DOI:
10.1039/C9CY01331H
(Minireview)
Catal. Sci. Technol., 2019,
9, 5233-5255
Recent advances in transition metal-catalysed cross-coupling of (hetero)aryl halides and analogues under ligand-free conditions
Received
11th July 2019
, Accepted 30th August 2019
First published on 2nd September 2019
Abstract
The formation of new bonds is pivotal in organic chemistry and a prerequisite to life because it allows the construction of complex molecules from simple precursors. This gives credence to the intensive study of metal-catalysed C–C bond formation via cross-coupling reactions over the past few decades. However, despite the tremendous success recorded for ligand-supported palladium in the catalytic transformation of (hetero)aryl halides, the challenges in their practical application lie in their cost and difficulty of preparation, sensitivities to air/moisture, toxicity and complete incompatibility with aqueous media, which remarkably limit their industrial applications. Non-ligated or ligand-free metal-catalysed cross-coupling reactions are attractive alternatives to ligand-supported counterparts due to their recyclability, cost-effectiveness, sustainability and full industrial applications. Over the years, various research groups have strived to advance ligand-free catalytic systems for cross-coupling of (hetero)aryl halides because of the aforementioned advantages. This review highlights the progress, challenges and prospects in ligand-free metal-catalysed cross-coupling of (hetero)aryl halides and their analogues.
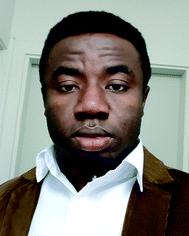 Jude I. Ayogu | Jude I. Ayogu obtained his B.Sc (First Class) in Pure and Industrial Chemistry from the University of Nigeria, Nsukka in 2011. He proceeded to obtain his Master's degree (Organic Chemistry) where he made parallel distinction in 2015. He is a recipient of the Outstanding Academic Excellence Award (2011) and UC Doctoral Scholarship (2016), among others. He started his teaching career as Graduate Assistant in Feb. 2013 in the University of Nigeria Nsukka. Besides other contract appointments such as lab demonstrator (2017), teaching assistant (2018), and senior teaching assistant (2019), he is currently a lecturer II in the Department of Pure & Industrial Chemistry, University of Nigeria. |
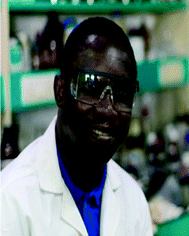 Efeturi A. Onoabedje | Efeturi A. Onoabedje completed his Ph. D in Organic Chemistry in 2014 at the University of Nigeria, with a research stint in Cardiff University, Cardiff, Wales, UK. His Ph.D research focused on the application of palladium catalysis in functionalisation of phenothiazine and phenoxazine scaffolds. He is a recipient of Petroleum Technology Development Fund (PTDF) Ph.D Scholarship (2012) and CSIR-TWAS Post-Doc Fellowship (2017) awards. He is presently a Senior Lecturer in the Department of Pure & Industrial Chemistry, University of Nigeria, where he teaches courses in organic chemistry and carries out research in the design and synthesis of anti-infective agents. |
1. Introduction
The emergence of organic transformations catalysed by transition metals, commonly known as transition metal cross-coupling reactions (Scheme 1),1 has virtually transformed the trend in organic synthesis and these reactions have remained as simple strategies for rapid construction of C–C, C–N, C–O and other C-heteroatom bonds from readily available appropriately functionalized substrates.2,3 Palladium metal has emerged as a choice catalyst for a wide range of cross-coupling reactions chiefly because reactions catalysed by palladium are generally mild and compatible with numerous functional groups.4 In addition, palladium catalysts generally allow selective reactions with high turnover numbers (TONs) and turnover frequencies (TOFs).5
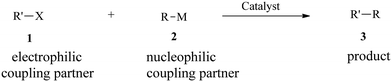 |
| Scheme 1 General equation for a transition metal-catalysed cross-coupling reaction. | |
Metal-catalysed methodologies have been proven to be the most powerful and find use in target- or diversity-oriented synthesis which was previously impossible or only possible using multi-step approaches. They have found utility in synthetic routes to biphenyls or substituted aromatic moieties, prochiral ketones, acrylates and other related compounds of interest in pharmaceuticals,6,7 including but not limited to indanone A, nifedipine, losartan, HIV-1 protease and taxol, which are subunits in many marketed drugs, agrochemicals, fine chemicals, conducting polymers, liquid crystalline materials, etc.8,9
Transition metal-catalysed cross-coupling reactions have been applied in the construction of C–N bonds10 including the complementary addition of an alkyl, alkenyl, or aryl group to an amide,11 breaking of classical inert amide bonds,12,13 non-conventional installation of an amino group on alkenes and alkynes,14etc. They have also been adapted to perform intra-cellular bio-orthogonal reactions for the intra-cellular synthesis of drugs including cytotoxic agents15 as well as site-selective derivatisation and cyclisation of peptides.16 Consequently, because of their several advantages over classical reactions,14 they have been under the spotlight of several academic and industrial research groups leading to significant improvements during the last two decades. They have been applied in the construction of an array of compounds including highly functionalized heteroaromatics14 which are significant substructures found in various natural or synthetic alkaloids, linear and fused ring-substituted phenothiazine and phenoxazine systems,17,18 chalcones as well as flavonoids,19 ynones,20 and steroids,21 to mention but a few. Due to the diversity of the structures encountered, as well as their biological and pharmaceutical relevance, several new economic, efficient and selective synthetic strategies to access these compounds are being developed.
The development of a ligand-free approach for aryl, heteroaromatic and vinyl fluorosulfates, halides, or traditional sulfates (triflates, mesylates and tosylates)22 as electrophilic partners for transition metal-catalysed cross-coupling reactions has attracted increasing attention because the process is more cost-effective and also avoids ligand and metal contaminations in the final product.23,24 In other words, the phosphine-based ligands used in conventional transition metal-catalysed cross-coupling reactions are expensive compared to the metal catalyst employed; thus the process is cheaper. Ligand-free activation of aryl chloride is one of the most challenging fields in synthetic organic chemistry.25–27 However, over the past two decades, the attention of many research groups has been directed towards catalytic activation/functionalization of aryl and heteroaryl halides under ligand-free conditions due to its economy and environmental friendliness. This review captures the recent advances in ligand-free transition metal-catalysed Suzuki–Miyaura,28,29 Sonogashira,30,31 Hiyama,32 Mizoroki-Heck,33,34 Negishi35 and Stille36 reactions.
2. Ligand-free Suzuki–Miyaura cross-coupling
The Suzuki cross-coupling reaction is one of the most versatile and utilised reactions for the selective construction of carbon–carbon bonds. It is mainly used in the formation of biaryl compounds, substituted aromatic compounds and alkene derivatives that are structural components of numerous natural products, agrochemicals, pharmaceuticals, and advanced materials, such as organic electroluminescent (EL) materials.37,38 The Suzuki reaction is a palladium or nickel-catalysed cross-coupling reaction of an organoboron reagent and an organic halide or pseudohalide in the presence of a base.39,40 Reports on the palladium-mediated Suzuki reaction of aryl boronic acids with aryl iodides or activated bromides in water as a solvent have been demonstrated with only one recent exception.41–43 The coupling of aryl chlorides has also been reported but involves the use of an oxime-carbapalladacycle as the catalyst.44 Several reports on the use of water as a co-solvent focusing on the use of aryl iodides or activated bromides have been made,45,46 and one recent report47 demonstrated the use of aryl chlorides in a 20
:
1 DMA/water mix. The first reports on microwave-mediated Suzuki reactions came in 1996, using Pd(PPh3)4 as a catalyst both for homogeneous48 and solid-phase49 biaryl syntheses. More recently, microwave heating has been used to facilitate the coupling of aryl boronic acids with poly(ethylene glycol) esters of an aryl iodide, triflate, and bromothiophene in water.50 Suzuki–Miyaura cross-coupling offers many advantages over other transition metal-catalysed reactions51 which include easy availability of organoboron compounds, stability to moisture and air, low toxicity, broad functional group tolerance, and relatively milder reaction conditions.52 Due to economic and environmental concerns, the Suzuki reaction has been adapted to ligand-free conditions. In recent years, a lot of ligand-free Suzuki cross-coupling reactions have been reported for the formation of essential molecules.
a. Cross-coupling of aryl boronic acid or sodium tetraphenylborate with aryl halides
In 1997, Bumagin and Bykov27 reported a ligand-free palladium-catalysed Suzuki cross-coupling reaction between aryl halides 4 and organoboron compounds in which Pd(0) complexes were generated in situ from PdCl2 or Pd(OAc)2 in aqueous alkali solution to afford polyfunctional biaryls 5 and 6 in 60–99% yields (Scheme 2).
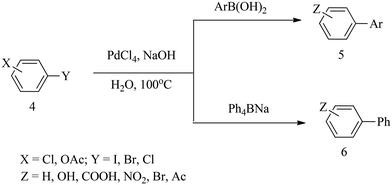 |
| Scheme 2 Ligand-free Pd-catalysed Suzuki reaction of aryl boronic acid and sodium tetraphenylborate with a variety of aryl halides. | |
The best conversion of p-chlorobenzoic acid 7 was achieved in the presence of 3 mol% PdCl2 pre-catalyst with NaOH within 3 h to furnish biphenyl 8 in 92% yield (Scheme 3). However, electron-rich chlorophenols reacted with sodium tetraphenylborate (Ph4BNa) to afford moderate yields of isolated products.
 |
| Scheme 3 Ligand-free Pd-catalysed synthesis of biphenyl-4-carboxylic acid. | |
A heterogeneous palladium catalytic system (Pd/C) in a dimethylacetamide–water (DMA–H2O) mixture cross-coupled phenylboronic acid with a variety of aryl halides without observable homocoupling by-products. Electron-poor aryl halides gave higher yields (79–95%) of biaryls, while neutral and deactivated aryl halides gave moderate yields, but an increase in the amount of catalytic loading improved the yields. The ability of Pd/C to activate the C–Cl bond in aryl chlorides was attributed to the synergistic anchimeric and electronic effect that takes place between the catalyst surface and the aryl chloride.27
b. Cross-coupling of 2-halogenopyridines with aryl boronic acids
Liu and co-workers53 demonstrated a very fast and efficient oxygen-promoted ligand-free Pd-catalysed cross-coupling reaction of substituted 2-halogenopyridines 10, 2-halogenopyrazines 11 and 5-halogenopyrimidines 12 with various boronic acids 9 for the synthesis of the corresponding 4-aryl substituted pyridine, pyrazine and pyrimidine derivatives 13–15 in aqueous isopropanol (Scheme 4). The cross-coupling reactions gave the biaryl products in 52–99% yields in a couple of hours.
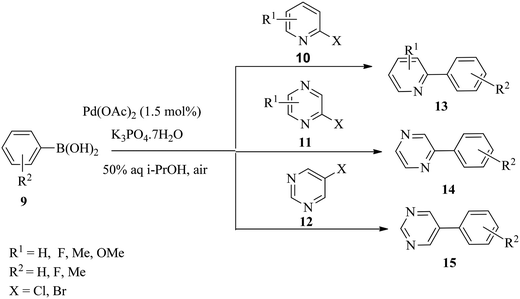 |
| Scheme 4 Oxygen-promoted Suzuki–Miyaura synthesis of 2-phenylpyridines. | |
However, heterocyclic compounds are of particular interest to the pharmaceutical industry because of their potential bioactivities,54,55 but their inclusion in metal-catalysed cross-coupling reactions remains a challenge as a result of the potential chelation of their donor groups such as the heteroatoms to metal centres.53,56 The binding either slows down the rate of the reaction or results in catalyst inactivity.57 Therefore, catalytic systems that are compatible with heterocyclic aryl halides will be advantageous in ligand-free cross-coupling reactions.
c. Microwave-assisted cross-coupling of aryl halides and aryl boronic acids
Leadbeater and Marco58 reported a ligand-free palladium-catalysed Suzuki reaction in water using microwave heating. Their methodology used a low palladium loading (0.4 mol%), was fast (5–10 min reaction time), and was usefully applied for coupling reactions involving aryl iodides, bromides and chlorides 16 with boronic acid 17 (Scheme 5).
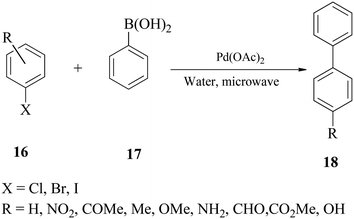 |
| Scheme 5 Microwave-mediated Suzuki coupling of aryl halides with aryl boronic acids in water using Pd(OAc)2. | |
d. Cross-coupling of aryl halides with aryl boronic acids
In 2011, Qiu and co-workers53 developed a convenient, effective and mild protocol for the palladium-catalysed ligand-free Suzuki reaction of aryl bromides 19 with aryl boronic acids 20 in aqueous N,N-dimethylformamide (DMF) in the presence of K2CO3 in air at room temperature (Scheme 6). The choice of volume ratio of DMF–H2O as well as the base is critical to the reaction. Hence, at a catalytic loading of 0.0025 mmol and a DMF–H2O ratio 1
:
1, a model coupling reaction of 4-bromoanisole with phenylboronic acid provided a quantitative yield within 5 min.
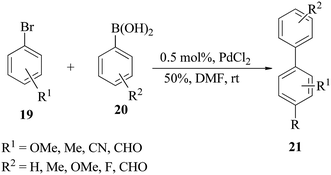 |
| Scheme 6 Suzuki reactions of aryl bromides with aryl boronic acids. | |
Furthermore, the optimised conditions of the protocol were extended to the cross-coupling reactions of nitrogen-based heteroaryl halides 23–25 with aryl boronic acids 22 in moderate to excellent yields (Scheme 7). A room temperature cross-coupling reaction of 2-chloropyrazine with phenylboronic acid and 4-methyl phenylboronic acid gave 83% and 88% yields of biaryls, respectively, within 24 h.
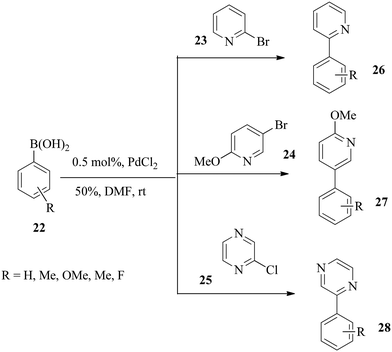 |
| Scheme 7 The Suzuki reaction of N-heteroaryl halides with aryl boronic acids. | |
e. Bio-supported cross-coupling of aryl halides with aryl boronic acids
Tang and co-workers57 described bio-supported and recyclable palladium nanoparticles as an efficient phosphine-free catalyst for the Suzuki reaction of an aryl halide 29 with substituted aryl boronic acids 30 in water (Scheme 8). The catalyst was highly recyclable, maintaining yields of 86% after five cycles in the coupling of iodobenzene with phenylboronic acids. The reaction conditions resulted in the coupling of chlorobenzene with phenylboronic acid in 89% yield at 95 °C. They also compared the coupling reaction mentioned above in DMF and an organic base. The result indicated that the reaction proceeded in less than 3.5 h at 85 °C, but the coupling gave a lower yield of 78%.57 This was probably due to a decrease in efficiency of heterogeneous catalysis with the dispersion of reactants into the bacterial cellulose network. With a recycled catalyst and when DMF is used as the reaction solvent, a lower yield was also recorded; so, it was concluded that environmentally friendly processes which employ water remain the best strategy.
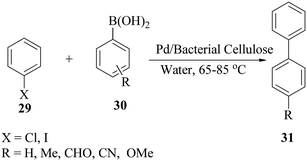 |
| Scheme 8 The Suzuki reaction of aryl halides with aryl boronic acids. | |
f. Bimetal catalysed cross-coupling of (hetero)aryl halides with an arylboronic acid
Kim and co-workers59 reported the application of carbon-supported bimetallic Pd–M (M = Ag, Ni, and Cu) nanoparticles synthesized by γ-irradiation at room temperature without reducing agents in Suzuki cross-coupling of a halide of thiophene, benzene and benzoic acid with phenylboronic acid in ethanol (Scheme 9). Evaluation of the catalytic efficiency determined by the yield of the reaction showed that the Pd–Cu bimetallic system supported on carbon is the most efficient (Pd–Cu/C > Pd/C > Pd–Ag/C > Pd–Ni/C) in the Suzuki cross-coupling of the halides of thiophene, benzene and benzoic acid with yields of 96.7%, 97.5%, and 88.7%, respectively.
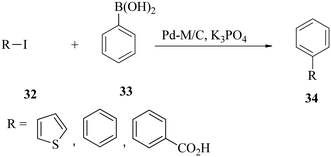 |
| Scheme 9 The Suzuki reaction of (hetero)aryl halides with phenylboronic acid. | |
g. Homeopathic cross-coupling of various aryl halides with boronic acids
The De Vries group demonstrated the application of a ‘homeopathic’ ligand-free palladium catalyst in the coupling of aryl bromides and chlorides.60 The catalyst (Pd(OAc)2) at a loading of 0.02–0.05 mol% generally performed well for both activated aryl bromides and chlorides, but mixed results were obtained with deactivated aryl bromides and chlorides (Scheme 10).
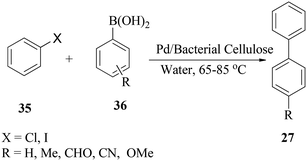 |
| Scheme 10 The Suzuki reaction of aryl halides with aryl boronic acids. | |
The cross-coupling of 4-chloronitrobenzene 38 with phenylboronic acid 39 in the presence of 0.05% Pd(OAc)2 at 130 °C for 17 h afforded 4-nitrobiphenyl 40 in 94% GC yield (Scheme 11).
 |
| Scheme 11 Homeopathic Suzuki reaction of aryl chlorides. | |
Interestingly, at a loading of as small as 0.005 mol%, the 4-nitrobiphenyl product was also obtained in 83% yield, but the reaction protocol failed to convert deactivated electron-rich aryl chlorides. The failure of the deactivated electron-rich aryl chlorides to react stems from increased C–Cl bond strength probably because of the contribution of both inductive and mesomeric effects.
3. Ligand-free Hiyama cross-coupling reaction
Cross-coupling reactions of organosilicon compounds have emerged as viable alternatives to Suzuki coupling reactions.43,61,62 The Hiyama cross-coupling reaction63 is a chemo- and stereoselective cross-coupling reaction of organosilanes with organic halides. It has gained popularity for asymmetric biaryl compound synthesis or construction of multi-substituted alkenes that are components of various functional materials. This is associated with low cost, low toxicity, commercial availability, easy preparation and handling, high tolerance to a variety of functional groups when compared to organomagnesium and organocopper, and high stability of organosilicon reagents to moisture and air compared to other organometallic compounds of zinc, aluminium, boron and zirconium conventionally used in cross-coupling reactions.32,64 Since the first report of the use of aryl(alkoxy)silanes for Hiyama coupling by Shibata and co-workers,65 reactive aryl bromides and iodides have been largely used instead of the relatively cheap and commercially available aryl chlorides.32,66 Several limitations have hampered the use of aryl chlorides in Hiyama coupling.64,67–71 Below are some of the recent ligand-less Hiyama cross-coupling reactions.
a. Cross-coupling of substituted aryl halides with arylalkoxysilanes
Sajiki and co-workers72 reported the first active palladium on carbon-catalysed ligand-free Hiyama cross-coupling of a variety of electron-rich and electron-poor aryl halides 41 with aryltriethoxysilanes 42 in good to excellent yields at 0.5 mol% Pd/C catalytic loading in refluxing toluene within 24 h (Scheme 12). The use of fluoride sources such as TBAF·3H2O or metal fluorides is critical for the reaction to proceed. The reaction was also extended to phenytrimethoxysilane derivatives, and good yields were obtained within 48 h.
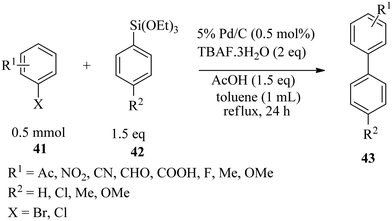 |
| Scheme 12 The Hiyama cross-coupling of various aryl halides with aryltriethoxysilanes. | |
In the same vein, Ranu and co-workers73 reported a simple one-pot fluoride-free operation using in situ generated palladium(0) nanoparticles for efficient Hiyama cross-coupling of a wide variety of substituted aryl bromides and iodides with aryl silanes in water (Scheme 13). The reaction proceeded very quickly and was performed in air with excellent chemoselectivity and high yields.
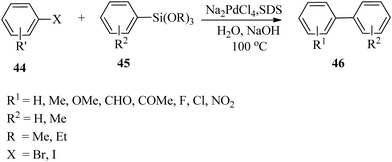 |
| Scheme 13 One-pot fluoride-free palladium(0) nanoparticle catalysed Hiyama coupling of aryl bromides/iodides with arylsiloxane. | |
A similar reaction was independently reported by Sarkar and co-workers74 using colloidal palladium nanoparticles (1 mol%) generated from the Fischer carbene complex of tungsten as the reductant and PEG as the capping agent in water.
b. Cross-coupling of allyl acetates with aryl/vinyl silanes
In 2008, Ranu and co-workers75 reported a simple and efficient palladium(0) nanoparticle catalysed Hiyama cross-coupling reaction of both activated and unactivated open-chain allylic acetates and organosiloxanes. They demonstrated that several structurally substituted allyl acetates 47 underwent regio- and stereoselective coupling reactions with phenyl, tolyl, and vinyl siloxanes 48 to produce the corresponding allylated products 49 in 65–82% yields over 7–10 h (Scheme 14). The coupling with vinyl siloxanes was a novel method for the synthesis of 1,4-pentadiene.
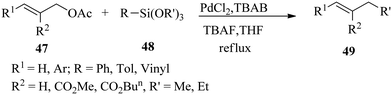 |
| Scheme 14 One-pot fluoride-free palladium(0) nanoparticle catalysed Hiyama coupling of allylic acetates with arylsiloxane. | |
c. Bimetallic-catalysed cross-coupling of haloaryls with phenylmethoxysilane
Rothenberg and co-workers76 reported the application of stable and highly mono-dispersed core–shell bimetallic Ni–Pd nanoparticles in the ligand-free Hiyama cross-coupling reaction of different haloaryls 50 and phenylmethoxysilane 51. With a variety of iodo- and bromoaryls, good product yields were obtained, thus confirming that they acted as a good source of biaryl-containing compounds which are vital intermediates for preparing biologically active molecules, organic semiconductors and liquid crystals (Scheme 15).77
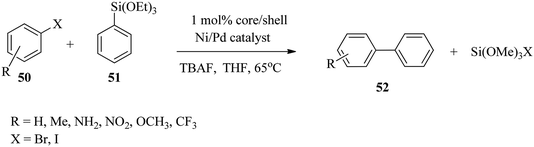 |
| Scheme 15 Bimetallic Ni–Pd nanoparticle catalysed ligand-free Hiyama cross-coupling reaction of phenylmethoxysilane with various haloaryls. | |
4. Ligand-free Sonogashira cross-coupling
The generation of sp–sp2 carbon–carbon bonds by cross-coupling of terminal alkynes with aryl halides or vinyl halides is termed Sonogashira cross-coupling.78,79 A palladium (Pd) catalyst, ligand, copper(I) salt co-catalyst and amine base are requirements for a standard Sonogashira cross-coupling reaction. The Sonogashira reaction has been reported for the synthesis of alkyne-containing aromatic compounds,80,81 natural products,82,83 medicinal products,84,85 oligomers,86,87 and polymers.88 The major setbacks of the reaction are the sensitivity of the copper co-catalyst to oxygen, causing oxidative homocoupling of acetylenes to form diacetylenes and copper acetylides which are potentially explosive,79 difficulty in removal of high boiling point solvents from the reaction mixture, increase in cost due to low reusability of the homogeneous Pd catalyst,89 and contamination of the product by toxic Pd metal.90,91 Significant efforts have been directed to improving Sonogashira reactions.89 Among the developments are ligand-free and copper-free Sonogashira reactions which reduce the cost, simplify the reaction procedures and avoid the formation of oxidative homocoupling by-products. In the same vein, high boiling solvents have been substituted with water and other benign solvents.92,93 The development of supported Pd for heterogeneous catalysis simplifies the separation and recyclability of the catalyst. Several catalyst supports such as perovskites,94 carbon,95,96 zeolites,97 hydrotalcite,97 silica,98 cellulose,99 and polymers100 have been employed. In the development of supported catalysts, the use of the right amount of catalyst loading on the supporting material to ensure catalyst reactivity and reduce catalyst leaching is a significant consideration.101,102 To date, most of the supported catalysts developed still have ample room for further improvement.103 From the green chemistry point of view, using recyclable supported catalysts in aqueous media is desirable.
a. Solid-supported coupling of nucleotide derivatives
Stoltz and co-workers104 explored the Pd/C and Amberlite IRA-67-mediated ligand-free Sonogashira coupling of several deoxynucleoside derivatives 54 with trifluoroacetyl 55 and phthalimido reagents 56. They modelled the strategy employed by Kotschy and co-workers105 replacing Pd/C with Pd(PPh3)4, lowering the catalyst loading to 5% and carrying out the reaction at 50 °C to afford deoxyuridine, uridine, dideoxyuridine and deoxycytidine derivatives 57–58 (Scheme 16). The dideoxyuridine derivatives are particularly crucial as critical intermediates in the synthesis of conventional Sanger DNA sequencing reagents.106
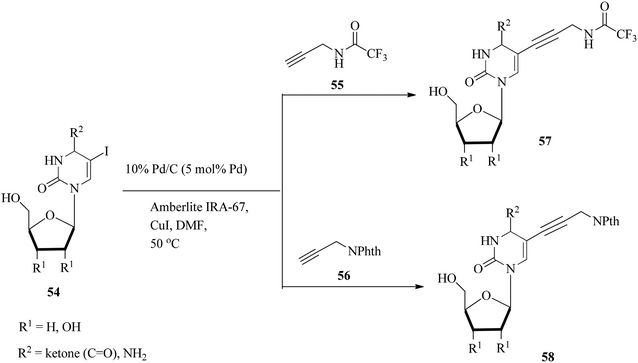 |
| Scheme 16 Solid-supported Sonogashira coupling of deoxynucleoside derivatives. | |
The conditions were extended to the synthesis of 2-deoxyadenosine and 2-deoxyguanosine derivatives 59 (Scheme 17). Although the coupling of 8-bromo-2-deoxyadenosine and 8-bromo-2-deoxyguanosine derivatives was sluggish under Pd/C-catalysis, the use of the Pd(PPh3)4/Amberlite IRA-67 system gave 78% and 98% yields of 60a and 60b in 11 h and 7 h, respectively.
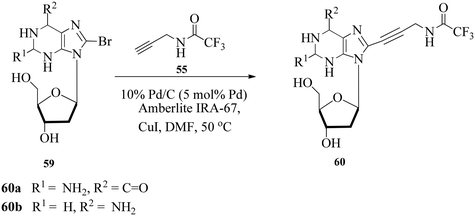 |
| Scheme 17 Solid-supported Sonogashira coupling of deoxyadenosine and deoxyguanosine derivatives. | |
b. Polymer-supported cross-coupling of aryl halides with phenylacetylene derivatives in water
Ligand-less Pd(0) loaded polymethyl methacrylate (PMMA) microspheres were employed for the Sonogashira cross-coupling reaction in aqueous media by Zhang and co-workers in 2012.103 A variety of terminal acetylenes 61 were coupled with different phenyl iodides, bromides and chlorides 62 (Scheme 18). Phenyl iodides containing electron-donating or electron-withdrawing groups coupled readily with phenylacetylenes in excellent product yields.
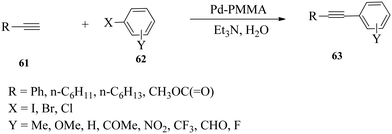 |
| Scheme 18 Polymer-supported cross-coupling of aryl halides with phenylacetylenes in water. | |
c. Copper nanocluster catalysed cross-coupling of alkynes with aryl halides
Rothenberg and co-workers107 were the first to obtain substituted diphenylalkynes 66 in good isolated yields and high selectivity from palladium-free and ligand-free Sonogashira cross-coupling of phenylacetylene 64 with various aryl halides 65 under stable copper cluster catalysis in 2004. 1.5 eq. of phenylacetylene and 1 eq. of each of the aryl halides were reacted in the presence of 1.5 eq. of the base and 5 mol% of metal clusters at 110 °C (Scheme 19). The stable metal cluster was prepared by reducing solutions of the chloride salt precursor with tetraoctylammonium formate (TOAF) in DMF at 65 °C.
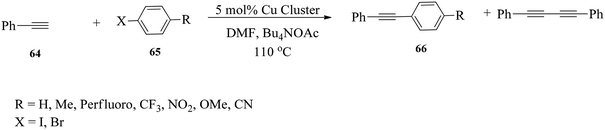 |
| Scheme 19 Copper nanocluster catalysed cross-coupling of alkynes and aryl halides. | |
d. Nanoparticle supported cross-coupling of alkynes and (hetero)aryl halides
Wei and co-workers108 reported the application of in situ generated palladium nanoparticles as a catalyst supported on Al(OH)3 (Scheme 20) in ligand- and copper-free Sonogashira cross-coupling reactions of (hetero)aryl bromides and chlorides.
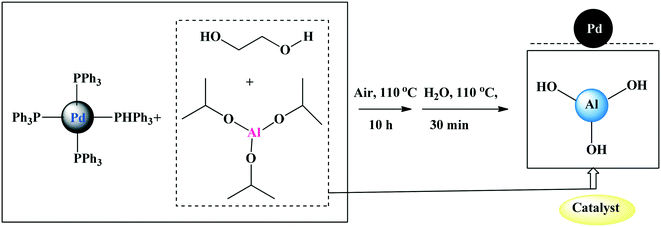 |
| Scheme 20 Preparation of the Pd nanoparticle catalyst.108 | |
The optimised reaction conditions were applied in cross-coupling of various (hetero)aryl halides 69 bearing neutral, electron-withdrawing or electron-donating substituents in the phenyl ring with phenylacetylene 68 bearing electron-donating and electron-withdrawing groups in the benzene ring to afford the 1,3-diyne products in 53–98% yields (Scheme 21).
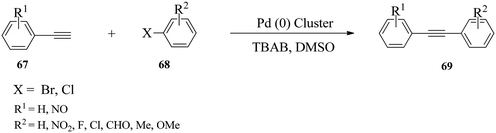 |
| Scheme 21 Cross-coupling of aryl halides with phenylacetylene. | |
Heteroaryl bromides derived from pyridines, thiophenes, quinolines, and pyrimidines 71 were converted to the corresponding cross-coupled products 72 in moderate to excellent yields (Scheme 22).
 |
| Scheme 22 Cross-coupling of (hetero)aryl acetylenes with bromobenzene. | |
e. Cross-coupling of aromatic and aliphatic terminal alkynes with aryl iodides
Sajiki and co-workers109 reported the cross-coupling of a variety of aryl iodides 73 with aromatic and aliphatic terminal alkynes 74 to give the corresponding 1,2-disubstituted aromatic alkynes 75 in good yields by using only 0.4 mol% of the heterogeneous 10% Pd/C as the catalyst without a ligand, copper salt, or amine in an aqueous medium (Scheme 23). They explored the scope and limitations of the substrates under the optimized reaction conditions (10% Pd/C and Na3PO4·12H2O (2.0 eq.) in 50% iPrOH at 80 °C). Iodobenzene and aryl iodides containing an electron-withdrawing group in the aromatic ring, such as nitro and acetyl functionalities and 3-iodopyridine, a π-deficient heteroaryl iodide, were smoothly coupled with a variety of both aliphatic and aromatic terminal alkynes. While the coupling of 4-iodoanisole containing an electron-donating methoxy group produced relatively lower efficiencies (38–72% yields), the coupling products were smoothly obtained. They also demonstrated the application of wet-type Pd/C to catalyze the Sonogashira coupling reaction, and the reaction proceeded efficiently under argon and aerobic conditions.
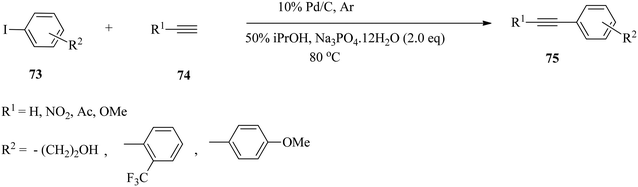 |
| Scheme 23 Copper-, ligand-, and amine-free Pd/C-catalysed Sonogashira coupling reactions of various aryl iodides with aliphatic and aromatic terminal alkynes. | |
5. Ligand-free Heck cross-coupling
The Heck reaction, a powerful method for synthesising several olefinic compounds, involves the carbon–carbon coupling of aryl halides with olefins.110–137 In the last few years, significant development involving the coupling of various pseudo-halides such as aryl triflates,111 acid chlorides,112 aryl anhydrides,113 aryl sulfonyl chlorides,114 and aromatic diazonium salts115 has been made. The synthesis of several drugs and intermediates such as naproxen (an anti-inflammatory agent),116 prosulfuron (herbicide),117 Singulair,118,119 eletriptan120,121 and 2-ethylhexyl-p-methoxycinnamate (UV B sunscreen agent)122 is carried out using the Heck reaction.123 It has also found utility in the synthesis of 2- and 4-vinyl toluene which serve as comonomers for styrene polymers.124 The catalytic system for an efficient Heck reaction consists of a palladium source, ligand, base and solvent. Usually, phosphine-containing ligands are used in the Heck reaction, as they play an essential role in stabilisation and in situ generation of Pd(0) species from Pd(II) complexes. Due to the high cost, toxicity and thermal instability of phosphine-containing ligands,125 various phosphine-free (ligand-less) catalytic systems have been developed.154–158
a. Cross-coupling reaction between 5-iodo-2-deoxyuridine and various acrylate derivatives
Len and Hervé126 reported a palladium-catalysed Heck cross-coupling reaction between 5-iodo-2-deoxyuridine and various acrylate derivatives using ligand-free conditions and microwave assistance in pure water. The reaction is particularly important because of its application for the greener total synthesis of antiviral BVDU in higher yield.126 The initial Heck cross-coupling reaction was performed using deprotected 5-iodo-2-deoxyuridine 76 and methyl acrylate 77 in the presence of Pd(II) and NEt3 in sole water at 80 °C under microwave irradiation (Scheme 24). NEt3 was chosen because it is an inexpensive joint base, and Shaughnessy demonstrated that NEt3 was able to reduce the Pd(II) pre-catalyst to a Pd(0) active species.
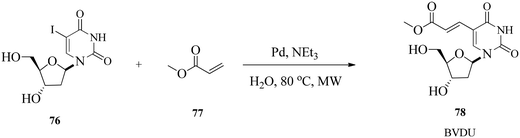 |
| Scheme 24 Ligand-free Heck coupling of 5-iodo-2-deoxyuridine with methyl acrylate in water as the sole solvent. | |
They noted that the Heck reaction is affected by the amount of the catalyst and the temperature. The palladium sources were not very efficient at 80 °C as a full reactant conversion was not achieved within 1 h. Side reactions were observed with an increase in temperature to 100 °C; hence, an increase in catalyst loading from 1.5–2 mol% to 10 mol% gave minimal side reactions. The optimal conditions were extended to the synthesis of derivatives (Scheme 25).
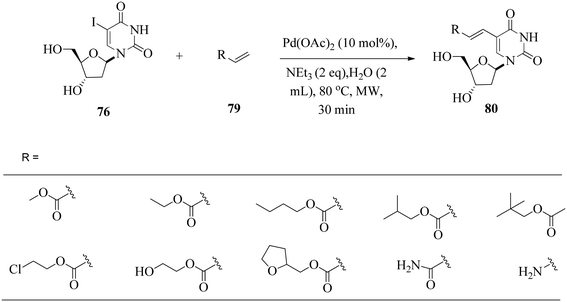 |
| Scheme 25 Ligand-free Heck coupling of 5-iodo-2-deoxyuridine with acrylate derivatives in water as the sole solvent. | |
b. Cross-coupling of halobenzene with methyl acrylate, ethyl acrylate and styrene
Biffis and co-workers127 efficiently used palladium on carbon as a catalyst for Heck C–C coupling reactions without a phosphine ligand. One of the most impressive results is the relatively high yield of stilbene 83 from the reaction of chlorobenzene 81 with styrene 82 in pure methanol in an autoclave (Scheme 26).127 Under these conditions, the added triphenylphosphine ligand acted as an inhibitor rather than a promoter.
 |
| Scheme 26 Pd/C-catalysed cross-coupling of chlorobenzene with styrene to afford stilbene. | |
In a related reaction, Kuang and co-workers128 investigated the catalytic performance of the greener ionic liquid H2O/TX-100/[BMIM]PF6 microemulsion containing Pd nanoparticles for the ligand-free Heck reaction of iodobenzene 84 with methyl acrylate, ethyl acrylate and styrene 85 (Scheme 27). Palladium chloride is dissolved in water before mixing with TX-100/[BMIM]PF6.128 The reduction of palladium chloride by the surfactant (TX-100) in the ionic liquid microemulsion occurs rapidly in situ without any further reducing agents.
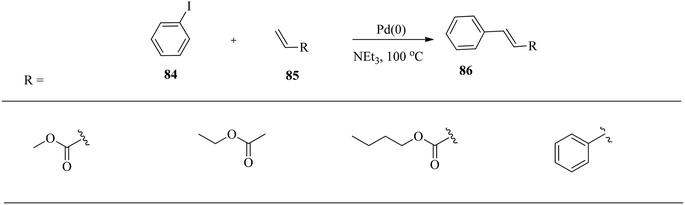 |
| Scheme 27 Product yields for other Heck reactions in an ionic liquid microemulsion. | |
c. Intermolecular Heck-type cross-coupling of aryl iodides with allylic acetates
Mariampillai and co-workers129 studied palladium-catalysed arylation of allylic acetates 88. They examined the choice of Pd catalyst and tetrabutylammonium salts in the initial coupling experiment. Optimisation of the Pd catalyst showed Pd/C to be the catalyst of choice and tetrabutylammonium chloride resulted in increased reactant conversion. The mechanism proceeds via β-acetoxy elimination, instead of β-hydride elimination, to furnish the olefinic products 89. Optimal reaction conditions were employed for ligand-free palladium on carbon coupling of various aryl iodides 87 with allyl acetate 88 in the presence of a trialkylamine base and water (Scheme 28).
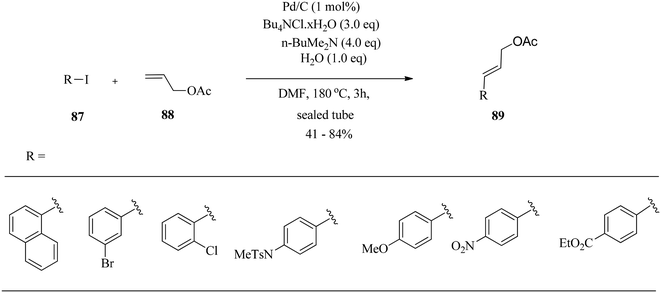 |
| Scheme 28 Heck-type coupling of allyl acetate with various aryl iodides. | |
The presence of electron-donating groups on the aryl iodide partner resulted in increased reaction times, probably due to slower oxidative addition of Pd into the aryl iodine bond.130 While electron-withdrawing substituents required a shorter reaction time, aryl chlorides and bromides were stable under the reaction conditions, providing a useful handle for further functionalization of the products. They further investigated the cross-coupling of 1-iodonaphthalene with various allylic acetates under the same reaction conditions.131
d. Heck reaction of aryl halides with alkenes
Nagarkar and co-workers130 employed palladium supported on zinc ferrite nanoparticles for Heck coupling reactions of aryl halides 90 with alkenes 91 under ligand-free conditions (Scheme 29). The efficient superparamagnetic solid catalyst was prepared by loading Pd(0) species during the synthesis of zinc ferrite nanoparticles by ultrasound assisted co-precipitation in the absence of a surface stabiliser or capping agent. The advantage of the reaction stems from the fact that an external magnet could quickly separate the nanocrystal catalyst from the reaction mixture, and the catalyst can be recycled six times without losing its activity.
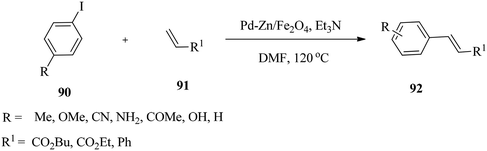 |
| Scheme 29 Pd–Zn/Fe3O4 nanoparticle-catalysed Heck coupling reactions of aryl halides with alkenes under ligand-free conditions. | |
In a related reaction, Kim and co-workers131 carried out the reaction using a Pd–Fe2O4 heterodimeric nanocrystal catalytic system and NaOAc as the base at a temperature of 110 °C for 24 h. They established the scope of the catalytic system by first screening various substituted aryl halide substrates 93 using styrene 94 or tertbutyl acrylate as an olefin counterpart (Scheme 30).131 Similarly, the heterodimer nanocrystals showed great advantages such as catalyst separation and reuse. The results showed acceptable yields from the reactions with aryl iodides having electron-rich or electron-poor substituents, except for the case of 1-iodonaphthalene which gave 46% yield. Most reactions using styrene or tert-butyl acrylate and aryl iodides gave good yields regardless of the position of the substituent, but the reactions with aryl bromides gave slightly lower yields while leaving some unreacted starting materials.
 |
| Scheme 30 Cross-coupling reactions using Pd–Fe3O4 nanocrystals. | |
e. PEG-400 supported cross-coupling of halobenzenes with methyl acrylate and styrene
In a related development, Jin and co-workers132 reported a ligand-free Heck reaction catalysed by in situ generated Pd nanoparticles in PEG-400. A variety of aryl iodides and bromides gave the cross-coupling products with good to excellent isolated yields. The cross-coupling reactions between aryl iodides 96 with electron-withdrawing groups or electron-donating groups and styrene or acrylic acid methyl ester 97 were achieved in good yields using a 1 mol% Pd loading in short times (Scheme 31).
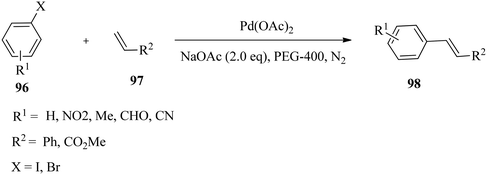 |
| Scheme 31 PEG-400/Pd(OAc)2-catalysed Heck reaction of aryl halides with olefins. | |
However, cross-coupling of aryl bromides with styrene or acrylic acid methyl ester took a more extended time. The reactivity of styrene was higher than that of acrylic acid methyl ester when using the same aryl bromide. But, no reaction was recorded between 4-bromobenzaldehyde and acrylic acid methyl ester.
f. Cross-coupling of aryldiazonium tetrafluoroborate with acrylates
Felpin and co-workers133 studied various palladium supported on charcoal (Pd/C) catalysts for the Heck reaction of aryldiazonium tetrafluoroborates 99 with acrylates 100 at room temperature in methanol (Scheme 32). Aryldiazonium tetrafluoroborate salts were prepared efficiently by the reaction of the corresponding anilines with sodium nitrite and tetrafluoroboric acid.134,135 The stable diazonium electrophiles compete favourably with less reactive and more expensive halogenated electrophiles as exemplified by reported industrial applications.136,137 Nitro-substituted diazonium salts reacted efficiently with methyl acrylate, and a high level of chemoselectivity for the diazonium group was observed with 2-bromobenzenediazonium tetrafluoroborate. While electron-poor diazonium salts reacted smoothly at 25 °C with loading as low as 0.1 mol% Pd, electron-rich substrates required higher Pd loading and eventually moderate heating activation. The use of CaCO3 as an additive proved to be beneficial in terms of yields and kinetics for less reactive arenediazonium salts, and the base could enhance the rate of the reductive elimination step.
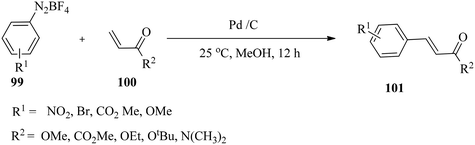 |
| Scheme 32 Heck reaction of aryldiazonium tetrafluoroborates with acrylates. | |
In a related reaction, Felpin and co-workers138 prepared cis-stilbenes 103 with good to excellent stereoselectivity by employing a Pd(OAc)2 catalyst for the Heck reaction of aryl diazonium salts 99 with 2-aryl acrylates 102 under the same conditions (Scheme 33). It was observed that the coupling furnished the cis-stilbene products with perfect control of the double bond geometry, which is unrelated to the stereoelectronic effect on the acrylates or diazonium salts. The reactions are particularly important because aryl acrylates with a NO2 group at C2 in the aromatic ring are valuable synthetic intermediates for the preparation of oxindoles and related heterocycles which are biologically active such as tenidap, etc.139,140
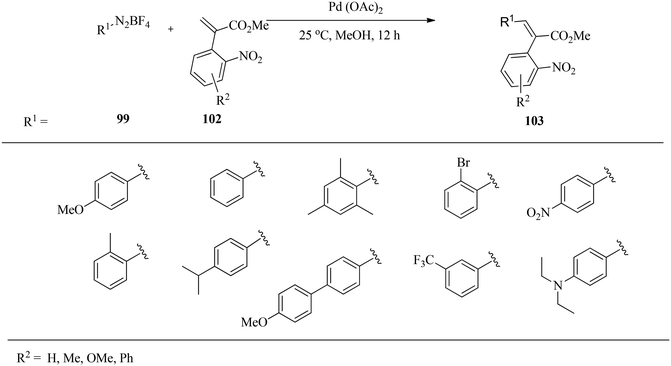 |
| Scheme 33 Preparation of various substituted stilbenes by Heck-type coupling. | |
With 2-aryl acrylate without a C2 substituent, an isomeric mixture of the product (E/Z ratio: 80/20) was obtained. However, 2-chloro-, 2-cyano-, 2-methyl-, and 2,5-dimethoxy-2-phenyl acrylates gave cis-stilbenes in high isomeric purity leading to the conclusion that 2-aryl acrylate having at least one substituent in the aromatic ring at C2 reacts with diazonium salts under palladium catalysis to give almost exclusively cis-stilbenes.139
g. Cross-coupling of aryl sulfinic acid salts with vinyl substrates
Hu and co-workers141 investigated the Pd(OAc)2-catalysed cross-coupling of aryl sulfinic acid salts 104 with vinyl substrates 105 under mild reaction conditions (Scheme 34). The high efficiency and reactivity of the aryl sulfinic acid salts could be associated with the formation of active Pd species via the release of SO2 during the catalytic cycle. The mild reaction conditions combined with the widely available sodium aryl sulfinate enable this transformation to be an attractive alternative to Heck-type cross-coupling.
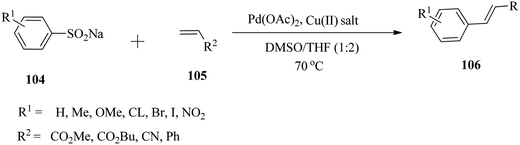 |
| Scheme 34 Pd-Catalysed Matsuda–Heck coupling of aryl sulfinic acid salts with a vinyl substrate. | |
h. Ligand-free palladium-catalysed cyanation of aryl halides
Weissman and co-workers142 described the ligand-free cyanation of aryl halides 107 using 0.1 mol% Pd(OAc)2 loading in combination with a non-toxic cyanide source, M4[Fe(CN)6], (M = K or Na). A series of aryl halides were reacted under standard conditions (Scheme 35).
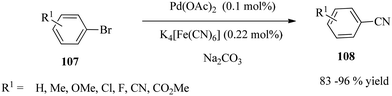 |
| Scheme 35 Ligand-free palladium-catalysed cyanation of aryl halides. | |
Rapid (≤5 h) and complete reactions were achieved for activated aryl bromides bearing electron-withdrawing groups. Moreover, electron-neutral systems and a mildly electron-rich arene, p-bromotoluene, were completely reacted within 5 h with assay yields >90%. The cyanation of an N-heteroaryl bromide (3-bromopyridine) 109 gave an 86% assay yield of the product (110) after 8 h at 120 °C (Scheme 36). High selectivity was observed for 3-bromochlorobenzene. The reaction is particularly crucial as aromatic nitriles constitute a vital component of numerous commercial compounds, including pharmaceuticals, agrochemicals (herbicides and pesticides), pigments and dyes.143
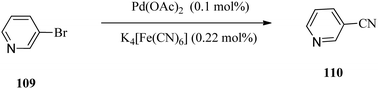 |
| Scheme 36 Ligand-free palladium-catalysed cyanation of 3-bromopyridine. | |
6. Ligand-free Stille cross-coupling reaction
Stille coupling is the palladium-catalysed cross-coupling of aryl halides or pseudohalides with organostannanes. It is one of the most potent strategies for the straightforward construction of carbon–carbon bonds in synthetic chemistry.144,145 The main advantages of the Stille coupling reaction range from high stability and functional group tolerance, the broad reaction scope of aryl halides and pseudohalides to its chemoselectivity. Therefore, the reaction has been widely applied in the synthesis of natural products146,147 and biological research.148 Stille coupling reactions are generally carried out in organic solvents under homogeneous catalysis. The development of a recyclable and reusable catalytic system is highly attractive and valuable from the viewpoints of green chemistry and practical application. Consequently, several new strategies involving heterogenized homogeneous catalysts have been developed for recycling and reusing catalysts, including the use of Pd complexes supported by silica gel,55,149 polymers,150,151 nanoparticles,152,153 porous metal–organic frameworks,154 and metal nanoparticles.155,156 Alternative approaches involving the combination of palladium complexes with several green solvents, such as ionic liquids,157,158 polyethene glycol,159,160 H2O,161–164 or H2O in the presence of surfactants,165–167 have also been applied as reusable catalytic systems.
a. Ligand-free cross-coupling of aryl bromides with organostannanes
In 2012, Prasad and Satyanarayana168 investigated the application of Fe3O4 supported Pd(0) nanoparticles (Pd/Fe3O4) for the Stille cross-coupling of aryl halides 111 with organotin compounds 112 under ligand-free conditions (Scheme 37). The best catalytic system for the cross-coupling was identified by screening the reaction with various bases in combination with different solvents.
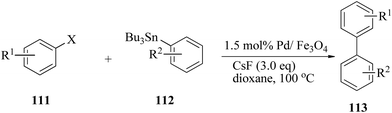 |
| Scheme 37 Pd/Fe3O4 nanoparticle-catalyzed cross-coupling of aryl halides under ligand-free conditions. | |
CsF and dioxane proved to be the best system for Pd/Fe3O4. The scope and generality of the reaction were established, applying different aryl halides 114 and butylarylstannanes 115 to obtain the corresponding biaryls 116 (Scheme 38).
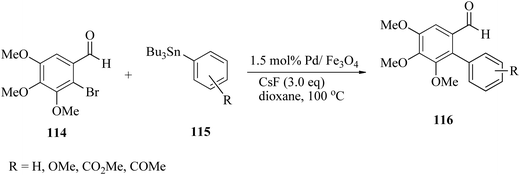 |
| Scheme 38 Fe3O4-Catalysed Stille cross-coupling of 2-bromo-3,4,5-trimethoxybenzaldehyde with different tributylarylstannanes. | |
The reaction was extended to a variety of neutral, electron-rich, and electron-poor bromoarenes 117 and substituted tributylphenylstannanes 118, and the corresponding biaryls 119 were obtained in good to excellent yields (Scheme 39). Several bioactive compounds such as allocolchicines, stegnacine and eupomatilones were prepared from 2-bromo-3,4,5-trimethoxy benzaldehyde as an aryl halide.168
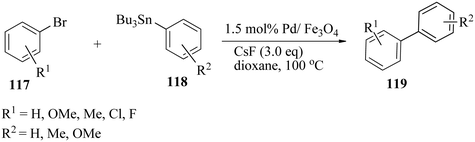 |
| Scheme 39 Pd/Fe3O4-catalysed Stille cross-coupling of different bromoarenes with different tributylarylstannanes. | |
7. Ligand-free Negishi cross-coupling
Among all the coupling reactions, the Negishi reaction has found to be a potent tool for the preparation of many chemical and pharmaceutically active compounds due to its compatibility with various functional groups, high chemoselectivity and excellent stereoselectivity of organozinc reagents, and easy preparation of a wide variety of organozinc halides (RZnX) and organozinc (R2Zn) compounds.35,169–172 This reaction is usually catalysed by nickel or palladium complexes, and ancillary ligands are traditionally needed to sufficiently enhance the reactivities of the palladium and nickel catalysts. Inexpensive nickel has positively received the researcher's attention from the very beginning due to the high cost of palladium precursors. Recently, Negishi cross-coupling reactions employing ligand-free conditions have been adapted for greener and economical synthesis of various products.
a. Cross-coupling of alkyl-, aryl-, and alkynyl zinc reagents with heteroaryl iodides
Recently, Thapa et al. in 2015173 reported a ligand-free, copper-catalysed Negishi cross-coupling reaction of various zinc reagents 120 with heteroaryl iodides 121 (Scheme 40). The reaction proceeds within three hours at room temperature for alkylzinc reagents; however, higher temperatures are required for coupling with aryl- and alkynyl zinc reagents to give the desired coupling products 122 in very high yields.
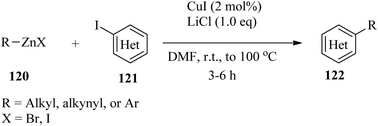 |
| Scheme 40 Coupling with alkyl, alkynyl or arylzinc reagents with (hetero)aryl halides. | |
b. Cross-coupling of 6-chloropurines with organozinc halides
Wang and co-workers174 developed an efficient method for the synthesis of 6-alkyl or 6-aryl purines (nucleosides) 125via nickel-catalysed Negishi cross-coupling reactions of 6-chloropurines 123 and organozinc halides 124. The optimised reaction conditions were employed in the cross-coupling reaction of several 6-chloropurines 123 with various substituents at N-9, including alkyl and sugar carbon substituents (Scheme 41).
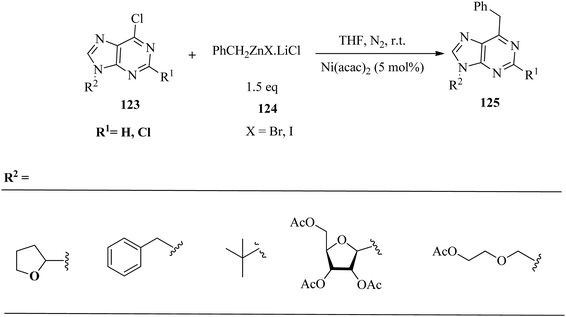 |
| Scheme 41 The cross-coupling reaction of 6-chloropurines with benzyl zinc bromide. | |
The reaction of N-9 alkyl or tetrahydropyranyl-substituted purines proceeded smoothly in more than 97% yields, whereas 2,3,5-triacetyl-6-chloropurine nucleoside gave the corresponding product in 80% isolated yield which serves as a useful access for the preparation of purine nucleoside analogues. Moreover, the cross-coupling reaction employing acetyl protected 2,6-dichloropurine nucleoside was able to selectively produce 6-benzyl-2-chloropurine nucleoside and 2,6-dibenzylpurine nucleoside by varying the quantity of benzyl zinc bromide. The same result was obtained for acyclovir side-chain protected 2,6-dichloropurine.
Other alkylzinc halides were also subjected to the reaction under the optimised conditions (Scheme 42). The primary alkylzinc halides 126, such as benzyl zinc, methylzinc, and pentylzinc, produced the corresponding products 127 in good to excellent yields. Cyclic alkyl zinc bromides, cyclopentylzinc and cyclohexylzinc gave the desired products in satisfactory yields. However, isopropyl zinc bromide gave a mixture of products due to rearrangement.174
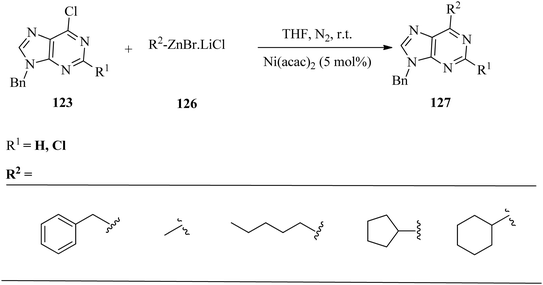 |
| Scheme 42 The cross-coupling reaction of 9-benzyl-6-chloro-purine with various alkylzinc halides. | |
Testing of the optimised reaction conditions on arylzinc chlorides gave low yields; however, satisfactory yields of the aryl and substituted arylpurines 129 were obtained with the corresponding iodides 128 (Scheme 43).
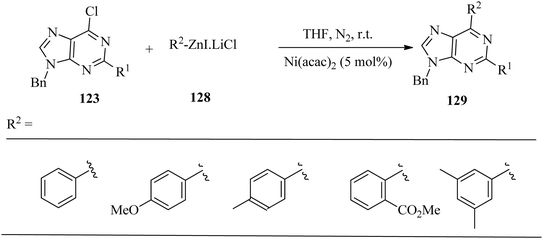 |
| Scheme 43 The Negishi cross-coupling reaction of 9-benzyl-6-chloro-purine with arylzinc iodide. | |
8. Mechanism of ligand-free cross-coupling
How can a pure ligand-free Pd-catalysed system be understood, given that it is generally believed that phosphine ligands exert stabilising and activating effects? To proffer an answer to this question, Reetz and Westermann reported a mechanistic study supported by transmission electron microscopy (TEM) of three phosphine-free catalytic systems, namely, Pd(OAc)2/DMG, Pd(OAc)2/(nBu)4N+Cl− and Pd(OAc)2 for Heck and Suzuki–Miyaura cross-coupling reactions.175 The result disclosed the presence of an in situ generated nano-sized Pd colloid as the active species in the phosphine-free reactions. The authors predicted the active catalyst in other related C–C forming reactions in the presence of Pd salts without a unique ligand to be nanosized Pd colloids. Nano-sized Pd colloids are highly active atoms as a result of their high surface-to-volume ratio compared to molecular Pd catalysts. Several polymers and ionic liquids stabilise these palladium colloids such as polyaniline and tetraalkylammonium salts. In recent years, numerous investigations have been made to ascertain whether the mechanism of ligand-free/nanoparticle-catalysed cross-coupling reactions is homogenous or heterogeneous.176–178 Claver et al. summarised the two major mechanisms that were proposed.179 One, palladium nanoparticles are real heterogeneous catalysts while the second says palladium nanoparticles act as a reservoir of molecular species which leached out ‘naked palladium atoms’. Lorenzo, in his critical and comprehensive investigation of the two mechanisms in palladium nanoparticle-catalysed Suzuki cross-coupling, stated that ‘rather than being mutually exclusive, both mechanisms should be considered as complementary and dependent on the immobilisation degree of the palladium nanoparticles’.180 More recently, reinvestigation of the transmetalation and reductive steps of ligand-free SMC cross-coupling of aryl halides using first-principles molecular dynamics simulations with an explicit solvent model showed that PdX− was the active species of the catalyst. The origin of the reduction of catalytic reactivity for aryl halides was attributed to the more substantial activation barrier in the oxidative addition step which causes aggregation of Pd catalysts.181 While ligand-less Pd-catalysed cross-coupling reactions are often conducted at a relatively faster rate than ligand-supported ones, the aggregation or agglomeration of Pd(0), which results in incomplete conversion of substrates, is a disadvantage to their applications, especially to cross-coupling of aryl chlorides and sterically hindered substrates.182
9. Conclusion
Ligand-free metal-catalysed cross-coupling is a cost effective and environmentally benign protocol for functionalization of (hetero)aryl halides and pseudohalides. Though it has achieved relative success in the transformation of aryl iodides and bromides, the cross-coupling of electron-rich/sterically encumbered aryl chlorides and heterocyclic substrates remains a big challenge. There have been steady efforts over the years to apply ligand-free protocols in cross-coupling of low reactivity aryl chlorides; however, the catalytic efficiency cannot be compared with ligand-supported catalysts. This is because the stereochemical requirement needed by the ligand with its electron donor groups to increase the electron density in the metal centre which activates oxidative addition step and the bulkiness of the ligand which facilitates the reductive elimination step conspicuously is lacking in nanoparticles.183 In various examples of ligand-free Pd-catalysed cross-coupling, electron-deficient aryl halides are more reactive than electron-rich ones under similar conditions but they could benefit tremendously from microwave-assisted heating.184
Therefore, the primary concern of ligand-free cross-coupling reactions remains finding suitable catalyst systems with low loading, reduced cost owing to their simple separation and multiple usages, less contamination of the product, least side reactions and environmentally friendly and benign solvents. In conclusion, the extensive applications of ligand-free cross-coupling reactions in Suzuki–Miyaura cross-coupling, Sonogashira cross-coupling, Hiyama cross-coupling, Heck reaction, Stille cross-coupling and Negishi cross-coupling have been highlighted and will remain economical and environmentally friendly protocols for future researchers in functionalization of a wide variety of compounds with industrial and therapeutic applications.
Conflicts of interest
There are no conflicts to declare.
References
- Y. Chen, W. Lai, L. Xie and W. Huang, Palladium-catalysed cross-couplings in organic synthesis: An introduction to the 2010 Nobel Prize in Chemistry, Chin. Sci. Bull., 2011, 56(13), 995–1006 CrossRef.
- H.-F. Zhang, Copper-catalysed ligand-free Suzuki–Miyaura coupling reaction of aryl halides with arylboronic acid, J. Med. Chem. Sci., 2019, 2(1), 1–40 Search PubMed , 38–40.
- L. Rocard and P. Hudhomme, Recent developments in the Suzuki–Miyaura reaction using nitroarenes as electrophilic coupling reagents, Catalysts, 2019, 9(3), 213 CrossRef.
- E. A. Onoabedje and U. C. Okoro, Ligand-supported palladium-catalysed cross-coupling reactions of (hetero) aryl chlorides, Synth. Commun., 2019, 49(17), 2117–2146 CrossRef CAS.
- M. Lamblin, L. Nassar-Hardy, J. C. Hierso, E. Fouquet and F. X. Felpin, Recyclable heterogeneous palladium catalysts in pure water: Sustainable developments in Suzuki, Heck, Sonogashira and Tsuji–Trost reactions, Adv. Synth. Catal., 2010, 352(1), 33–79 CrossRef CAS.
-
A. O. King and N. Yasuda, Palladium-catalysed cross-coupling reactions in the synthesis of pharmaceuticals, in Organometallics in Process Chemistry, Springer, 2004, pp. 205–245 Search PubMed.
- J. Magano, Large-scale applications of transition metal-catalysed couplings for the synthesis of pharmaceuticals, Chem. Rev., 2011, 111(3), 2177–2250 CrossRef CAS PubMed.
- N. Miyaura, A new stereospecific cross-coupling by the palladium-catalysed reaction of 1-alkenylboranes with 1-alkenyl or 1-alkynyl halides, Tetrahedron Lett., 1979, 20(36), 3437–3440 CrossRef.
- R. K. Arvela, A Re-assessment of the transition-metal-free Suzuki-type coupling methodology, J. Org. Chem., 2005, 70(1), 161–168 CrossRef CAS.
- P. Ruiz-Castillo and S. L. Buchwald, Applications of palladium-catalysed C–N cross-coupling reactions, Chem. Rev., 2016, 116(19), 12564–12649 CrossRef CAS.
- C. L. Allen and J. M. Williams, Metal-catalysed approaches to amide bond formation, Chem. Soc. Rev., 2011, 40(7), 3405–3415 RSC.
- N. A. Weires, E. L. Baker and N. K. Garg, Nickel-catalysed Suzuki-Miyaura coupling of amides, Nat. Chem., 2016, 8(1), 75–79 CrossRef CAS.
- C. Liu and M. Szostak, Twisted amides: From obscurity to broadly useful transition-metal-catalysed reactions by N-C amide bond activation, Chemistry, 2017, 23(30), 7157–7173 CrossRef CAS.
- S. Guo, J. C. Yang and S. L. Buchwald, A practical electrophilic nitrogen source for the synthesis of chiral primary amines by copper-catalysed hydroamination, J. Am. Chem. Soc., 2018, 140(46), 15976–15984 CrossRef CAS.
- Y. Bai, J. Chen and S. C. Zimmerman, Designed transition metal catalysts for intracellular organic synthesis, Chem. Soc. Rev., 2018, 47(5), 1811–1821 RSC.
- T. Willemse, W. Schepens, H. W. van Vlijmen, B. U. Maes and S. Ballet, The Suzuki–Miyaura cross-coupling as a versatile tool for peptide diversification and cyclization, Catalysts, 2017, 7(3), 74 CrossRef.
- J. I. Ayogu, B. E. Ezema and C. G. Ezema, Utility of Suzuki-Miyaura cross-coupling reaction in synthesis of benzo[a]phenothiazine and benzo[a]phenoxazine rerivatives and their antimicrobial screening, Asian J. Chem., 2015, 27(12), 4467–4473 CrossRef CAS.
- C. Ike, B. Ezema, J. Ayogu, D. Ugwu, C. Ezema, A. Nwasi and E. Adekola, Synthesis and antimicrobial screening of novel benzoxazinophenothiazine derivatives, Asian J. Chem., 2015, 27(11), 4115–4119 CrossRef CAS.
- N. Eleya, I. Malik, S. Reimann, K. Wittler, M. Hein, T. Patonay, A. Villinger, R. Ludwig and P. Langer, Efficient synthesis of frylated flavones by site-selective Suzuki–Miyaura cross-coupling reactions of the bis(triflate) of 5,7- and 7,8-dihydroxyflavone, Eur. J. Org. Chem., 2012, 2012(8), 1639–1652 CrossRef CAS.
- R. E. Whittaker, A. Dermenci and G. Dong, Synthesis of ynones and recent application in transition-metal-catalysed
reactions, Synthesis, 2016, 48(02), 161–183 CAS.
- R. Skoda-Földes and L. Kollár, Transition-metal-catalysed reactions in steroid synthesis, Chem. Rev., 2003, 103(10), 4095–4130 CrossRef.
- Q. Liang, P. Xing, Z. Huang, J. Dong, K. B. Sharpless, X. Li and B. Jiang, Palladium-catalysed, ligand-free Suzuki reaction in water using aryl fluorosulfates, Org. Lett., 2015, 17(8), 1942–1945 CrossRef CAS.
- J. P. Simeone and J. R. Sowa, Palladium on carbon as a precatalyst for the Suzuki–Miyuara cross-coupling of aryl chlorides, Tetrahedron, 2007, 63(51), 12646–12654 CrossRef CAS.
- N. Bumagin, P. More and I. Beletskaya, Synthesis of substituted cinnamic acids and cinnamonitriles via palladium catalysed coupling reactions of aryl halides with acrylic acid and acrylonitrile in aqueous media, J. Organomet. Chem., 1989, 371(3), 397–401 CrossRef CAS.
- Y. Ben-David, M. Portnoy and D. Milstein, Chelate-assisted, palladium-catalysed efficient carbonylation of aryl chlorides, J. Am. Chem. Soc., 1989, 111(23), 8742–8744 CrossRef CAS.
- C. R. LeBlond, A. T. Andrews, Y. Sun and J. R. Sowa, Activation of aryl chlorides for Suzuki cross-coupling by ligandless, heterogeneous palladium, Org. Lett., 2001, 3(10), 1555–1557 CrossRef CAS.
- N. A. Bumagin and V. V. Bykov, Ligandless palladium catalysed reactions of arylboronic acids and sodium tetraphenylborate with aryl halides in aqueous media, Tetrahedron, 1997, 53(42), 14437–14450 CrossRef CAS.
- N. Miyaura and A. Suzuki, Palladium-catalysed cross-coupling reactions of organoboron compounds, Chem. Rev., 1995, 95(7), 2457–2483 CrossRef CAS.
- A. Kumbhar, S. Jadhav, S. Kamble, G. Rashinkar and R. Salunkhe, Palladium supported hybrid cellulose–aluminum oxide composite for Suzuki–Miyaura cross coupling reaction, Tetrahedron Lett., 2013, 54(11), 1331–1337 CrossRef CAS.
- Y. Feng, X. Jiang and J. K. De Brabander, Studies toward the unique pederin family member psymberin: full structure elucidation, two alternative total syntheses, and analogs, J. Am. Chem. Soc., 2012, 134(41), 17083–17093 CrossRef CAS.
- S. N. Jadhav, A. S. Kumbhar, S. S. Mali, C. K. Hong and R. S. Salunkhe, A Merrifield resin supported Pd–NHC complex with a spacer (Pd–NHC@SP–PS) for the Sonogashira coupling reaction under copper-and solvent-free conditions, New J. Chem., 2015, 39(3), 2333–2341 RSC.
- Y. Nakao and T. Hiyama, Silicon-based cross-coupling reaction: an environmentally benign version, Chem. Soc. Rev., 2011, 40(10), 4893–4901 RSC.
-
J. Williams, Preparation of alkenes: A practical approach, Oxford University Press, 1996 Search PubMed.
- S. Jadhav, A. Kumbhar and R. Salunkhe, Palladium supported on silica-chitosan hybrid material (Pd-CS@SiO2) for Suzuki-Miyaura and Mizoroki-Heck cross-coupling reactions, Appl. Organomet. Chem., 2015, 29(6), 339–345 CrossRef CAS.
- C. Han and S. L. Buchwald, Negishi coupling of secondary alkylzinc halides with aryl bromides and chlorides, J. Am. Chem. Soc., 2009, 131(22), 7532–7533 CrossRef CAS.
- J. K. Stille, The palladium-catalysed cross-coupling reactions of organotin reagents with organic electrophiles [new synthetic methods (58)], Angew. Chem., Int. Ed. Engl., 1986, 25(6), 508–524 CrossRef.
- J. Hassan, M. Sevignon, C. Gozzi, E. Schulz and M. Lemaire, Aryl-aryl bond formation one century after the discovery of the Ullmann reaction, Chem. Rev., 2002, 102(5), 1359–1470 CrossRef CAS.
- I. Hussain, Synthesis of biaryls ligand-free Suzuki-Miyaura cross-coupling reactions: A review of homogeneous and heterogeneous catalytic developments, Adv. Synth. Catal., 2016, 358(21), 3320–3349 CrossRef CAS.
- N. Miyaura and A. J. C. R. Suzuki, Palladium-catalysed cross-coupling reactions of organoboron compounds, Chem. Rev., 1995, 95(7), 2457–2483 CrossRef CAS.
- I. Maluenda and O. Navarro, Recent developments in the Suzuki-Miyaura reaction: 2010–2014, Molecules, 2015, 20(5), 7528–7557 CrossRef CAS.
- J. P. Genet and M. Savignac, Recent developments of palladium(0) catalysed reactions in aqueous medium, J. Organomet. Chem., 1999, 576(1–2), 305–317 CrossRef.
- H. Sakurai, T. Tsukuda and T. Hirao, Pd/C as a reusable catalyst for the coupling reaction of halophenols and arylboronic acids in aqueous media, J. Org. Chem., 2002, 67(8), 2721–2722 CrossRef CAS.
- D. Badone, M. Baroni, R. Cardamone, A. Ielmini and U. Guzzi, Highly efficient palladium-catalysed boronic acid coupling reactions in water: scope and limitations, J. Org. Chem., 1997, 62(21), 7170–7173 CrossRef CAS PubMed.
- L. Botella and C. Nájera, A convenient oxime-carbapalladacycle-catalysed Suzuki cross-coupling of aryl chlorides in water, Angew. Chem., Int. Ed., 2002, 41(1), 179–181 CrossRef CAS.
- K. H. Shaughnessy and R. S. Booth, Sterically demanding, water-soluble alkylphosphines as ligands for high activity Suzuki coupling of aryl bromides in aqueous solvents, Org. Lett., 2001, 3(17), 2757–2759 CrossRef CAS.
- C. Dupuis, K. Adiey, L. Charruault, V. Michelet, M. Savignac and J.-P. Genêt, Suzuki cross-coupling of arylboronic acids mediated by a hydrosoluble Pd(0)/TPPTS catalyst, Tetrahedron Lett., 2001, 42(37), 6523–6526 CrossRef CAS.
- C. R. LeBlond, A. T. Andrews, Y. Sun and J. R. J. O. l. Sowa, Activation of aryl chlorides for Suzuki cross-coupling by ligandless, heterogeneous palladium, Org. Lett., 2001, 3(10), 1555–1557 CrossRef CAS.
- M. Larhed and A. Hallberg, Microwave-promoted palladium-catalysed coupling reactions, J. Org. Chem., 1996, 61(26), 9582–9584 CrossRef CAS.
- C. G. Blettner, W. A. König, W. Stenzel and T. Schotten, Microwave-assisted aqueous Suzuki cross-coupling reactions, J. Org. Chem., 1999, 64(11), 3885–3890 CrossRef CAS.
- C. G. Blettner, W. A. König, W. Stenzel and T. Schotten, Microwave-assisted aqueous Suzuki cross-coupling reactions, J. Org. Chem., 1999, 64(11), 3885–3890 CrossRef CAS.
-
E.-I. Negishi and A. de Meijere, Handbook of organopalladium chemistry for organic synthesis, Wiley-Interscience, 2002, vol. 2 Search PubMed.
- R. Martin and S. L. Buchwald, Palladium-catalysed Suzuki-Miyaura cross-coupling reactions employing dialkylbiaryl phosphine ligands, Acc. Chem. Res., 2008, 41(11), 1461–1473 CrossRef CAS PubMed.
- C. Liu, Q. Ni, F. Bao and J. Qiu, A simple and efficient protocol for a palladium-catalysed ligand-free Suzuki reaction at room temperature in aqueous DMF, Green Chem., 2011, 13(5), 1260–1266 RSC.
- A. Thakur, K. Zhang and J. Louie, Suzuki-Miyaura coupling of heteroaryl boronic acids and vinyl chlorides, Chem. Commun., 2012, 48(2), 203–205 RSC.
- D.-H. Lee, J.-Y. Jung and M.-J. Jin, Highly active and recyclable silica gel-supported palladium catalyst for mild cross-coupling reactions of unactivated heteroaryl chlorides, Green Chem., 2010, 12(11), 2024–2029 RSC.
- K. L. Billingsley, K. W. Anderson and S. L. Buchwald, A Highly active catalyst for Suzuki–Miyaura cross-coupling reactions of heteroaryl compounds, Angew. Chem., Int. Ed., 2006, 45(21), 3484–3488 CrossRef CAS.
- P. Zhou, H. Wang, J. Yang, J. Tang, D. Sun and W. Tang, Bio-supported palladium nanoparticles as a phosphine-free catalyst for the Suzuki reaction in water, RSC Adv., 2012, 2(5), 1759–1761 RSC.
- N. E. Leadbeater and M. Marco, Ligand-free palladium catalysis of the Suzuki reaction in water using microwave heating, Org. Lett., 2002, 4(17), 2973–2976 CrossRef CAS.
- S.-J. Kim, S.-D. Oh, S. Lee and S.-H. Choi, Radiolytic synthesis of Pd-M (M=Ag, Ni, and Cu)/C catalyst and their use in Suzuki-type and Heck-type reaction, J. Ind. Eng. Chem., 2008, 14(4), 449–456 CrossRef CAS.
- A. Alimardanov, L. Schmieder-van de Vondervoort, A. H. de Vries and J. G. de Vries, Use of “homeopathic” ligand-free palladium as catalyst for aryl-aryl coupling reactions, Adv. Synth. Catal., 2004, 3346(13–15), 1812–1817 CrossRef.
-
S. E. Denmark and R. F. Sweis, Organosilicon compounds in cross-coupling reactions, Metal-catalysed cross-coupling reactions, 2004, pp. 163–216 Search PubMed.
- A. C. Spivey, C. J. Gripton and J. P. Hannah, Recent advances in group 14 cross-coupling: Si and Ge-based alternatives to the Stille reaction, Curr. Org. Synth., 2004, 1(3), 211–226 CrossRef CAS.
- S. E. Denmark and C. S. Regens, Palladium-catalysed cross-coupling reactions of organosilanols and their salts: practical alternatives to boron-and tin-based methods, Acc. Chem. Res., 2008, 41(11), 1486–1499 CrossRef CAS.
- O. Y. Yuen, C. M. So, H. W. Man and F. Y. Kwong, A general palladium-catalysed Hiyama cross-coupling reaction of aryl and heteroaryl chlorides, Chem. – Eur. J., 2016, 22(19), 6471–6476 CrossRef CAS PubMed.
- K. Shibata, K. Miyazawa and Y. Goto, Preparation of functionalised biaryl compounds via cross-coupling reactions of aryltrialkoxysilanes with arylbromides, Chem. Commun., 1997,(14), 1309–1310 RSC.
- H. F. Sore, W. R. Galloway and D. R. Spring, Palladium-catalysed cross-coupling of organosilicon reagents, Chem. Soc. Rev., 2012, 41(5), 1845–1866 RSC.
- L. Ackermann, C. J. Gschrei, A. Althammer and M. Riederer, Cross-coupling reactions of aryl and vinyl chlorides catalysed by a palladium complex derived from an air-stable H-phosphonate, Chem. Commun., 2006,(13), 1419–1421 RSC.
- C. Dash, M. M. Shaikh and P. Ghosh, Fluoride-free Hiyama and copper- and amine-free Sonogashira coupling in air in a mixed aqueous medium by a series of PEPPSI-themed precatalysts, Eur. J. Inorg. Chem., 2009, 2009(12), 1608–1618 CrossRef.
- J. Yang and L. Wang, Synthesis and characterization of dinuclear NHC–palladium complexes and their applications in the Hiyama reactions of aryltrialkyoxysilanes with aryl chlorides, Dalton Trans., 2012, 41(39), 12031–12037 RSC.
- S. Modak, M. K. Gangwar, M. N. Rao, M. Madasu, A. C. Kalita, V. Dorcet, M. A. Shejale, R. J. Butcher and P. Ghosh, Fluoride-free Hiyama coupling by palladium abnormal N-heterocyclic carbene complexes, Dalton Trans., 2015, 44(40), 17617–17628 RSC.
- J.-H. Li, C.-L. Deng, W.-J. Liu and Y.-X. Xie, Pd(OAc)2/DABCO as an inexpensive and efficient catalytic system for Hiyama cross-coupling reactions of aryl halides with aryltrimethoxysilanes, Synthesis, 2005, 2005(18), 3039–3044 CrossRef.
- T. Yanase, Y. Monguchi and H. Sajiki, Ligand-free Hiyama cross-coupling reaction catalysed by palladium on carbon, RSC Adv., 2012, 2(2), 590–594 RSC.
- B. C. Ranu, R. Dey and K. Chattopadhyay, A one-pot efficient and fast Hiyama coupling using palladium nanoparticles in water under fluoride-free conditions, Tetrahedron Lett., 2008, 49(21), 3430–3432 CrossRef CAS.
- D. Srimani, S. Sawoo and A. Sarkar, Convenient synthesis of palladium nanoparticles and catalysis of Hiyama coupling reaction in water, Org. Lett., 2007, 9(18), 3639–3642 CrossRef CAS.
- R. Dey, K. Chattopadhyay and B. C. Ranu, Palladium(0) nanoparticle catalysed cross-coupling of allyl acetates and aryl and vinyl siloxanes, J. Org. Chem., 2008, 73(23), 9461–9464 CrossRef CAS PubMed.
- L. D. Pachón, M. B. Thathagar, F. Hartl and G. Rothenberg, Palladium-coated nickel nanoclusters: new Hiyama cross-coupling catalysts, Phys. Chem. Chem. Phys., 2006, 8(1), 151–157 RSC.
- R. W. Scott, H. Ye, R. R. Henriquez and R. M. Crooks, Synthesis, characterization, and stability of dendrimer-encapsulated palladium nanoparticles, Chem. Mater., 2003, 15(20), 3873–3878 CrossRef CAS.
- K. Sonogashira, Y. Tohda and N. Hagihara, A convenient synthesis of acetylenes: catalytic
substitutions of acetylenic hydrogen with bromoalkenes, iodoarenes and bromopyridines, Tetrahedron Lett., 1975, 16(50), 4467–4470 CrossRef.
- R. Chinchilla and C. Nájera, Recent advances in Sonogashira reactions, Chem. Soc. Rev., 2011, 40(10), 5084–5121 RSC.
- M. Eckhardt and G. C. Fu, The first applications of carbene ligands in cross-couplings of alkyl electrophiles: Sonogashira reactions of unactivated alkyl bromides and iodides, J. Am. Chem. Soc., 2003, 125(45), 13642–13643 CrossRef CAS PubMed.
- A. Soheili, J. Albaneze-Walker, J. A. Murry, P. G. Dormer and D. L. Hughes, Efficient and general protocol for the copper-free Sonogashira coupling of aryl bromides at room temperature, Org. Lett., 2003, 5(22), 4191–4194 CrossRef CAS PubMed.
- J. W. Grissom, G. U. Gunawardena, D. Klingberg and D. Huang, The chemistry of enediynes, enyne allenes and related compounds, Tetrahedron, 1996, 52(19), 6453–6518 CrossRef CAS.
- A. P. Thankachan, K. Sindhu, K. K. Krishnan and G. Anilkumar, An efficient zinc-catalysed cross-coupling reaction of aryl iodides with terminal aromatic alkynes, Tetrahedron Lett., 2015, 56(41), 5525–5528 CrossRef CAS.
- K. Nicolaou and W. M. Dai, Chemistry and biology of the enediyne anticancer antibiotics, Angew. Chem., Int. Ed. Engl., 1991, 30(11), 1387–1416 CrossRef.
- M. De Kort, V. Correa, A. R. P. Valentijn, G. A. Van Der Marel, B. V. Potter, C. W. Taylor and J. H. Van Boom, Synthesis of potent agonists of the d-myo-Inositol 1,4,5-trisphosphate receptor based on clustered disaccharide polyphosphate analogues of adenophostin A, J. Med. Chem., 2000, 43(17), 3295–3303 CrossRef CAS PubMed.
-
K. T. Nielsen, Light harvesting by dye linked conducting polymers, 2006 Search PubMed.
- S. Huang and J. M. Tour, Rapid bi-directional synthesis of oligo (1,4-phenylene ethynylene) s, Tetrahedron Lett., 1999, 40(17), 3447–3450 CrossRef.
- V. Francke, T. Mangel and K. Müllen, Synthesis of α,ω-difunctionalized oligo-and poly (p-phenyleneethynylene)s, Macromolecules, 1998, 31(8), 2447–2453 CrossRef CAS.
- A. Molnar, Efficient, selective, and recyclable palladium catalysts in carbon-carbon coupling reactions, Chem. Rev., 2011, 111(3), 2251–2320 CrossRef CAS PubMed.
- A. Thayer, Removing impurities, Chem. Eng. News, 2005, 83(36), 55–58 CrossRef.
- C. E. Garrett and K. Prasad, The art of meeting palladium specifications in active pharmaceutical ingredients produced by Pd-catalysed reactions, Adv. Synth. Catal., 2004, 346(8), 889–900 CrossRef CAS.
- C.-J. Li, Organic reactions in aqueous media with a focus on carbon-carbon bond formations: a decade update, Chem. Rev., 2005, 105(8), 3095–3166 CrossRef CAS PubMed.
-
A. Lubineau and J. Augé, Water as solvent in organic synthesis, in Modern solvents in organic synthesis, Springer, 1999, pp. 1–39 Search PubMed.
- S. Lohmann, S. P. Andrews, B. J. Burke, M. D. Smith, J. P. Attfield, H. Tanaka, K. Kaneko and S. V. Ley, Copper-and palladium-containing perovskites: catalysts for the Ullmann and Sonogashira reactions, Synlett, 2005, 2005(08), 1291–1295 CrossRef.
- A. L. D. Ramos, P. da Silva Alves, D. A. Aranda and M. Schmal, Characterization of carbon supported palladium catalysts: inference of electronic and particle size effects using reaction probes, Appl. Catal., A, 2004, 277(1–2), 71–81 CrossRef.
- S. Raju, P. R. Kumar, K. Mukkanti, P. Annamalai and M. Pal, Facile synthesis of substituted thiophenes via Pd/C-mediated sonogashira coupling in water, Bioorg. Med. Chem. Lett., 2006, 16(24), 6185–6189 CrossRef CAS PubMed.
- A. Corma, H. García and A. Primo, Palladium and copper supported on mixed oxides derived from hydrotalcite as reusable solid catalysts for the Sonogashira coupling, J. Catal., 2006, 241(1), 123–131 CrossRef CAS.
- C. M. Crudden, M. Sateesh and R. Lewis, Mercaptopropyl-modified mesoporous silica: A remarkable support for the preparation of a reusable, heterogeneous palladium catalyst for coupling reactions, J. Am. Chem. Soc., 2005, 127(28), 10045–10050 CrossRef CAS PubMed.
- K. R. Reddy, N. S. Kumar, P. S. Reddy, B. Sreedhar and M. L. Kantam, Cellulose supported palladium(0) catalyst for Heck and Sonogashira coupling reactions, J. Mol. Catal. A: Chem., 2006, 252(1–2), 12–16 CrossRef CAS.
- A. C. Albéniz and N. Carrera, Polymers for green C–C couplings, Eur. J. Inorg. Chem., 2011, 2011(15), 2347–2360 CrossRef.
- N. E. Leadbeater, Fast, easy, clean chemistry by using water as a solvent and microwave heating: the Suzuki coupling as an illustration, Chem. Commun., 2005,(23), 2881–2902 RSC.
- B. M. Dioos, I. F. Vankelecom and P. A. Jacobs, Aspects of immobilisation of catalysts on polymeric supports, Adv. Synth. Catal., 2006, 348(12–13), 1413–1446 CrossRef CAS.
- W.-B. Yi, X.-L. Shi, C. Cai and W. Zhang, Polymer-supported Pd(0) catalyst for copper-and ligand-free Sonogashira reactions in aqueous media, Green Processes Synth., 2012, 1(2), 175–180 CAS.
- N. K. Garg, C. C. Woodroofe, C. J. Lacenere, S. R. Quake and B. M. Stoltz, A ligand-free solid-supported system for Sonogashira couplings: applications in nucleoside chemistry, Chem. Commun., 2005,(36), 4551–4553, 10.1039/B505737J.
- Z. Novák, A. Szabó, J. Répási and A. Kotschy, Sonogashira coupling of aryl halides catalysed by palladium on charcoal, J. Org. Chem., 2003, 68(8), 3327–3329 CrossRef PubMed.
- J. M. Prober, A system for rapid DNA sequencing with fluorescent chain-terminating dideoxynucleotides, Science, 1987, 238(4825), 336–341 CrossRef CAS PubMed.
- M. B. Thathagar, J. Beckers and G. Rothenberg, Palladium-free and ligand-free Sonogashira cross-coupling, Green Chem., 2004, 6(4), 215–218 RSC.
- X. Li, X. Gong, Z. Li, H. Chang, W. Gao and W. Wei, Ligand- and copper-free Sonogashira and Heck couplings of (het)aryl chlorides and bromides catalysed by palladium nanoparticles supported on in situ generated Al(OH)3, RSC Adv., 2017, 7(5), 2475–2479, 10.1039/C6RA25416K.
- S. Mori, T. Yanase, S. Aoyagi, Y. Monguchi, T. Maegawa and H. Sajiki, Ligand-free Sonogashira coupling reactions with heterogeneous Pd/C as the catalyst, Chem. – Eur. J., 2008, 14(23), 6994–6999 CrossRef CAS PubMed.
- I. P. Beletskaya and A. V. Cheprakov, The Heck reaction as a sharpening stone of palladium catalysis, Chem. Rev., 2000, 100(8), 3009–3066 CrossRef CAS PubMed.
- D. E. Frantz, D. G. Weaver, J. P. Carey, M. H. Kress and U. H. Dolling, Practical synthesis of aryl triflates under aqueous conditions, Org. Lett., 2002, 4(26), 4717–4718 CrossRef CAS PubMed.
- H.-U. Blaser and A. Spencer, The palladium-catalysed arylation of activated alkenes with aroyl chlorides, J. Organomet. Chem., 1982, 233(2), 267–274 CrossRef CAS.
- M. S. Stephan, A. J. Teunissen, G. K. Verzijl and J. G. de Vries, Heck reactions without salt formation: aromatic carboxylic anhydrides as arylating agents, Angew. Chem., Int. Ed., 1998, 37(5), 662–664 CrossRef CAS PubMed.
- M. Miura, H. Hashimoto, K. Itoh and M. Nomura, Palladium-catalysed desulphonylative vinylation of arenesulphonyl chlorides under solid–liquid phase-transfer conditions, J. Chem. Soc., Perkin Trans. 1, 1990,(8), 2207–2211 RSC.
- S. Cacchi, G. Fabrizi, A. Goggiamani and A. Sferrazza, Heck reaction of arenediazonium salts with N,N-diprotected allylamines. Synthesis of cinnamylamines and indoles, Org. Biomol. Chem., 2011, 9(6), 1727–1730 RSC.
-
L. S. Hegedus, Transition metals in the synthesis of complex organic molecules, University Science Books, 1999 Search PubMed.
- M. Beller, A. Zapf and W. Mägerlein, Efficient synthesis of fine chemicals and organic building blocks applying palladium-catalysed coupling reactions, Chem. Eng. Technol., 2001, 24(6), 575–582 CrossRef CAS.
- I. Shinkai, A. King and R. Larsen, A practical asymmetric synthesis of LTD4 antagonist, Pure Appl. Chem., 1994, 66(7), 1551–1556 CAS.
-
J. E. Macor and M. J. Wythes, Indole derivatives, 1996 Search PubMed.
-
H. C. Shen, Selected Applications of Transition Metal-Catalysed Carbon–Carbon Cross-Coupling Reactions in the Pharmaceutical Industry, in Application of transition metal catalysis in drug discovery and development, 2012, pp. 25–95 Search PubMed.
-
J. Perkins, Eur. Pat., 1088817, 2001 CAS;
J. E. Macor and M. J. Wythes, Int. Pat., WO9206973, 1992 to Pfizer Inc. CAS; C. Q. Meng, Curr. Opin. Cent. Peripher. Nerv. Syst. Invest. Drugs, 2000, 2, 186 CAS.
-
F. E. Herkes, Catalysis of organic reactions, CRC Press, 1998, vol. 75 Search PubMed.
-
H. Sigel, Metal ions in biological systems, Metal complexes in tumor diagnosis and as anticancer agents, CRC Press, 2004, vol. 42 Search PubMed.
-
V. Polshettiwar and T. N. Asefa, Synthesis and applications, Wiley Online Library, 2013 Search PubMed.
- C. Yang, H. M. Lee and S. P. Nolan, Highly efficient Heck reactions of aryl bromides with n-butyl acrylate mediated by a palladium/phosphine-imidazolium salt system, Org. Lett., 2001, 3(10), 1511–1514 CrossRef CAS PubMed.
- G. Hervé and C. Len, First ligand-free, microwave-assisted, Heck cross-coupling reaction in pure water on a nucleoside–Application to the synthesis of antiviral BVDU, RSC Adv., 2014, 4(87), 46926–46929 RSC.
- A. Biffis, M. Zecca and M. Basato, Palladium metal catalysts in Heck C-C coupling reactions, J. Mol. Catal. A: Chem., 2001, 173(1), 249–274 CrossRef CAS.
- G. Zhang, H. Zhou, J. Hu, M. Liu and Y. Kuang, Pd nanoparticles catalysed ligand-free Heck reaction in ionic liquid microemulsion, Green Chem., 2009, 11(9), 1428–1432 RSC.
- B. Mariampillai, C. Herse and M. Lautens, Intermolecular Heck-type coupling of aryl iodides and allylic acetates, Org. Lett., 2005, 7(21), 4745–4747 CrossRef CAS PubMed.
- A. S. Singh, U. B. Patil and J. M. Nagarkar, Palladium supported on zinc ferrite: A highly active, magnetically separable catalyst for ligand free Suzuki and Heck coupling, Catal. Commun., 2013, 35, 11–16 CrossRef CAS.
- J. Chung, J. Kim, Y. Jang, S. Byun, T. Hyeon and B. M. Kim, Heck and Sonogashira cross-coupling reactions using recyclable Pd–Fe3O4 heterodimeric nanocrystal catalysts, Tetrahedron Lett., 2013, 54(38), 5192–5196 CrossRef CAS.
- W. Han, N. Liu, C. Liu and Z. L. Jin, A ligand-free Heck reaction catalysed by the in situ-generated palladium nanoparticles in PEG-400, Chin. Chem. Lett., 2010, 21(12), 1411–1414 CrossRef CAS.
- F. X. Felpin, E. Fouquet and C. Zakri, Heck cross-coupling of aryldiazonium tetrafluoroborate with acrylates catalysed by palladium on charcoal, Adv. Synth. Catal., 2008, 350(16), 2559–2565 CrossRef CAS.
- P. Hanson, S. C. Rowell, A. B. Taylor, P. H. Walton and A. W. Timms, Sandmeyer reactions. Part 6. 1 A mechanistic investigation into the reduction and ligand transfer steps of Sandmeyer cyanation, J. Chem. Soc., Perkin Trans. 2, 2002,(6), 1126–1134 RSC.
- P. Hanson, J. R. Jones, A. B. Taylor, P. H. Walton and A. W. Timms, Sandmeyer reactions. Part 7. 1 An investigation into the reduction steps of Sandmeyer hydroxylation and chlorination reactions, J. Chem. Soc., Perkin Trans. 2, 2002,(6), 1135–1150 RSC.
- P. Baumeister, W. Meyer, K. Oertle, G. Seifert and H. Steiner, Invention and development of a novel catalytic process for the production of a benzenesulfonic acid-building block, Chimia, 1997, 51(4), 144 CAS.
- U. Siegrist, T. Rapold and H.-U. Blaser, Process development
for a herbicide intermediate via catalytic carboxylation of an aromatic diazonium compound, Org. Process Res. Dev., 2003, 7(3), 429–431 CrossRef CAS.
- F. X. Felpin, K. Miqueu, J. M. Sotiropoulos, E. Fouquet, O. Ibarguren and J. Laudien, Room-temperature, ligand-and base-free Heck reactions of aryl diazonium salts at low palladium loading: Sustainable preparation of substituted stilbene derivatives, Chem. – Eur. J., 2010, 16(17), 5191–5204 CrossRef CAS.
- F.-X. Felpin, O. Ibarguren, L. Nassar-Hardy and E. Fouquet, Synthesis of oxindoles by tandem Heck-Reduction-Cyclization (HRC) from a single bifunctional, in situ generated Pd/C catalyst, J. Org. Chem., 2008, 74(3), 1349–1352 CrossRef.
- O. Ibarguren, C. Zakri, E. Fouquet and F.-X. Felpin, Heterogeneous palladium multi-task catalyst for sequential Heck-reduction–cyclization (HRC) reactions: influence of the support, Tetrahedron Lett., 2009, 50(36), 5071–5074 CrossRef CAS.
- S. Hu, P. Xia, K. Cheng and C. Qi, Pd-catalysed ligand-free desulfitative Heck reaction with arenesulfinic acid salts under air, Appl. Organomet. Chem., 2013, 27(3), 188–190 CrossRef CAS.
- S. A. Weissman, D. Zewge and C. Chen, Ligand-free palladium-catalysed cyanation of aryl halides, J. Org. Chem., 2005, 70(4), 1508–1510 CrossRef CAS.
-
M. J. O'Neil, The Merck index: an encyclopedia of chemicals, drugs, and biologicals, RSC Publishing, 2013 Search PubMed.
- J. K. Stille, The Palladium-catalysed cross-coupling reactions of organotin reagents with organic electrophiles[New Synthetic Methods(58)], Angew. Chem., Int. Ed. Engl., 1986, 25(6), 508–524 CrossRef.
- C. Cordovilla, The Stille reaction, 38 years later, ACS Catal., 2015, 5(5), 3040–3053 CrossRef CAS.
- K. C. Nicolaou, Total synthesis of rapamycin, J. Am. Chem. Soc., 1993, 115(10), 4419–4420 CrossRef CAS.
- M. M. Heravi, Recent developments of the Stille reaction as a revolutionized method in total synthesis, Tetrahedron, 2014, 70(1), 7–21 CrossRef CAS.
- K. C. Nicolaou, Total synthesis of Epothilone E and related side-chain modified analogues via a stille coupling based strategy1This paper is dedicated with admiration and respect to the memory of Sir Derek H. R. Barton.1, Bioorg. Med. Chem., 1999, 7(5), 665–697 CrossRef CAS.
- S. M. McAfee, J. S. J. McCahill, C. M. Macaulay, A. D. Hendsbee and G. C. Welch, Utility of a heterogeneous palladium catalyst for the synthesis of a molecular semiconductor via Stille, Suzuki, and direct heteroarylation cross-coupling reactions, RSC Adv., 2015, 5(33), 26097–26106 RSC.
- M. M. Dell'Anna, Stille coupling reactions catalysed by a polymer supported palladium complex, J. Organomet. Chem., 2006, 691(1–2), 131–137 CrossRef.
- S. Bahari, Diphenylphosphinoethane-functionalized polystyrene resin-supported Pd(0) complex — A highly active and recyclable catalyst for the Stille reaction under aerobic conditions, Can. J. Chem., 2012, 90(9), 784–789 CrossRef CAS.
- M.-J. Jin, A Practical heterogeneous catalyst for the Suzuki, Sonogashira, and Stille coupling reactions of unreactive aryl chlorides, Angew. Chem., Int. Ed., 2010, 49(6), 1119–1122 CrossRef CAS.
- A. Ghorbani-Choghamarani, Palladium supported on modified magnetic nanoparticles: a phosphine-free and heterogeneous catalyst for Suzuki and Stille reactions Suzuki and Stille reactions catalysed by FeO/SiO-PAP-Pd, Appl. Organomet. Chem., 2016, 30(3), 140–147 CrossRef CAS.
- D. Saha, Anchoring of palladium onto surface of porous metal–organic framework through post-synthesis modification and studies on Suzuki and Stille coupling reactions under heterogeneous condition, Langmuir, 2013, 29(9), 3140–3151 CrossRef CAS.
- B. M. Choudary, Layered double hydroxide supported nanopalladium catalyst for Heck-, Suzuki-, Sonogashira-, and Stille-type coupling reactions of chloroarenes, J. Am. Chem. Soc., 2002, 124(47), 14127–14136 CrossRef CAS.
- G. Xu, Y. Zhang, K. Wang, Y. Fu and Z. Du, Microwave-assisted Stille cross-coupling reaction catalysed by in situ formed palladium nanoparticles, J. Chem. Res., 2015, 39(7), 399–402 CrossRef CAS.
- S. T. Handy, Organic synthesis in ionic liquids: The Stille coupling, Org. Lett., 2001, 3(2), 233–236 CrossRef CAS.
- C. Chiappe, G. Imperato, E. Napolitano and D. Pieraccini, Ligandless Stille cross-coupling in ionic liquids, Green Chem., 2004, 6(1), 33–36 RSC.
- W. J. Zhou, K. H. Wang and J. X. Wang, Atom-efficient, palladium-catalysed Stille coupling reactions of tetraphenylstannane with aryl iodides or aryl bromides in polyethylene glycol 400 (PEG-400), Adv. Synth. Catal., 2009, 351(9), 1378–1382 CrossRef CAS.
- A. Ghorbani-Choghamarani, A. Naghipour, F. Heidarizadi, R. Shirkhani and B. Notash, Bis [(2-methylacetatobenzyl) tri(p-tolyl) phosphonium] hexabromodipalladate (II); synthesis, characterization, structural study and application as a retrievable heterogeneous catalyst for the amination of aryl halides and Stille cross-coupling reaction, Inorg. Chim. Acta, 2016, 446, 97–102 CrossRef CAS.
- A. I. Roshchin, N. A. Bumagin and I. P. Beletskaya, Palladium-catalysed cross-coupling reaction of organostannoates with aryl halides in aqueous medium, Tetrahedron Lett., 1995, 36(1), 125–128 CrossRef CAS.
- R. Rai, K. B. Aubrecht and D. B. Collum, Palladium-catalysed stille couplings of aryl-, vinyl-, and alkyltrichlorostannanes in aqueous solution, Tetrahedron Lett., 1995, 36(18), 3111–3114 CrossRef CAS.
- C. Wolf and R. Lerebours, Efficient Stille cross-coupling reaction using aryl chlorides or bromides in water, J. Org. Chem., 2003, 68(19), 7551–7554 CrossRef CAS.
- S. Ogo, Y. Takebe, K. Uehara, T. Yamazaki, H. Nakai, Y. Watanabe and S. Fukuzumi, pH-dependent C−C coupling reactions catalysed by water-soluble palladacyclic aqua catalysts in water, Organometallics, 2006, 25(2), 331–338 CrossRef CAS.
- J.-H. Li, X.-C. Hu, Y. Liang and Y.-X. Xie, PEG-400 promoted Pd(OAc)2/DABCO-catalysed cross-coupling reactions in aqueous media, Tetrahedron, 2006, 62(1), 31–38 CrossRef CAS.
- W. Susanto, C.-Y. Chu, W. J. Ang, T.-C. Chou, L.-C. Lo and Y. Lam, Fluorous oxime palladacycle: a precatalyst for carbon–carbon coupling reactions in aqueous and organic medium, J. Org. Chem., 2012, 77(6), 2729–2742 CrossRef CAS.
- G.-P. Lu, C. Cai and B. H. Lipshutz, Stille couplings in water at room temperature, Green Chem., 2013, 15(1), 105–109 RSC.
- A. S. Prasad and B. Satyanarayana, Magnetically recoverable Pd/Fe3O4-catalysed Stille cross-coupling reaction of organostannanes with aryl bromides, Bull. Korean Chem. Soc., 2012, 33(8), 2789–2792 CrossRef CAS.
- A. Krasovskiy, V. Malakhov, A. Gavryushin and P. Knochel, Efficient synthesis of functionalized organozinc compounds by the direct insertion of zinc into organic iodides and bromides, Angew. Chem., Int. Ed., 2006, 45(36), 6040–6044 CrossRef CAS.
- E.-I. Negishi, Transition metal-catalysed organometallic reactions that have revolutionized organic synthesis, Bull. Chem. Soc. Jpn., 2007, 80(2), 233–257 CrossRef CAS.
- V. B. Phapale and D. J. Cárdenas, Nickel-catalysed Negishi cross-coupling reactions: scope and mechanisms, Chem. Soc. Rev., 2009, 38(6), 1598–1607 RSC.
- S. Sase, M. Jaric, A. Metzger, V. Malakhov and P. Knochel, One-pot Negishi cross-coupling reactions of in situ generated zinc reagents with aryl chlorides, bromides, and triflates, J. Org. Chem., 2008, 73(18), 7380–7382 CrossRef CAS.
- S. Thapa, A. Kafle, S. K. Gurung, A. Montoya, P. Riedel and R. Giri, Ligand-free Copper-catalysed Negishi coupling of alkyl-, aryl-, and alkynylzinc reagents with heteroaryl iodides, Angew. Chem., Int. Ed., 2015, 54(28), 8236–8240 CrossRef CAS.
- D.-C. Wang, H.-Y. Niu, G.-R. Qu, L. Liang, X.-J. Wei, Y. Zhang and H.-M. Guo, Nickel-catalysed Negishi cross-couplings of 6-chloropurines with organozinc halides at room temperature, Org. Biomol. Chem., 2011, 9(22), 7663–7666 RSC.
- M. T. Reetz and E. Westermann, Phosphane-free palladium-catalysed coupling reactions: the decisive role of Pd nanoparticles, Angew. Chem., Int. Ed., 2000, 39(1), 165–168 CrossRef CAS.
- K. Köhler, W. Kleist and S. S. Pröckl, Genesis of co-ordinatively unsaturated palladium complexes dissolved from solid precursors during Heck coupling reactions and their role as catalytically active species, Inorg. Chem., 2007, 46(6), 1876–1883 CrossRef PubMed.
- N. T. Phan, M. Van Der Sluys and C. W. Jones, On the nature of the active species in palladium catalysed Mizoroki–Heck and Suzuki–Miyaura couplings–homogeneous or heterogeneous catalysis, a critical review, Adv. Synth. Catal., 2006, 348(6), 609–679 CrossRef CAS.
- P. Mpungose, Z. Vundla, G. Maguire and H. Friedrich, The current status of heterogeneous palladium catalysed Heck and Suzuki cross-coupling reactions, Molecules, 2018, 23(7), 1676 CrossRef PubMed.
- A. Balanta, C. Godard and C. Claver, Pd nanoparticles for C–C coupling reactions, Chem. Soc. Rev., 2011, 40(10), 4973–4985, 10.1039/C1CS15195A.
- M. Pérez-Lorenzo, Palladium nanoparticles as efficient catalysts for Suzuki cross-coupling reactions, J. Phys. Chem. Lett., 2012, 3(2), 167–174 CrossRef.
- T. Hirakawa, Y. Uramoto, S. Yanagisawa, T. Ikeda, K. Inagaki and Y. Morikawa, First-principles molecular dynamics analysis of ligand-free Suzuki–Miyaura cross-coupling in water: Transmetalation and reductive elimination, J. Phys. Chem. C, 2017, 121(36), 19904–19914 CrossRef CAS.
- S. N. Jadhav, A. S. Kumbhar, C. V. Rode and R. S. Salunkhe, Ligand-free Pd catalysed cross-coupling reactions in an aqueous hydrotropic medium, Green Chem., 2016, 18(7), 1898–1911 RSC.
-
D. Astruc, Transition-metal nanoparticles in catalysis: from historical background to the state-of-the art, in Nanoparticles and Catalysis, 2008, pp. 1–48 Search PubMed.
- T. Akiyama, T. Taniguchi, N. Saito, R. Doi, T. Honma, Y. Tamenori, Y. Ohki, N. Takahashi, H. Fujioka and Y. Sato, Ligand-free Suzuki–Miyaura coupling using ruthenium(0) nanoparticles and a continuously irradiating microwave system, Green Chem., 2017, 19(14), 3357–3369 RSC.
|
This journal is © The Royal Society of Chemistry 2019 |
Click here to see how this site uses Cookies. View our privacy policy here.