DOI:
10.1039/C8AY01998C
(Paper)
Anal. Methods, 2019,
11, 36-43
An exceptionally stable Zr-based fluorescent metal–organic framework for highly selective detection of pH†
Received
12th September 2018
, Accepted 21st November 2018
First published on 23rd November 2018
Abstract
In this study, we synthesized metal–organic frameworks (MOFs) UiO-67-bpydc using H2bpydc as ligands and Zr6 clusters as vertexes. Due to the protonation of the bipyridine group, UiO-67-bpydc shows pH-dependent fluorescence (365 nm/530 nm) in the pH range from 1 to 9, which shows its feasibility as a wide-range pH sensor. Further study indicated that UiO-67-bpydc exhibits stability, reusability, selectivity and has an excellent linear relationship in the pH range 4.0–6.5, and the mechanism of the UiO-67-bpydc fluorescence intensity with the variation of pH has been studied in detail. This MOF-based fluorescent sensor has been applied to specifically detect the pH of solutions in the presence of the body fluid matrix. Compared with reported pH-MOF sensors, UiO-67-bpydc can be practically applied in real samples and is easily prepared with excellent stability in different buffer solutions.
Introduction
pH is a pivotal parameter in many applications, including industrial production, environmental monitoring, and biomedical diagnostics and treatment. In environmental fields, abnormal pH values of water and soil are related to algal bloom and the emission of pollutants.1 Moreover, the detection of pH plays a critical role in all living organisms, because some diseases such as type 2 Diabetes,2 stroke3 and cancer4 always lead to slight pH fluctuation in the human body. Therefore, monitoring the values and changes in pH is significant. Compared to other conventional pH measurement methods such as the pH test paper method, electrochemical methods and NMR,5–7 fluorescence methods have gained much attention due to high sensitivity, rapid response, few interferences, facilitated detection and manipulation as well as noninvasive properties,8–10 and fluorescent probes have been considered as promising technologies in the detection of pH.
Metal–organic frameworks (MOF) are a relatively new family of hybrid materials formed by metal ions and organic molecules. MOFs have been widely studied as luminescent materials over the last decade. For instance, luminescent metal–organic frameworks (LMOFs) were successfully used in the detection of cations, anions, small molecules, explosives, etc.11–18 Most LMOFs are not stable in water systems, especially in buffer solutions, thus, only a few pH-MOF sensors have been reported, and some flaws still exist among them.19–23 For example, the fluorescence intensities of some pH-MOF sensors have no excellent correlation with the pH value,19,20 and some reported MOFs cannot be applied extensively due to the shortcomings of poor selectivity and linear relationship, resulting in no quantitative sample analysis.21–23 Thus, the development of easily synthesized, stable, selective, and accurate fluorescent pH sensors based on MOFs for practical application is still a challenging task.
In order to obtain effective pH-MOF sensors in biosystems, a linear response in the pH range of about 4.00–7.80 is required, which covers the common range of some organelles and body fluids.4,24 Nitrogen atoms in pyridine can undergo protonation under acidic conditions25–29 and 2,2′-bipyridine has a pKa value of about 4.40, which may lead to a sudden change of pH in this pH range. Moreover, the Zr6 cluster is one of the most stable building units for MOF construction. Herein, we selected an organic ligand based on 2,2′-bipyridine and synthesized a robust UiO type framework, UiO-67-bpydc (bpydc = 2,2-bipyridine-5,5′-dicarboxylic acid) by facile hydrothermal method (Scheme 1). As expected, UiO-67-bpydc presents pH-dependent fluorescence with a significant linear correlation in the pH range of 4.00–6.50. This linearity between fluorescence intensity and pH is also common in molecular probes and quantum dots,30,31 which is easier to use than exponential relationships. It also responds to pH in multiple buffer systems and it was successfully applied to detect the pH of solutions in the body fluid matrix.
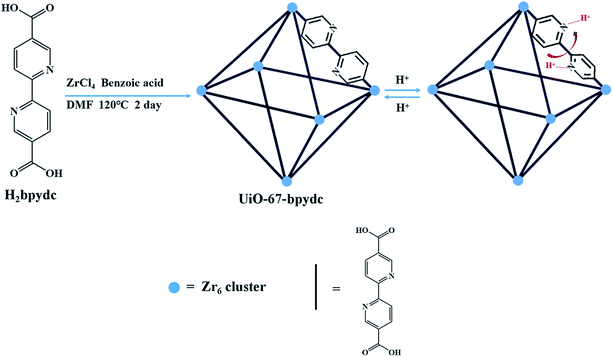 |
| Scheme 1 Synthesis of UiO-67-bpydc and protonation/deprotonation of nitrogen involved in the UiO-67-bpydc framework in experimental acidic media. The topology is shown in a simplified form as an octahedral cage. | |
Experimental
Materials
2,2-Bipyridine-5,5′-dicarboxylic acid (H2bpydc, 97%) was purchased from Bide Pharmatech Ltd. (Shanghai, China). Zirconium chloride (ZrCl4, 98%) was purchased from Aladdin (Shanghai, China). Benzoic acid (≥99.5%), N,N-dimethylformamide (DMF, 99.5%), anhydrous methanol (≥99.5%), sodium hydroxide (NaOH, ≥96.0%), hydrogen chloride (HCl, 36.0–38.0%), acetic acid (HAc, ≥99.5%), sodium acetate trihydrate (NaAc, ≥99.5%), citric acid monohydrate (≥99.5%), trisodium citrate dihydrate (≥99.0%), potassium hydrogen phthalate (KHP, ≥99.5%) and sodium chloride (NaCl, ≥99.5%) were purchased from Sinopharm Chemical Reagent Limited Corporation (Shanghai, China) and used without further purification.
Physical measurements
Powder Xray diffraction (PXRD) patterns were obtained on the Bruker D8 advance X-ray diffractometer (Bruker AXS GmbH, Karlsruhe, Germany) in the 2θ range of 5–40°. Thermal gravimetric analysis (TGA) was performed with a simultaneous thermal analyzer, STA 449C (SETARAM, France), from 25 °C to 800 °C (10 °C min−1, N2 atmosphere). Scanning electron microscope (SEM) images were recorded on the SIGMA FE-SEM microscope (Zeiss Merlin Compact, England). All the samples were mounted on a carbon tape and coated with gold prior to measurement. Fluorescence and UV-absorption measurements were taken with an RF-6000 Fluorescence Spectrophotometer (SHIMADZU, Japan) and UV-2600 UV-VIS Spectrometer (SHIMADZU, Japan), respectively. N2 adsorption measurements were carried out by NOVA 4200e (Quantachrome, US) at 77 K.
Synthesis of UiO-67-bpydc
The synthesis of UiO-67-bpydc was carried out by following a modified procedure reported previously.32 ZrCl4 (49 mg, 0.21 mmol), H2bpydc (52 mg, 0.21 mmol) and benzoic acid (1.5 g, 12.3 mmol) were dissolved in 8 mL of DMF in a small capped vial and placed in a 120 °C oven for 2 days. The product was isolated by centrifugation and washed with DMF and anhydrous methanol several times, respectively. Finally, UiO-67-bpydc was activated by removing the volatile methanol under vacuum for 12 h at 65 °C.
pH-Dependent luminescence studies of UiO-67-bpydc
UiO-67-bpydc suspension (200 μL, 3 mg mL−1) was added to 1 mL water solutions with pH value from 1.03 to 9.02 (adjusted using HCl or NaOH) and then diluted with deionized water to 2 mL. The resulting mixture was sonicated to reach uniformly dispersion. After reacting for 3 min at room temperature, the fluorescence spectra (λex = 365 nm) were recorded from 380 nm to 680 nm. The fluorescence spectra of UiO-67-bpydc suspensions in different kinds of buffer systems were recorded under the same conditions as above.
Quantum-chemical calculations
All the quantum-chemical calculations were done with the Gaussian 09 suite.33 The geometry optimizations of the structures were performed by the use of density functional theory (DFT) with Becke's three-parameter hybrid exchange function with Lee–Yang–Parr gradient-corrected correlation functional (B3LYP functional). No constraints to bonds/angles/dihedral angles were applied in the calculations and all atoms were free to optimize.
Stability test of UiO-67-bpydc
The as-synthesized UiO-67-bpydc was soaked in HCl–NaOH aqueous solutions (0.1 M) with pH values of 1.00 and 9.00 or HAc–NaAc buffer solution (0.1 M) with pH values of 3.00 and 6.50 for 24 h. After that, the UiO-67-bpydc was collected, dried and then characterized by PXRD and SEM. The chemical stability of UiO-67-bpydc in different kinds of buffer solutions was studied under the same conditions as above.
Reversibility of the response of UiO-67-bpydc to H+
To test the reusability of UiO-67-bpydc, 3 mg of UiO-67-bpydc was added to 2 mL of HAc–NaAc buffer (0.1 M, pH 3.00) and the fluorescence intensity of the suspension was recorded. The precipitate was isolated by centrifugation and washed with HAc–NaAc buffer (0.1 M, pH 6.50) several times. Then, the precipitate was resuspended in 2 mL of HAc–NaAc buffer (0.1 M, pH 6.50) and the fluorescence intensity of the suspension with pH 6.50 was measured. This cycle was repeated four times.
Analysis of real samples
Human urine samples were collected from healthy volunteers. After filtration, the fluorescence intensities of UiO-67-bpydc in HAc–NaAc buffer from 4.50 to 6.51 with the addition of urine sample and without the addition of urine sample were recorded.
These studies were approved by the hospital's Institutional Ethics Committee (Renmin Hospital of Wuhan University, China), and written informed consent was obtained from all volunteers.
Results and discussion
Characterization of UiO-67-bpydc
The colorless octahedral crystals of UiO-67-bpydc (Fig. 1) were harvested according to the literature32 and characterized by powder X-ray diffraction (PXRD) (Fig. 1a), thermal gravimetric analysis (TGA) (Fig. 1b), and scanning electron microscopy (SEM) (Fig. 1c). The PXRD pattern of the as-synthesized UiO-67-bpydc agrees well with the simulated one, which confirms the phase purity of the product. As expected, the results of SEM showed an octahedral morphology of UiO-67-bpydc with a diameter of 5–10 μm. These results are similar to the reported data.32
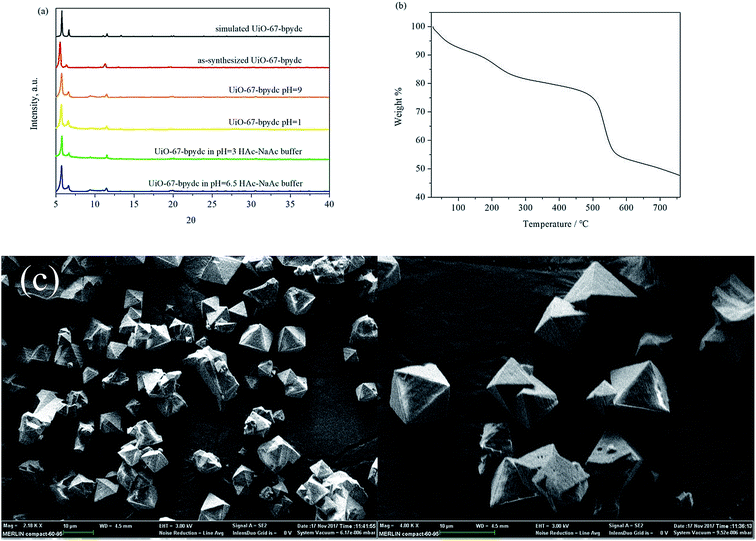 |
| Fig. 1 Characterization of UiO-67-bpydc. (a) PXRD patterns of simulated UiO-67-bpydc (black), as-synthesized UiO-67-bpydc (red), UiO-67-bpydc soaked in aqueous solutions with pH value of 9 (orange) and 1 (yellow), and UiO-67-bpydc soaked in HAc–NaAc buffer with pH value of 3 (green) and 6.50 (blue). (b) TGA curve, and (c) SEM image of UiO-67-bpydc. | |
The stability of MOFs toward different chemical surroundings is crucial for sensing applications. As shown in Fig. 1a, the PXRD patterns show retained crystallinity and unchanged structures after being soaked in aqueous solutions with pH values of 1.00 and 9.00, and the UiO-67-bpydc also remains intact in some kinds of buffer systems like HAc–NaAc buffer after 24 h. As shown in Fig. S2,† the morphology of UiO-67-bpydc is almost constant before and after treatment, which proves that it is exceptionally stable and has the potential for use as a pH sensor. As a sensor for detecting the pH of acid solution, its stability in acid solution was examined by N2 adsorption. As demonstrated in Fig. S3† the results of nitrogen adsorption of UiO-67-bpydc before and after treatment in pH 1.0 solution are similar, which proved that UiO-67-bpydc is stable in acid solutions for 24 hours. From the TGA data (Fig. 1b), UiO-67-bpydc loses its occluded solvent molecules on heating and starts to collapse around 500 °C. At 550 °C, the entire framework collapsed, indicating its good thermal stability.
Fluorescence response of UiO-67-bpydc to H+
Considering the advantages of the protonation of pyridyl group nitrogen atoms and the excellent chemical stability of UiO-67-bpydc, we sought to explore its application in detecting pH in water through fluorescence sensing. The luminescence properties of H2bpydc and UiO-67-bpydc were checked at the same pH. H2bpydc and UiO-67-bpydc exhibited fluorescence emissions at 558 and 530 nm upon 365 nm excitation (Fig. S4†), and the emission spectrum of UiO-67-bpydc is similar to that of the H2bpydc ligand, except for a slight blue-shift of the wavelength, which indicates that the fluorescence of UiO-67-bpydc can be regarded as ligand-centered emission. The blue-shift of the fluorescence emissions can be attributed to the deprotonation and coordination of the ligand acids to Zr4+ cations. The fluorescence behaviour of UiO-67-bpydc based on ligand-centered emission together with high water stability prompted us to explore its fluorescence sensing of pH.21
Our preliminary results of pH-dependent luminescence studies of UiO-67-bpydc were encouraging. Time-dependent fluorescence of UiO-67-bpydc towards H+ showed that after the UiO-67-bpydc and H+ were in contact for about 3 minutes, the fluorescence decreased and tended to balance, indicating that the reaction was almost finished within 3 min at room temperature (Fig. S5†). As demonstrated in Fig. 2, UiO-67-bpydc exhibits a wide pH response range. The fluorescence intensity of UiO-67-bpydc distinctly increased with pH in the pH range of 1.03–7.03, but decreased with pH higher than 7.03.
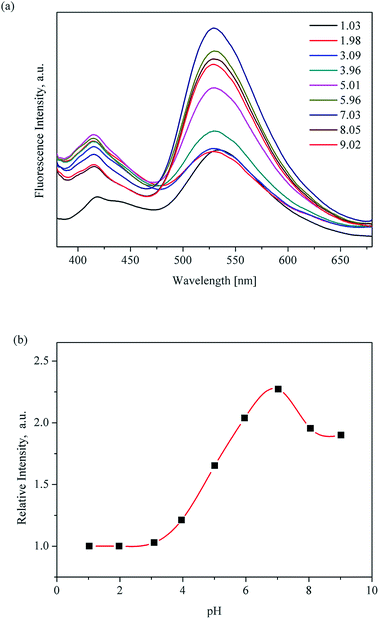 |
| Fig. 2 pH-Dependent fluorescence (a) and linear variation (b) of UiO-67-bpydc in aqueous solution from 1.03 to 9.02; measured under excitation of 365 nm. | |
Significantly, there is a linear relationship between fluorescence intensity and pH values ranging from 3.96 to 7.03. The linear relationship between pH value and fluorescence intensity in different kinds of buffer systems was studied, and an excellent linear relationship between the fluorescence intensity of UiO-67-bpydc and the pH value was found in some kinds of buffers including HAc–NaAc buffer, KHP buffer and citrate buffer systems (Fig. 3, S6 and S7†). Various buffer have slight differences in the accurate pKa values of UiO-67-bpydc, which might cause minor changes in the linear range.34 There is no distinctive linear relationship in phosphate buffered solution, which may be responsible for some disruption of the crystalline structure. According to the literature (Ksp(Zr3(PO4)4) = 1 × 10−132),35 the coordination between the Zr ion and PO43− is easier and faster as compared to the Zr ion and carboxy groups on organic ligands. The results indicate the reliability and universality of the proposed method, which has potential in different kinds of buffer systems.
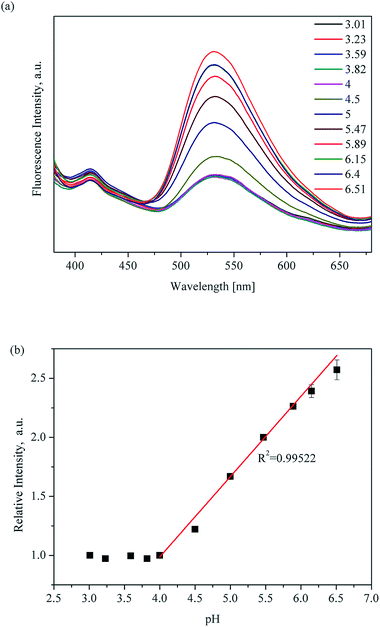 |
| Fig. 3 pH-Dependent fluorescence (a) and linear variation (b) of UiO-67-bpydc in HAc–NaAc buffer from 3.01 to 6.51; measured under excitation of 365 nm. | |
A possible mechanism of pH sensing
To deeply study the mechanism of the fluorescence response of UiO-67-bpydc to H+, we have recorded the effect of the acidity or basicity on the absorption spectra of UiO-67-bpydc and H2bpydc. Interestingly, from Fig. 4, the maximum UV absorption of UiO-67-bpydc and H2bpydc at 296 nm disappeared with decreasing pH value, and this could be the main reason for the decrease in the MOF's fluorescence intensity. We believe that the mechanism for the disappearing of UV absorption could be induced by the protonation of the bipyridine group. The maximum UV absorption at 296 nm is obviously longer as compared to pyridine (about 250 nm), which could be attributed to the π–π* transitions of the large conjugated and coplanar 2,2′-bipyridine structure. Such coplanar unprotonated 2,2′-bipyridine structure can also be found in other reported MOFs.32 After protonation of the bipyridine group, the additional proton on one pyridine ring generated obvious steric hindrance with hydrogen atoms on another pyridine ring. Thus, the two pyridine rings should not be coplanar and the large conjugated system will be divided into two small individual conjugated systems (two protonated pyridine rings). The structure of protonated bipyridine is similar to biphenyl, and biphenyl as the ligand in reported MOFs might be non-coplanar, which is in line with our speculation.36
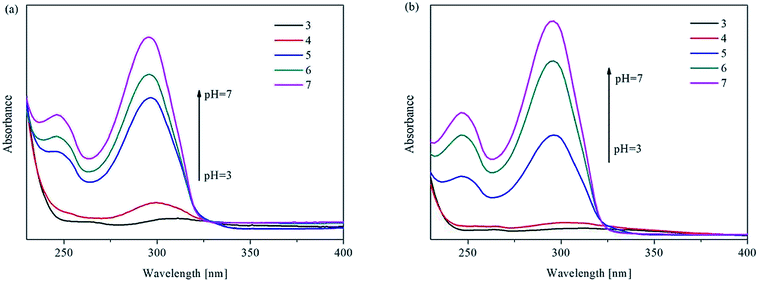 |
| Fig. 4 Absorption spectra of UiO-67-bpydc (a) and H2bpydc (b) under acidic conditions: pH 3–7. | |
To confirm our speculation, some experiments were performed. First, UiO-67-bpdc was synthesized using biphenyl-4,4′-dicarboxylic acid (H2bpdc) as the ligand to compare its fluorescence properties with UiO-67-bpydc. As shown in Fig. S8,† we found that the relationship between fluorescence intensity of UiO-67-bpdc and pH is completely different as compared to UiO-67-bpydc. The reason for this difference could be attributed to the presence and protonation of nitrogen atoms in pyridine. We also performed quantum chemical calculations to predict the stereostructures of unprotonated and protonated H2bpydc.
From the results shown in Fig. 5, the two pyridine rings of the unprotonated ligand were completely coplanar, which means that the two rings formed a larger conjugated system and should have an absorption band with a longer wavelength (296 nm) than pyridine. However, the dihedral angle between the two pyridine rings in the completely protonated bipyridine ligand was 43.46°, causing the destruction of the large conjugated system. This led to the disappearance of the max absorption band at 296 nm. Moreover, protons added to the nitrogen atoms could be considered as electron-withdrawing substituents of 2,2′-bipyridine. The fluorescence of 2,2′-bipyridine would drastically decrease due to the presence of such electron-withdrawing substituents.29,37 Consequently, the destruction of the conjugate system and electron-withdrawing substituents are ascribed to H+ binding with the N atom in the bipyridine group, which neatly explains why the protonation of the bipyridine group causes luminescence reduction.
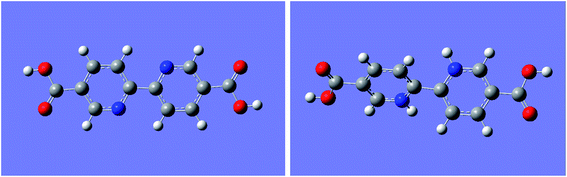 |
| Fig. 5 Optimized structure of the unprotonated and completely protonated H2bpydc. | |
Reusability of the UiO-67-bpydc
Ideally, a smart and effective sensor should be structurally sound and reusable after a number of regeneration cycles. To accomplish this, the pH-dependent luminescence intensities of UiO-67-bpydc were recorded between pH 3 and pH 6.50 for four consecutive cycles. As shown in Fig. 6, the quenching efficiencies up to four cycles are basically unchanged, demonstrating excellent reusability and stability for these detection applications.
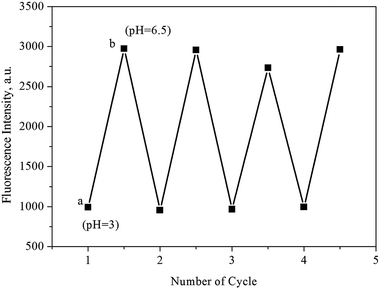 |
| Fig. 6 Reversibility and reproducibility of pH tests using UiO-67-bpydc. The measurement was carried out in HAc–NaAc buffer (0.1 M) solutions with different pH values in turn: (a) 6.50, (b) 3.00. | |
Comparison with reported pH-MOF sensors
Only a few pH-MOF sensors have been reported thus far. Due to some defects like poor stability and non-linear relationships, quantitative analysis and application of these pH-MOF sensors in actual samples are rare. We compared some parameters of UiO-67-bpydc with other pH-MOFs including synthetic procedure, stability, reversibility, regression equation and application. As listed in Table 1, the UiO-67-bpydc is easily synthesized with good stability in buffer solutions, which is better than most other sensors (stable in water or HCl/NaOH solutions). Moreover, UiO-67-bpydc presents pH-dependent fluorescence with an excellent linear relationship in the pH range of 4.00–6.50 in different buffer solutions. Further research on applications in biological systems should be carried out.
Table 1 Comparison of synthetic procedure, stable conditions, reversibility, regression equation and application of pH sensors
MOFs |
Synthetic procedure |
Stable conditions |
Reversibility |
Regression equation |
Application in biological system |
Ref. |
1-(3,5-Dicarboxybenzyl)-4,4′-bipyridinium chloride (H2L+Cl−) |
Specific ligand synthesis + solvent-thermal method |
pH 2–7 HCl |
Reversible |
Linear, pH 2–7 |
— |
26
|
ITQMOF-3-Eu |
Solvent-thermal method |
pH 5–7, 1 h |
Reversible |
Linear, pH 5–7.5 |
— |
41
|
PCN-225 |
Specific ligand synthesis + solvent-thermal method |
pH 1–11, 12 h |
— |
Non-linear |
— |
19
|
UiO-66-N N-ind |
Solvent-thermal method + postsynthetic modification (PSM) |
pH 12 NaOH, 3 h |
— |
Non-linear |
— |
23
|
[H3O][Eu3(HBPTC)2(BPTC)(H2O)2]·4DMA |
Solvent-thermal method |
pH 10 NaOH, 0.5 h |
— |
Linear, pH 7.5–10.0 |
— |
20
|
Eu0.034Tb0.966 – NMOF |
Water-in-oil microemulsion method |
pH 3–11, HAc–NaOH |
— |
Exponential, pH 3–7 |
Living cells |
42
|
MOF-253-Eu-TTA |
Solvent-thermal method + PSM |
pH 5–7.5, HCl or NaOH |
Reversible |
Linear, pH 5.0–7.2 |
— |
34
|
ER-H (dye) |
Organic synthesis (2 steps) |
— |
— |
Linear, pH 4.0–5.0 |
Living cells |
30
|
FA-G QDs |
Microwave method (2 steps) |
— |
— |
Linear, pH 5.0–8.0 |
Living cells |
31
|
UiO-67-bpydc |
Solvent-thermal method |
pH 1–9, HCl–NaOH & HAc–NaOH, 24 h |
Reversible |
Linear, pH 4–6.5 |
Human urine |
This work |
Analysis of real samples
To determine whether coexistent components in body fluids could cause a change in fluorescence intensity, we tested the fluorescence of UiO-67-bpydc in the presence of K+, Na+, Ca2+, NH4+, Zn2+, Mg2+, Fe3+, Cu2+, H2PO4−, Cre, urea and uric acid. As shown in Fig. S9,† no intensity changes were observed in the case of these interferents at reasonable concentrations.38,39
To test the potential and accuracy of our approach for pH sensing in real sample analysis, the proposed sensor was used to detect the pH of human urine. We recorded the fluorescence intensities of UiO-67-bpydc in HAc–NaAc buffer from 4.50 to 6.51 with the addition of urine sample and without the addition of urine sample and evaluated the differences. As shown in Fig. 7, the fluorescence intensities of UiO-67-bpydc in the human urinary matrix were much the same as those in HAc–NaAc buffer, and no significant difference was observed. To verify the potential application of this UiO-67-bpydc, the proposed sensor was used to detect the pH of solutions with human urine samples. As summarized in Tables 2 and S1,† the results obtained by the UiO-67-bpydc were consistent with that of a pH meter and the limit of detection (LOD) was 0.044 pH, indicating the reliability of the proposed method, which is feasible for detecting pH in the human urinary matrix.
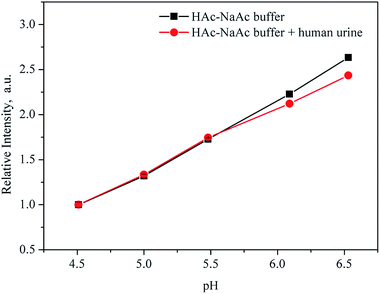 |
| Fig. 7 Fluorescence intensity of UiO-67-bpydc in HAc–NaAc buffer from 4.50 to 6.51 with the addition of urine sample (red) and without the addition of urine sample (black). | |
Table 2 Determination of the pH using UiO-67-bpydc in human urine
Samples |
True pH value |
Detected pH valuea by proposed method |
RSDb (%) |
Recovery (%) |
All concentrations are expressed as mean value (n = 3) ± standard deviation (SD).
The relative standard deviation (RSD) is defined as (SD/mean) × 100%.
|
A |
5.79 |
5.79 ± 0.005 |
0.08% |
100.09% |
B |
6.37 |
6.46 ± 0.024 |
0.37% |
101.41% |
It has been reported that the pH of human urine is related to some diseases such as urolithiasis and type 2 diabetes.2,40 Epidemiologic studies have also shown that urolithiasis, type 2 diabetes, and overweight or obesity can lead to low urine pH (≤6.5). Thus, the determination of the pH of human urine is associated with the prevention, diagnosis and therapy of these diseases. The analytical performance of the UiO-67-bpydc sensor has potential applications in biology.
Conclusions
A typical UiO-type MOF, UiO-67-bpydc, has been synthesized and applied as a pH sensor. UiO-67-bpydc shows excellent chemical stability and variable luminescence in aqueous buffer solutions with pH values ranging from 1 to 9. Due to the protonation–deprotonation equilibrium of the bipyridine group in ligands upon varying pH, the most sensitive pH range is from 4.00 to 6.50 with a good correlation coefficient, and cycling experiments have demonstrated the reusability of the sensor. Moreover, UiO-67-bpydc was successfully used for the detection of pH in the human urinary matrix with satisfactory results. Considering that the pH values of some body fluids and most groundwater are less than seven, we believe that UiO-67-bpydc and the proposed method have great potential for pH sensing in such samples.
Author information
The manuscript was written through contributions of all authors. All authors have given approval to the final version of the manuscript.
Conflicts of interest
The authors declare no competing financial interest.
Acknowledgements
This work was supported by the National Natural Science Foundation of China (No. 31670370 and 21777126, Beijing, China).
References
- C. Gago, M. L. F. Marcos and E. Alvarez, Fluoride, 2002, 35, 110–121 CAS
.
- N. M. Maalouf, M. A. Cameron, O. W. Moe and K. Sakhaee, Clin. J. Am. Soc. Nephrol., 2010, 5, 1277–1281 CrossRef CAS PubMed
.
- R. D. Vaughan-Jones, K. W. Spitzer and P. Swietach, J. Mol. Cell. Cardiol., 2009, 46, 318–331 CrossRef CAS PubMed
.
- B. A. Webb, M. Chimenti, M. P. Jacobson and D. L. Barber, Nat. Rev. Cancer, 2011, 11, 671–677 CrossRef CAS PubMed
.
- D. Wencel, T. Abel and C. McDonagh, Anal. Chem., 2014, 86, 15–29 CrossRef CAS PubMed
.
- D. M. Kim, S. J. Cho, C. H. Cho, K. B. Kim, M. Y. Kim and Y. B. Shim, Biosens. Bioelectron., 2016, 79, 165–172 CrossRef CAS PubMed
.
- S. Mori, S. M. Eleff, U. Pilatus, N. Mori and P. C. M. van Zijl, Magn. Reson. Med., 1998, 40, 36–42 CrossRef CAS
.
- T. Berbasova, M. Nosrati, C. Vasileiou, W. J. Wang, K. Sing, S. Lee, I. Yapici, J. H. Geiger and B. Borhan, J. Am. Chem. Soc., 2013, 135, 16111–16119 CrossRef CAS
.
- B. F. Shi, Y. B. Su, L. L. Zhang, R. J. Liu, M. J. Huang and S. L. Zhao, Biosens. Bioelectron., 2016, 82, 233–239 CrossRef CAS
.
- X. Q. Chen, T. Pradhan, F. Wang, J. S. Kim and J. Yoon, Chem. Rev., 2012, 112, 1910–1956 CrossRef CAS
.
- F.-Y. Yi, D. Chen, M.-K. Wu, L. Han and H.-L. Jiang, ChemPlusChem, 2016, 81, 675–690 CrossRef CAS
.
- D. Zhao, Y. Cui, Y. Yang and G. Qian, CrystEngComm, 2016, 18, 3746–3759 RSC
.
- B. Wang, X. L. Lv, D. Feng, L. H. Xie, J. Zhang, M. Li, Y. Xie, J. R. Li and H. C. Zhou, J. Am. Chem. Soc., 2016, 138, 6204–6216 CrossRef CAS PubMed
.
- Y. Y. Cao, X. F. Guo and H. Wang, Sens. Actuators, B, 2017, 243, 8–13 CrossRef CAS
.
- M. Jin, Z. L. Mou, R. L. Zhang, S. S. Liang and Z. Q. Zhang, Biosens. Bioelectron., 2017, 91, 162–168 CrossRef CAS PubMed
.
- W. Yan, C. Zhang, S. Chen, L. Han and H. Zheng, ACS Appl. Mater. Interfaces, 2017, 9, 1629–1634 CrossRef CAS
.
- Y. Cui, R. Song, J. Yu, M. Liu, Z. Wang, C. Wu, Y. Yang, Z. Wang, B. Chen and G. Qian, Adv. Mater., 2015, 27, 1420–1425 CrossRef CAS PubMed
.
- J. Wang, C. He, P. Wu, J. Wang and C. Duan, J. Am. Chem. Soc., 2011, 133, 12402–12405 CrossRef CAS PubMed
.
- H. L. Jiang, D. Feng, K. Wang, Z. Y. Gu, Z. Wei, Y. P. Chen and H. C. Zhou, J. Am. Chem. Soc., 2013, 135, 13934–13938 CrossRef CAS PubMed
.
- Q. Meng, X. Xin, L. Zhang, F. Dai, R. Wang and D. Sun, J. Mater.
Chem. A, 2015, 3, 24016–24021 RSC
.
- X. Zhang, K. Jiang, H. He, D. Yue, D. Zhao, Y. Cui, Y. Yang and G. Qian, Sens. Actuators, B, 2018, 254, 1069–1077 CrossRef CAS
.
- X. Y. Xu and B. Yan, Dalton Trans., 2016, 45, 7078–7084 RSC
.
- J. Aguilera-Sigalat and D. Bradshaw, Chem. Commun., 2014, 50, 4711–4713 RSC
.
- N. M. Maalouf, M. A. Cameron, O. W. Moe, B. Adams-Huet and K. Sakhaee, Clin. J. Am. Soc. Nephrol., 2007, 2, 883–888 CrossRef CAS
.
- Z. W. Qi and Y. Chen, Biosens. Bioelectron., 2017, 87, 236–241 CrossRef CAS PubMed
.
- H. Y. Li, Y. L. Wei, X. Y. Dong, S. Q. Zang and T. C. W. Mak, Chem. Mater., 2015, 27, 1327–1331 CrossRef CAS
.
- M. Bouachrine, J. P. Lere-Porte, J. J. E. Moreau, F. Serein-Spirau and C. Torreilles, J. Mater. Chem., 2000, 10, 263–268 RSC
.
- M. H. Zheng, J. Y. Jin, W. Sun and C. H. Yan, New J. Chem., 2006, 30, 1192–1196 RSC
.
- J. M. Kim, T. E. Chang, J. H. Kang, K. H. Park, D. K. Han and K. D. Ahn, Angew. Chem., Int. Ed., 2000, 29, 1780–1782 CrossRef
.
- H. Xiao, R. Zhang, C. Wu, P. Li, W. Zhang and B. Tang, Sens. Actuators, B, 2018, 273, 1754–1761 CrossRef CAS
.
- X. Hai, Y. Wang, X. Hao, X. Chen and J. Wang, Sens. Actuators, B, 2018, 268, 61–69 CrossRef CAS
.
- L. Li, S. Tang, C. Wang, X. Lv, M. Jiang, H. Wu and X. Zhao, Chem. Commun., 2014, 50, 2304–2307 RSC
.
- Y. Ji, L. J. Xia, L. Chen, X. F. Guo, H. Wang and H. J. Zhang, Talanta, 2018, 181, 104–111 CrossRef CAS PubMed
.
- Y. Lu and B. Yan, Chem. Commun., 2014, 50, 13323–13326 RSC
.
-
J. G. Speight, Lange's handbook of chemistry, McGraw-Hill, New York, 2005 Search PubMed
.
- M. J. Katz, Z. J. Brown, Y. J. Colon, P. W. Siu, K. A. Scheidt, R. Q. Snurr, J. T. Hupp and O. K. Farha, Chem. Commun., 2013, 49, 9449–9451 RSC
.
- S.-Z. Wen, W.-Q. Kan, L.-L. Zhang and Y.-C. He, Cryst. Res. Technol., 2017, 52, 1700105 CrossRef
.
- S. Meret and R. I. Henkin, Clin. Chem., 1971, 17, 369–373 CAS
.
- P. O. Wester, J. Intern. Med., 1973, 194, 505–512 CAS
.
- W. M. Li, Y. H. Chou, C. C. Li, C. C. Liu, S. P. Huang, W. J. Wu, C. W. Chen, C. Y. Su, M. H. Lee, Y. C. Wei and C. H. Huang, Urol. Res., 2009, 37, 193–196 CrossRef PubMed
.
- B. V. Harbuzaru, A. Corma, F. Rey, J. L. Jorda, D. Ananias, L. D. Carlos and J. Rocha, Angew. Chem., Int. Ed., 2009, 48, 6476–6479 CrossRef CAS PubMed
.
- T. F. Xia, F. L. Zhu, K. Jiang, Y. J. Cui, Y. Yang and G. D. Qian, Dalton Trans., 2017, 46, 7549–7555 RSC
.
Footnotes |
† Electronic supplementary information (ESI) available. See DOI: 10.1039/c8ay01998c |
‡ These authors contributed equally to this manuscript. |
|
This journal is © The Royal Society of Chemistry 2019 |