Fisher Chemical (Fair Lawn, NJ, USA), Sigma-Aldrich Chemical Co (St Louis, MO, USA), and J. T. Baker Chemical Co. (Phillipsburg, NJ, USA).
Table 1 Relative fatty acid composition (mol%) of menhaden oil and structured lipid, SL
Sample |
Fatty acidc (mol%) |
Position |
C10:0 |
C20:5n3 |
C22:6n3 |
Mean ± STD, n = 3, ND = not detected. SL reaction conditions: Menhaden oil: ethyl caprate substrate molar ratio 1 : 3, 10% Lipozyme® 435 lipase (w/w), 60 °C, stirred at 250 rpm for 16 h in a 1 L batch reactor. sn-1,3 mol% determined using equation: sn-1,3 (mol%) = [3 × total mol% − sn-2 mol%]/2. Other major fatty acids were C14:0, C16:0, & C16:1n-7. |
Menhaden oil |
Total |
ND |
22.82 ± 1.85 |
16.97 ± 1.83 |
sn-2 |
ND |
15.32 ± 0.74 |
29.34 ± 3.08 |
sn-1,3b |
ND |
26.57 ± 2.41 |
10.79 ± 1.21 |
SLa |
Total |
26.73 ± 0.05 |
17.09 ± 0.39 |
10.57 ± 0.23 |
sn-2 |
20.33 ± 0.66 |
12.95 ± 0.36 |
19.53 ± 0.45 |
sn-1,3 |
29.93 ± 0.26 |
19.16 ± 0.41 |
6.09 ± 0.12 |
Organogel preparation
The phytosterol organogels were formed in triplicate by dissolving the blend of β-sitosterol and γ-oryzanol at various concentrations in 10 g of oil (menhaden oil or SL) at 90 °C. The varying blend molar ratios and phytosterol
:
oil (w/w%) were based on previous literature and are shown in Table 2.12 The mixture was stirred constantly for 10 min until fully dissolved and were then poured into a vial. Samples were flushed with nitrogen and cooled at 4 °C to set the gel and stored until further analysis.
Table 2 Parameters for producing the phytosterol organogels under varying phytosterol blend molar ratios and phytosterol blend
:
oil (w/w%) ratios
Gel number |
β-Sitosterol/γ-oryzanol molar ratio |
Blend to oil ratio (w/w%) |
1 |
2 : 1 |
4 |
2 |
6 |
3 |
8 |
4 |
1 : 1 |
4 |
5 |
6 |
6 |
8 |
7 |
1 : 2 |
4 |
8 |
6 |
9 |
8 |
Selection of sucrose stearate with HLB value of 2 was based upon previous literature that formed organogels with sucrose stearate (HLB value of 3) and Tween 80.7 There is a need for a balance between solubility and insolubility of the gelators within the solvent.4 Individually, it was found that neither sucrose stearate (HLB values 1–15) or ascorbyl palmitate formed organogels at concentrations between 5–25% with menhaden oil or SL but formed viscous solutions or precipitates. Too much insolubility resulted in a precipitate (HLB values >8) while too much solubility resulted in a solution, that is, not a gel (HLB values <8). Sucrose stearate with HLB value 2 resulted in a more viscous appearing solution than sucrose stearate of other HLB values less than 8. The extensive hydrogen bonding between polar hydroxyl groups of both the sucrose stearate and ascorbyl palmitate may contribute to the stabilization of the organogel.13 In triplicate, the SSAP organogels were formed by dissolving the blend of sucrose stearate (HLB value 2) and ascorbyl palmitate at various concentrations in 10 g of oil (menhaden oil or SL) at 110 °C. The varying blend weight ratios and blend
:
oil (w/w%) are shown in Table 3. The solution was stirred constantly for 10 min until fully dissolved and were then poured into a vial. Samples were flushed with nitrogen and cooled at 4 °C to set the gel and stored until further analysis.
Table 3 Parameters for producing the sucrose stearate/ascorbyl palmitate (SSAP) organogels under varying SSAP blend molar ratios and SSAP blend
:
oil (w/w%) ratios
Gel number |
Sucrose stearate/ascorbyl palmitate molar ratio |
Blend to oil ratio (w/w%) |
1 |
3 : 1 |
8 |
2 |
10 |
3 |
12 |
4 |
1 : 1 |
8 |
5 |
10 |
6 |
12 |
7 |
1 : 3 |
8 |
8 |
10 |
9 |
12 |
Thermal behavior
Thermal behavior of products was analyzed using a 204 F-1 Phoenix differential scanning calorimeter (DSC) (Netzsch-Gerätebau GmbH, Selb, Germany). DSC analysis was performed using the AOCS Official Method Cj 1-94.14 Crystallization onset and melting completion temperatures were determined using Proteus thermal analysis software (Netzsch-Gerätebau GmbH, Selb, Germany). Samples were analyzed in triplicate and average values were reported.
Rheological properties
Rheological analyses were performed using an HR-3 Discovery Hybrid Rheometer (TA Instruments, New Castle, DE, USA). A parallel plate (P50 Ti L, diameter 50 nm, gap = 1 ± 0.2 nm) was used for measurements. Temperature was controlled with a Peltier Plate Temperature System (TA Instruments, New Castle, DE, USA). All experiments were conducted in triplicate. Data was analyzed using Trios software (TA Instruments, New Castle, DE, USA).
Heating–cooling sweeps were performed between 5 and 90 °C at 1 °C min−1 with a frequency fixed at 1 Hz to evaluate the gel formation process. Changes in the slope of complex modulus (G*) as a function of temperature were evaluated using the Savitzky and Golay filter to better visualize the thermal transitions.15 All measurements were done within the linear viscoelastic region.
Flow curves were obtained using a shear rate ranging from 5 to 200 s−1 at 5 and 25 °C. The organogels underwent three shear rate sweeps (up–down–up) to eliminate thixotropy and data was obtained in steady state. Apparent viscosity was also evaluated at 5 and 25 °C at shear rates of 10, 50, and 100 s−1 to mimic changes during common food processing conditions. The Power-law model was used to fit the non-Newtonian fluid behavior and is defined by the equation: σ = k (γ)n, σ is shear stress (Pa) and (γ) is shear rate (s−1).16
Polarized light microscopy
The morphology of the organogels was characterized and imaged by a Leica DMRXA2 Microscope (Leica Micro-systems Canada Inc., Richmond Hill, Canada). To prepare slides, samples were heated to 60 °C and 1 drop of melted sample was placed between a stationary and moving glass plate. The samples were then crystallized by storing at 4 °C overnight.17 Images were acquired using a charged coupled device (CCD) camera (QImaging Retiga, Burnaby, BC, Canada).
X-Ray diffraction
Samples were first annealed before analysis. Samples and sample pans were placed at 60 °C for 1 h in the oven. Melted samples were transferred to the warm sample pans, then held at 25 °C for 4 h. Cooled samples were then placed at 4 °C for an additional 12 h. Finally, samples were stored at −80 °C until analysis. A Bruker D8 Advance X-ray powder diffractometer (Billerica, MA, USA) was used to analyze the polymorphism. The operating conditions were Co Kα radiation (λ = 1.79037 Å), voltage 35 kV, amperage 40 mA, scanning rate of 0.2° s−1, and a diffraction angle (2θ) range from 10–40°. Samples were analyzed in triplicate and short d-spacings (Å) of the crystalline structures were determined using EVA-diffraction software (Billerica, MA, USA).
Solid fat content
Solid fat content (SFC) was analyzed using a Benchtop NMR MQC Analyzer (Oxford Instruments, Oxfordshire, UK) following the AOCS Official Method Cd 16b-93 for non-stabilizing fats.14 Calibration standards (Oxford Instruments, Oxfordshire, UK) had SFC values of 0, 32.6, and 70.5%. Samples were melted at 100 °C and held for 15 min then filled in NMR tube to 1.5 cm height. Samples in NMR tubes were tempered at 60 °C for 5 min, cooled to 0 °C for 60 min, and then held at each measuring temperature for 30 min. Measuring temperatures were at 5 °C intervals from 0–40 °C. Samples were analyzed in triplicate and average values were reported.
Oxidative stability
Oxidative stability was conducted by measuring peroxide (PV) and p-anisidine (p-AV) values over 12 days. 5 g of sample was weighed into glass vials, closed, and placed in a Reacti-Therm™ Heating and Stirring Module (Thermo Fisher Scientific, Waltham, MA, USA) at 65 °C. PV was determined using a method from the International Dairy foundation.18 The p-AV was determined using AOCS Official Method Cd 18-90.14 Using PV and p-AV data, the total oxidation (TOTOX) value was calculated. Oil stability index (OSI) of products was determined using an Oxidative Stability Instrument (Omnion Inc., Rockland, MA, USA) and AOCS Official Method Cd 12b-92.14
Statistical analysis
Statistical analysis was performed on the data using JMP® software (Version 10, SAS Institute Inc., Cary, NC, USA). Data was analyzed using ANOVA and significant differences (p < 0.05) were determined by the Tukey test.
Results and discussion
Organogel formation
Fig. 1 shows the phytosterol and SSAP organogels at various organogelator blend ratios. Organogels were formed for all molar ratios except 2
:
1 and 3
:
1 for the phytosterol and SSAP blend, respectively. Because these two ratios did not form organogels, they were not considered for further analysis.
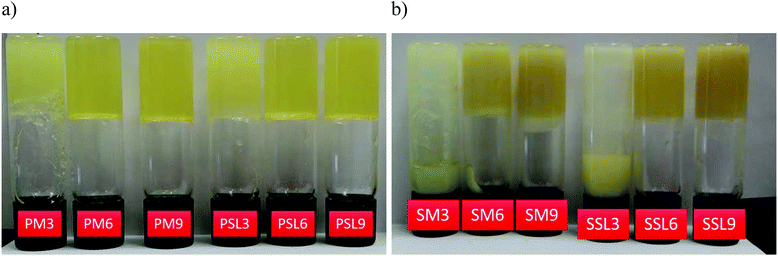 |
| Fig. 1 Phytosterol (a) and SSAP (b) organogels. P: Phytosterol organogel; M: menhaden oil; SL: structured lipid, S: SSAP organogel; 6: 1 : 1 molar ratio of organogelators, corresponds to gel number from Tables 2 and 3 for phytosterol and SSAP organogels, respectively. | |
The phytosterol blend formed organogels that appeared translucent with both oil phases at organogelator concentrations of 6 and 8% (w/w) for the 1
:
1 molar ratio and only at 8% (w/w) for the 1
:
2 molar ratio. However, when the phytosterol organogels were stored over a 1-month period at 4 °C, it was noted that only the 8% (w/w) for the 1
:
1 molar ratio showed no phase separation. Similar results were seen in previous literature that the 1
:
1 molar ratio was optimal for the phytosterol blend.12 Due to the higher stability over time, this blend was chosen for further analysis, labeled as PM6 and PSL6 for the phytosterol blend organogels that were formed with menhaden oil or SL, respectively.
The SSAP blend formed organogels that were opaque with both oil phases at organogelator concentrations of 10 and 12% (w/w) for the 1
:
1 molar ratio, and only at 12% for the 1
:
3 molar ratio. However, when the SSAP organogels were stored over a 1-month period, it was noted that only the 12% (w/w) for the 1
:
1 molar ratio showed no phase separation. A previous study found that the stability of organogels produced with sorbitan monostearate, a hydrophobic surfactant, was stabilized by addition of polysorbate 20, a hydrophilic surfactant.19 The hydroxyl groups of the ascorbic acid in ascorbyl palmitate may function to help stabilize the organogel. Due to the higher stability over time, this blend was chosen for further analysis, labeled as SM6 and SSL6, for the SSAP blend organogels that were formed with menhaden oil or SL, respectively.
Thermal behavior
Fig. 2 shows DSC thermograms of the crystallization (a) and melting (b) profiles of menhaden oil, SL, and organogels (PM6, PSL6, SM6, and SSL6). The SL did not have significantly different (p > 0.05) crystallization onset (C0) and melting completion (Mc) temperatures than the unmodified menhaden oil at −6.5 and 14.3 °C versus −5.3 and 13.6 °C, respectively. Menhaden oil had a higher melting peak, and this is likely a TAG molecular species containing stearic acid. Menhaden oil was found to contain a little less than 1 mol% tristearin TAG.11 PSL6 and PM6 C0 and Mc temperatures were not significantly different (p > 0.05), at 64.2 and 68.4 °C versus 64.9 and 69.2 °C, respectively. The phytosterol organogels had higher C0 and Mc temperatures (p < 0.05) when compared to the original oils. A previous study on the phytosterol organogel showed that C0 and Mc temperatures were between 40–60 °C and 60–80 °C, respectively, depending on the organogelator ratio and concentration in sunflower oil.20 The SSAP organogels had significantly higher (p < 0.05) C0 and Mc temperatures than the phytosterol blend organogels, at 66.5 and 72.4 °C, and 66.4 and 72.3 °C, for SM6 and SSL6, respectively. There was no significant difference in C0 and Mc temperatures between SM6 and SSL6 (p > 0.05). The SSAP organogels likely have higher Mc temperatures due to the higher melting stearic and palmitic acid found in sucrose stearate and ascorbyl palmitate, respectively, that interact with the non-polar oil phases.
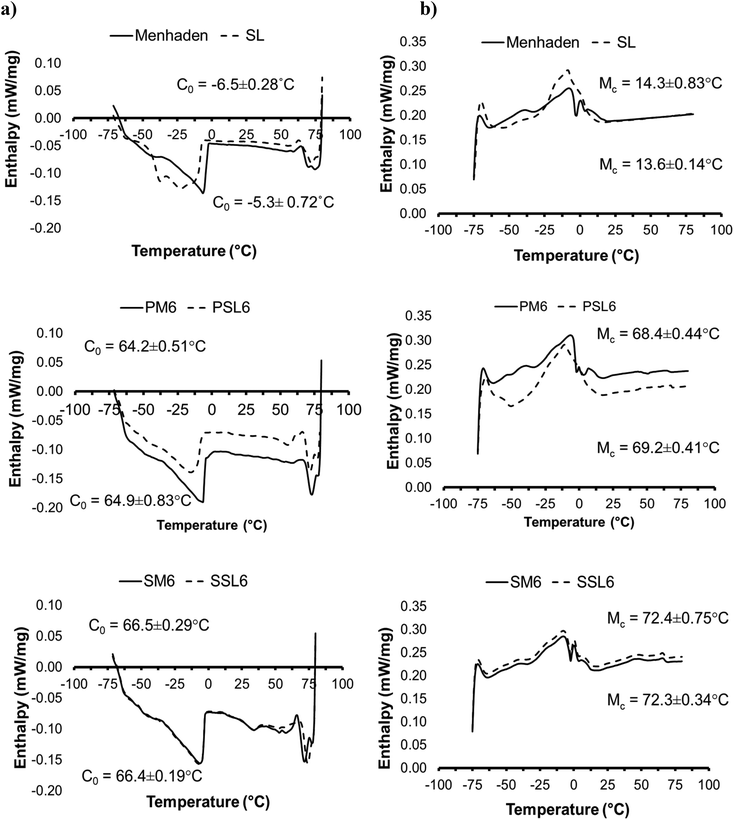 |
| Fig. 2 Differential scanning calorimetry (DSC) thermograms: (a) crystallization and (b) melting profiles of menhaden oil, SL, and organogels (PM6, PSL6, SM6, and SSL6)*. C0 and Mc are crystallization onset and melting completion temperatures, respectively. For both (a) and (b), the top temperature value is for SL, PSL6, and SSL6, while the bottom temperature is for menhaden oil, PM6, and SM6. *P: Phytosterol organogel; M: menhaden oil; SL: structured lipid, S: SSAP organogel; 6: 1 : 1 molar ratio of organogelators, corresponds to gel number from Tables 2 and 3 for phytosterol and SSAP organogels, respectively. | |
Looking at the DSC curves in Fig. 2 there was a lower enthalpy peak, with a broad melting and crystallization range at higher temperatures for the phytosterol and SSAP organogels. This suggests that there are likely two different events occurring. The reason why the second peaks have a lower enthalpy is likely due to the small quantity of sample that is melting at the higher temperature, which may not release enough heat to be resolved by DSC.21 The bulk of the organogel is menhaden oil or SL, which have relatively low melting points compared to the organogelators that are present in small quantities. Another reason is that the organogel network may have low thermal resistance.16 The thermal behavior results were further discussed when comparing the DSC values to the rheological data.
Rheological properties
Fig. 3 shows rheological properties during heating and cooling sweeps for the phytosterol and SSAP organogels (PM6, PSL6, SM6, and SSL6). These results showed that the organogels exhibited thermoreversibility. During heating, shown in Fig. 3a, complex modulus (G*) values decreased after 30 °C. The organogels formed with the SL generally exhibited higher G* values than organogels formed with menhaden oil. This is likely due to the higher amount of saturated fatty acids in the SL. A higher SFC typically results in higher rheological values.22 At temperatures above 40 °C, the G* values of the SSAP organogels were higher than the phytosterol organogels. At temperatures above 50 °C, the behavior of the phytosterol and SSAP organogels were similar, likely due to the complete melting of the organogel network, similarly observed by the thermal behavior. Melting temperatures were estimated from the rheological measurements using Savitzky and Golay filter on inflection point, presenting values of 42.0 °C, 45.2 °C, 52.1 °C, and 53.1 °C for PM6, PSL6, SM6, and SSL6, respectively.15 These values correspond to the higher temperature peak on the DSC results (Fig. 2) and is related to the breakage of the organogel network.
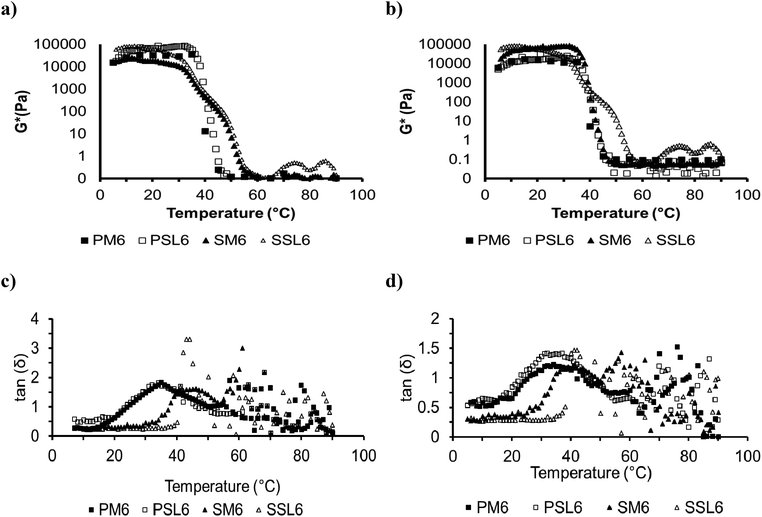 |
| Fig. 3 Temperature sweep of phytosterol organogels (PM6 and PSL6), and SSAP organogels (SM6 and SSL6) *, (a) complex modulus of heating sweep, (b) complex modulus of cooling sweep, (c) tan delta of heating sweep, and (d) tan delta of cooling sweep. *P: Phytosterol organogel; S: SSAP organogel; M: menhaden oil; SL: structured lipid, 6: 1 : 1 molar ratio of organogelators, corresponds to gel number from Tables 2 and 3 for phytosterol and SSAP organogels, respectively. | |
Fig. 3b shows the G* values during the cooling temperature sweep. A similar rheological pattern was observed for both the heating and cooling sweeps. Only SM6 had G* values that increased dramatically at a lower temperature (44.1 °C) than the heating sweep (52.1 °C). There was also no significant difference in G* values between organogels formed with menhaden oil or the SL for the SSAP organogels. Crystallization temperatures were also estimated as 40.1 °C, 43.1 °C, 44.1 °C, 53.1 °C, for PM6, PSL6, SM6, and SSL6, respectively. In this temperature range the point at which tan(δ) = 1, or gelation point, was also observed. These values correspond to the second peak on the DSC thermograms (Fig. 2) and are related to the formation of the organogel network.
Fig. 3c and d show the loss tangent curves for the phytosterol and SSAP organogels (PM6, PSL6, SM6, and SSL6). At temperatures lower than 25.1 °C or 38.1–42.1 °C for phytosterol or SSAP organogels, respectively, tan(δ) < 1, indicating that the values of G′ were higher than G′′. At higher temperatures, the tangent values returned to be lower than 1. The point at which tan(δ) = 1 (G′ to G′′ crossover) may serve as a simple indicator of gelation point. When comparing the heating and cooling sweeps, there was no significant difference in gelation point for the phytosterol organogels (p > 0.05). However, for the SSAP organogels there was a decrease (p < 0.05) in gelation points of SM6 (38.1 and 34.3 °C) and SSL6 (42.1 and 39.9 °C) for heating and cooling sweeps, respectively.
Fig. 4 shows the flow curves for the phytosterol (PM6 and PSL6) and SSAP organogels (SM6 and SSL6) at (a) 5 and (b) 25 °C. Table 4 shows the estimated apparent viscosity and Power-law parameters for the organogels. The Power-law model for S2 curves (steady state) was fitted to all organogels and rheological parameters (flow index n and consistency index k) were estimated. The flow index values for the phytosterol organogels were slightly higher than the SSAP organogels. The SSAP organogel showed higher pseudoplasticity (lower n), consistency index, and viscosity than the phytosterol organogels, which is characteristic of a more complex and dense gel network.16 The apparent viscosities show that the SSAP organogels had higher apparent viscosities than the phytosterol organogels, and the organogels formed with the SL had higher apparent viscosities than organogels formed with menhaden oil.
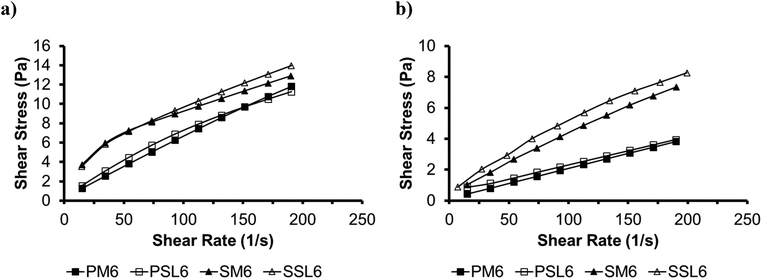 |
| Fig. 4 Flow curves of phytosterol organogels (PM6 and PSL6), and SSAP organogels (SM6 and SSL6) * at (a) 5 °C and (b) 25 °C obtained at steady state. *P: Phytosterol organogel; S: SSAP organogel; M: menhaden oil; SL: structured lipid, 6: 1 : 1 molar ratio of organogelators, corresponds to gel number from Tables 2 and 3 for phytosterol and SSAP organogels, respectively. | |
Table 4 Estimated Power-law parameters and apparent viscosity of phytosterol organogels (PM6 and PSL6), and sucrose stearate/ascorbyl palmitate (SSAP) organogels (SM6 and SSL6)
Samplea |
PM6 |
PSL6 |
SM6 |
SSL6 |
5 °C |
25 °C |
5 °C |
25 °C |
5 °C |
25 °C |
5 °C |
25 °C |
P: Phytosterol organogel; S: SSAP organogel; M: menhaden oil; SL: structured lipid, reaction conditions and relative fatty acid composition as described in Table 1; 6: 1 : 1 molar ratio of organogelators, corresponds to gel number from Tables 2 and 3 for phytosterol and SSAP organogels, respectively.
|
n
|
0.891 ± 0.01 |
0.740 ± 0.02 |
0.739 ± 0.03 |
0.700 ± 0.02 |
0.529 ± 0.41 |
0.501 ± 0.05 |
0.508 ± 0.01 |
0.467 ± 0.02 |
k (Pa sn) |
0.153 ± 0.02 |
0.141 ± 0.01 |
0.345 ± 0.01 |
0.330 ± 0.25 |
0.823 ± 0.18 |
0.166 ± 0.73 |
1.043 ± 0.02 |
0.838± 0.15 |
R
2
|
0.999 |
0.998 |
0.998 |
0.999 |
0.999 |
0.999 |
0.997 |
0.999 |
η
10 (Pa s) |
0.0861 |
0.0592 |
0.127 |
0.104 |
0.240 |
0.0690 |
0.252 |
0.183 |
η
50 (Pa s) |
0.0707 |
0.0272 |
0.0825 |
0.0606 |
0.132 |
0.0494 |
0.134 |
0.0971 |
η
100 (Pa s) |
0.0662 |
0.0226 |
0.0703 |
0.0503 |
0.0916 |
0.0432 |
0.0967 |
0.0658 |
Polarized light microscopy
Polarized light micrographs of phytosterol and SSAP organogels are shown in Fig. 5. The micrographs show that the crystal morphology of the organogels were influenced by both oil and organogelator type. The crystalline structures of all organogels exhibited birefringence. The crystal morphology of the organogels produced with menhaden oil (PM6 and SM6) were fibrous or plate-like while the crystal morphology of the organogels produced with the SL (PSL6 and SSL6) were needle-like. The needle-like morphology was found in previous studies to have a higher propensity for oil binding.9,23 Previous studies have found that larger particle size and needle-like morphology correspond to higher viscosity.20 The SSAP organogels were shown to exhibit higher apparent viscosities (Fig. 4). The SSAP organogel networks were also denser and filled with many small crystals, which typically corresponds to a harder, more viscous fat.
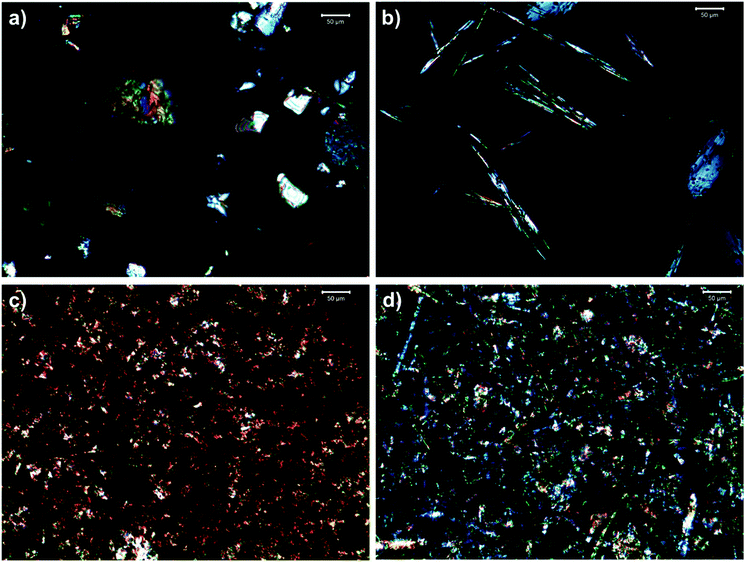 |
| Fig. 5 Polarized light micrographs of (a) PM6, (b) PSL6, (c) SM6, and (d) SSL6. P: Phytosterol organogel; S: SSAP organogel; M: menhaden oil; SL: structured lipid, 6: 1 : 1 molar ratio of organogelators, corresponds to gel number from Tables 2 and 3 for phytosterol and SSAP organogels, respectively. | |
The differences in morphology based on oil type are likely due to the differences in oil composition. The menhaden oil and SL have different fatty acid compositions, TAG molecular species, and thermal behavior.11 When comparing organogelator type, the SSAP blend organogels had a crystal matrix that was more densely packed with smaller crystals. The phytosterol blend had fewer, more sporadic nuclei, despite both types of organogels being cooled under the same conditions. The SSAP blend may form gel structure more rapidly than the phytosterol blend upon cooling. Samples that form a gel more rapidly have been shown to result in smaller and more numerous crystals.21 In Fig. 3b, the SSAP blend organogel generally exhibited higher G* values than the phytosterol organogels. This result, along with the smaller crystals, may indicate the SSAP blend organogels have stronger intermolecular interactions. The phytosterol blend may form gel structure slowly and may form larger crystal structures over time.
Another factor that influences crystallization rate is HLB value, as a lower HLB value typically results in a faster crystallization rate.24 The sucrose stearate used had an HLB value of 2, which is likely a contributing factor to the growth of numerous small crystals. The formation of organogels from the SSAP blend showed that there were intermolecular interactions between the organogelators and oil phase.9 Since crystal size, shape, and density all affect the final physicochemical properties, it may be of interest in the future to evaluate the effects of cooling rate and storage temperature on the formation of these organogels to determine the effects on the physicochemical properties.
X-Ray diffraction
Fig. 6 shows the X-ray diffraction patterns of menhaden oil, SL, and all organogels (PM6, PSL6, SM6, and SSL6). All the samples were β′ polymorphs with strong short spacing peaks at 4.20, 3.97, and 3.71 Å. The β′ polymorphic form is characteristic of having small to moderate crystal sizes and a smooth soft texture. However, the samples differed in peak intensity. Differences in peak intensity may correspond to differences in morphology. It has been shown that stronger short spacing peaks correspond to more needle-like morphology.9Fig. 6 shows that the organogels produced with the SL had stronger short spacing peaks (particularly at 4.20 Å) and a needle-like morphology (Fig. 5). On the other hand, organogels produced with menhaden oil had lower intensity short spacing peaks and exhibited spherulitic crystal morphology (Fig. 5). There were two additional higher intensity peaks, at 3.50 and 2.70 Å, likely due to the presence of minor components in the menhaden oil such as small amounts of monoacylglycerols, certain TAG species such as POP, cholesterol, or small amounts of crystalline organogelators.11,25–27
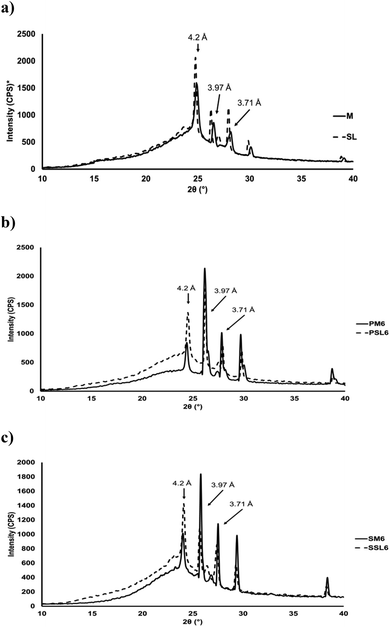 |
| Fig. 6 Crystalline structures of (a) menhaden oil and SL, (b) phytosterol organogels (PM6 and PSL6), and SSAP organogels (SM6 and SSL6). P: phytosterol organogel; S: SSAP organogel; M: menhaden oil; SL: structured lipid, 6: 1 : 1 molar ratio of organogelators, corresponds to gel number from Tables 2 and 3 for phytosterol and SSAP organogels, respectively. *CPS: Counts per second. | |
Solid fat content
Fig. 7 shows the SFC of the phytosterol (PM6 and PSL6) and SSAP organogels (SM6 and SSL6). The phytosterol organogels had SFC values that started around 2% at 0 °C while the SSAP organogels had SFC values that started between 8–11%. The phytosterol had significantly lower SFC values for all temperatures but 35 and 40 °C. PSL6 had significantly higher (p < 0.05) SFC values between 0–10 °C. SSL6 had significantly higher SFC values than SM6 for all temperatures but 35 and 40 °C. The SSAP organogels likely have higher SFC values, particularly at low temperatures, because they contained higher melting stearic and palmitic acids from the sucrose stearate and ascorbyl palmitate, respectively. The organogels formed with the SL had higher SFC values than the organogels formed with menhaden oil. This is likely due to the SL having altered TAG molecular species. During enzymatic modification with ethyl caprate, significant amounts of capric acids were esterified to the sn-1,3 positions, which increased the melting point. Melting point can be correlated to both the saturated fatty acid content and SFC. The SL has a higher melting point and higher amounts of saturated fatty acids, which correlated to having higher SFC values. SFC can also be used to predict firmness/hardness of fats, as a higher SFC typically results in a firmer fat.21 These results align well with the rheological properties and morphology described above. The SSAP organogels had higher SFC values and exhibited a denser gel network of small crystals, which typically indicates a harder fat due to the larger number of crystal–crystal interactions.21 In terms of rheological properties, the SSAP organogels had higher apparent viscosities.
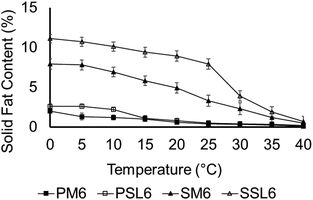 |
| Fig. 7 Solid fat content of phytosterol organogels (PM6 and PSL6), and SSAP organogels (SM6 and SSL6). P: Phytosterol organogel; S: SSAP organogel; M: menhaden oil; SL: structured lipid, 6: 1 : 1 molar ratio of organogelators, corresponds to gel number from Tables 2 and 3 for phytosterol and SSAP organogels, respectively. | |
Oxidative stability
Table 5 shows the OSI values and Fig. 8 shows the changes in TOTOX values of the organogels (PM6, PSL6, SM6, and SSL6), menhaden oil, and SL. The menhaden oil was relatively stable over the 12 days of storage while the TOTOX value of the SL increased more pronouncedly after day 3 (Fig. 8). The OSI values (Table 5) were significantly lower for the SL than the unmodified menhaden oil (p < 0.05). Both the OSI and TOTOX results showed that the SL had lower oxidative stability than the menhaden oil. Though production of SL enzymatically occurs under milder conditions, the reduced oxidative stability is likely due to both the enzymatic reaction process and short-path distillation. Menhaden oil is highly sensitive to oxidation due to the high amounts of polyunsaturated fatty acids. Purification (by removal of free fatty acids or fatty acid ethyl esters) of SL by short-path distillation has been shown in other studies to reduce the tocopherols or antioxidants present in the modified oil.28 Also, the unmodified menhaden oil contained 200 ppm TBHQ and 1000 ppm mixed tocopherols from the manufacturer (Omega Protein Inc., Reedville, VA, USA) which helped improve its oxidative stability.
Table 5 Oil stability index (OSI) values for menhaden oil, SL, phytosterol organogels (PM6 and PSL6), and SSAP organogels (SM6 and SSL6)
Samplea |
Oxidative stability index (OSI)b (h) |
Mean ± STD, n = 3. Different letters in the same column are significantly different. P: Phytosterol organogel; S: SSAP organogel; M: menhaden oil; SL: structured lipid, reaction conditions and relative fatty acid composition as described in Table 1; 6: 1 : 1 molar ratio of organogelators, corresponds to gel number from Tables 2 and 3 for phytosterol and SSAP organogels, respectively. OSI conducted at 80 °C. |
M |
17.55 ± 1.21a |
SL |
5.52 ± 0.29b |
PM6 |
20.88 ± 1.03c |
PSL6 |
18.03 ± 0.08a |
SM6 |
24.92 ± 3.70d |
SSL6 |
23.33 ± 2.06c |
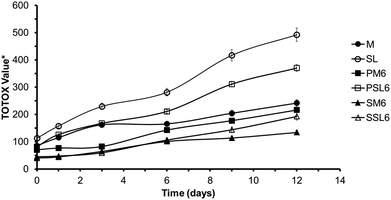 |
| Fig. 8 TOTOX values for menhaden oil, SL, phytosterol organogels (PM6 and PSL6), and SSAP organogels (SM6 and SSL6). P: Phytosterol organogel; S: SSAP organogel; M: menhaden oil; SL: structured lipid, 6: 1 : 1 molar ratio of organogelators, corresponds to gel number from Tables 2 and 3 for phytosterol and SSAP organogels, respectively. *TOTOX value = 2(PV) + pAV, PV = peroxide value, pAV = p-anisidine value. | |
The SSAP organogels exhibited significantly improved (p < 0.05) oxidative stability when compared to the phytosterol organogels and the original oils. The SSAP organogels were relatively stable over the 12 days of storage (Fig. 8) and had significantly higher OSI values (Table 5) than the respective phytosterol organogels and original oils (p < 0.05). This is likely due to the antioxidant contribution of ascorbyl palmitate in the SSAP blend. The ascorbyl palmitate functioned as both an organogelator and antioxidant in this study. The TOTOX values were lower for PSL6 and SSL6 than the original SL for all days indicating PSL6 and SSL6 have a higher oxidative stability than the SL. The OSI values were also higher in the phytosterol organogels than the respective original oils. These results show that the organogels did improve the oxidative stability of the menhaden oil and SL. Therefore, organogelation of SL may be a very good process to improve the oxidative stability of purified SL produced through enzymatic modification.
Conclusions
Both organogels have potential for use as nutraceuticals by utilizing menhaden oil and an MLM-type SL containing health beneficial PUFAs and medium chain fatty acids that provide a quick energy source. Due to their desirable physicochemical properties, these organogels may also find use as low saturated, zero trans-fats in the formulation of margarines, shortenings, baked goods, confections, and other food products. Both organogel networks had a relatively low thermal resistance, though the rheological properties indicated that the SSAP organogel presented higher resistance under shear, likely due to the different morphology and higher SFC values. The differences in physicochemical properties are likely due to the different interactions of the organogelators and oils during the formation of the organogels and may be attributed to the differences in their structures and TAG compositions, respectively.
Abbreviations
CCD | Charge coupled device |
DSC | Differential scanning calorimeter |
FA | Fatty acid |
HLB | Hydrophile-lipophile balance |
M | Menhaden oil |
MLM-type TAG | Medium-long-medium-type triacylglycerol |
MUFA | Monounsaturated fatty acid |
OSI | Oil stability index |
P | Phytosterol organogel |
p-AV |
p-Anisidine value |
PM6 | Phytosterol organogel produced with menhaden oil at organogelator blend 1 : 1 molar ratio |
PSL6 | Phytosterol organogel produced with structured lipid (SL) at organogelator blend 1 : 1 molar ratio |
PUFA | Polyunsaturated fatty acid |
PV | Peroxide value |
S | Sucrose stearate/ascorbyl palmitate (SSAP) organogel |
SFC | Solid fat content |
SL | Structured lipid |
SM6 | Sucrose stearate/ascorbyl palmitate (SSAP) organogel produced with menhaden oil at organogelator blend 1 : 1 molar ratio |
SSAP | Sucrose stearate/ascorbyl palmitate |
SSL6 | Sucrose stearate/ascorbyl palmitate (SSAP) organogel produced with SL at organogelator blend 1 : 1 molar ratio |
TAG | Triacylglycerol |
TOTOX | Total oxidation value |
Conflicts of interest
There are no conflicts of interest to declare.
Acknowledgements
Research was supported in part by Food Science Research, University of Georgia. Special thanks to Omega Protein Inc. for providing the menhaden oil.
References
- K. He, Fish, long-chain omega-3 polyunsaturated fatty acids and prevention of cardiovascular disease- eat fish or take fish oil supplement?, Prog. Cardiovasc. Dis., 2009, 52, 95–114 CrossRef CAS PubMed.
- R. G. Ackman, Characteristics of the fatty acid composition and biochemistry of some fresh-water fish oils and lipids in comparison with marine oils and lipids, Comp. Biochem. Physiol., 1967, 22, 907–922 CAS.
- P. A. Lucca and B. J. Tepper, Fat replacers and the functionality of fat in foods, Trends Food Sci. Technol., 1994, 5, 12–19 CrossRef CAS.
- E. D. Co and A. G. Marangoni, Organogels: an alternative edible oil-structuring method, J. Am. Oil Chem. Soc., 2012, 89, 749–780 CrossRef.
- J. Y. Kim, B. Yi, M. J. Kim and J. H. Lee, Oxidative stability of solid fats containing ethylcellulose determined based on the headspace oxygen content, Food Sci. Biotechnol., 2014, 23, 1779–1784 CrossRef CAS.
- K. F. Chaves, D. Barrera-Arellano and A. P. Badan Ribeiro, Potential application of lipid organogels for food industry, Food Res. Int., 2018, 105, 863–872 CrossRef CAS PubMed.
- M. Lu, Y. Cao, C. T. Ho and Q. Huang, Development of organogel-derived capsaicin nanoemulsion with improved bioaccessibility and reduced gastric mucosa irritation, J. Agric. Food Chem., 2016, 64, 4735–4741 CrossRef CAS PubMed.
- W. M. Cort, Antioxidant activity of tocopherols, ascorbyl palmitate, and ascorbic acid and their mode of action, J. Am. Oil Chem. Soc., 1974, 51, 321 CrossRef CAS.
- L. S. Dassanayake, D. R. Kodali, S. Ueno and K. Sato, Crystallization kinetics of organogels prepared by rice bran wax and vegetable oils, J. Oleo Sci., 2012, 61, 1–9 CrossRef.
- B. H. Kim and C. C. Akoh, Recent research trends on the enzymatic synthesis of structured lipids, J. Food Sci., 2015, 80, 1713–1724 CrossRef.
- S. A. Willett and C. C. Akoh, Application of Taguchi method in the enzymatic modification of menhaden oil to incorporate capric acid, J. Am. Oil Chem. Soc., 2018, 95, 299–311 CrossRef CAS.
- A. Bot and W. G. M. Agterof, Structuring of edible oils by mixtures of γ-oryzanol with β-sitosterol or related phytosterols, J. Am. Oil Chem. Soc., 2006, 83, 513–521 CrossRef CAS.
-
M. Samateh, S. S. Sagiri and G. John, in Edible oleogels: structure and health implications, ed. A. G. Marangoni and N. Garti, AOCS Press, Champaign-Urbana, 2nd edn, 2011, ch. 18, pp. 425–426 Search PubMed.
-
American Oil Chemists’ Society, Official methods and recommended practices of the AOCS, AOCS Press, Champaign-Urbana, 7th edn, 2017 Search PubMed.
- A. Savitzky and M. J. E. Golay, Smoothing and differentiation of data by simplified least squares procedures, Anal. Chem., 1964, 36, 1627–1639 CrossRef CAS.
- J. C. B. Rocha, J. D. Lopes, M. C. N. Mascarenhas, D. B. Arellano, L. M. R. Guerreiro and R. Lopes da Cunha, Thermal and rheological properties of organogels formed by sugarcane or candelilla wax in soybean oil, Food Res. Int., 2013, 50, 318–323 CrossRef CAS.
- S. Da Pieve, S. Calligaris, E. Co, M. C. Nicoli and A. G. Marangoni, Shear nanostructuring of monoglyceride organogels, Food Biophys., 2010, 5, 211–217 CrossRef.
- N. C. Shantha and E. A. Decker, Rapid, sensitive, iron-based spectrophotometric methods for determination of peroxide values of food lipids, J. AOAC Int., 1994, 77, 421–424 CAS.
- S. Murdan, G. Gregoriadis and A. T. Florence, Novel sorbitan monostearate organogels, J. Pharm. Sci., 1999, 88, 608–614 CrossRef CAS PubMed.
- A. Bot, R. D. Adel and E. C. Roijers, Fibrils of γ-oryzanol+β-sitosterol in edible oil organogels, J. Am. Oil Chem. Soc., 2008, 85, 1127–1134 CrossRef CAS.
-
J. M. Aquilera and P. J. Lillford, Food materials science: Principles and practice, Science+Business Media, LLC, New York, 2008 Search PubMed.
- R. Campos, S. S. Narine and A. G. Marangoni, Effect of cooling rate on the structure and mechanical properties of milk fat and lard, Food Res. Int., 2002, 35, 971–981 CrossRef CAS.
- G. Fayaz, S. A. H. Goli and M. Kadivar, A novel propolis wax-based organogel: effect of oil type on its formation, crystal structure and thermal properties, J. Am. Oil Chem. Soc., 2017, 94, 47–55 CrossRef CAS.
- A. Yuki, K. Matsuda and A. Nishimura, Effect of sucrose polyesters on crystallization behavior of vegetable shortening and margarine fat, J. Jpn. Oil Chem. Soc., 1990, 39, 236–244 CrossRef CAS.
- H. Sawalha, P. Venema, A. Bot, E. Floter, R. den Adel and E. van der Linden, The phase behavior of γ-oryzanol and β-sitosterol in edible oil, J. Am. Oil Chem. Soc., 2015, 92, 1651–1659 CrossRef CAS.
- V. D'Souza, J. M. deMan and L. deMan, Short spacings and polymorphic forms of natural and commercial solid fats: a review, J. Am. Oil Chem. Soc., 1990, 67, 835–843 CrossRef.
- M. Takeuchi, S. Ueno and K. Sato, Crystallization kinetics of polymorphic forms of a molecular compound constructed by SOS (1,3-distearoyl-2-oleoyl-sn-glycerol) and SSO (1,2-distearoyl-3-oleoyl-rac-glycerol), Food Res. Int., 2002, 35, 919–926 CrossRef CAS.
- L. Zou and C. C. Akoh, Identification of tocopherols, tocotreinols, and their fatty acid esters in residues and distillates of structured lipids purified by short-path distillation, J. Agric. Food Chem., 2013, 61, 238–246 CrossRef CAS PubMed.
|
This journal is © The Royal Society of Chemistry 2019 |