DOI:
10.1039/C7SC05411D
(Edge Article)
Chem. Sci., 2018,
9, 2981-2985
Tailored trisubstituted chiral CpxRhIII catalysts for kinetic resolutions of phosphinic amides†
Received
21st December 2017
, Accepted 3rd February 2018
First published on 5th February 2018
Abstract
A trisubstituted chiral Cpx ligand family is introduced. Based on the disubstituted atropchiral Cpx ligand scaffold, the introduction of a bulky third substituent at the central position of the Cp ring leads to substantially increased selectivities for rhodium(III)-catalyzed kinetic resolutions and allowed for s-factors of up to 50. Their superiority is demonstrated by kinetic resolutions of phosphinic amides providing access to compounds with stereogenic phosphorus(V) atoms. The unreacted acyclic phosphinic amide and the cyclized product are both obtained in good yields and enantioselectivities. The ligand synthesis capitalizes on a late stage modification and expands the accessible ligand Cpx ligand portfolio.
Introduction
Molecules possessing a chiral phosphorus(III)1 as well as phosphorus(V) center2 are important compounds classes with widespread applications. The catalytic and stereoselective synthesis of molecules with chiral phosphorus centers received significant attention,3 but remains a challenging task. Additional and complementary methods for their selective preparations are highly desirable and would be synthetically very valuable. In this respect, catalytic enantioselective C–H functionalizations have emerged a complementary tactic to access chiral building blocks from simple starting materials.4 To the best of our knowledge, the synthesis of P-stereogenic centers by catalytic C–H functionalizations is so far limited to desymmetrization reactions of achiral precursors.5 For instance, we have recently reported the formation of cyclic phosphinic amides catalyzed by chiral CpxRhIII-catalysts.5g,6,7 This desymmetrization approach is only suitable for substrates containing the same two aromatic substituents with identical prochiral C–H groups, thus restricting the obtainable structural diversity. Notably, acyclic P-stereogenic phosphinic amides8 are not accessible by this approach.
Herein, we report a flexible route to new trisubstituted Cpx ligands. We showcase their potential for an enantioselective CpxRhIII-catalyzed C–H functionalization of racemic phosphinic amides through a kinetic resolution9 (Scheme 1). s-Factors of up to 50 were realized with the new tri-substituted Cpx ligands, largely outperforming any previous Cpx ligand.
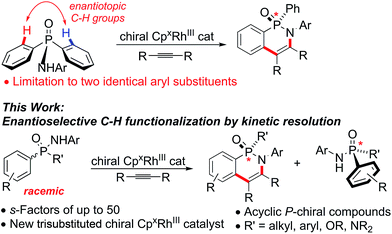 |
| Scheme 1 RhIII-catalyzed desymmetrization and kinetic resolution approaches for the synthesis P-chiral compounds. | |
Conceptually, both acyclic starting material enantiomers can coordinate to the CpxRhIII catalyst forming diastereomeric intermediates I and IV (Scheme 2). In the subsequent – likely rate-limiting step of the process – the matching enantiomer would undergo C–H activation leading to rhodacycle II much faster than IV to V, corresponding to k2 ≫ k5. Experimental support of this assumption consists in the absence of ortho-C-deuteration of unreacted starting material (see ESI† for details). Rhodacycle II can further react with alkyne viaIII to deliver cyclic phosphinic amide 2a. The unreactive complex IV reverts back to the RhIII-catalyst and substrate 1a. Over time, the reacting enantiomer is removed from the starting material, get enriched over time.
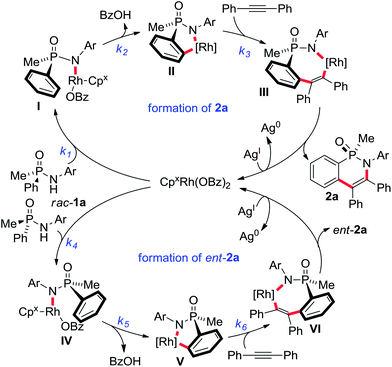 |
| Scheme 2 Kinetic resolution by a difference in the cyclometalation rates k2 > k5. | |
Results and discussion
The initial feasibility of the kinetic resolution was explored with racemic phenyl methyl phosphinic amide rac-1a and diphenyl acetylene (Table 1). Complex Rh1 with our standard second generation Cpx ligand (R = OMe)10a caused a moderate rate difference in the reaction of the two starting material enantiomers, correlating to an s-factor of 14 (entry 1).11,12 Cpx ligands with other substitutions R10 were largely inferior (entries 2–5). We hypothesized that a new trisubstituted Cpx ligand class with increased bulk may enhance the selection between matched and mismatched substrate. These ligands are straightforward prepared from L1 by condensation with a ketone forming the corresponding fulvenes 3 and 4 (Scheme 3).13 Reduction of 3 with LiAlH4 gave isopropyl-substituted ligand L6. X-ray crystallographic analysis of [L6RhCl2]2 showed the orientation of the installed isopropyl group.14 Moreover, facile addition of lithium organometallics across the fulvene double bond provided access to ligands L7-L10 with bulky tert-butyl analogues as third Cp substituent. The corresponding rhodium complexes were subsequently evaluated for the kinetic resolution. In this respect, Rh6 with an isopropyl group R′ largely improved the s-value to 32 (entry 6). Rh7 featuring a larger tert-butyl group further improved the selectivity to an s-value of 42 (entry 7). Rh8 and Rh9 were slightly inferior (entries 8 and 9). Rh10 featuring a 1-butylcyclohexyl group R′ allowed for a significant reduction in reaction time to 3 h maintaining a high s value of 41 (entry 10). Moreover, the amount of diphenyl acetylene could be lower to 0.6 equivalents, improving the selectivity further (entries 11–12).
Table 1 Screening of the Cpx ligands for the kinetic resolutiona
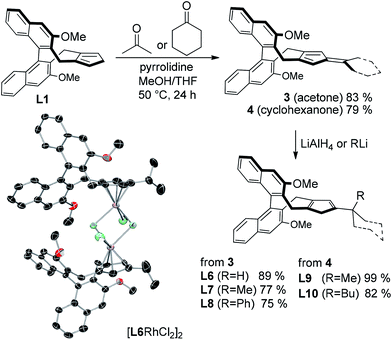 |
| Scheme 3 Synthesis of trisubstituted L6–L10 from L1. | |
Next, the scope of the kinetic resolution procedure was evaluated (Table 2). Steric and electronic variations of R on the aryl group in the para- and meta-position had little influence on the reaction performance (entries 1–8). In all cases, the reaction of rac-1a–1h could be stopped at around 50% conversion. Both products were isolated in good to excellent enantioselectivities, resulting in s-values ranging from 26–50. An ortho-substituted aryl group (rac-1i) largely equalled the reaction rates of both starting material enantiomers and reduced the s-values. Besides variations of the aryl group, substituent R′ can be varied, maintaining synthetically useful selectivities (entries 10–11). In addition to phosphinic amides, we evaluated phosphonodiamidates rac-1l and rac-1m (entries 12–13) as well as phosphonamidate esters rac-1n and rac-1o (entries 14–15) towards the resolutions conditions.15 The additional hetero atom on the phosphorus center had only a weak influence on the reactivity, whereas the s-values were lower with the current ligand system.
Table 2 Kinetic resolution of aryl phosphinic amides rac-1a
The nature of alkyne 5 can be varied as well (Table 3). Whereas electron-rich diaryl alkyne 5b reacted less selective, unsymmetrically substituted internal alkynes such as 5c, 5d and 5e provided comparable selectivities. Moreover, they are incorporated in a highly regioselective manner, giving 2ac, 2ad and 2ae with excellent regioselectivities.
Table 3 Variation of the alkyne 5a
In addition, we have as well investigated racemic phosphinic amide substrate rac-1p having two different aryl groups (eqn (1)). In this case, a parallel kinetic resolution16 becomes operative, yielding the two cyclic phosphinic amide products 2p and 2p′ in excellent yield and good enantioselectivity.
| 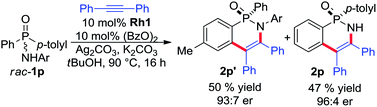 | (1) |
The P-chiral phosphinic amides were subsequently evaluated as chiral Lewis-bases in enantioselective reductive aldol additions17 between enone 6 and benzaldehyde (Scheme 4). Aldol adduct syn-7 was obtained in 96
:
4 er with 13
:
1 syn/anti ratio using methyl-substituted 2a as the catalyst. In stark contrast, related phenyl-substituted phosphinic amide 8 obtained by the previous desymmetrization method5g provided isomer anti-7 preferentially (1
:
4 syn/anti), with almost no enantioselectivity. Moreover, an enantiospecific access to corresponding PIII-compound was achieved by transfer reduction with P(NMe2)3via its thiophosphinic amide 9,18 providing 10 in 90% yield and 99.5: 0.5 er with retention of the configuration. Subsequent use of 10 as a ligand in rhodium catalyzed asymmetric hydrogenation19 of 11 provided reduced product 12 in 99% yield and 86
:
14 er.
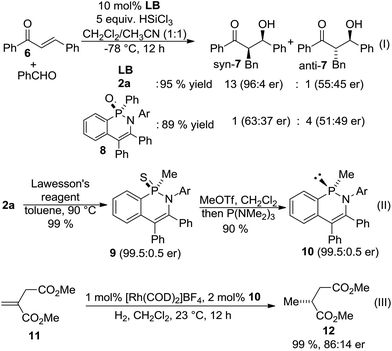 |
| Scheme 4 (I) Comparison of phosphinamides 2a and 8 in enantioselective reductive aldol reactions. (II) Enantiospecific reduction of 2a (2a: >99.5 : 0.5 er). (III) Rh-catalyzed asymmetric hydrogenation with 10 as chiral ligand. | |
Conclusions
In summary, we have developed a new trisubstituted Cpx ligand family. Based on our atropchiral Cpx ligand scaffold, we have shown that the introduction of a bulky third substituent at the central position of the Cp ring leads to substantially increased selectivities for kinetic resolutions and allowed for s-factors of up to 50. The superiority of them are showcased by kinetic resolutions amides providing access to compounds with stereogenic phosphorus(V) atoms. The ligand synthesis capitalizes on a late stage modification and expands the accessible ligand Cpx ligand portfolio for further catalytic-enantioselective transformations with additional metals.
Conflicts of interest
There are no conflicts of interest to declare.
Acknowledgements
This work is supported by the Swiss National Science Foundation (no 157741). We thank Dr R. Scopelliti and Dr F. Fadaei Tirani for X-ray crystallographic analysis of compounds [L6RhCl2]2 and (S)-1a.
Notes and references
-
(a)
P-Stereogenic Ligands in Enantioselective Catalysis ed. A. Grabulosa, RSC, Cambridge, 2011 Search PubMed
;
(b)
Phosphorus(III) Ligands in Homogeneous Catalysis: Design and Synthesis, ed. P. C. J. Kamer and P. W. N. M. v. Leeuwen, Wiley, Hoboken, 2012 Search PubMed
.
-
(a) S. E. Denmark and G. L. Beutner, Angew. Chem., Int. Ed., 2008, 47, 1560 CrossRef CAS PubMed
;
(b)
Phosphorus Chemistry I: Asymmetric Synthesis and Bioactive Compounds, Top. Curr. Chem., ed. J. L. Montchamp, 2015, vol. 360 Search PubMed
;
(c) U. Pradere, E. C. Garnier-Amblard, S. J. Coats, F. Amblard and R. F. Schinazi, Chem. Rev., 2014, 114, 9154 CrossRef CAS PubMed
.
- Recent overviews:
(a) D. S. Glueck, Chem.–Eur. J., 2008, 14, 7108 CrossRef CAS PubMed
;
(b) J. S. Harvey and V. Gouverneur, Chem. Commun., 2010, 46, 7477 RSC
;
(c) M. Dutarte, J. Bayardon and S. Jugé, Chem. Soc. Rev., 2016, 45, 5771 RSC
;
(d)
Asymmetric Synthesis in Organophosphorus Chemistry, ed. O. I. Kolodiazhnyi, Wiley-VCH, Weinheim, 2016 Search PubMed
; selected examples: chiral auxilliary-based:
(e) Z. S. Han, N. Goyal, M. A. Herbage, J. D. Sieber, B. Qu, Y. Xu, Z. Li, J. T. Reeves, J.-N. Desrosiers, S. Ma, N. Grinberg, H. Lee, H. P. R. Mangunuru, Y. Zhang, D. Krishnamurthy, B. Z. Lu, J. J. Song, G. Wang and C. H. Senanayake, J. Am. Chem. Soc., 2013, 135, 2474 CrossRef CAS PubMed
; desymmetrization with a chiral base:
(f) J. J. Gammon, V. H. Gessner, G. R. Barker, J. Granander, A. C. Whitwood, C. Strohmann, P. O'Brien and B. Kelly, J. Am. Chem. Soc., 2010, 132, 13922 CrossRef CAS PubMed
; desymmetrization of enantiotopic alkyne groups:
(g) G. Nishida, K. Noguchi, M. Hirano and K. Tanaka, Angew. Chem., Int. Ed., 2008, 47, 3410 CrossRef CAS PubMed
; organo-catalytic esterification of enantiotopic phenols:
(h) Z. Huang, X. Huang, B. Li, C. Mou, S. Yang, B.-A. Song and Y. R. Chi, J. Am. Chem. Soc., 2016, 138, 7524 CrossRef CAS PubMed
; enantioselective addition to secondary phosphines and phosphine oxides:
(i) C. Scriban and D. S. Glueck, J. Am. Chem. Soc., 2006, 128, 2788 CrossRef CAS PubMed
;
(j) V. S. Chan, M. Chiu, R. G. Bergman and F. D. Toste, J. Am. Chem. Soc., 2009, 131, 6021 CrossRef CAS PubMed
;
(k) Y. Huang, Y. Li, P.-H. Leung and T. Hayashi, J. Am. Chem. Soc., 2014, 136, 4865 CrossRef CAS PubMed
;
(l) R. Beaud, R. J. Phipps and M. J. Gaunt, J. Am. Chem. Soc., 2016, 138, 13183 CrossRef CAS PubMed
.
-
(a) R. Giri, B.-F. Shi, K. M. Engle, N. Maugel and J.-Q. Yu, Chem. Soc. Rev., 2009, 38, 3242 RSC
;
(b) J. Wencel-Delord and F. Colobert, Chem.–Eur. J., 2013, 19, 14010 CrossRef CAS PubMed
;
(c) C. Zheng and S.-L. You, RSC Adv., 2014, 4, 6173 RSC
;
(d)
Asymmetric Functionalization of C–H Bonds, ed. S.-L. You, RSC, Cambridge, 2015 Search PubMed
;
(e) C. G. Newton, S.-G. Wang, C. C. Oliveira and N. Cramer, Chem. Rev., 2017, 117, 8908 CrossRef CAS PubMed
.
-
(a) Z.-Q. Lin, W.-Z. Wang, S.-B. Yan and W.-L. Duan, Angew. Chem., Int. Ed., 2015, 54, 6265 CrossRef CAS PubMed
;
(b) L. Liu, A.-A. Zhang, Y. Wang, F. Zhang, Z. Zuo, W.-X. Zhao, C.-L. Feng and W. Ma, Org. Lett., 2015, 17, 2046 CrossRef CAS PubMed
;
(c) G. Xu, M. Li, S. Wang and W. Tang, Org. Chem. Front., 2015, 2, 1342 RSC
;
(d) Z.-J. Du, J. Guan, G.-J. Wu, P. Xu, L.-X. Gao and F.-S. Han, J. Am. Chem. Soc., 2015, 137, 632 CrossRef CAS PubMed
;
(e) D. Gwona, S. Park and S. Chang, Tetrahedron, 2015, 71, 4504 CrossRef
;
(f) T. T. Nguyen, L. Grigorjeva and O. Daugulis, ACS Catal., 2016, 6, 551 CrossRef CAS PubMed
;
(g) Y. Sun and N. Cramer, Angew. Chem., Int. Ed., 2017, 56, 364 CrossRef CAS PubMed
;
(h) Y.-S. Jang, M. Dieckmann and N. Cramer, Angew. Chem., Int. Ed., 2017, 56, 15088 CrossRef CAS PubMed
.
- Seminal work and overviews of chiral Cpx-metal catalyst for asymmetric C–H functionalization:
(a) T. K. Hyster, L. Knörr, T. R. Ward and T. Rovis, Science, 2012, 338, 500 CrossRef CAS PubMed
;
(b) B. Ye and N. Cramer, Science, 2012, 338, 504 CrossRef CAS PubMed
;
(c) B. Ye and N. Cramer, Acc. Chem. Res., 2015, 48, 1308 CrossRef CAS PubMed
;
(d) C. G. Newton, D. Kossler and N. Cramer, J. Am. Chem. Soc., 2016, 138, 3935 CrossRef CAS PubMed
.
- Recent examples of catalytic applications in Rh-catalysis:
(a) B. Ye, P. A. Donets and N. Cramer, Angew. Chem., Int. Ed., 2014, 53, 507 CrossRef CAS PubMed
;
(b) B. Ye and N. Cramer, Angew. Chem., Int. Ed., 2014, 53, 7896 CrossRef CAS PubMed
;
(c) J. Zheng and S.-L. You, Angew. Chem., Int. Ed., 2014, 53, 13244 CrossRef CAS PubMed
;
(d) J. Zheng, S.-B. Wang, C. Zheng and S.-L. You, J. Am. Chem. Soc., 2015, 137, 4880 CrossRef CAS PubMed
;
(e) S. R. Chidipudi, D. J. Burns, I. Khan and H. W. Lam, Angew. Chem., Int. Ed., 2015, 54, 13975 CrossRef PubMed
;
(f) M. V. Pham and N. Cramer, Chem.–Eur. J., 2016, 22, 2270 CrossRef CAS PubMed
;
(g) J. Zheng, W.-J. Cui, C. Zheng and S.-L. You, J. Am. Chem. Soc., 2016, 138, 5242 CrossRef CAS PubMed
;
(h) J. Zheng, S.-B. Wang, C. Zheng and S.-L. You, Angew. Chem., Int. Ed., 2017, 56, 4540 CrossRef CAS PubMed
;
(i) Z.-J. Jia, C. Merten, R. Gontla, C. G. Daniliuc, A. P. Antonchick and H. Waldmann, Angew. Chem., Int. Ed., 2017, 56, 2429 CrossRef CAS PubMed
;
(j) G. Smits, B. Audic, M. D. Wodrich, C. Corminboeuf and N. Cramer, Chem. Sci., 2017, 8, 7174 RSC
.
- For the pharmaceutical relevance of acyclic P-stereogenic compounds, see:
(a) S. Pikul, K. L. McDow Dunham, N. G. Almstead, B. De, M. G. Natchus, M. V. Anastasio, S. J. McPhail, C. E. Snider, Y. O. Taiwo, L. Chen, C. M. Dunaway, F. Gu and G. E. Mieling, J. Med. Chem., 1999, 42, 87 CrossRef CAS PubMed
;
(b) M. J. Sofia, D. Bao, W. Chang, J. Du, D. Nagarathnam, S. Rachakonda, P. G. Reddy, B. S. Ross, P. Wang and H. R. Zhang,
et al.
, J. Med. Chem., 2010, 53, 7202 CrossRef CAS PubMed
;
(c) F.-R. Alexandre, A. Amador, S. Bot, C. Caillet, T. Convard, J. Jakubik, C. Musiu, B. Poddesu, L. Vargiu, M. Liuzzi, A. Roland, M. Seifer, D. standring, R. storer and C. B. Dousson, J. Med. Chem., 2011, 54, 392 CrossRef CAS PubMed
;
(d) D. A. DiRocco, Y. Ji, E. C. Sherer, A. Klapars, M. Reibarkh, J. Dropinski, R. Mathew, P. Maligres, A. M. Hyde, J. Limanto, A. Brunskill, R. T. Ruck, L. C. Campeau and I. W. Davies, Science, 2017, 356, 426 CrossRef CAS PubMed
.
- Recent examples in C–H functionalization:
(a) C. González-Rodríguez, S. R. Parsons, A. L. Thompson and M. C. Willis, Chem.–Eur. J., 2010, 16, 10950 CrossRef PubMed
;
(b) D.-W. Gao, Q. Gu and S.-L. You, ACS Catal., 2014, 4, 2741 CrossRef CAS
;
(c) L. Chu, K.-J. Xiao and J.-Q. Yu, Science, 2014, 346, 451 CrossRef CAS PubMed
;
(d) K.-J. Xiao, L. Chu, G. Chen and J.-Q. Yu, J. Am. Chem. Soc., 2016, 138, 7796 CrossRef CAS PubMed
;
(e) K. J. Xiao, L. Chu and J.-Q. Yu, Angew. Chem., Int. Ed., 2016, 55, 2856 CrossRef CAS PubMed
.
-
(a) B. Ye and N. Cramer, J. Am. Chem. Soc., 2013, 135, 636 CrossRef CAS PubMed
;
(b) B. Ye and N. Cramer, Synlett, 2015, 26, 1490 CrossRef CAS
;
(c) D. Kossler and N. Cramer, Chem. Sci., 2017, 8, 1862 RSC
.
- Definition s-factor: s = ln[(1 − c)(1 − ee)]/ln[(1 − c)(1 + ee)] (c: conversion; ee: recovered substrate' see).
- The absolute configuration of recovered 1a was determined to be (S) by X-ray-crystallographic analysis.
- N. Cokun and I. Erden, Tetrahedron, 2011, 67, 8607 CrossRef PubMed
.
- CCDC 1588292 ((S)-1a) and 1588293 ([L6RhCl2]2) contain the supplementary crystallographic data for this paper.†.
- For examples of the biological properties of these P(V)-compounds, see:
(a) M. Sawa, T. Kiyoi, K. Kurokawa, H. Kumihara, M. Yamamoto, T. Miyasaka, Y. Ito, R. Hirayama, T. Inoue, Y. Kirii, E. Nishiwaki, H. Ohnoto, Y. Maeda, F. Ishibushi, Y. Inoae, K. Yoshino and H. Kondo, J. Med. Chem., 2002, 45, 919 CrossRef CAS PubMed
;
(b) G. Ruiz-Gómez, A. Francesch, M. J. Iglesias, F. López-Ortiz, C. Cuevas and M. Serrano-Ruiz, Org. Lett., 2008, 10, 3981 CrossRef PubMed
.
- For a review, see:
(a) J. R. Dehli and V. Gotor, Chem. Soc. Rev., 2002, 31, 365 RSC
; for selected recent examples in C–H functionalization:
(b) K. Tanaka and G. C. Fu, J. Am. Chem. Soc., 2003, 125, 8078 CrossRef CAS PubMed
;
(c) D. Katayev, M. Nakanishi, T. Bürgi and E. P. Kündig, Chem. Sci., 2012, 3, 1422 RSC
;
(d) T. Lee, T. W. Wilson, R. Berg, P. Ryberg and J. F. Hartwig, J. Am. Chem. Soc., 2015, 137, 6742 CrossRef CAS PubMed
;
(e) D. Grosheva and N. Cramer, ACS Catal., 2017, 7, 7417 CrossRef CAS
;
(f) J. Pedroni and N. Cramer, J. Am. Chem. Soc., 2017, 139, 12398 CrossRef CAS PubMed
.
- M. Sugiura, N. Sato, Y. Sonoda, S. Kotani and M. Nakajima, Chem.–Asian J., 2010, 5, 478 CrossRef CAS PubMed
.
- Y.-H. Chen, X.-L. Qin and F.-S. Han, Chem. Commun., 2017, 53, 5826 RSC
.
- T. Jerphagnon, J.-L. Renaud and C. Bruneau, Tetrahedron: Asymmetry, 2004, 15, 2101 CrossRef CAS
.
Footnote |
† Electronic supplementary information (ESI) available: Experimental procedures and characterisation of all new compounds. CCDC 1588292 and 1588293. For ESI and crystallographic data in CIF or other electronic format see DOI: 10.1039/c7sc05411d |
|
This journal is © The Royal Society of Chemistry 2018 |
Click here to see how this site uses Cookies. View our privacy policy here.