DOI:
10.1039/C8FO01453A
(Paper)
Food Funct., 2019,
10, 397-409
The ameliorative effect of a Lactobacillus strain with good adhesion ability against dextran sulfate sodium-induced murine colitis†
Received
20th July 2018
, Accepted 23rd November 2018
First published on 3rd December 2018
Abstract
The objective of this study was to effectively screen out a Lactobacillus strain with excellent adhesion ability and ameliorative effect on the disease symptoms of a murine ulcerative colitis model. The auto-aggregation rate (RAA), co-aggregation rate with Escherichia coli O157 (RCA), and cell surface hydrophobicity (AHC) of 17 Lactobacillus strains were measured for primary selection. The results indicate that Lactobacillus plantarum AR326 displayed the topmost overall aggregation performance among all strains examined. A positive and linear relationship between RCA and RAA values was observed for 15 Lactobacillus strains with RAA = 16–46% and RCA = 15–27%. For adhesion ability to human adenocarcinoma HT-29 cells, five representative Lactobacillus strains showed a good, positive dependence on RAA, where L. plantarum AR326 and L. fermentum AR184 showed a higher adhesion ability than the others. Using the 5 (6)-carboxyfluorescein diacetate N-succinimidyl ester (cFDA SE)-labeling technique, L. plantarum AR326 was confirmed to adhere to and colonize well in the intestinal mucosa of mice mainly in the ileum and colon. Finally, L. plantarum AR326 at the dosage applied (daily 2 × 109 cfu per mouse) could attenuate murine dextran sulfate sodium (DSS)-induced colitis by effectively decreasing the body weight loss, disease activity index, colon length shortening, myeloperoxidase activity, and colon epithelial damage of experimental animals. The protective effects involved the restoration of the tight junction protein expression and reduction of the abnormal expression of pro-inflammatory cytokines. In conclusion, L. plantarum AR326 can be used as promising probiotics to ameliorate DSS-induced colitis.
1. Introduction
Some Lactobacillus strains have been used in food products as probiotics due to their beneficial effects on human health.1–4 The functionality of probiotic Lactobacillus strains has been reported such as restoring the balance of the gastro-intestinal microbiota, reducing intestinal inflammation, and helping to manage inflammatory bowel disease (IBD).5–7
Inflammatory bowel disease (IBD) is a chronic relapsing disorder that encompasses a range of intestinal pathologies, e.g. the most common ulcerative colitis and Crohn's disease.4,8 For in vivo animal studies, the dextran sulfate sodium (DSS)-induced ulcerative colitis model has been widely used as a representative IBD model, because of its simplicity and similarities to human IBD.8 Several studies have been conducted using the strategy of oral administration with probiotic lactobacilli to prevent or ameliorate ulcerative colitis in vivo. Three Lactobacillus plantarum strains (21, K68, and CLP-0611) have been reported to ameliorate experimental colitis in mice or rats to a good effect.9–11L. plantarum NCIMB8826 can reduce the weight loss and colon length shortening in DSS-induced colitis mice, without significant impact on reducing disease activity index (DAI) and histological damage.12 Two L. fermentum strains (BR11 and CCTCC M206110) are proved to be effective for alleviating DSS-induced colitis in mice or rats.12,13 However, L. rhamnosus attenuated insignificantly the severity of DSS-induced colitis, in comparison with Bifidobacterium breve that attenuated significantly.14 Exceptionally, L. crispatus CCTCC M206119 appeared to aggravate DSS-induced colitis in BALB/c mice.12
Generally, different Lactobacillus strains may exert positive or opposite effects on the disease severity of ulcerative colitis in vivo. This diversity is strain-specific and closely related to several key factors, especially the prerequisite one, the adhesion ability of Lactobacillus strains in the gut.15,16 The other factors include the dosage, survival rate, and immunomodulatory or anti-inflammatory activities of the Lactobacillus strains applied.
For saving the cost of screening the strains with good probiotic potential in vivo, three adhesion-related abilities in vitro are necessarily considered together: firstly, the adhesion ability to the intestinal epithelial cells that could be mimicked by human adenocarcinoma cell lines such as HT-29 or Caco-2;17–19 secondly, the auto-aggregation ability that is potentially and positively correlated with lactobacilli adhesion and colonization in the intestinal tract;19–22 and thirdly, the co-aggregation ability between lactobacilli and pathogens (e.g. Escherichia coli), which has been shown to be a competitively excluding activity against pathogen adhesion to the gut.23,24 The role of the adhesion ability of the Lactobacillus strains to the intestinal epithelial cells in ameliorating the colitis-associated symptoms of animals has not yet been discovered in the literature. Discovering Lactobacillus strains with high adhesion ability and excellent colitis-ameliorating potential in vivo is crucial for the probiotic therapeutic industry and still under investigation.
Accordingly, in this study, several Lactobacillus strains with excellent adhesion abilities to HT-29 cells were screened from 17 Lactobacillus strains with characterized auto-aggregation rates, co-aggregation rates, and hydrophobicity. One Lactobacillus strain was specially selected for studying its colonization and distribution in the mouse intestine and its ameliorative effects on the disease severity of mice with DSS-induced colitis. The colonization and distribution of the selected Lactobacillus strain in the mouse intestine were monitored with the 5 (6)-carboxyfluorescein diacetate N-succinimidyl ester (cFDA SE)-labeling technique.25
2. Materials and methods
2.1 Bacterial strains, cells, animals and culture conditions
Bacterial strains were isolated from fermented pickles and dairy products. The identification of these isolates was based on the sequences of the 16S rRNA gene. To amplify the 16S rRNA gene, the universal primers 27F (5′-AGAGT TTGATCCTGGCTCA-3′) and 1492R (5′-GGTTACCTTGTTACGACTT-3′) were used. The 16s rRNA gene sequence was queried against the NCBI genetic database using the BLAST search algorithm. The Mega software package, version 7.0, was used for the phylogenetic tree construction based on the neighbor-joining method. Sixteen strains were identified as Lactobacillus species. These isolated Lactobacillus strains, Lactobacillus rhamnosus GG (LGG) (Jiangnan University, Wuxi, Shanghai) and Escherichia coli O157 (provided by Professor Qing Liu, University of Shanghai for Science and Technology, Shanghai) were stored in Shanghai Engineering Research Center of Food Microbiology (Shanghai, China). All Lactobacillus strains were anaerobically cultured in de Man, Rogosa and Sharpe (MRS) broth at 37 °C for 20 h before centrifugation (8000g for 3 min) for the following experiments. Escherichia coli O157 was aerobically cultured in brain heart infusion broth (BHIB) at 37 °C for 18 h before centrifugation for the co-aggregation experiments.
The human adenocarcinoma cell line HT29 was purchased from the Cell Resources Center of Shanghai Institutes for Biological Sciences (Shanghai, China). HT29 cells were cultivated in 1640 medium (Gibco™, Thermo Fisher Scientific, Grand Island, USA) containing 10% fetal bovine serum at 37 °C under 5% CO2. The passage number was 4 for all experiments performed. The culture medium was refreshed every second day.
Female ICR mice were purchased from Shanghai SLRC Laboratory Animal Co. Ltd (Shanghai, China). The mice were housed in an air-conditioned room with the laboratory temperature maintained at 23 ± 2 °C and a relative humidity of 50 ± 10%. All mice were housed for acclimatization for at least one week before the following experiments, and the procedures were performed according to institutional and governmental regulations about the use of experimental animals. All animal procedures were performed in accordance with the Guidelines for Care and Use of Laboratory Animals of Shanghai Jiao Tong University and approved by the Animal Ethics Committee of Shanghai Jiao Tong University.
2.2 Detection of Lactobacilli auto-aggregation
An auto-aggregation assay was carried out as described previously with modification.26 A bacterial pellet was washed with phosphate buffered saline (PBS) twice and resuspended in PBS to an initial absorbance (A0) of 0.25 ± 0.05 at 600 nm. The bacterial suspension was maintained in PBS at 37 °C for 5 h in a thermostatic incubator and then measured for absorbance at 600 nm (noted as At). The measurement was conducted in triplicate. The auto-aggregation rate of Lactobacilli (RAA) was then calculated using eqn (1). | RAA = [1 − (At/A0)] × 100% | (1) |
where A0 and At are the absorbances of Lactobacilli suspensions at a staying time of 0 and t h, respectively.
2.3 Detection of Lactobacilli co-aggregation
A co-aggregation assay for lactobacilli and E. coli O157 was carried out according to Collado et al.26 with modification. Lactobacilli and E. coli suspensions were gently mixed in an isovolumetric manner. The mixed bacterial suspension was maintained at 37 °C for 5 h and its absorbance examined at 600 nm. The measurement was conducted in triplicate. The co-aggregation rate (RCA) was calculated using eqn (2). | RCA = {[(Alacto + AE. coli)/2 − Amix]/(Alacto + AE. coli)/2} × 100% | (2) |
where Alacto and AE. coli are the initial absorbances of lactobacilli and E. coli suspensions, respectively, and Amix is the absorbance of the lactobacilli–E. coli mixed suspension after maintaining for 5 h.
2.4 Cell surface hydrophobicity measurement
Cell surface hydrophobicity was determined as the affinity to hydrocarbons, using the xylene extraction method according to Collado et al.26 Xylene (3 mL, Sinopharm Chemical Reagent Co., Ltd, Shanghai, China) was added into a test tube containing 3 mL Lactobacillus suspension (A0 = 0.25 ± 0.05). The mixture was well mixed for 3 min by vortex oscillation, followed by 1 h of incubation at room temperature before absorbance measurement at 600 nm. The measurement was conducted in triplicate. The strain surface hydrophobicity (AHC) was calculated according to eqn (3). | AHC = [(A0 − A)/A0] × 100% | (3) |
where A0 is the initial absorbance of the Lactobacillus suspension at a holding time of 0 h, and A is the absorbance of the aqueous phase of the Lactobacillus–xylene suspension after 1 h of incubation at room temperature.
2.5
Lactobacillus adhesion experiment on HT29 cells
The concentration of the Lactobacillus suspension was adjusted to 108 cfu mL−1. HT29 cells were cultured on 12-well plates. When they grew to cover 80%–90% of the cell culture plates, they were rinsed with PBS twice and added to 1 mL of Lactobacillus suspension and 1 mL of 1640 culture medium per well. Then, they were placed in a cell incubator at 37 °C for 2 h, followed by successive PBS rinsing twice, methanol fixation for 20 min, PBS rinsing twice, Gram staining, and microscopic observation. Twenty views were randomly chosen for shooting. The number of lactobacilli adhered to HT29 cells was calculated on the basis of 100 cells. The experiment was repeated in triplicate.
2.6 Animal experiment
2.6.1 Fluorescent labelling of L. plantarum AR326.
A fluorescent probe was prepared as described in the previous research.25 The concentration of the Lactobacillus suspension was adjusted to 1010 cfu mL−1. The bacterial suspension was then mixed with cFDA-SE (50 μM in ethanol) in an isovolumetric manner, followed by incubation in a 37 °C water bath for 20 min in the dark. The cell mass was collected by centrifugation at 8000g for 3 min and rinsed with PBS twice to remove unreacted cFDA SE. Flow cytometry (FACSCalibur, Becton, Dickinson and Company, New Jersey, USA) was used to detect cell fluorescent labelling percentage. The percentage of fluorescent-labelled positive alive cells was 99.74%.
2.6.2 Fluorescent labelling of L. plantarum AR326.
Female ICR mice at 7 weeks of age were divided into two groups. In the experimental group, the mice were orally administered with fluorescent-labelled L. plantarum AR326 once at a dosage of 2 × 109 cfu in 0.2 mL. For the control group, the mice were orally administered with sterilized PBS solution (0.2 mL). On the 1st, 2nd, 3rd, 4th, 5th, and 6th day post administration, five mice in each group were sacrificed by cervical dislocation. The duodenum, jejunum, ileum and colon were taken and further split vertically to remove the large particles inside. Then 150 μL cm−1 PBS was used to rinse repeatedly. The bacteria in the washing liquid were collected and fixed with 0.75% oxymethylene for bacteria number analysis with flow cytometry.25 Data were measured in five replications.
2.6.3 Mice with DSS-induced colitis and L. plantarum intervention.
Female ICR mice at 7 weeks of age were divided into three groups: normal control, DSS-induced colitis, and L. plantarum intervention groups. The treatment schedule is depicted in Fig. 1. The normal group took a normal diet and drank water freely for the whole experimental period (14 days). The DDS-induced colitis group was fed with 3% DSS-containing drinking water by drinking freely for the first 5 days and then with a normal diet and water for the next 7 days of the experiment. In the L. plantarum intervention group, the above DSS-treated mice were orally administered with 2 × 109 cfu of L. plantarum AR326 in 0.2 mL by oral gavage daily for the next 7 days of the experiment. At the end of the experiments, the mice were sacrificed by cervical dislocation for the following histological examinations.
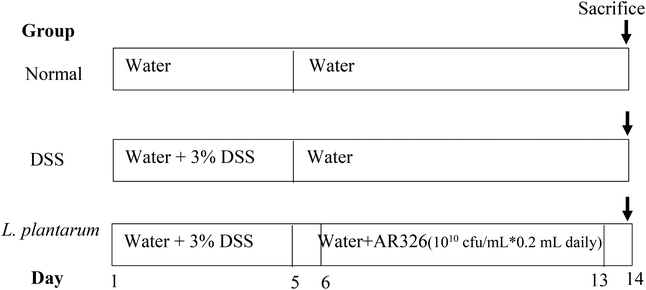 |
| Fig. 1 Feeding schedules for normal, DSS-induced colitis, and L. plantarum intervention models using ICR mice. | |
The disease activity index (DAI) is a clinical parameter reflecting the severity of weight loss, stool consistency and faecal bleeding of experimental animals.27 Accordingly, the severity of the DSS-induced disease was determined by calculating the body weight change, disease activity index (DAI), colon length, and myeloperoxidase (MPO) activity.14 Body weight change was evaluated as a score: 0, normal, without change; 1, 1–5% weight loss; 2, 6–10% weight loss; 3, 11–15% weight loss; and 4, >15% weight loss. DAI was determined from the following eqn (4).
| DAI = (body weight change + stool consistency + faecal bleeding)/3 | (4) |
where stool consistency was evaluated as a score: 0 = normal; 2 = soft with normal form; and 4 = loss of form/diarrhea. Faecal bleeding was evaluated as a score: 0 = no blood; 2 = detectable blood with the colorectal test kit (Baso Diagnostics Inc., Zhuhai, China); and 4 = visible blood without test. Colon length was measured based on the fact that DSS-induced colitis in mice can be characterized by colonic shortening.
8 MPO activity was measured using the MPO assay kit according to the manufacturer's protocol (Nanjing Jiancheng Bioengineering Institute, Nanjing, China). MPO activity was measured in units per g of fresh colon tissue, where one unit of MPO was defined as the amount needed to degrade 1.0 μmol of hydrogen peroxide per minute at 37 °C.
Colonic histology was assessed by H&E staining. The colon tissues were fixed in 10% neutral buffered formalin for 24 h and embedded in paraffin. Sections of 6 μm for each sample were stained with hematoxylin and eosin (H&E) for light microscopic evaluation. The samples were observed at ×100 magnification. The severity of colonic histological injury was scored using a scoring system taking into account the severity of inflammation (from 0 to 3 as the maximum score), crypt damage (from 0 to 5 as the maximum score) and ulcerations (from 0 to 3 as the maximum score).28 Values were added to give a maximal histological score of 11.28 All data were examined in five replications.
2.7 Quantitative real-time PCR
The total cellular RNA was extracted using TRIzol reagent (Sangon Biotech, Shanghai, China) according to the manufacturer's instructions. Quantitative real-time PCR amplification was performed using 2xSYBR® Premix Ex Taq (Takara Bio (Dalian) Co., Ltd, Dalian, China) to detect MUC2, E-cadherin 1, zonula occludens-1 (ZO-1), claudin 3, TNF-α, IL-1β, IL-6, and β-actin gene expression. The results were analysed by the method of 2−ΔΔCt. The PCR primer pairs used in the study are shown in Table S1.†
2.8 Statistical analysis
Significant differences among data were examined with the one-way ANOVA program and Duncan's test of Statistical Product and Service Solutions (SPSS Inc., Chicago, USA). The significance of the correlation coefficient was also examined. Data are presented as the means with standard deviations and differ significantly as presented with different letters at P < 0.05. SigmaPlot 12.3 software (Systat Software Inc., CA, USA) was used for plotting and obtaining linear relationships.
3. Results
3.1 Auto-aggregation, co-aggregation and hydrophobicity
The sixteen isolated strains were identified to the species level (Table 1). They belong to the Lactobacillus species. Fig. 2 shows the phylogenetic tree based on the 16S rRNA gene sequences showing the relationships of the sixteen isolated Lactobacillus species and LGG. Lactobacillus brevis, Lactobacillus fermentum and L. plantarum are grouped within a single branch, separately. The position of Lactobacillus casei and Lactobacillus paracasei in the phylogenetic tree reflects their close evolutionary relationship. The strains, even in the same species, are separated well in the phylogenetic tree. These indicated that these strains are different and probably have different properties.
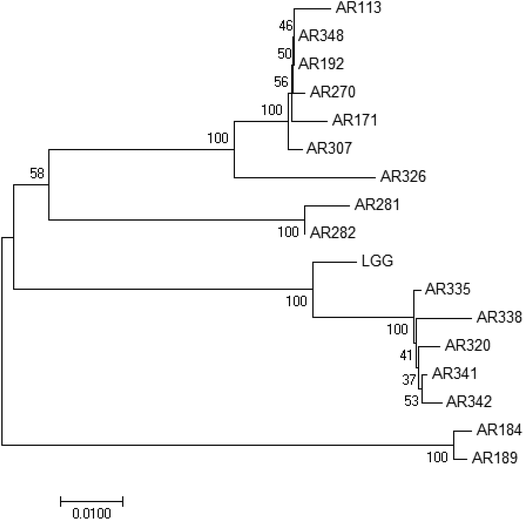 |
| Fig. 2 Phylogenetic tree based on the 16S rRNA gene sequences showing the relationships of these strains. | |
Table 1 Auto-aggregation rates, co-aggregation rates, and hydrophobicity of 17 Lactobacillus strains examined
Strain |
Auto-aggregation rate, RAA (%) |
Co-aggregation rate, RCA (%) |
Hydrophobicity, AHC (%) |
Data are presented as means ± standard deviations (n = 3). Data followed with different capital letters differed significantly (P < 0.05).
|
L. brevis AR281 |
41.7 ± 0.2A a |
27.5 ± 1.1A |
24.6 ± 1.3D |
L. brevis AR282 |
24.6 ± 3.8CD |
22.0 ± 6.2ABCDE |
90.7 ± 2.7A |
L. casei AR341 |
23.8 ± 1.6CD |
18.1 ± 4.3DE |
81.1 ± 2.2A |
L. casei AR342 |
13.7 ± 5.5E |
8.6 ± 0.9F |
88.6 ± 5.5A |
L. paracasei AR320 |
16.4 ± 5.4DE |
15.1 ± 2.2E |
81.8 ± 5.4A |
L. paracasei AR335 |
20.7 ± 4.5DE |
19.0 ± 4.1CDE |
83.1 ± 2.1A |
L. paracasei AR338 |
21.5 ± 4.5DE |
6.1 ± 1.1F |
83.9 ± 2.9A |
L. fermentum AR184 |
42.4 ± 1.0A |
23.5 ± 2.3ABCD |
86.8 ± 4.7A |
L. fermentum AR189 |
17.5 ± 1.3DE |
19.3 ± 4.1CDE |
83.6 ± 5.9A |
L. plantarum AR113 |
38.3 ± 0.6AB |
23.0 ± 1.1ABCD |
81.3 ± 3.2A |
L. plantarum AR171 |
23.0 ± 3.2CD |
19.9 ± 1.1BCDE |
89.0 ± 6.1A |
L. plantarum AR192 |
22.6 ± 4.1CD |
18.9 ± 1.1CDE |
85.1 ± 7.0A |
L. plantarum AR270 |
24.5 ± 5.1CD |
20.3 ± 2.4BCDE |
83.5 ± 2.3A |
L. plantarum AR307 |
41.9 ± 1.1A |
26.5 ± 0.2AB |
45.0 ± 3.5C |
L. plantarum AR326 |
45.5 ± 1.4A |
25.4 ± 1.8ABC |
15.0 ± 0.9D |
L. plantarum AR348 |
20.8 ± 4.1DE |
16.5 ± 2.4DE |
89.1 ± 5.5A |
L. rhamnosus GG (LGG) |
30.5 ± 0.5BC |
23.3 ± 1.4ABCD |
66.2 ± 3.5B |
The auto-aggregation rate (RAA), co-aggregation rate (RCA), and hydrophobicity (AHC) of 17 strains of the lactobacilli examined were measured, including LGG as the positive control. The results are tabulated in Table 1. Generally, the obtained RAA values ranged from 13.7% (L. casei AR342) to 45.5% (L. plantarum AR326). The five strains that showed an RAA value significantly (P < 0.05) higher than that of LGG (30.5%) included L. brevis AR 281, L. fermentum AR184, and L. plantarum AR 113, AR307, and AR326. The RCA values ranged from 6.1% to 27.5%; the highest values were for L. brevis AR281 and L. plantarum AR307 and AR326 which were greater than that for LGG (23.3%). The AHC values ranged from 15.0% (L. plantarum AR326) to 90.7% (L. brevis AR282). Most of the strains, except L. brevis AR281 and L. plantarum AR307 and AR326, showed an average AHC value of 81–91%, significantly (P < 0.05) greater than that of LGG (66.2%) and hardly correlating to their RAA or RCA values. Generally, the RAA, RCA, and AHC values were partly strain-specific. Both aggregation rates for the strains from the L. plantarum group (RAA = 21–46%; RCA = 17–27%) were greater than those from the L. casei group (including L. paracasei) (RAA = 14–24%; RCA = 6–19%).
Fig. 3A illustrates that, for most of the Lactobacillus examined except L. casei AR342 and L. paracasei AR338 with extraordinarily low RCA values, the obtained RCA value appeared to linearly and positively correlate with the RAA value, following the equation RCA = 0.321 × RAA + 11.9 (R2 = 0.811, P < 0.05). The seven L. plantarum strains detected were also committed to a similar relationship (RCA = 0.325 × RAA + 11.5, R2 = 0.895, P < 0.05; equation not shown). For affinity to hydrocarbons (AHC) as an index for cell surface hydrophobicity (Fig. 3B), the AHC values varied with RAA in a roughly negative way for all strains (AHC = −1.63 × RAA + 119, R2 = 0.527, P < 0.05). It is interesting to note that those strains with RAA > 30% could be grouped into high-AHC (L. fermentum AR184 and L. plantarum AR113) and low-AHC (L. brevis AR281 and L. plantarum AR307 and AR326) groups, implying distinct cell surface hydrophobicity or compositions.
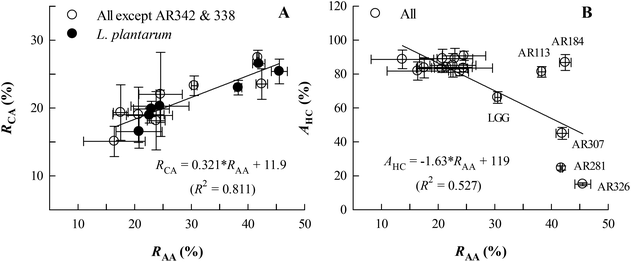 |
| Fig. 3 Changes in the co-aggregation rate (RCA) (A) and affinity to hydrocarbons (AHC, an index for hydrophobicity) (B) with the auto-aggregation rate (RAA) for the Lactobacillus strains studied. AR342 = L. casei AR342; AR338 = L. paracasei AR338. Data are presented as means ± standard deviations (n = 3) for two variables correlated. | |
3.2 Adhesion of selected Lactobacillus strains onto HT29 cells
From different species, three strains with high RAA and RCA values (L. brevis AR281, L. fermentum AR184, and L. plantarum AR326) and one strain with a low RAA or RCA (L. casei AR342) were selected for studying adhesion to HT-29 cells, in reference to LGG as the positive control. The results are given in Table 2. It is shown that the cell adhesion abilities were in the range of 171–430 bacterial counts per 100 cells, significantly (P < 0.05) greatest for L. plantarum AR326 and L. fermentum AR184, followed by L. brevis AR281 and LGG, and the least for L. casei AR342. Interestingly, the adhesion abilities of these five lactobacilli were closely and positively correlated with RAA (AA = 8.17 × RAA + 38.5, R2 = 0.909, P < 0.05, Fig. 4A), rather than with RCA, and negatively correlated with AHC, except for L. fermentum AR184 (AA = −3.24 × AHC + 456, R2 = 0.969, P < 0.05, Fig. 4B). Obviously, the strain with a high RAA value exhibited high adhesion ability to HT-29 cells, implying high adhesion potential to the intestinal epithelial cells.
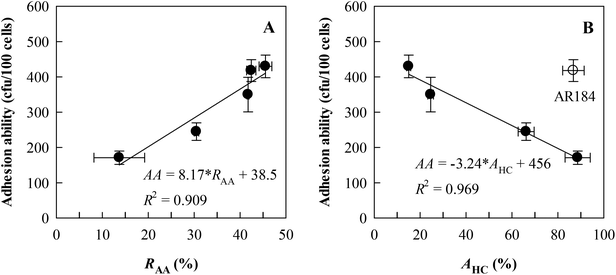 |
| Fig. 4 Relationships between adhesion ability to auto-aggregation rates (RAA) (A) and affinity to hydrocarbons (AHC) (B) for five representative Lactobacillus strains studied as shown in Table 2, including L. brevis AR281, L. casei AR342, L. fermentum AR184, L. plantarum AR326, and L. rhamnosus GG (LGG). In the equations, AA = adhesion ability. Data are presented as means ± standard deviations (n = 3) for two variables correlated. | |
Table 2 Adhesion abilities of 5 selected Lactobacillus strains onto HT29 cells
Strain |
Adhesion ability (bacterial count per 100 cells) |
Data are presented as means ± standard deviations (n = 3). Data followed with different capital letters differed significantly (P < 0.05).
|
L. brevis AR281 |
350 ± 49B a |
L. casei AR342 |
171 ± 19D |
L. fermentum AR184 |
418 ± 31A |
L. plantarum AR326 |
430 ± 32A |
L. rhamnosus GG (LGG) |
245 ± 25C |
From the above in vitro experiments, L. plantarum AR326 had the best overall performance, i.e., maximal auto-aggregation, co-aggregation with E. coli, and adhesion ability to HT-29 cells. Accordingly, it was selected for the following in vivo experiments.
3.3 Colonization and distribution of L. plantarum AR326 in the mouse intestines
The number changes of cFDA SE-labelled L. plantarum AR326 distributed in different intestinal parts of the mice after oral administration once at a dosage of 2 × 109 cfu in 0.2 mL are depicted in Fig. 5, with respect to the post administration day. It is indicated that L. plantarum AR326 survived and colonized very well inside mouse intestines. On day 1 after oral administration, the detected level of positive L. plantarum AR326 was high up to 7.80 × 104 in the ileum, followed by 4.45 × 104 in the colon, and ∼2 × 103 in the jejunum and duodenum. This suggests that the AR326 colonized well in the ileum and colon. The bacterial count reduced slowly with the day post oral administration and still remained at 2 × 104 in the ileum and colon on the sixth day post administration. Generally, the colonization potential of L. plantarum AR326 was in the order of ileum > colon > jejunum and duodenum.
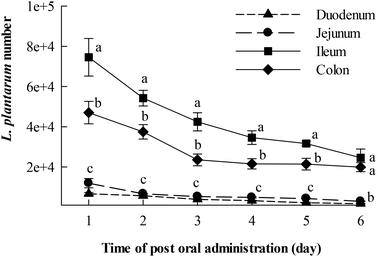 |
| Fig. 5 Changes in the fluorescence-labelled L. plantarum AR326 number adhered on various sections of the intestinal tract in ICR mice with respect to time after oral administration (lactobacilli dosage = 1010 CFU mL−1 in 0.2 mL on day 1). Data are represented as means ± standard deviations (n = 5). Data indicated with different letters differ significantly (P < 0.05). | |
3.4 Intervention of L. plantarum AR326 on mice with DDS-induced colitis
The intervention effects of orally administered L. plantarum AR326 on the severity of DDS-induced colitis in mice were evaluated from the viewpoint of body weight loss, disease activity index (DAI), colon length, myeloperoxidase (MPO) activity, and histopathology, where the DAI value was the average score of body weight loss, stool consistency, and faecal bleeding scores for the experimental animals according to Tao et al.27 Mice were fed according to the schedule in Fig. 1.
Fig. 6A depicts the body weight changes of normal, DSS-treated, and DSS-treated plus L. plantarum administered mice with respect to feeding time. In contrast to the normal group, the body weight of the DSS-treated mice decreased significantly after the 6th day (i.e. post DSS treatment), maximized on the 9th day, and recovered gradually thereafter. With L. plantarum intervention, the DSS-treated mice showed body weight recovery partly and significantly after the 8th day until the end of the experiment. Clearly, L. plantarum intervention at the dosage applied could effectively reduce the body weight loss of DSS-treated mice. For the DAI scores of mice where the normal DAI = 0–0.25 (Fig. 6B), the DAI score of DSS-treated mice increased linearly and remarkably with the feeding time during the first 9 days, maximized at 3.1, and decreased gradually thereafter. With L. plantarum intervention, the DAI decreased on the 6th day (i.e. the first day of L. plantarum administration) and maintained the reduced DAI score during the rest of experimental time, in contrast to the positive control. The final DAI score of the L. plantarum intervention group on the 14th day was less than 1.0, approaching the normal one. This suggests that oral administration with L. plantarum AR326 at the dosage applied did reduce the DAI score of ICR mice with DSS-induced colitis.
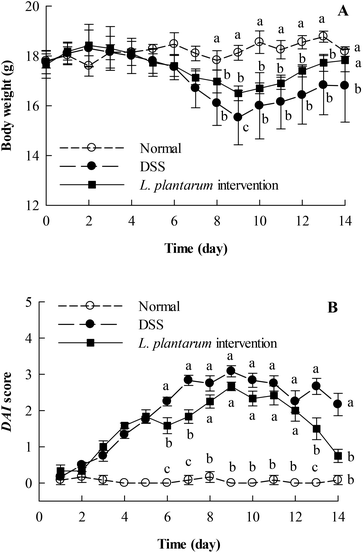 |
| Fig. 6 Effects of L. plantarum AR326 intervention on the body weight (A) and disease activity index (DAI) score (B) of ICR mice with DSS-induced colitis (lactobacilli dosage = 2 × 109 CFU per mice). Data are represented as means ± standard deviations (n = 5). Data indicated with different letters differ significantly (P < 0.05). | |
Colonic shortening is well known as one of the characteristics of DSS-induced colitis in mice.8Fig. 7A shows that the DSS group exhibited a significant colon length reduction (4.8 ± 0.2 cm) at the end of the experiment (14th day), in contrast to the normal one (6.3 ± 0.2 cm) (P < 0.05). Promisingly, the L. plantarum intervention group displayed a colon length of 5.83 ± 0.49 cm, significantly (P < 0.05) longer than the DSS counterpart and resembled the normal one. Fig. 7B depicted that the MPO activity, a marker of polymorphonuclear neutrophil primary granules,29 increased significantly (P < 0.05) in the DSS group (0.60 U g−1) by an increment almost double that of the normal one (0.22 U g−1). In contrast to the DSS group, the L. plantarum intervention group showed a significantly (P < 0.05) decreased MPO activity. Evidently, the intervention of L. plantarum AR326 at the dosage applied could recover the DSS-damaged colon length to a normal level and alleviate the DSS-induced hyper-activated MPO activity of the DSS group to a significantly decreased level. The decreased MPO activity implies the reduced number and distribution of neutrophils in the tissues, i.e. decreased neutrophil infiltration and inflammatory symptoms.29Fig. 8 shows the pathological examination of the colons with H&E staining. Epithelial erosion, disappearance of crypts, and cellular infiltration were observed in the DSS group. The evaluation of colons from the L. plantarum intervention group revealed a significant reduction in epithelial erosion and inflammatory cellular infiltration compared to the DSS group and the epithelium morphology resembled that observed in samples from the normal group (Fig. 8A). The score value measured for DSS-treated mice was significantly higher than that of the normal group (Fig. 8B). In contrast, the score value of the L. plantarum intervention group was lower than that of the DSS group (Fig. 8B). These further confirmed that L. plantarum AR326 can attenuate DSS-induced colitis.
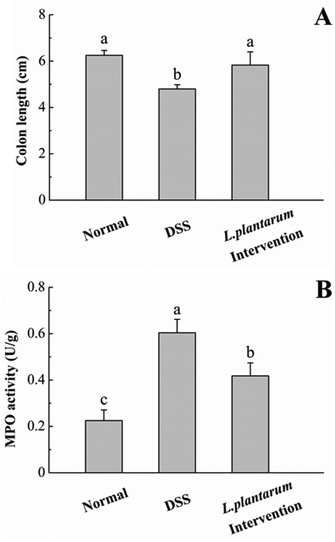 |
| Fig. 7 Effects of L. plantarum AR326 intervention on the colon length (A) and myeloperoxidase (MPO) activity (B) of ICR mice with DSS-induced colitis (lactobacilli dosage = 2 × 109 CFU per mice). Data are represented as means ± standard deviations (n = 5). Means with different letters differ significantly (P < 0.05). | |
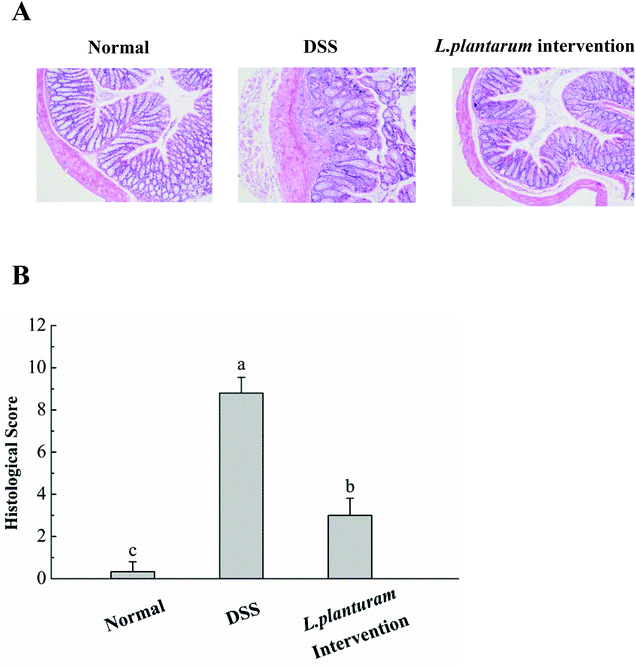 |
| Fig. 8 Effects of L. plantarum AR326 intervention on the colon histology. (A) The representative section of the colons was determined by H&E staining (magnification 100×). (B) Associated histological scores. Data are represented as means ± standard deviations (n = 5). Means with different letters differ significantly (P < 0.05). | |
3.5 Intervention of L. plantarum AR326 on the expression of the tight junction proteins and inflammatory cytokines
DSS administration could induce changes in the expression of tight junction proteins and increased the expression of pro-inflammatory cytokines.5,10 To determine whether the intervention of L. plantarum AR326 could maintain the integrity of the intestinal epithelial barrier, we examined the expression of tight junction proteins, including MUC2, ZO-1, claudin 3 and E-cadherin 1 using quantitative real-time PCR. Compared to the normal group, the DSS group showed significant reductions in these genes (P < 0.05, Fig. 9). In contrast to the DSS group, the L. plantarum intervention group could recover the expression of these tight junction proteins to a normal level (Fig. 9).
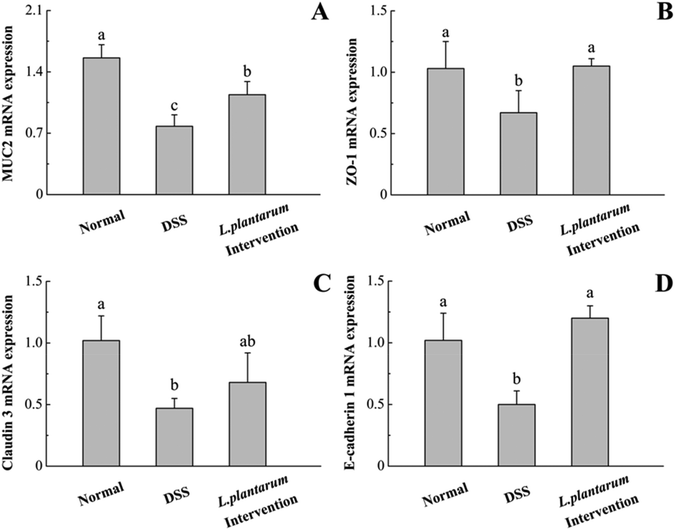 |
| Fig. 9 The mRNA levels of MUC2 (A), ZO-1 (B), claudin 3 (C) and E-cadherin 1 (D) were determined using quantitative real-time PCR in the colon. Data are represented as means ± standard deviations (n = 5). Means with different letters differ significantly (P < 0.05). | |
Previous studies have demonstrated that the pro-inflammatory cytokines TNF-α, IL-1β and IL-6 are associated with the severity of colitis.5,10,30 Accordingly, we evaluated the mRNA expression of colonic TNF-α, IL-1β, and IL-6 using quantitative real-time PCR. In the DSS group, the levels of these pro-inflammatory cytokines were significantly higher than that of the normal group (P < 0.05, Fig. 10). The abnormal increases of these proinflammatory cytokines induced by DSS were significantly attenuated by the intervention of L. plantarum AR326 (P < 0.05, Fig. 10). The cytokine levels in the L. plantarum intervention group were comparable to those of the normal group (Fig. 10).
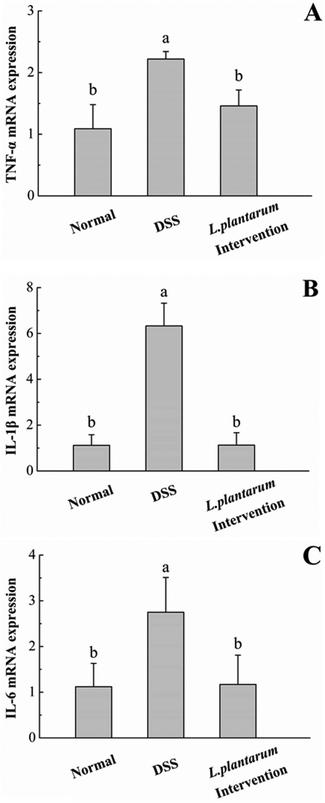 |
| Fig. 10 The mRNA levels of TNF-α (A), IL-1β (B) and IL-6 (C) were determined using quantitative real-time PCR in the colon. Data are represented as means ± standard deviations (n = 5). Means with different letters differ significantly (P < 0.05). | |
4. Discussion
In this study, a positively linear relationship between auto-aggregation (RAA) and co-aggregation (RCA) rates was observed for 15 Lactobacillus strains with RAA = 16–46% and RCA = 15–27%, except for L. casei AR342 and L. paracasei AR338 with a very low RCA value (6–8%) (Fig. 3A). This relationship had not been discovered before for the 22 Lactobacillus strains,19 which show variations in RAA (24–41%) and RCA (21–32%) mostly comparable to those found in this study. For co-aggregation with E. coli O157, the obtained RCA values for the L. casei group (L. casei and L. paracasei strains) in this study (RCA = 6–19%) and the study by Tuo et al.19 (RCA = 22–32%) are lower than the findings for L. paracasei subsp. paracasei BGSJ2-8 (RCA ∼ 45%).31
Based on five representative Lactobacillus strains with diverse RAA values (14–46%) (Fig. 4A), the adhesion ability to HT-29 cells appeared to change as a linear function of RAA with a positive slope and high correlation coefficient. This correlation is evidently better than those for the 22 Lactobacillus strains adhering to Caco-2 cells, another type of human adenocarcinoma cell, and for Lactobacillus and Bifidobacterium strains adhering to Caco-2 cells.19,20,22
As for the hydrophobicity index (AHC), its dependence on RAA is generally insignificant for the Lactobacillus strains of high AHC values (>60%), but significant for medium- to low-AHC strains as found in this study (Fig. 3B) and the study by Tuo et al.19 The adhesion ability relied linearly on the AHC value for L. brevis AR281, L. plantarum AR326, LGG, and L. casei AR342, rather than L. fermentum AR184 (Fig. 4B). This suggests that the adhesion ability is partly species-specific and cannot be described by hydrophobicity alone, supporting the viewpoint of previous reports.19,20,32 This further explains why different reports conclude different correlations between adhesion ability and hydrophobicity for lactobacilli.19,20,32,33
Evidently, there is likely another cell surface factor besides hydrophobicity that is important for auto-aggregation, co-aggregation, and adhesion abilities. And, RAA, more than AHC, may be a good index for evaluating the in vivo adhesion ability and colonization potential of lactobacilli.
Using cFDA-SE labelled L. plantarum AR326 for verifying the real adhesion capability and colonization of lactobacilli in mouse intestine, L. plantarum AR326 was confirmed to adhere to and colonize well in the intestinal mucosa of mice, mainly in the ileum, followed by the colon, and partly in the jejunum and duodenum (Fig. 5). This distribution is similar to the case of L. casei25 and provides direct evidence that L. plantarum AR326 is able to colonize well in the intestinal tract for a long time. This prolonged adhesion and colonization favour probiotic effects.
In this study, after DSS treatment daily for 5 days, oral administration with L. plantarum AR326 did reduce significantly the body weight loss and DAI score, recover the DSS-damaged colon length to a normal level, and significantly reduced the DSS-induced hyper-activated MPO activity and histopathological changes of ICR mice with DSS-induced colitis. The histological examination showed that oral administration with L. plantarum AR326 reversed the mucosal erosion and disruption of intestinal epithelial integrity that were induced by DSS. These results were consistent with the recovery of the expression of tight junction proteins (MUC2, ZO-1, claudin 3 and E-cadherin 1) by L. plantarum AR326. The pro-inflammatory cytokines TNF-α, IL-1β and IL-6 are associated with the severity of colitis.5,10,30 The levels of pro-inflammatory cytokines increased in IBD patients and also in DDS-induced colitis animal models.10,34 Previous studies have demonstrated that reducing the expression of cytokines, such as TNF-α, IL-1β or IL-6, can attenuate the development of colitis clinically and in DSS-induced experimental colitis.35,36Lactobacilli and Bifidobacterium have been reported to mediate the inflammatory role by the inhibition of pro-inflammatory cytokines, such as TNF-α, IL-1β and IL-6.4,5,10,11,13,27,30 In the present study, the levels of pro-inflammatory cytokines (TNF-α, IL-1β and IL-6) increased in the DSS-induced mice and oral administration with L. plantarum AR326 significantly attenuated these cytokine levels. In summary, our results suggest that L. plantarum AR326 attenuates the severity of colitis by restoring the tight junction protein expressions and by inhibiting pro-inflammatory mediators.
Lactobacillus plantarum AR326 was discovered to have the topmost overall performance in aggregation and adhesion abilities among the selected strains. Also, L. plantarum AR326 was confirmed to adhere to and colonize well in the intestinal mucosa of mice for a long time. The daily dosage of L. plantarum AR326 used in this study (2 × 109 cfu per mouse, i.e. 1 × 1011 cfu per kg bw, for 8 days, after DSS treatment) is effective for ameliorating the disease symptoms associated with DSS-induced colitis of ICR mice. Comparatively, oral administration with L. fermentum BR11 at a daily dosage of (1.1–2.3) × 1011 cfu per kg bw for 7 days prior to DSS treatment and during the 7-day DSS treatment alleviates the disease symptoms of male Sprague Dawley rats with DSS-induced colitis, with an effectiveness better than that of LGG.13 A similar effectiveness was also discovered in the case of L. plantarum K68 at a daily dosage of ∼5 × 1010 cfu per kg bw and with the same intervention schedule for female BALB/c mice with DSS-induced colitis.10
Oral administration of L. fermentum CCTCC M206110 at a daily dosage of 6 × 109 cfu per kg bw for 3 days prior to DSS treatment and during DSS treatment for 6 days also alleviates effectively the disease symptoms of female BALB/c mice with DSS-induced colitis, with an effectiveness greater than that of L. plantarum NCIMB8826 which can only reduce the extents of weight loss and colon length shortening, rather than alleviating DAI and histological damage.12 Pre-oral administration of LGG NutRes1 at a dosage of ∼4 × 1010 cfu per kg bw once every second day five times prior to DSS treatment shows mildly ameliorative effects on colon length shortening, histological score, and feces scores, but not MPO, for female C57BL/6 mice with DSS-induced colitis, with an effectiveness significantly less than that of Bifidobacterium breve NutRes204.14 Besides, intra-gastric injection of L. plantarum 21 at a daily dosage of 8 × 1010 cfu per kg bw for 2 weeks for female Wistar rats11 and L. plantarum CLP-0611 at a daily dosage of 4 × 1010 cfu per kg bw for 3 days for male ICR mice9 can ameliorate effectively the disease symptoms of trinitrobenzenesulfonic acid-induced colitis.
5. Conclusions
In this study, seventeen Lactobacillus strains were evaluated for their auto-aggregation rate (RAA), co-aggregation rate (RCA), and cell surface hydrophobicity (AHC), illustrating a positive, linear RCA–RAA relationship for most of the Lactobacillus strains examined except L. casei AR342 and L. paracasei AR338 with extraordinarily low RCA values. Lactobacillus plantarum AR326 was discovered to have the topmost overall performance in aggregation and adhesion abilities among the selected strains concerned. This strain was found to colonize and sustain very well in the ileum and colon of ICR mice. In this study, L. plantarum AR326 could effectively alleviate the disease symptoms of ICR mice with DSS-induced colitis by reducing body weight loss, disease activity index, colon length shortening, MPO activity and histological damage. Further studies showed that the intervention of L. plantarum AR326 could restore the tight junction protein expression and reduce the abnormal expression of pro-inflammatory cytokines. In conclusion, L. plantarum AR326 can be considered as an excellent probiotic with high potential for ameliorating DSS-induced colitis. It can be applied as a nutraceutical supplement or pharmaceutical adjuvant.
Conflicts of interest
There are no conflicts to declare.
Acknowledgements
This work was supported by the National Natural Science Foundation of China (No. 31771956) and the “Shu Guang” project supported by Shanghai Municipal Education Commission.
References
- Q. Mu, V. J. Tavella and X. M. Luo, Role of Lactobacillus reuteri in Human Health and Diseases, Front. Microbiol., 2018, 9, 757 CrossRef PubMed.
- M. J. Claesson, D. van Sinderen and P. W. O'Toole, The genus Lactobacillus–a genomic basis for understanding its diversity, FEMS Microbiol. Lett., 2007, 269, 22–28 CrossRef CAS PubMed.
- A. Sharma, B. Viswanath and Y. S. Park, Role of probiotics in the management of lung cancer and related diseases: An update, J. Funct. Foods, 2018, 40, 625–633 CrossRef.
- L. Prisciandaro, M. Geier, R. Butler, A. Cummins and G. Howarth, Probiotics and their derivatives as treatments for inflammatory bowel disease, Inflammatory Bowel Dis., 2009, 15, 1906–1914 CrossRef PubMed.
- M. J. Saez-Lara, C. Gomez-Llorente, J. Plaza-Diaz and A. Gil, The role of probiotic lactic acid bacteria and bifidobacteria in the prevention and treatment of inflammatory bowel disease and other related diseases: a systematic review of randomized human clinical trials, Biomed. Res. Int., 2015, 2015, 505878 Search PubMed.
- M. Kechagia, D. Basoulis, S. Konstantopoulou, D. Dimitriadi, K. Gyftopoulou, N. Skarmoutsou and E. M. Fakiri, Health benefits of probiotics: a review, ISRN Nutr., 2013, 481651 Search PubMed.
- R. C. Martinez, R. Bedani and S. M. Saad, Scientific evidence for health effects attributed to the consumption of probiotics and prebiotics: an update for current perspectives and future challenges, Br. J. Nutr., 2015, 114, 1993–2015 CrossRef CAS PubMed.
- M. Perse and A. Cerar, Dextran sodium sulphate colitis mouse model: traps and tricks, J. Biomed. Biotechnol., 2012, 718617 Search PubMed.
- S. E. Jang, M. J. Han, S. Y. Kim and D. H. Kim,
Lactobacillus plantarum CLP-0611 ameliorates colitis in mice by polarizing M1 to M2-like macrophages, Int. Immunopharmacol., 2014, 21, 186–192 CrossRef CAS PubMed.
- Y. W. Liu, Y. W. Su, W. K. Ong, T. H. Cheng and Y. C. Tsai, Oral administration of Lactobacillus plantarum, K68 ameliorates DSS-induced ulcerative colitis in BALB/c mice via the anti-inflammatory and immunomodulatory activities, Int. Immunopharmacol., 2011, 11, 2159–2166 CrossRef CAS PubMed.
- C. S. Satish Kumar, K. Kondal Reddy, A. G. Reddy, A. Vinoth, S. R. Ch, G. Boobalan and G. S. Rao, Protective effect of Lactobacillus plantarum, 21, a probiotic on trinitrobenzenesulfonic acid-induced ulcerative colitis in rats, Int. Immunopharmacol., 2015, 25, 504–510 CrossRef CAS PubMed.
- Y. Cui, H. Wei, F. Lu, X. Liu, D. Liu, L. Gu and C. Ouyang, Different effects of three selected Lactobacillus strains in dextran sulfate sodium-induced colitis in BALB/c Mice, PLoS One, 2016, 11, e0148241 CrossRef PubMed.
- M. S. Geier, R. N. Butler, P. M. Giffard and G. S. Howarth,
Lactobacillus fermentum BR11, a potential new probiotic, alleviates symptoms of colitis induced by dextran sulfate sodium (DSS) in rats, Int. J. Food Microbiol., 2007, 114, 267–274 CrossRef PubMed.
- B. Zheng, J. van Bergenhenegouwen, S. Overbeek, H. J. van de Kant, J. Garssen, G. Folkerts, P. Vos, M. E. Morgan and A. D. Kraneveld,
Bifidobacterium breve attenuates murine dextran sodium sulfate-induced colitis and increases regulatory T cell responses, PLoS One, 2014, 9, e95441 CrossRef PubMed.
- A. K. Yadav, A. Tyagi, A. Kumar, A. C. Saklani, S. Grover and V. K. Batish, Adhesion of indigenous Lactobacillus plantarum to gut extracellular matrix and its physicochemical characterization, Arch. Microbiol., 2015, 197, 155–164 CrossRef CAS PubMed.
- A. Ljungh and T. Wadstrom, Lactic acid bacteria as probiotics, Curr. Issues Intest. Microbiol., 2006, 7, 73–89 CAS.
- K. Papadimitriou, G. Zoumpopoulou, B. Foligne, V. Alexandraki, M. Kazou, B. Pot and E. Tsakalidou, Discovering
probiotic microorganisms: in vitro, in vivo, genetic and omics approaches, Front. Microbiol., 2015, 6, 58 Search PubMed.
-
M. Botes, Survival of probiotic lactic acid bacteria in the intestinal tract, their adhesion to epithelial cells and their ability to compete with pathogenic microorganisms, Degree of Doctor of Philosophy (Microbiology) at the University of Stellenbosch, 2008 Search PubMed.
- Y. Tuo, H. Yu, L. Ai, Z. Wu, B. Guo and W. Chen, Aggregation and adhesion properties of 22 Lactobacillus strains, J. Dairy Sci., 2013, 96, 4252–4257 CrossRef CAS PubMed.
- B. Del Re, B. Sgorbati, M. Miglioli and D. Palenzona, Adhesion, autoaggregation and hydrophobicity of 13 strains of Bifidobacterium longum, Lett. Appl. Microbiol., 2000, 31, 438–442 CrossRef CAS PubMed.
- M. Gueimonde, L. Jalonen, F. He, M. Hiramatsu and S. Salminen, Adhesion and competitive inhibition and displacement of human enteropathogens by selected lactobacilli, Food Res. Int., 2006, 39, 467–471 CrossRef CAS.
- C. Botta, T. Langerholc, A. Cencic and L. Cocolin, In vitro selection and characterization of new probiotic candidates from table olive microbiota, PLoS One, 2014, 9, 1–15 Search PubMed.
- M. C. Collado, J. Meriluoto and S. Salminen, Measurement of aggregation properties between probiotics and pathogens: In vitro evaluation of different methods, J. Microbiol. Methods, 2007, 71, 71–74 CrossRef CAS PubMed.
- Y. Tuo, X. Song, Y. Song, W. Liu, Y. Tang, Y. Gao, S. Jiang, F. Qian and G. Mu, Screening probiotics from Lactobacillus strains according to their abilities to inhibit pathogen adhesion and induction of pro-inflammatory cytokine IL-8, J. Dairy Sci., 2018, 101, 4822–4829 CrossRef CAS PubMed.
- Y. K. Lee, P. S. Ho, C. S. Low, H. Arvilommi and S. Salminen, Permanent colonization by Lactobacillus casei is hindered by the low rate of cell division in mouse gut, Appl. Environ. Microbiol., 2004, 70, 670–674 CrossRef CAS PubMed.
- M. C. Collado, J. Meriluoto and S. Salminen, Adhesion and aggregation properties of probiotic and pathogen strains, Eur. Food Res. Technol., 2008, 226, 1065–1073 CrossRef CAS.
- F. Tao, C. Qian, W. Guo, Q. Luo, Q. Xu and Y. Sun, Inhibition of Th1/Th17 responses via suppression of STAT1 and STAT3 activation contributes to the amelioration of murine experimental colitis by a natural flavonoid glucoside icariin, Biochem. Pharmacol., 2013, 85, 798–807 CrossRef CAS PubMed.
- H. Laroui, S. A. Ingersoll, H. C. Liu, M. T. Baker, S. Ayyadurai, M. A. Charania, F. Laroui, Y. Yan, S. V. Sitaraman and D. Merlin, Dextran Sodium Sulfate (DSS) induces colitis in mice by forming nano-lipocomplexes with medium-chain-length fatty acids in the colon, PLoS One, 2012, 7, e32084 CrossRef CAS PubMed.
- V. Loria, I. Dato, F. Graziani and L. M. Biasucci, Myeloperoxidase: a new biomarker of inflammation in ischemic heart disease and acute coronary syndromes, Mediators Inflammation, 2008, 2008, 135625 CrossRef PubMed.
- S. Seo, J. Shin, W. Lee, Y. k. Rhee, C. Cho, H. Hong and K. Lee, Anti-colitis effect of Lactobacillus sakei K040706 via suppression of inflammatory responses in the dextran sulfate sodium-induced colitis mice model, J. Funct. Foods, 2017, 29, 256–268 CrossRef CAS.
- M. Nikolic, B. Jovcic, M. Kojic and L. Topisirovic, Surface properties of Lactobacillus and Leuconostoc isolates from homemade cheeses showing auto-aggregation ability, Eur. Food Res. Technol., 2010, 231, 925–931 CrossRef CAS.
- E. Vlková, V. Rada, M. Šmehilová and J. Killer, Auto-aggregation and Co-aggregation ability in bifidobacteria and clostridia, Folia Microbiol., 2008, 53, 263–269 CrossRef PubMed.
- H. Xu, H. S. Jeong, H. Y. Lee and J. Ahn, Assessment of cell surface properties and adhesion potential of selected probiotic strains, Lett. Appl. Microbiol., 2009, 49, 434–442 CrossRef CAS PubMed.
- Y. Yang, J. He, Y. Suo, L. Lv, J. Wang and C. Huo, Anti-inflammatory effect of taurocholate on TNBS-induced ulcerative colitis in mice, Biomed. Pharmacother., 2016, 81, 424–430 CrossRef CAS PubMed.
- K. H. Kwon, A. Murakami, R. Hayashi and H. Ohigashi, Interleukin-1β targets interleukin-6 in progressing dextran sulfate sodium-induced experimental colitis, Biochem. Biophys. Res. Commun., 2005, 337, 647–654 CrossRef CAS PubMed.
- M. M. Lawson, A. G. Thomas and A. K. Akobeng, Tumour necrosis factor alpha blocking agents for induction of remission in ulcerative colitis, Cochrane Database Syst. Rev., 2006, 3, CD005112 Search PubMed.
Footnote |
† Electronic supplementary information (ESI) available. See DOI: 10.1039/c8fo01453a |
|
This journal is © The Royal Society of Chemistry 2019 |
Click here to see how this site uses Cookies. View our privacy policy here.