DOI:
10.1039/C8FO01699B
(Paper)
Food Funct., 2019,
10, 422-431
The protective role of phloretin against dextran sulfate sodium-induced ulcerative colitis in mice
Received
28th August 2018
, Accepted 21st December 2018
First published on 21st December 2018
Abstract
Phloretin, a dihydrogen chalcone flavonoid, is mainly isolated from apples and strawberries. Phloretin has been proven to have many biological activities such as anti-inflammatory and anti-oxidative. Herein, we investigated the protective efficacy and potential mechanism of phloretin in dextran sulfate sodium (DSS)-induced ulcerative colitis (UC) in mice. The results showed that phloretin resulted in a reduced DSS-induced disease activity index (DAI), colon length shortening and colonic pathological damage. The levels of pro-inflammatory cytokines in the colon were also decreased by the administration of phloretin. Exploration of the potential mechanism demonstrated that phloretin suppressed the inflammatory response by regulating the nuclear factor-κB (NF-κB), toll-like receptor 4 (TLR4) and peroxisome proliferator-activated receptor γ (PPARγ) pathways. Phloretin also inhibited the DSS-induced (NOD)-like receptor family and pyrin domain containing 3 (NLRP3) inflammasome activations. Further studies found that phloretin reduced key markers of oxidative stress as well as regulated the expression of zonula occludens-1 (ZO-1) and occludin. Interestingly, the concentration of serum lipopolysaccharide (LPS) was significantly decreased. Escherichia coli (E. coli) and Lactobacillus levels were also re-balanced after phloretin treatment. These results indicate that phloretin might be a new dietary strategy for the treatment of UC.
1. Introduction
Ulcerative colitis (UC) is a common gastrointestinal inflammatory disease that not only affects the quality of life, but also induces colon cancer. Many factors can lead to the occurrence of UC. In terms of immune response, it has been confirmed that the activation of the nuclear factor-κB (NF-κB) and peroxisome proliferator-activated receptor γ (PPARγ) plays an important role in the pathogenesis of UC.1 Our previous study confirmed that NLRP3 deletion has a protective effect on dextran sulfate sodium (DSS)-induced UC in mice.2 In addition, the roles of the gut microbiota and intestinal barrier in maintaining intestinal health and inhibiting UC have also been confirmed.3,4 Despite an understanding of the pathogenesis of UC, there is still no effective treatment.
Currently, the main drugs for treating UC are salicylazosulfapyridine (SASP), immunosuppressive agents and anti-TNF-α monoclonal antibody. However, these drugs have significant side effects and are expensive, especially for long-term use, which limits their use.5 In recent years, the application of functional foods has been highly advocated in the prevention and treatment of diseases.6–8
Phloretin (Fig. 1) is a naturally occurring phytochemical that belongs to the chalcone class of flavonoids. Since phloretin is abundant in apples and strawberries, it has become a part of the human daily diet. Previous studies have reported that phloretin has numerous biological activities such as anti-inflammatory, anti-oxidative and anti-cancer. For example, phloretin suppressed interleukin-1β (IL-1β)-induced pro-inflammatory cytokines in human lung epithelial cells by regulating NF-κB pathway activation.9 Furthermore, Sabzevari et al. reported that phloretin had strong anti-oxidative and anti-inflammatory effects in a rat model of cecal ligation and puncture-induced sepsis.10 A recent study also showed that phloretin was a potent natural TLR2/1 inhibitor to treat TLR2-mediated inflammatory immune diseases.11 More importantly, Lee et al. discovered that phloretin has the ability to alleviate colon inflammation in rats,12 indicating that phloretin may be beneficial for anti-UC. Therefore, we examined the protective effect and explored the potential mechanism of phloretin on DSS-induced UC in mice.
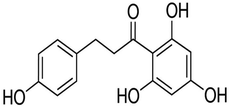 |
| Fig. 1 Chemical structure of phloretin. | |
2. Materials and methods
2.1. Ethics statement
Forty-eight male C57BL/6 mice (6–8 weeks old, weighing 21–23 g) were provided by the Center of Experimental Animals of Jilin University (Jilin, China). The mice received water and food ad libitum and were housed in an air-conditioned room with the laboratory temperature maintained at 24 ± 1 °C. All experimental protocols were in accordance with the approval of the Institutional Animal Care and Use Committee of our university under the approved protocol number SCXXK (JI-2016-0003).
2.2. Induction of colitis and evaluations
Mice were randomly assigned into six equal groups of eight mice each: control group, DSS group, phloretin (25, 50 and 100 mg kg−1) + DSS groups, and phloretin (100 mg kg−1) group. The experimental timelines for the animal model are described in Fig. 2A. In phloretin (25, 50 and 100 mg kg−1) + DSS groups, mice were treated with an indicated dose of phloretin 14 days before DSS treatment via oral gavage once per day. Acute colitis was induced by providing drinking water containing 2.5% (w/v) DSS for 7 days (from day 15 to day 21). In addition, mice were also treated with an indicated dose of phloretin during the DSS treatment for the phloretin groups. Mice in the control group and DSS group were given the same volume of water. In the phloretin (100 mg kg−1) group, mice were administered with phloretin throughout the experiment. The mice weight, stool characteristics and blood in the stool were recorded daily. The disease activity index (DAI) data are presented as an average score of the body weight change, stool consistency and hemoccult bleeding (Table 1).13 After the mice were euthanized, the colon was excised and the length was measured. For histological analysis, the colon specimens were fixed in 10% formalin and embedded in paraffin. Paraffin-embedded sections were deparaffinized with xylene, rehydrated and then stained with haematoxylin and eosin (H&E) according to standard protocols. Histological scoring was conducted according to a previously described method (Table 2).13
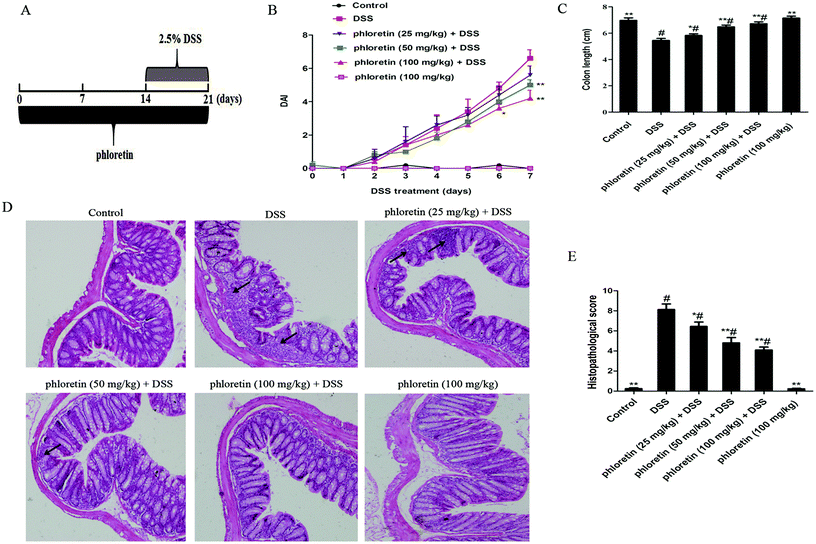 |
| Fig. 2 Phloretin alleviated DSS-induced colitis. (A) The experimental protocol with phloretin in the UC model. (B) DAI score. (C) The colon length. (D) H&E staining, 100×, scale bar, 25 μm. Arrows: inflammatory infiltration. (E) Histopathological scores of each group were determined. Data are presented as means ± SD (n = 8 per group). *p < 0.05 and **p < 0.01 vs. the DSS group; #p < 0.05 vs. the control group. | |
Table 1 Disease activity index (DAI) scoring system
DAI score |
Weight loss (%) |
Stool consistency |
Occult/gross bleeding |
0 |
None |
Normal |
Normal |
1 |
1–5 |
|
|
2 |
5–10 |
Loose stools |
Hemoccult positive |
3 |
10–20 |
|
|
4 |
>20 |
Diarrhea |
Gross bleeding |
Table 2 Histological scoring system
Score |
Inflammation |
Mucosal damage |
Crypt damage |
Range of lesions (%) |
0 |
None |
None |
None |
0 |
1 |
Mild |
Mucous layer |
1/3 |
1–25 |
2 |
Moderate |
Submucosa |
2/3 |
26–50 |
3 |
Severe |
Muscularis and serosa |
100% |
51–75 |
4 |
— |
— |
100% + epithelium loss |
76–100 |
2.3. SOD, GSH, MDA and inflammatory cytokines assay
The colon samples were weighed and homogenized with PBS (w/v, 1
:
9) in ice. Then, the homogenate was centrifuged at 10
000 rpm for 20 min at 4 °C. The supernatant was collected. Superoxide dismutase (SOD), glutathione (GSH) and malondialdehyde (MDA) were examined as per the manufacturer's instruction provided by Nanjing Jiancheng Bioengineering Institute. The levels of the tumor necrosis factor alpha (TNF-α), interleukin-12 (IL-12) and IL-1β were detected by ELISA kits as per the manufacturer's protocol.
2.4. Quantitative real-time polymerase chain reaction (qRT-PCR)
Total RNA was extracted from colon samples using the TRIzol reagent (Invitrogen). The RNA was reverse-transcribed into cDNA using a Revert Aid First Strand cDNA Synthesis Kit (Thermo Scientific). The quantification of relative interleukin-17A (IL-17A), interferon-γ (IFN-γ) and β-actin mRNA was performed by qRT-PCR using a 7500 Fast Real-Time PCR System (Applied Biosystems) and the SYBR Green Plus reagent kit (Roche). The primer sequences are listed in Table 3. β-Actin was the reference gene.
Table 3 Oligonucleotide primers used for qRT-PCR
Name |
Primer sequence |
IL-17A |
Sense: 5′-ACGTTTCTCAGCAAACTTAC-3′ |
Anti-sense: 5′-CCCCTTTACACCTTCTTTTC-3′ |
IFN-γ |
Sense: 5′-GCTCTGAGACAATGAACGCTACAC-3′ |
Anti-sense: 5′-TTCTTCCACATCTATGCCACTTGAG-3′ |
β-Actin |
Sense: 5′-CTACCGTCGTGACTTCGC-3′ |
Anti-sense: 5′-GGGTGACATCTCCCTGTT-3′ |
2.5. Western blot analysis
The colon sample was homogenized. The extracted protein was quantified using the Pierce BCA protein assay kit (23227, Thermo) and transferred onto the polyvinylidene difluoride (PVDF) membrane. The PVDF membrane was then blocked with 5% nonfat milk for 2 h at room temperature. Afterwards, the PVDF membrane was incubated at 4 °C overnight with primary antibodies against p65 (MW, 65 kDa), p-p65 (65 kDa), IκB (39 kDa), p-IκB (40 kDa), TLR4 (110 kDa), PPARγ (53 kDa), NLRP3 (110 kDa), ASC (22 kDa), Caspase-1 (20 kDa), IL-1β (17 kDa), ZO-1 (191 kDa), occludin (59 kDa) and β-actin (42 kDa). Next, the secondary antibodies were incubated at room temperature for 2 h. The protein bands were visualized using a western blot detection program.
2.6. Determination of LPS
Serum lipopolysaccharide (LPS) contents were analyzed using the Limulus amebocyte lysate (LAL) assay kit as per the manufacturer's protocol. Briefly, serum was diluted 1
:
10 in pyrogen-free water and then inactivated at 70 °C for 10 min. Afterwards, the serum was incubated with a LAL solution at 37 °C for 30 min. Finally, the reagents were added to form a magenta derivative that absorbed at 545 nm.
2.7. Detection of E. coli and Lactobacillus
Individual mice were previously marked to allow tracking throughout the experiment. Fecal pellets were collected, weighed and then homogenized with 1 mL sterile PBS on day 21. The supernatant was serially diluted and plated on MRS medium and MacConkey medium. MRS plates were incubated anaerobically for 2–3 days at 37 °C. MacConkey plates were incubated aerobically overnight at 37 °C. Typical colonies were counted for plates containing 30–300 colonies.
2.8. Statistical analysis
Results were expressed as mean ± standard deviation (SD). DAI was assessed with the unpaired two tailed Student's t-test. Multiple comparisons were evaluated using one-way ANOVA followed by Tukey's multiple-comparison test. P < 0.05 was considered significant.
3. Results
3.1. Phloretin alleviated DSS-induced colitis
It is well known that the DAI score is an important indicator for assessing the severity of colitis. In the present study, we found a significant increase in the DAI score after DSS treatment compared to those of the control group. However, treatment with phloretin significantly decreased the DSS-induced DAI score compared to those of the DSS group (Fig. 2B). As an indicator of the indirect reflection of colonic inflammation, we also measured the length of the colon. The results showed that phloretin significantly inhibited DSS-induced colon shortening (Fig. 2C). From histopathological observations, we found that there were massive epithelial destruction, crypt abscesses and loss of goblet cells in the DSS group. However, these histopathological changes were improved by phloretin treatment. Only phloretin (100 mg kg−1) did not generate a histopathological change (Fig. 2D and E).
3.2. Inhibition of pro-inflammatory cytokines
The results are shown in Fig. 3A. TNF-α, IL-1β and IL-12 levels in the DSS group were significantly higher than those in the control group. However, phloretin significantly inhibited a DSS-induced increase of the three cytokines. In addition, we also detected IL-17A and IFN-γ mRNA levels by qRT-PCR. Consistent with the above results, phloretin also significantly inhibited the DSS-induced increase in IL-17A and IFN-γ gene levels (Fig. 3B).
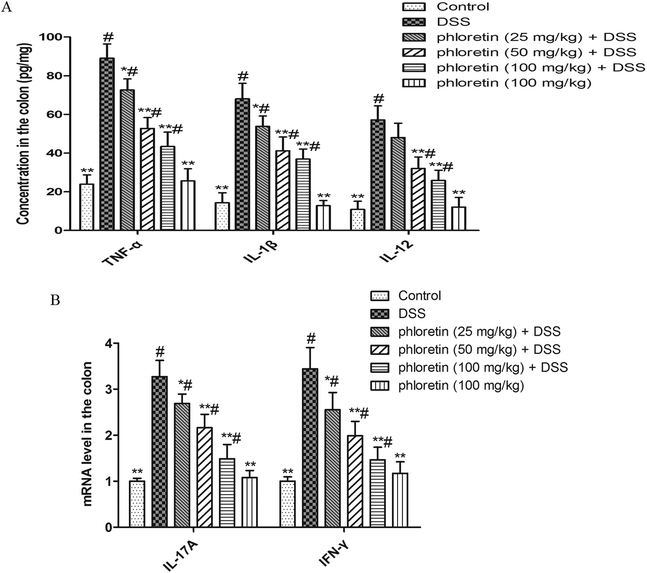 |
| Fig. 3 Inhibition of pro-inflammatory cytokines. (A) TNF-α, IL-1β and IL-12 levels in colon tissues were measured by ELISA. (B) IL-17A and IFN-γ mRNA levels in colon tissues were measured by qRT-PCR. Data are presented as means ± SD (n = 8). *p < 0.05 and **p < 0.01 vs. the DSS group; #p < 0.05 vs. the control group. | |
3.3. Suppression of NF-κB pathway activation
To investigate the inhibitory mechanism of pro-inflammatory cytokines, we examined the key proteins in the NF-κB pathway. As shown in Fig. 4, the NF-κB pathway was significantly activated in the DSS group. However, treatment with phloretin significantly inhibited NF-κB pathway activation by decreasing the phosphorylation of NF-κB p65 and IκB.
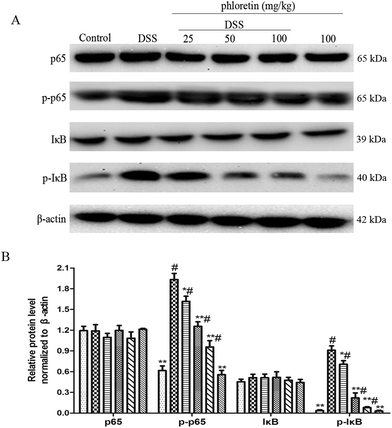 |
| Fig. 4 Suppression of NF-κB pathway activation. (A) NF-κB p65 and IκB protein levels in colon tissues. (B) Phosphorylation of NF-κB p65 and IκB in colon tissues was normalized to β-actin. Data are presented as means ± SD (n = 3). *p < 0.05 and **p < 0.01 vs. the DSS group; #p < 0.05 vs. the control group. | |
3.4. Regulation of the expression of TLR4 and PPARγ
TLR4 and PPARγ are two upstream pathways of NF-κB. In the present study, we found that phloretin had a role in regulating the NF-κB pathway. To further investigate the anti-UC mechanism of phloretin, we examined the expression of TLR4 and PPARγ. The results showed that TLR4 expression was significantly increased after DSS treatment, but PPARγ expression was significantly decreased compared to those of the control group. Treatment with phloretin significantly regulated TLR4 and PPARγ levels in a dose-dependent manner (Fig. 5).
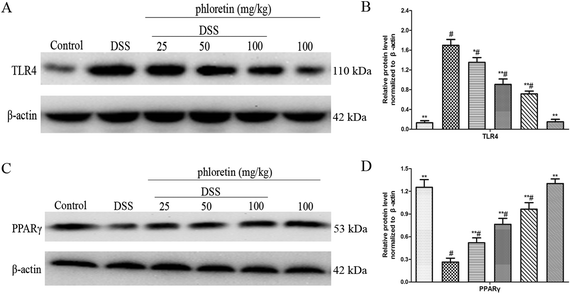 |
| Fig. 5 Regulation of the expression of TLR4 and PPARγ. (A) Protein level of TLR4 in colon tissues. (B) The expression of TLR4 in colon tissues was normalized to β-actin. (C) Protein level of PPARγ in colon tissues. (D) The expression of PPARγ in colon tissues was normalized to β-actin. Data are presented as means ± SD (n = 3). *p < 0.05 and **p < 0.01 vs. the DSS group; #p < 0.05 vs. the control group. | |
3.5. Inhibition of NLRP3 inflammasome activation
We also evaluated the role of phloretin in NLRP3 inflammasome activation. The results showed that the expression of NLRP3, ASC, Caspase-1 and IL-1β was significantly increased after DSS intake compared to control group. However, phloretin was capable of regulating these changes (Fig. 6).
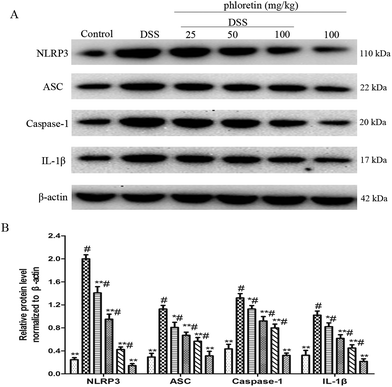 |
| Fig. 6 Inhibition of NLRP3 inflammasome activation. (A) The expression of NLRP3, ASC, caspase-1 and IL-1β in colon tissues. (B) The expression of NLRP3, ASC, caspase-1 and IL-1β in colon tissues was normalized to β-actin. Data are presented as means ± SD (n = 3). *p < 0.05 and **p < 0.01 vs. the DSS group; #p < 0.05 vs. the control group. | |
3.6. Improvement of oxidative stress
To further assess whether phloretin had an anti-oxidative effect in UC mice, we examined three markers of oxidative stress. As shown in Fig. 7, DSS-induced colitis caused an increase in the level of MDA when compared to the control group. On the contrary, mice in the DSS group exhibited a tendency to inhibit SOD and GSH activities. After phloretin treatment, all three markers were significantly regulated.
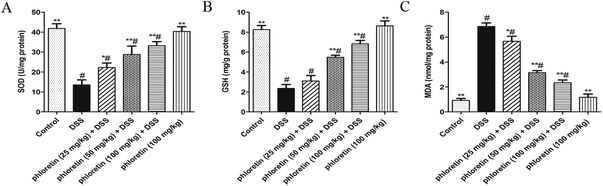 |
| Fig. 7 Improvement of oxidative stress. Samples were obtained from the colonic homogenate of the experimental mice. (A) SOD activity change. (B) GSH activity change. (C) MDA production. Data are presented as means ± SD (n = 8). *p < 0.05 and **p < 0.01 vs. the DSS group; #p < 0.05 vs. the control group. | |
3.7. Increase of the expression of ZO-1 and occludin
The epithelial tight junction (TJ) protein plays an important role in ensuring a relatively stable internal environment in the intestine and reducing inflammation levels. Because phloretin improved colonic histopathological changes and inhibited oxidative stress, we speculated that phloretin may have a regulatory effect on DSS-induced TJ molecules. Thus, we detected the intestinal TJ proteins (ZO-1 and occludin). As expected, DSS-induced colitis resulted in a significant reduction in the expression of ZO-1 and occludin compared to the control group. However, phloretin (25, 50 and 100 mg kg−1) significantly increased DSS-induced levels of ZO-1 and occludin (Fig. 8).
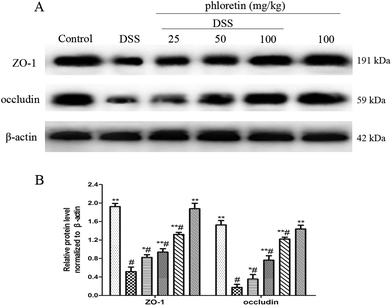 |
| Fig. 8 Increase of the expression of ZO-1 and occludin. (A) The expression of ZO-1 and occludin in colon tissues. (B) The expression of ZO-1 and occludin in colon tissues was normalized to β-actin. Data are presented as means ± SD (n = 3). *p < 0.05 and **p < 0.01 vs. the DSS group; #p < 0.05 vs. the control group. | |
3.8. Decrease of serum LPS content, and re-balance of E. coli and Lactobacillus
As shown in Fig. 9A, the concentration of LPS in the DSS group was significantly increased compared to those in the control group. However, the oral administration of phloretin inhibited the serum LPS level in a dose-dependent manner. In addition, we detected representative bacteria (E. coli and Lactobacillus) in mice feces. The result showed that the commensal E. coli level was significantly increased in DSS-induced UC mice. However, the level of Lactobacillus was significantly decreased after DSS treatment compared to those in the control group. After administration of phloretin, the number of E. coli and Lactobacillus in the feces was re-balanced compared to that in the DSS group (Fig. 9B and C).
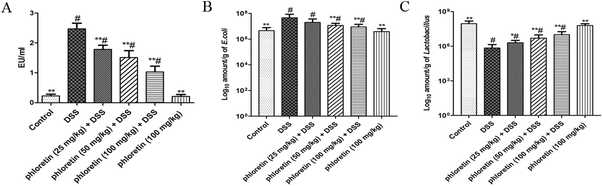 |
| Fig. 9 Decrease of serum LPS content and re-balance of E. coli and Lactobacillus. (A) Serum LPS concentration. (B) E. coli and (C) Lactobacillus counts. Data are presented as means ± SD (n = 8). *p < 0.05 and **p < 0.01 vs. the DSS group; #p < 0.05 vs. the control group. | |
4. Discussion
Ulcerative colitis (UC) is a complicated gastrointestinal inflammatory disease that seriously affects the quality of life and increases the risk of colon cancer.14 There are still no effective and suitable drugs available to treat UC. Phloretin is a component of functional foods mainly found in apples and strawberries. Increasing studies have showed that phloretin has many biological activities such as anti-inflammatory and anti-oxidative.9,10 However, direct evidence for the effect of phloretin on DSS-induced UC in mice has not yet been reported. Herein, we assessed the effect of phloretin on UC and explored the potential mechanism. As a direct and indirect indicator of the severity of UC, we found that phloretin could significantly reduce DSS-induced DAI scores and inhibit colon shortening. More importantly, DSS-induced colonic pathological damage was also significantly improved after phloretin administration. Lee et al. reported that phloretin ameliorated trinitrobenzene sulfonic acid (TNBS)-induced colon inflammation and body weight loss in rats.12 It is well known that inflammatory bowel diseases include UC and Crohn's disease. The DSS-induced colitis represents a well-established model that could be used to study the pathogenesis of UC and is similar to human UC.15,16 However, TNBS-induced colitis resembles Crohn's disease in humans.17,18 Therefore, our results are consistent with this finding, but there were some notable differences.
Although the precise etiology of UC is complex and remains uncertain, many studies have shown that the NF-κB pathway plays a key pathogenic role.19 NF-κB is a key signaling pathway in regulating inflammation. Activation of NF-κB promotes the secretion of pro-inflammatory cytokines, leading to inflammatory responses. TNF-α is the earliest endogenous mediator in the inflammatory process. It can promote the proliferation and differentiation of T cells, destroy the intestinal barrier and accelerate the formation of intestinal inflammation.20 IL-1β is essential in the early stages of inflammation and can mediate persistent inflammatory responses in the intestine.21 IL-12 promotes the secretion of TNF-α by regulating natural killer cells and T cells, thereby further mediating inflammatory responses.22 Our previous study also indicated that TNF-α, IL-1β and IL-12 levels were significantly increased in DSS-induced colitis in mice, whereas the inhibition of three cytokine secretions had a protective effect on colitis.23 The levels of pro-inflammatory cytokines (TNF-α, IL-1β and IL-12) in our study were higher in the DSS group than in the control group. However, DSS-induced highly expressed pro-inflammatory cytokines were significantly inhibited by phloretin. Furthermore, IL-17A and IFN-γ levels were also detected. IL-17 is produced by highly differentiated Th17 cells and is an effective mediator of inflammation.24 IFN-γ is mainly secreted by Th1 cells and is able to increase mucosal permeability by impairing TJ structure. An increasing number of studies have shown that cytokines from Th1 and Th17 patterns were closely associated with the pathology of UC.25,26 IL-17A and IFN-γ levels in our study were higher in the DSS group than in the control group. In addition, it has been reported that Th1 and Th17 cells promote the activation of NF-κB pathway, leading to the aggravation of intestinal inflammation.27,28 In the present study, phloretin significantly reduced DSS-induced IL-17A and IFN-γ levels. Meanwhile, the NF-κB pathway was also significantly inhibited by phloretin. A previous study indicated that phloretin has the ability to inhibit LPS-stimulated activation of the NF-κB pathway in dendritic cells.29 Thus, our results suggest that phloretin might decrease the secretion of cytokines by suppressing NF-κB activation, thereby exerting an anti-UC effect.
TLR4 is an important inflammatory pathway and is highly expressed in the intestine. As an upstream pathway of NF-κB, the activation of TLR4 plays an important role in the etiology of UC. Increasing studies have reported the up-regulation of TLR4 in intestinal tissue of patients with IBD.30 In our previous study, DSS-induced activation of TLR4 was also observed.23 NF-κB and MAPK are two major downstream pathways of TLR4. A previous study indicated that phloretin alleviated LPS-induced acute lung injury in mice via regulation of NF-κB and MAPK pathways.31 In addition, LPS is an agonist of TLR4. The above reports indicate that phloretin might play an anti-UC role by regulating TLR4. Indeed, DSS-induced TLR4 activation in our study was significantly inhibited after phloretin treatment in a dose-dependent manner. However, our results are still preliminary and further verification is needed.
PPARγ, a member of the nuclear receptor superfamily, is an important anti-inflammatory mediator. PPARγ is also an important upstream pathway of NF-κB and the activation of this pathway has been reported to protect mice from UC.32,33 To further study the inhibition mechanism of NF-κB, we investigated the effect of phloretin on PPARγ. Contrary to the results for TLR4, the PPARγ level was significantly decreased after DSS intake. However, we found that phloretin inhibited the DSS-induced decrease in PPARγ expression. A recent article also reported that phloretin regulated the PPARγ level,34 which is consistent with our results. Our results suggest that the role of phloretin in exerting anti-UC might be related to its regulation of PPARγ expression.
In the present study, we found that phloretin had a significant inhibitory effect on the DSS-induced increase in IL-1β. Unlike other cytokines, bioactive IL-1β secretion relies on inflammasome activation. The NLRP3 inflammasome is a molecular platform and its activation combines with ASC to induce the activation of pro-caspase-1, which leads to the maturation and release of IL-1β.35 Although NLRP3 has been shown to be involved in the pathogenesis of UC, its specific role in the pathogenesis of UC is still debatable. Bauer et al. showed decreased sensitivity to DSS in NLRP3-deficient mice.36 However, Allen et al. reported that mice lacking NLRP3 were more likely to induce colitis.37 In a previous study, we found that mice deficient of NLRP3 have a protective effect on DSS-induced UC.2 In the present study, NLRP3 inflammasome was significantly activated in DSS-induced UC mice. Meanwhile, phloretin inhibited the activation of NLRP3 inflammasome induced by DSS. This result is consistent with the result of our previous study, indicating that the beneficial role of phloretin in DSS-induced UC might be attributed to its suppression of NLRP3 inflammasome activation.
Targeted inflammation currently has become a well-known therapeutic strategy for UC. However, excessive inflammation augments oxidative stress during the development of UC by stimulating reactive oxygen/nitrogen species generating systems. Previous studies have also shown that excessive oxygen radicals are important factors in tissue damage and UC formation.38 In the present study, we examined three main markers of oxidative stress. The results showed that DSS-induced colitis caused a significant increase in the MDA level. On the contrary, SOD and GSH activities were significantly inhibited after DSS treatment. Administration of phloretin significantly regulated all three markers, thereby inhibiting oxidative stress. Intestinal epithelium is an important barrier to maintain intestinal health and inhibit intestinal inflammation. The abnormal structure of colonic TJ proteins, including the down-regulation of ZO-1 and occludin, is one of the important factors leading to the destruction of the intestinal barrier in IBD patients.39 TJ proteins are currently used as a target for the novel therapeutic treatment of IBD. Oxidative stress is an important factor in intestinal tissue damage. Because phloretin inhibits colonic oxidative stress and improves histopathological changes, we speculated that phloretin could also regulate TJ molecules during DSS-induced UC. To this end, major TJ proteins, including ZO-1 and occludin were analyzed. As expected, the expression of ZO-1 and occludin was significantly decreased in DSS-induced UC mice. However, such change was improved by phloretin treatments, indicating that the improvement of the intestinal barrier may be one of the mechanisms by which phloretin exerts anti-UC. In addition, it was reported that the increased permeability of the intestinal mucosa promoted the passage of foreign substances such as LPS through the intestinal mucosa, which resulted in promoting inflammation.40,41 In the present study, we found that DSS-induced mice showed a significant increase in the serum LPS level. However, the oral administration of phloretin inhibited this change. As we all know, LPS is a component of Gram-negative bacteria. The increased permeability of intestinal mucosa and disturbance of the intestinal microflora are responsible for the increase in serum LPS. In our study, the intestinal barrier was destroyed and the serum LPS level was significantly increased in DSS-induced UC mice, suggesting that the balance between the intestinal barrier and intestinal microflora may be destroyed. E. coli and Lactobacillus are two representative bacteria in the intestine. Studies have confirmed that these bacteria are closely related to the occurrence of UC.42,43 Indeed, the DSS-induced E. coli level was significantly increased while the Lactobacillus level was significantly reduced in our study. Surprisingly, phloretin has the effect of rebalancing E. coli and Lactobacillus.
In conclusion, our study explores a new dietary strategy for UC. Phloretin treatment alleviates DSS-induced UC outcomes. The protection may depend not only on its anti-inflammatory and anti-oxidative effects, but also on its regulation of intestinal bacteria and epithelium barrier. Therefore, phloretin may be a useful dietary natural product with anti-UC benefits.
Conflicts of interest
The authors declare no conflict of interest.
Acknowledgements
This study was supported by the Key Project of Chinese National Programs for Research and Development (no. 2016YFD0501009) and the National Natural Science Foundation of China (no. 31472248 and 31572582).
References
- K. Celinski, T. Dworzanski, A. Korolczuk, R. Piasecki, M. Slomka, A. Madro and R. Fornal, Effects of peroxisome proliferator-activated receptors-gamma ligands on dextran sodium sulphate-induced colitis in rats, J. Physiol. Pharmacol., 2011, 62, 347–356 CAS.
- Z. Zhang, P. Shen, X. Lu, Y. Li, J. Liu, B. Liu, Y. Fu, Y. Cao and N. Zhang, In Vivo and In Vitro Study on the Efficacy of Terpinen-4-ol in Dextran Sulfate Sodium-Induced Mice Experimental Colitis, Front. Immunol., 2017, 8, 558 CrossRef PubMed.
- M. A. McGuckin, R. Eri, L. A. Simms, T. H. Florin and G. Radford-Smith, Intestinal barrier dysfunction in inflammatory bowel diseases, Inflammatory Bowel Dis., 2009, 15, 100–113 CrossRef PubMed.
- T. Ohkusa and S. Koido, Intestinal microbiota and ulcerative colitis, J. Infect. Chemother., 2015, 21, 761–768 CrossRef PubMed.
- K. Matsuoka, E. Saito, T. Fujii, K. Takenaka, M. Kimura, M. Nagahori, K. Ohtsuka and M. Watanabe, Tacrolimus for the Treatment of Ulcerative Colitis, Intest. Res., 2015, 13, 219–226 CrossRef PubMed.
- Y. Mine and H. Zhang, Anti-inflammatory Effects of Poly-L-lysine in Intestinal Mucosal System Mediated by Calcium-Sensing Receptor Activation, J. Agric. Food Chem., 2015, 63, 10437–10447 CrossRef CAS PubMed.
- Z. Zhang, P. Shen, J. Liu, C. Gu, X. Lu, Y. Li, Y. Cao, B. Liu, Y. Fu and N. Zhang, In Vivo Study of the Efficacy of the Essential Oil of Zanthoxylum bungeanum Pericarp in Dextran Sulfate Sodium-Induced Murine Experimental Colitis, J. Agric. Food Chem., 2017, 65, 3311–3319 CrossRef CAS PubMed.
- T. Fukuda, K. Majumder, H. Zhang, P. V. Turner, T. Matsui and Y. Mine, Adenine Inhibits TNF-alpha Signaling in Intestinal Epithelial Cells and Reduces Mucosal Inflammation in a Dextran Sodium Sulfate-Induced Colitis Mouse Model, J. Agric. Food Chem., 2016, 64, 4227–4234 CrossRef CAS PubMed.
- W. C. Huang, S. J. Wu, R. S. Tu, Y. R. Lai and C. J. Liou, Phloretin inhibits interleukin-1 beta-induced COX-2 and ICAM-1 expression through inhibition of MAPK, Akt, and NF-kappa B signaling in human lung epithelial cells, Food Funct., 2015, 6, 1960–1967 RSC.
- M. Aliomrani, M. R. Sepand, H. R. Mirzaei, A. R. Kazemi, S. Nekonam and O. Sabzevari, Effects of phloretin on oxidative and inflammatory reaction in rat model of cecal ligation and puncture induced sepsis, Daru, J. Pharm. Sci., 2016, 24, 15 CrossRef PubMed.
- J. Kim, P. Durai, D. Jeon, I. D. Jung, S. J. Lee, Y. M. Park and Y. Kim, Phloretin as a Potent Natural TLR2/1 Inhibitor Suppresses TLR2-Induced Inflammation, Nutrients, 2018, 10, E868 CrossRef PubMed.
- J. H. Lee, S. C. Regmi, J. A. Kim, M. H. Cho, H. Yun, C. S. Lee and J. Lee, Apple Flavonoid Phloretin Inhibits Escherichia coli O157:H7 Biofilm Formation and Ameliorates Colon Inflammation in Rats, Infect. Immun., 2011, 79, 4819–4827 CrossRef CAS PubMed.
- N. Kihara, S. G. de la Fuente, K. Fujino, T. Takahashi, T. N. Pappas and C. R. Mantyh, Vanilloid receptor-1 containing primary sensory neurones mediate dextran sulphate sodium induced colitis in rats, Gut, 2003, 52, 713–719 CrossRef CAS PubMed.
- J. Terzic, S. Grivennikov, E. Karin and M. Karin, Inflammation and Colon Cancer, Gastroenterology, 2010, 138, 2101–U2119 CrossRef CAS PubMed.
- V. Valatas, G. Bamias and G. Kolios, Experimental colitis models: Insights into the pathogenesis of inflammatory bowel disease and translational issues, Eur. J. Pharmacol., 2015, 759, 253–264 CrossRef CAS PubMed.
- C. O. Elson, R. B. Sartor, G. S. Tennyson and R. H. Riddell, Experimental models of inflammatory bowel disease, Gastroenterology, 1995, 109, 1344–1367 CrossRef CAS.
- C. O. Elson, K. W. Beagley, A. T. Sharmanov, K. Fujihashi, H. Kiyono, G. S. Tennyson, Y. Cong, C. A. Black, B. W. Ridwan and J. R. McGhee, Hapten-induced model of murine inflammatory bowel disease: mucosa immune responses and protection by tolerance, J. Immunol., 1996, 157, 2174–2185 CAS.
- C. van Montfrans, R. J. Bennink, K. de Bruin, W. de Jonge, H. J. Verberne, F. J. Ten Kate, S. J. van Deventer and A. A. Te Velde, In vivo evaluation of 111In-labeled T-lymphocyte homing in experimental colitis, J. Nucl. Med., 2004, 45, 1759–1765 CAS.
- E. Ardite, J. Panes, M. Miranda, A. Salas, J. I. Elizalde, M. Sans, Y. Arce, J. M. Bordas, J. C. Fernandez-Checa and J. M. Pique, Effects of steroid treatment on activation of nuclear factor kappaB in patients with inflammatory bowel disease, Br. J. Pharmacol., 1998, 124, 431–433 CrossRef CAS PubMed.
- T. Billiet, P. Rutgeerts, M. Ferrante, G. Van Assche and S. Vermeire, Targeting TNF-alpha for the treatment of inflammatory bowel disease, Expert Opin. Biol. Ther., 2014, 14, 75–101 CrossRef CAS PubMed.
- J. K. Yamamoto-Furusho, J. J. Santiago-Hernandez, N. Perez-Hernandez, S. Ramirez-Fuentes, J. M. Fragoso and G. Vargas-Alarcon, Interleukin 1 beta (IL-1B) and IL-1 antagonist receptor (IL-1RN) gene polymorphisms are associated with the genetic susceptibility and steroid dependence in patients with ulcerative colitis, J. Clin. Gastroenterol., 2011, 45, 531–535 CrossRef CAS PubMed.
- X. M. Wang, Y. Lu, L. Y. Wu, S. G. Yu, B. X. Zhao, H. Y. Hu, H. G. Wu, C. H. Bao, H. R. Liu, J. H. Wang, Y. Yao, X. G. Hua, H. Y. Guo and L. R. Shen, Moxibustion inhibits interleukin-12 and tumor necrosis factor alpha and modulates intestinal flora in rat with ulcerative colitis, World J. Gastroenterol., 2012, 18, 6819–6828 CrossRef CAS PubMed.
- Z. Zhang, J. Liu, P. Shen, Y. Cao, X. Lu, X. Gao, Y. Fu, B. Liu and N. Zhang, Zanthoxylum bungeanum pericarp extract prevents dextran sulfate sodium-induced experimental colitis in mice via the regulation of TLR4 and TLR4-related signaling pathways, Int. Immunopharmacol., 2016, 41, 127–135 CrossRef CAS PubMed.
- L. Rovedatti, T. Kudo, P. Biancheri, M. Sarra, C. H. Knowles, D. S. Rampton, G. R. Corazza, G. Monteleone, A. Di Sabatino and T. T. MacDonald, Differential regulation of interleukin 17 and interferon gamma production in inflammatory bowel disease, Gut, 2009, 58, 1629–1636 CrossRef CAS PubMed.
- P. Alex, N. C. Zachos, T. Nguyen, L. Gonzales, T. E. Chen, L. S. Conklin, M. Centola and X. Li, Distinct cytokine patterns identified from multiplex profiles of murine DSS and TNBS-induced colitis, Inflammatory Bowel Dis., 2009, 15, 341–352 CrossRef PubMed.
- Y. Yan, V. Kolachala, G. Dalmasso, H. Nguyen, H. Laroui, S. V. Sitaraman and D. Merlin, Temporal and spatial analysis of clinical and molecular parameters in dextran sodium sulfate induced colitis, PLoS One, 2009, 4, e6073 CrossRef PubMed.
- G. Muzes, B. Molnar, Z. Tulassay and F. Sipos, Changes of the cytokine profile in inflammatory bowel diseases, World J. Gastroenterol., 2012, 18, 5848–5861 CrossRef CAS PubMed.
- F. Zhou, Z. Zhu, J. Gao, C. Yang, L. Wen, L. Liu, X. Zuo, X. Zheng, Y. Shi, C. Zhu, B. Liang, X. Yin, W. Wang, H. Cheng, S. Shen, X. Tang, H. Tang, L. Sun, A. Zhang, S. Yang, X. Zhang and Y. Sheng, NFKB1 mediates Th1/Th17 activation in the pathogenesis of psoriasis, Cell. Immunol., 2018, 331, 16–21 CrossRef CAS PubMed.
- C. C. Lin, C. L. Chu, C. S. Ng, C. Y. Lin, D. Y. Chen, I. H. Pan and K. J. Huang, Immunomodulation of phloretin by impairing dendritic cell activation and function, Food Funct., 2014, 5, 997–1006 RSC.
- L. Chen, M. J. Lin, L. L. Zhan and X. P. Lv, Analysis of TLR4 and TLR2 polymorphisms in inflammatory bowel disease in a Guangxi Zhuang population, World J. Gastroenterol., 2012, 18, 6856–6860 CrossRef CAS PubMed.
- W. C. Huang, C. L. Lai, Y. T. Liang, H. C. Hung, H. C. Liu and C. J. Liou, Phloretin attenuates LPS-induced acute lung injury in mice via modulation of the NF-kappaB and MAPK pathways, Int. Immunopharmacol., 2016, 40, 98–105 CrossRef CAS PubMed.
- G. Pedersen and J. Brynskov, Topical rosiglitazone treatment improves ulcerative colitis by restoring peroxisome proliferator-activated receptor-gamma activity, Am. J. Gastroenterol., 2010, 105, 1595–1603 CrossRef CAS PubMed.
- M. Zhang, C. Deng, J. Zheng, J. Xia and D. Sheng, Curcumin inhibits trinitrobenzene sulphonic acid-induced colitis in rats by activation of peroxisome proliferator-activated receptor gamma, Int. Immunopharmacol., 2006, 6, 1233–1242 CrossRef CAS PubMed.
- A. Takeno, I. Kanazawa, M. Notsu, K. I. Tanaka and T. Sugimoto, Phloretin Promotes Adipogenesis via Mitogen-Activated Protein Kinase Pathways in Mouse Marrow Stromal ST2 Cells, Int. J. Mol. Sci., 2018, 19, E1772 CrossRef PubMed.
- A. Lu, V. G. Magupalli, J. Ruan, Q. Yin, M. K. Atianand, M. R. Vos, G. F. Schroder, K. A. Fitzgerald, H. Wu and E. H. Egelman, Unified polymerization mechanism for the assembly of ASC-dependent inflammasomes, Cell, 2014, 156, 1193–1206 CrossRef CAS PubMed.
- C. Bauer, P. Duewell, C. Mayer, H. A. Lehr, K. A. Fitzgerald, M. Dauer, J. Tschopp, S. Endres, E. Latz and M. Schnurr, Colitis induced in mice with dextran sulfate sodium (DSS) is mediated by the NLRP3 inflammasome, Gut, 2010, 59, 1192–1199 CrossRef CAS PubMed.
- I. C. Allen, E. M. TeKippe, R. M. T. Woodford, J. M. Uronis, E. K. Holl, A. B. Rogers, H. H. Herfarth, C. Jobin and J. P. Y. Ting, The NLRP3 inflammasome functions as a negative regulator of tumorigenesis during colitis-associated cancer, J. Exp. Med., 2010, 207, 1045–1056 CrossRef CAS PubMed.
- H. Zhu and Y. R. Li, Oxidative stress and redox signaling mechanisms of inflammatory bowel disease: updated experimental and clinical evidence, Exp. Biol. Med., 2012, 237, 474–480 CrossRef CAS PubMed.
- T. Kucharzik, S. V. Walsh, J. Chen, C. A. Parkos and A. Nusrat, Neutrophil transmigration in inflammatory bowel disease is associated with differential expression of epithelial intercellular junction proteins, Am. J. Pathol., 2001, 159, 2001–2009 CrossRef CAS PubMed.
- T. Suzuki, Regulation of intestinal epithelial permeability by tight junctions, Cell. Mol. Life Sci., 2013, 70, 631–659 CrossRef CAS PubMed.
- J. J. Jeong, K. A. Kim, S. E. Jang, J. Y. Woo, M. J. Han and D. H. Kim, Orally administrated Lactobacillus pentosus var. plantarum C29 ameliorates age-dependent colitis by inhibiting the nuclear factor-kappa B signaling pathway via the regulation of lipopolysaccharide production by gut microbiota, PLoS One, 2015, 10, e0116533 CrossRef PubMed.
- K. Matsuoka and T. Kanai, The gut microbiota and inflammatory bowel disease, Semin. Immunopathol., 2015, 37, 47–55 CrossRef CAS PubMed.
- L. B. Vong, T. Yoshitomi, K. Morikawa, S. Saito, H. Matsui and Y. Nagasaki, Oral nanotherapeutics: effect of redox nanoparticle on microflora in mice with dextran sodium sulfate-induced colitis, J. Gastroenterol., 2014, 49, 806–813 CrossRef CAS PubMed.
|
This journal is © The Royal Society of Chemistry 2019 |