DOI:
10.1039/C8SC01062E
(Edge Article)
Chem. Sci., 2018,
9, 4104-4108
How does chiral self-sorting take place in the formation of homochiral Pd6L8 capsules consisting of cyclotriveratrylene-based chiral tritopic ligands?†
Received
6th March 2018
, Accepted 26th March 2018
First published on 26th March 2018
Abstract
The chiral self-sorting process during the self-assembly of homochiral Pd6L8 capsules from cyclotriveratrylene (CTV)-based chiral tritopic ligands (L) and
(Py*: 3-chloropyridine) was investigated by an NMR-based approach (QASAP: quantitative analysis of the self-assembly process). From the beginning to the formation of the
immature capsules (ICs), enantiomeric ligands are distributed in the intermediates in a non-self-sorting manner, which leads to the isomers of heterochiral ICs over 99% yield. The mismatch of the chirality in the heterochiral ICs prevents intramolecular ligand exchanges in ICs to form the heterochiral capsules. The correction of the chirality in the heterochiral ICs (chiral self-sorting) takes place very slowly to finally lead to the homochiral capsules. The reason why the chiral self-sorting took place in the late stage of the self-assembly (after the formation of the heterochiral ICs) would be due to the relatively high flexibility of the CTV-based ligand.
Introduction
Chiral self-sorting is a phenomenon of chiral recognition where homochiral or heterochiral assemblies are favored over a statistical distribution under thermodynamic control arising from mutual discrimination between enantiomeric components.1 Cyclotriveratrylene (CTV) derivatives possessing two kinds of substituents alternately introduced on the rim of CTV have helical chirality.2 Upon the complexation of a racemic mixture of the CTV-based tritopic ligand, 1, with Pd(II) ions, homochiral octahedron-shaped Pd618 capsules are self-assembled through the homochiral self-sorting of enantiomeric ligands, 1P and 1M (Fig. 1).3 Which is favored, homochiral or heterochiral assemblies, is determined by relative thermodynamic stabilities of the diastereomeric isomers, but how chiral self-sorting takes place has been an unresolved question. For the understanding of the chiral self-sorting process, it is necessary to obtain information concerning intermediates that are transiently produced. To achieve this, a reliable method to investigate molecular self-assembly processes was recently developed by the authors (QASAP: quantitative analysis of the self-assembly process).4 In most cases, most of the intermediates cannot be observed by spectroscopy, which has prevented us from investigating molecular self-assembly processes. On the other hand, QASAP enables us to obtain information about the intermediates as an average composition of all the intermediates by quantifying all the substrates and the products, so it is possible to reveal molecular self-assembly processes even if any intermediates are not observed. Here we report the chiral self-sorting process of homochiral Pd618 capsules assembled from Pd(II) ions (
, Py* = 3-chloropyridine) and CTV-based chiral tritopic ligands, 1, by QASAP (Fig. 1). The self-assembly takes place without chiral discrimination between the enantiomeric ligands until the formation of
immature capsules (ICs). Most of the isomers of ICs are heterochiral species, which are kinetically trapped at 298 K, and their transformation into the heterochiral capsules is prevented. The correction of the chirality in the heterochiral ICs took place significantly slowly and with the aid of free 1 to lead to the homochiral ones, which are finally converted into the homochiral capsules by intramolecular ligand exchanges.
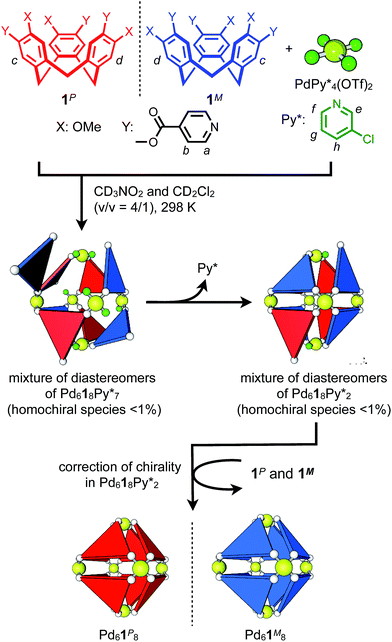 |
| Fig. 1 Chiral self-sorting process of homochiral Pd618 capsules from a racemic mixture of 1 and (Py* = 3-chloropyridine) in CD3NO2 and CD2Cl2 (v/v = 4/1) at 298 K. | |
Results and discussion
Self-assembly of the Pd618 capsule from one-handed tritopic ligand and Pd(II) ions
Racemization of chiral CTV derivatives takes place by heating through the crown-to-crown flip of the CTV ring (Eact = 26.5 kcal mol−1),5 so the racemization of the tritopic ligands (1) in the intermediates is also involved in the chiral self-sorting when the self-assembly is carried out at high temperature. To investigate a strict chiral self-sorting process, avoiding the racemization of the chiral ligand, mild conditions under which the capsule formation takes place without heating are needed. Recently we found that the leaving ligands (X) on a Pd(II) ion source, [PdX4]2+, affect the coordination self-assembly process of a capsule6 and a cage.7 Compared to the generally employed [Pd(CH3CN)4]2+,
(Py* = 3-chloropyridine) tends to produce thermodynamically stable assembled structures much faster at room temperature, despite the coordination ability of Py* being stronger than that of CH3CN. Thus the self-assembly of the Pd618 capsule from one-handed 1, which is indicated as 1* hereafter, and
in CD3NO2 and CD2Cl2 (v/v = 4/1) at 298 K was carried out and monitored by 1H NMR spectroscopy (Fig. 2a). As expected, the self-assembly of the capsule from 1* smoothly took place at 298 K. On the other hand, when Pd(CH3CN)4(OTf)2 was used as a metal source instead of
, the signals assigned to the capsule were not observed (Fig. S1a†). A similar result was found even when the self-assembly was carried out in a strongly coordinative solvent, DMSO-d6, at 298 K (Fig. S1b†).
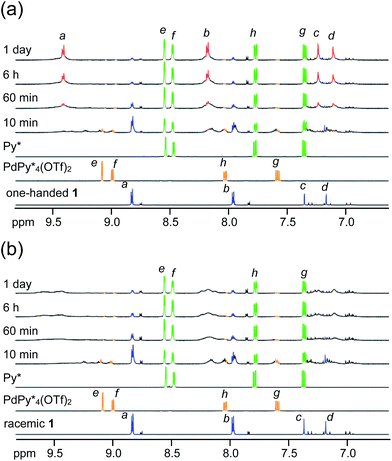 |
| Fig. 2 Partial 1H NMR spectra (500 MHz, aromatic region, CD3NO2 and CD2Cl2 (v/v = 4/1), 298 K) of the reaction mixture of the self-assembly of the homochiral Pd618 capsule(s) from and (a) one-handed 1, 1* ([1*]0 = 2.2 mM) and (b) a racemic mixture of 1 ([1]0 = 2.2 mM). The lettering refers to that shown in Fig. 1. | |
QASAP for the self-assembly of the Pd618 capsule from one-handed tritopic ligands and Pd(II) ions
The self-assembly process of the capsule from 1* was analyzed by QASAP. The existence ratios of the substrates (1* and
), the products (
and Py*), and all the intermediates (Int), whose 1H NMR signals were not observed (Fig. 2a), are shown in Fig. 3a and b, S2a and Tables S1–S4.† One of the features of this self-assembly is that both the substrates remain until the end of the self-assembly. Every intermediate produced in the self-assembly is expressed as
, where a–c are positive integers or 0. As the information about the intermediates obtained by QASAP is the average composition of all the intermediates,
, a further investigation was carried out by n–k analysis. The change in the intermediates is quantitatively analyzed by the time variation of two parameters, 〈n〉 and 〈k〉, which are derived from
. The n value indicates the average number of Pd(II) ions binding to a single tritopic ligand (〈n〉 = (4〈a〉 − 〈c〉)/〈b〉), while the 〈k〉 value indicates the composition ratio of the Pd(II) ion and the tritopic ligand (〈k〉 = 〈a〉/〈b〉).4
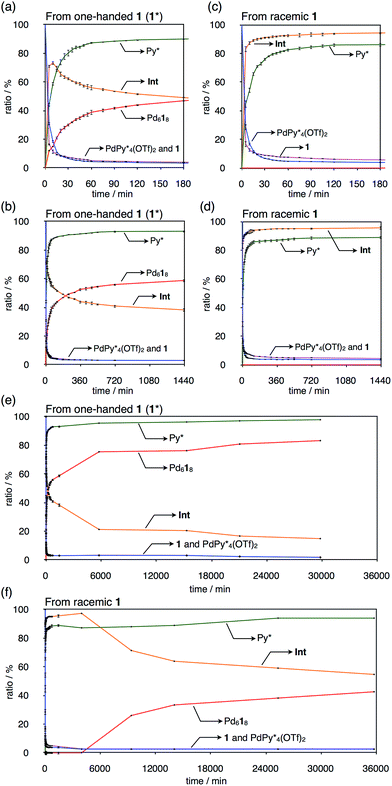 |
| Fig. 3 Existence ratios of the substrates ( and 1), the products (Pd618 and Py*), and all the intermediates (Int) for the self-assembly of the homochiral Pd618 capsule(s) from 1 and in CD3NO2 and CD2Cl2 (v/v = 4/1) at 298 K. (a) From one-handed 1, 1* ([1*]0 = 2.2 mM) (from 0 to 180 min). (b) From one-handed 1, 1* ([1*]0 = 2.2 mM) (from 0 to 1 day). (c) From a racemic mixture of 1 ([1]0 = 2.2 mM) (from 0 to 180 min). (d) From a racemic mixture of 1 ([1]0 = 2.2 mM) (from 0 to 1440 min). (e) From a racemic mixture of 1 ([1]0 = 2.2 mM) (from 0 to 30 000 min). (f) From a racemic mixture of 1 ([1]0 = 2.2 mM) (from 0 to 36 000 min). Blue, magenta, red, green, and orange lines indicate PdPy4(OTf)2, 1, Pd618, Py*, and Int, respectively. | |
The change in the 〈n〉 and 〈k〉 values with time is shown in Fig. 4a and b. At 5 min, the (〈n〉, 〈k〉) value was plotted around the (n, k) values of species containing fewer components than the capsule and then both 〈n〉 and 〈k〉 values increased with time to reach around (6, 8, 7) and (6, 8, 6) at 20 min ((a, b, c) indicates
). The increase in the 〈n〉 and 〈k〉 values reflects the incorporation of free ligands (1*) and Pd(II) ions with a release of Py* molecules through intra- and/or intermolecular ligand exchanges. After 20 min, the 〈n〉 value increased with no significant change in the 〈k〉 value and the (〈n〉, 〈k〉) value finally converged to the (n, k) values of
and
(Fig. 4b). This result indicates that the intramolecular ligand exchanges in
took place after 20 min and led to a mixture of
and
and that the further intramolecular ligand exchanges within them became slow. A similar trend that the intramolecular ligand exchange in the late stage of the self-assembly becomes the rate-determining step was found in the self-assembly of Pd6L8 capsules from hexaphenylbenzene-based tritopic ligands8 and of a Pd2L4 cage from ditopic ligands possessing two pyridyl groups connected to a benzene ring through an ethynyl spacer.7
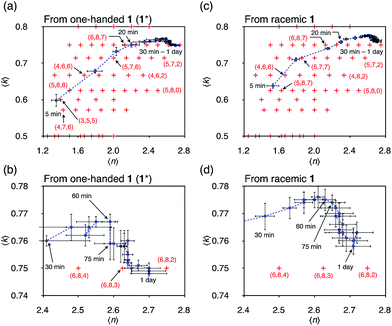 |
| Fig. 4
n–k plots for the self-assembly of the homochiral Pd618 capsule(s) from 1 and in CD3NO2 and CD2Cl2 (v/v = 4/1) at 298 K. (a and b) From one-handed 1, 1* ([1*]0 = 2.2 mM). (c and d) From a racemic mixture of 1 ([1]0 = 2.2. mM). Red crosshairs indicate the (n, k) value of (a ≤ 6 and b ≤ 8). (a, b, c) indicates . | |
Monitoring of the self-assembly of the Pd618 capsule from one-handed tritopic ligands and Pd(II) ions by mass spectrometry
The self-assembly of the capsule from 1* and
was monitored by ESI-TOF mass spectrometry (Fig. S3†) and the mainly observed species are summarized in Table 1. The species that contain more components than the capsule were not found. In most species (indicated by underlining in Table 1), Pd(II) ion(s) is/are coordinately unsaturated. As no liberation of Py* from
occurred in CD3NO2 and CD2Cl2 (v/v = 4/1) at 298 K (confirmed by 1H NMR), these coordinately unsaturated species should be produced during the ionization process,6 which suggests that the intermediates should have more Py* molecules than were detected by mass spectrometry. With this in mind, the signals for
(=(6, 8, 0)) should derive from not only the capsule but also
(c > 0). Thus
(c = 2–7), whose existence is proposed by n–k analysis, should be detected as
by mass spectrometry. On the other hand,
(=(3, 8, 3)) has more Py* molecules than the maximum number of 2, which would be generated by the addition of Py* to
(c = 0–2) during the ionization.
Table 1 Time variation of the
designated (a, b, c) species detected by ESI-TOF mass spectrometry for the self-assembly of the
capsule from
and 1* ([1*] = 2.2 mM) in CD3NO2 and CD2Cl2 (v/v = 4/1) at 298 K
5 min |
10 min |
30 min |
60 min |
120 min |
180 min |
360 min |
Underlines indicate the species whose Pd(II) ion center(s) is/are coordinately unsaturated.
|
|
|
|
|
|
|
|
|
|
|
|
|
|
|
|
|
|
|
|
|
|
|
|
|
|
|
|
|
|
|
|
|
|
|
|
|
|
|
|
|
|
|
|
|
|
|
|
|
|
|
|
|
|
|
|
|
Chiral self-sorting process in the formation of the Pd618 capsule revealed by QASAP
The self-assembly of the capsule from a racemic mixture of 1 under the same conditions was monitored by 1H NMR spectroscopy (Fig. 2b). Though the consumption of the substrates and the release of Py* were observed, no signals for the homochiral capsule were found until 1 day and the signals for the homochiral capsules appeared after 1 week (Fig. 2b and 3f). The change in the existence ratios of the substrates and Py* in the beginning of the self-assembly from a racemic mixture of 1 is similar to that from 1* but the formation of the homochiral capsules was significantly retarded (Fig. 3e and f). This result indicates that the heterochiral species that are difficult to convert into homochiral ones were predominantly produced. The change in the 〈n〉 and 〈k〉 values with time for a racemic mixture of 1 is similar to that for 1* (Fig. 4c and d). Monitoring the self-assembly by ESI-TOF mass spectrometry also detected the same species as in the self-assembly from 1* (Fig. S4†). These results indicate that the self-assembly of the capsule from a racemic mixture of 1 takes place as follows. In the beginning of the self-assembly, small species are produced and then grow by the incorporation of the substrates to lead to the heterochiral isomers of
(c = 6 and 7), which are converted into the heterochiral
immature capsules (ICs) by intramolecular ligand exchanges. As the capsule formation was not observed until 1 day,
should be kinetically trapped. The heterochiral Pd618 capsules have many diastereomeric isomers with different composition ratios of the enantiomers of 1 and with different arrangements of the enantiomers on the faces of the octahedron, which should cause many chemically inequivalent 1H NMR signals. Thus there remains the possibility that the signals for the heterochiral capsules are included in significant broad signals (Fig. 2b). However, the formation of the heterochiral capsules would be excluded by the fact that in the case of the self-assembly from a racemic mixture of 1 the release ratio of Py* is lower than that from 1* (Fig. 3), and that the (〈n〉, 〈k〉) values in both cases after convergence are similar to each other (Fig. 4). If a non-negligible amount of the heterochiral capsules is included in Int, higher 〈n〉 values for a racemic mixture of 1 than that for 1* are expected. The difficulty in the intramolecular ligand exchanges in the heterochiral ICs is due to the mismatch between the enantiomers of 1, which would cause higher energy barriers of the intramolecular ligand exchanges in the heterochiral ICs.
If the self-assembly of the capsule takes place under the statistical situation, the formation ratio of the homochiral capsules, which are composed of eight ligands with the same chirality (SSSSSSSS and RRRRRRRR), is 0.78%. The statistical formation of the mixture of the ICs is thus consistent with no observation of the signals for the homochiral capsules until 1 day and the formation of the mixture of the ICs should occur in a statistical manner if there is no preference of some of the heterochiral species. The statistical self-assembly is reasonable considering the high flexibility of the pyridyl groups connected to the rim of CTV by ester bonds. The signals for the homochiral capsules appeared after 1 week and the yield of the homochiral capsules was as high as 40% at 25 days (Fig. 3f), which indicates that the homochiral self-sorting took place after 1 day. Considering the associative ligand exchange mechanism on Pd(II) centers, the free tritopic ligands 1 remaining in the reaction mixture would trigger biasing the chirality of the heterochiral ICs toward homochirality.9
Conclusions
In conclusion, the chiral self-sorting process in the formation of homochiral capsules from a racemic mixture of CTV-based tritopic ligands, 1P and 1M, and
was revealed by QASAP under mild conditions for which the racemization of 1 does not take place. As far as the composition ratio of the components in the intermediates is concerned, the intermediates produced in the self-assembly from one-handed 1 (1*) and that from a racemic mixture of 1 are quite similar. It was found that no chiral self-sorting took place until the formation of a diastereomeric and structural mixture of the
immature capsules (ICs), whose intramolecular ligand exchanges were prevented by the mismatch of the chirality between the chiral ligands in the heterochiral ICs and that the homochiral self-sorting took place by the correction of the chirality through the rearrangement of the components in the heterochiral ICs. Although the homochiral capsules are the most stable under thermodynamic control, significantly low or no chiral self-sorting occurred until the late stage of the self-assembly, which is because of the high flexibility of the pyridyl groups connected to the rim of CTV by ester bonds.
Conflicts of interest
There are no conflicts to declare.
Acknowledgements
This research was supported by JSPS Grants-in-Aid for Scientific Research on Innovative Areas “Dynamical Ordering of Biomolecular Systems for Creation of Integrated Functions” (25102001 and 25102005), The Asahi Glass Foundation, and the Leverhulme Trust (RPG-2014-148).
References
-
(a) H. Jędrzejewska and A. Szumna, Chem. Rev., 2017, 117, 4863–4899 CrossRef PubMed;
(b) L.-J. Chen, H.-B. Yang and M. Shionoya, Chem. Soc. Rev., 2017, 46, 2555–2576 RSC;
(c) M. Liu, L. Zhang and T. Wang, Chem. Rev., 2015, 115, 7304–7397 CrossRef CAS PubMed.
-
(a) J. Canceill, A. Collet, J. Gabard, G. Gottarelli and G. P. Spada, J. Am. Chem. Soc., 1985, 107, 1299–1308 CrossRef CAS;
(b) J. Canceill, A. Collet and G. Gottarelli, J. Am. Chem. Soc., 1984, 106, 5997–6003 CrossRef CAS;
(c) A. Collet and G. Gottarelli, J. Am. Chem. Soc., 1981, 103, 204–205 CrossRef CAS;
(d) A. Collet and J. Jacques, Tetrahedron Lett., 1978, 19, 1265–1268 CrossRef.
-
(a) T. K. Ronson, J. Fisher, L. P. Harding and M. J. Hardie, Angew. Chem., Int. Ed., 2007, 46, 9086–9088 CrossRef CAS PubMed;
(b) T. K. Ronson, C. Carruthers, J. Fisher, T. Brotin, L. P. Harding, P. J. Rizkal-lah and M. J. Hardie, Inorg. Chem., 2010, 49, 675–685 CrossRef CAS PubMed;
(c) J. J. Henkelis, J. Fisher, S. L. Warriner and M. J. Hardie, Chem.–Eur. J., 2014, 20, 4117–4125 CrossRef CAS PubMed;
(d) N. J. Cookson, J. J. Henkelis, R. J. Ansell, C. W. G. Fishwick, M. J. Hardie and J. Fisher, Dalton Trans., 2014, 43, 5657–5661 RSC.
-
(a) S. Hiraoka, Chem. Rec., 2015, 15, 1144–1147 CrossRef CAS PubMed;
(b) A. Baba, T. Kojima and S. Hiraoka, J. Am. Chem. Soc., 2015, 137, 7664–7667 CrossRef CAS PubMed.
- A. Collet and J. Gabard, J. Org. Chem., 1980, 45, 5400–5401 CrossRef CAS.
- S. Kai, Y. Sakuma, T. Mashiko, T. Kojima, M. Tachikawa and S. Hiraoka, Inorg. Chem., 2017, 56, 12652–12663 CrossRef CAS PubMed.
- S. Kai, V. Marti-Centelles, Y. Sakuma, T. Mashiko, T. Kojima, U. Nagashima, M. Tachikawa, P. J. Lusby and S. Hiraoka, Chem.–Eur. J., 2018, 24, 663–671 CrossRef CAS PubMed.
- Y. Tsujimoto, T. Kojima and S. Hiraoka, Chem. Sci., 2014, 5, 4167–4172 RSC.
-
(a)
M. L. Tobe and J. Burgess, Inorganic Reaction Mechanisms, Longman, London, UK, 1999 Search PubMed;
(b)
F. Basolo and R. G. Pearson, Mechanisms of Inorganic Reactions: A Study of Metal Complexes in Solution, John Wiley, and Sons, Inc., New York, 1967 Search PubMed;
(c) J. Cooper and T. A. Ziegler, Inorg. Chem., 2002, 41, 6614–6622 CrossRef CAS PubMed.
Footnote |
† Electronic supplementary information (ESI) available: Experimental details, additional data, 1H NMR spectra, ESI-TOF mass spectra and QASAP data. See DOI: 10.1039/c8sc01062e |
|
This journal is © The Royal Society of Chemistry 2018 |
Click here to see how this site uses Cookies. View our privacy policy here.