DOI:
10.1039/C8SC00210J
(Edge Article)
Chem. Sci., 2018,
9, 2986-2990
Copper-catalyzed formylation of alkenyl C–H bonds using BrCHCl2 as a stoichiometric formylating reagent†
Received
14th January 2018
, Accepted 13th February 2018
First published on 14th February 2018
Abstract
The first example of copper-catalyzed direct formylation of alkenyl C–H bonds for the facile synthesis of α,β-unsaturated aldehydes has been developed. This transformation has demonstrated high reactivity, mild reaction conditions and a broad substrate scope. BrCHCl2 is expected to be developed as an efficient stoichiometric C1 building block in organic synthesis.
Introduction
Developing novel methods for the synthesis of complex molecules in an efficient and expeditious manner still remains a major challenge in organic synthesis. Accordingly, many novel strategies for the quick construction of complex molecular skeletons have been developed and used for the total synthesis of complex compounds.1,2 Among all of these strategies, domino reactions are emerging as efficient reactions in the facile synthesis of key intermediates. Of significant interest are cascade reactions that provide access to fundamental building blocks in a chemoselective manner from readily available starting materials in a step-economical fashion.3 As a key material used in cascade transformations, especially in organocatalyzed domino reactions, α,β-unsaturated aldehydes have been used in a broad range of unconventional transformations to make complex molecules or key intermediates.4 Thus, considerable efforts have been devoted to developing novel methods for the facile synthesis of α,β-unsaturated aldehydes from readily available simple raw materials in efficient and quick ways.5
As they are produced on a large scale industrially and have served as an important feedstock for the petrochemical industry, simple alkenes have long been realized as one of the most widely used raw materials for a great variety of organic transformations.6 The direct formylation of simple alkenes thus offers the most economical and efficient method for the facile construction of α,β-unsaturated aldehydes (Scheme 1). Indeed, the Vilsmeier–Haack reaction has long been developed as a useful approach to introduce a formyl group into alkenes.7 On the other hand, using the Grubbs II catalyst as the radical initiator succeeded in the atom transfer radical addition (ATRA) of styrene, which afforded the alkenyl aldehyde via the subsequent elimination of hydrochloric acid under strong acid conditions or a stoichiometric amount of silver salts at high temperature.8 Apart from this interesting progress, both known methods still suffer from a limited scope of alkenes and the requirement for a large amount of poisonous reagents (POCl3 as a precursor of the Vilsmeier reagent or CHCl3 as the solvent), which have definitely hampered their practical application in organic synthesis. Herein, we report a novel copper-catalyzed formylation of alkenyl C–H bonds for the facile synthesis of α,β-unsaturated aldehydes, in which high reactivity, mild conditions and a broad substrate scope have been demonstrated. The key to success is the use of commercially available BrCHCl2 as a stoichiometric formylating reagent instead of CHCl3, which is normally used as a solvent in such reactions. Alkenyl aldehydes were then furnished by the bifunctionalization of alkenes via a carbocation process and subsequent dehydration.
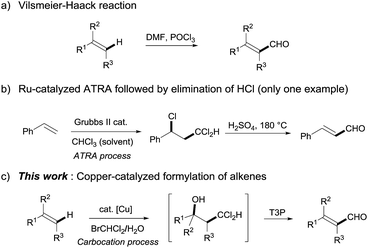 |
| Scheme 1 Facile synthesis of α,β-unsaturated aldehydes from alkenes. | |
Results and discussion
Our initial investigation commenced with 4-methoxystyrene (1a) as the model substrate, and bromodichloromethane as the stoichiometric formylating reagent in the presence of a catalytic amount of a copper catalyst (10 mol%) and 1.0 equiv. of PMDTA (1,1,4,7,7-pentamethyl-diethylenetriamine) in CH3CN at 40 °C (Table 1). Unfortunately, none of the desired product 2a was obtained when CuBr was used as the catalyst. Considering that halogen atom exchange may improve the reactivity of CHBrCl2,9 to our delight, the addition of 1.0 equiv. of KI into the reaction system afforded 2a successfully, albeit with a relatively low yield (23%, entry 2). A careful examination of copper catalysts was next performed, which indicated that Cu(OH)2 gave the best result with a 31% yield (entry 4). Meanwhile, further investigation into various solvents gave no improvement in yield and CH3CN was still the best choice. When a trace amount of the bifunctionalized alcohol 2aa was isolated as a byproduct, it was conjectured that water acted as the hydroxyl source for this bifunctional reaction. As we expected, up to a 28% yield of 2aa was obtained along with a 24% yield of 2a when water was used directly as the solvent. To further improve the yield, co-solvents were investigated and CH3CN/H2O (1/1) gave the dichloromethylated alcohol 2aa in 74% yield along with the aldehyde 2a in 7% yield. After a simple work-up without purification and further dehydration with T3P, 2a was finally furnished in 80% yield (entry 8). Considering the key role that PMDTA played in this transformation,10 other bases were also screened instead of PMDTA, but they did not give the desired product 2a (Table 1, entries 11–13). Notably, by the replacement of KI with NaI, the yield of 2a was further improved to 90% after this two-step formylation of 4-methoxystyrene (entry 15). Finally, control experiments indicated that none of the desired product was obtained without the addition of a copper catalyst or base (for details, see the ESI†).
Table 1 Copper-catalyzed formylation of 4-methoxystyrene: optimization of conditionsa
With the optimized conditions in hand, we next investigated the scope of the alkene (Table 2). Initially, the study of the substituent effect on the aryl rings of the styrene derivatives showed that both electron-donating groups, such as 4-methoxy, 4-phenoxy, 4-methyl and 4-methylthio (1a–d), and weak electron-withdrawing groups, such as fluorine, chlorine and bromine (1f–h), were all compatible with this catalytic system and were able to furnish the desired products in moderate to high yields. Not surprisingly, the substrates with electron-withdrawing groups installed on the aryl rings typically gave lower yields and required relatively high reaction temperatures (2f–h). Meanwhile, a variety of styrene derivatives with ortho- and meta- as well as para-substituents were smoothly formylated to afford the corresponding α,β-unsaturated aldehydes 2i–k in acceptable yields.
General conditions: 1 (0.2 mmol), Cu(OH)2 (10 mol%), CHBrCl2 (3.0 equiv.), PMDTA (1.0 equiv.) and NaI (1.0 equiv.) in CH3CN/H2O (v/v = 1 : 1, 1 mL) at 40 °C for 24 h, then dehydration with T3P (3.0 equiv.) in EtOAc at 100 °C; for 2x–y, 1 (0.2 mmol), Cu(hfacac)2·xH2O (10 mol%), CHBrCl2 (3.0 equiv.), PMDTA (1.0 equiv.) and KI (1.0 equiv.) in CH3CN (1 mL) at 80 °C for 24 h, and aldehydes 2x–y were obtained directly. Isolated yields are reported.
80 °C.
|
|
The ortho-chloro- and meta-methyl-substituted styrenes (1g and 1k, respectively), styrene (1e) and 2-vinylnaphthalene (1l) also required a much higher temperature and gave moderate yields. Although the ortho- and meta-methoxystyrene (1i and 1j, respectively) did not need high temperatures, the yields of the reactions were only moderate. Of interest to us was that heteroarene-derived styrene substrates, including 2-vinylthiophene 1p and 3-vinylbenzothiophene 1q, were also well-tolerated in this novel transformation with synthetically useful yields (2p, 63%; 2q, 75%). Just as expected, 1,1-diphenylethenes 1r–t normally worked as better radical trappers than styrene, and generated tri-substituted unsaturated aldehydes 2r–t in relatively high yields (Table 2, 2r–t). Of note was that the formylation of tri-substituted internal styrene 1u also proceeded smoothly to give the corresponding tetra-substituted vinyl aldehyde with a fairly good yield (2u, 83%). Importantly, the subjection of 1,2-dihydronaphthalene 1v to the optimized conditions resulted in the successful formylation of a C–H bond that was part of the cyclic double bond system. It was also noteworthy that the conjugated alkene 1w underwent the reaction efficiently, with an acceptable yield (2w, 50%) and a good E/Z selectivity (8
:
1). To our satisfaction, N-vinyl substrates could also be directly formylated at the end of terminal alkenes to afford β-aminoacrylaldehydes in moderate yields, by simply altering the conditions to a combination of Cu(hfacac)2·xH2O, KI and CH3CN.14
To gain some insights into the mechanism of this transformation, a series of control experiments were then performed accordingly (Scheme 2). First, the reaction was completely quenched when 1.0 equivalent of 2,2,6,6-tetramethyl-1-piperidinyloxy (TEMPO) was added to the standard conditions. Meanwhile, the addition of 1.0 equivalent of butylated hydroxytoluene (BHT) to the standard conditions afforded the corresponding dichloromethyl adduct 3 in 58% yield. Both results indicated that this transformation may proceed via a radical path. To further test this theory, the well known radical clock 1-(1-cyclopropylvinyl)-4-methoxybenzene 4 was synthesized and added into this catalytic system, furnishing the ring-opened product 5 in 65% yield, strengthening the hypothesis of the dichloromethyl radical (˙CCl2H) being involved in the catalytic cycle.
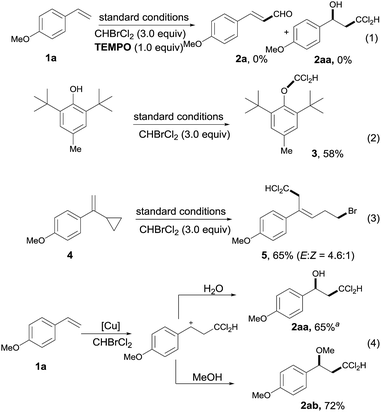 |
| Scheme 2 Mechanistic studies. a For details of the isolation of 2aa, see the ESI.† | |
As alcohol 2aa was isolated as the key intermediate along with the vinyl aldehyde 2a in the model reaction (eqn (4), Scheme 2), it was suggested that a carbocation process was possibly involved in the catalytic cycle. Indeed, the replacement of H2O with methanol as the cation scavenger afforded the corresponding bifunctional product 2ab in 72% yield, which further strengthened this possibility.11
On the basis of our preliminary results and previous reports,12 a plausible mechanism involving a ˙CHCl2 radical is proposed as shown in Scheme 3. The copper salt is reduced to generate a low-valent Cu complex, which can transfer a single electron to CHBrCl2 and then produce the free radical compound ˙CHCl2 (B), and the radical subsequently reacts with the styrene. The ˙CHCl2 addition-compound D can be oxidized by a high-valent Cu complex to the cationic intermediate E. Finally, the high-valent Cu complex is reduced to a low-valent Cu complex to complete the catalytic cycle. There are two possible pathways for the sequential transformation of cation E to vinyl aldehyde F: Path a, which consists of deprotonation to regenerate the C
C bond, and F is obtained by the subsequent hydrolysis of the dichloromethyl group to the aldehyde; or Path b, where the cation E is captured by H2O to give the alcohol intermediate G, followed by dehydration with T3P and subsequent hydrolysis of the dichloromethyl group to afford F.13
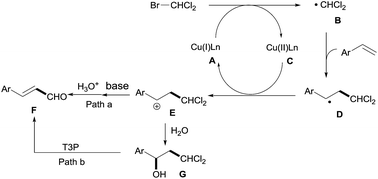 |
| Scheme 3 Proposed mechanism. | |
To demonstrate the synthetic potential of this transformation, we applied this two-step transformation to the late-stage formylation of complex biologically active molecules. As shown in Scheme 4, the estrone-derived aryl alkene 6, which was synthesized from estrone in two steps, was transformed to the desired vinyl aldehyde 7 in a 50% yield. This outcome clearly showed the great potential of this methodology as a facile strategy for the synthesis of various analogues or intermediates in drug discovery and screening.
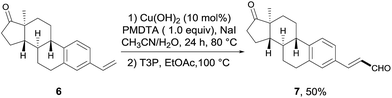 |
| Scheme 4 Formylation of the estrone derivative 6. | |
Conclusions
In summary, we have developed a novel copper-catalyzed formylation of alkenyl C–H bonds for the facile synthesis of α,β-unsaturated aldehydes. This transformation has demonstrated high reactivity, mild conditions and a broad substrate scope. An important point is that commercially available BrCHCl2 was used as a stoichiometric formylating reagent instead of CHCl3. Mechanistic studies indicated that alkenyl aldehydes were furnished by bifunctionalization of alkenes via a carbocation process and subsequent dehydration. Further applications of this method for the modification of bioactive molecules and the use of BrCHCl2 as an interesting C1 building block in organic synthesis are still underway in our laboratory.
Conflicts of interest
There are no conflicts to declare.
Acknowledgements
We gratefully acknowledge the Strategic Priority Research Program of the Chinese Academy of Sciences (grant no. XDB20000000), the National Basic Research Program of China (973 program 2015CB856600), and the National Science Foundation of China (21522208 and 21772187) for financial support.
Notes and references
-
(a)
E. J. Corey and X. M. Cheng, The Logic of Chemical Synthesis, John Wiley & Sons, New York, 1989 Search PubMed;
(b)
B. M. Trost and I. Fleming, Comprehensive Organic Synthesis, vol. 3, Pergamon, Oxford, 1991 Search PubMed;
(c)
P. R. Mackie and C. E. Foster, in Comprehensive Organic Functional Group Transformations II, Pergamon, Oxford, 2005, vol. 3 Search PubMed.
- For selected reviews, see:
(a) A. E. Shilov and G. B. Shul'pin, Chem. Rev., 1997, 97, 2879 CrossRef CAS PubMed;
(b) X. Chen, K. M. Engle, D.-H. Wang and J.-Q. Yu, Angew. Chem., Int. Ed., 2009, 48, 5094 CrossRef CAS PubMed;
(c) O. Daugulis, H.-Q. Do and D. Shabashov, Acc. Chem. Res., 2009, 42, 1074 CrossRef CAS PubMed;
(d) Y. Ishihara and P. S. Baran, Synlett, 2010, 12, 1733 Search PubMed;
(e) S. E. Allen, R. R. Walvoord, R. Padilla-Salinas and M. C. Kozlowski, Chem. Rev., 2013, 113, 6234 CrossRef CAS PubMed;
(f) M. D. Kärkäs, J. A. Porco Jr and C. R. J. Stephenson, Chem. Rev., 2016, 116, 9683 CrossRef PubMed;
(g) J. E. Zweig, D. E. Kim and T. R. Newhouse, Chem. Rev., 2017, 117, 11680 CrossRef CAS PubMed.
- For selected reviews, see:
(a) L. F. Tietze, Chem. Rev., 1996, 96, 115 CrossRef CAS PubMed;
(b) K. C. Nicolaou, D. J. Edmonds and P. G. Buleger, Angew. Chem., Int. Ed., 2006, 45, 7134 CrossRef CAS PubMed;
(c) A. Grossmann and D. Enders, Angew. Chem., Int. Ed., 2012, 51, 314 CrossRef CAS PubMed;
(d) R. Volla, I. Atodiresei and M. Rueping, Chem. Rev., 2014, 114, 2390 CrossRef PubMed;
(e) L. J. Sebren, J. J. Devery III and C. R. J. Stephenson, ACS Catal., 2014, 4, 703 CrossRef CAS PubMed;
(f) Q. Liao, X. Yang and C. Xi, J. Org. Chem., 2014, 79, 8507 CrossRef CAS PubMed.
- For selected reviews, see:
(a) I. K. Mangion and D. W. C. MacMillan, J. Am. Chem. Soc., 2005, 127, 3696 CrossRef CAS PubMed;
(b) S. Brandau, E. Maerten and K. A. Jørgensen, J. Am. Chem. Soc., 2006, 128, 14986 CrossRef CAS PubMed;
(c) D. Enders, C. Grondal and M. R. M. Hüttl, Angew. Chem., Int. Ed., 2007, 46, 1570 CrossRef CAS PubMed;
(d) K. L. Jensen, G. Dickmeiss, H. Jiang, Ł. Albercht and K. A. Jørgensen, Acc. Chem. Res., 2011, 45, 248 CrossRef PubMed;
(e) J.-L. Li, T.-Y. Liu and Y.-C. Chen, Acc. Chem. Res., 2012, 45, 1491 CrossRef CAS PubMed;
(f) C. Graaff, E. Ruijter and R. V. A. Orru, Chem. Soc. Rev., 2012, 41, 3969 RSC;
(g) X. Jiang and R. Wang, Chem. Rev., 2013, 113, 5515 CrossRef CAS PubMed;
(h) S. Afewerki and A. Córdova, Chem. Rev., 2016, 116, 13512 CrossRef CAS PubMed;
(i) V. Marcos and J. Alemán, Chem. Soc. Rev., 2016, 45, 6812 RSC;
(j) L. Klier, F. Tur, P. H. Poulsen and K. A. Jørgensen, Chem. Soc. Rev., 2017, 46, 1080 RSC.
- For selected examples on the synthesis of α,β-unsaturated aldehydes, see:
(a)
I. Escher and F. Glorius, in Science of Synthesis, ed. R. Bruckner, Gerog Thieme, Stuttgart, 2007, vol 25, pp. 733–777 Search PubMed;
(b) T. Jeffery, J. Chem. Soc., Chem. Commun., 1984, 1287 RSC;
(c) A. Zaks and D. R. Dodds, J. Am. Chem. Soc., 1995, 117, 10419 CrossRef CAS;
(d) B. G. V. Hoven and H. Alper, J. Org. Chem., 1999, 64, 9640 CrossRef;
(e) G. Battistuzzi, S. Cacchi and G. Fabrizi, Org. Lett., 2003, 5, 777 CrossRef CAS PubMed;
(f) F. Goettmann, P. L. Floch and C. Sanchez, Chem. Commun., 2006, 180 RSC;
(g) H. Neymann, A. Sergeev and M. Beller, Angew. Chem., Int. Ed., 2008, 47, 4887 CrossRef PubMed;
(h) J. Zhu, J. Liu, R. Ma, H. Xie, J. Li, H. Jiang and W. Wang, Adv. Synth. Catal., 2009, 351, 1229 CrossRef CAS;
(i) J. Liu, J. Zhu, H. Jiang, W. Wang and J. Li, Chem. Commun., 2010, 46, 415 RSC;
(j) H. Chen, H. Jiang, C. Cai, J. Dong and W. Fu, Org. Lett., 2011, 13, 992 CrossRef CAS PubMed;
(k) T. Wang, S.-K. Xiang, C. Qin, J.-A. Ma, L.-H. Zhang and N. Jiao, Tetrahedron Lett., 2011, 52, 3208 CrossRef CAS;
(l) W. Gao, Z. He, Y. Qian, J. Zhao and Y. Huang, Chem. Sci., 2012, 3, 883 RSC;
(m) Z. Zhang, Q. Wang, C. Chen, Z. Han, X.-Q. Dong and X. Zhang, Org. Lett., 2016, 18, 3290 CrossRef CAS PubMed;
(n) R. Haraguchi, S. Tanazawa, N. Tokunaga and S. Fukuzawa, Org. Lett., 2017, 19, 1646 CrossRef CAS PubMed;
(o) M.-M. Wang, X.-S. Ning, J.-P. Qu and Y.-B. Kang, ACS Catal., 2017, 7, 4000 CrossRef CAS;
(p) R. Haraguchi, S. Tanazawa, N. Tokunaga and S. Fukuzawa, Org. Lett., 2017, 19, 1646 CrossRef CAS PubMed;
(q) H. Huang, X. Li, C. Yu, Y. Zhang, P. S. Mariano and W. Wang, Angew. Chem., Int. Ed., 2017, 56, 1500 CrossRef CAS PubMed;
(r) H. Huang, C. Yu, X. Li, Y. Zhang, X. Chen, P. S. Mariano, H. Xie and W. Wang, Angew. Chem., Int. Ed., 2017, 56, 8201 CrossRef CAS PubMed.
-
(a) N. Calderon, Acc. Chem. Res., 1972, 5, 127 CrossRef CAS;
(b) R. R. Schmidt, Acc. Chem. Res., 1986, 19, 250 CrossRef CAS;
(c) T. V. RajanBabu, Chem. Rev., 2003, 103, 2845 CrossRef CAS PubMed;
(d) A. M. Lozano-Vila, S. Monsaert, A. Bajek and F. Verpoort, Chem. Rev., 2010, 110, 4865 CrossRef CAS PubMed;
(e) X.-F. Wu, X. Fang, L. Wu, R. Jackstell, H. Neumann and M. Beller, Acc. Chem. Res., 2014, 47, 1041 CrossRef CAS PubMed.
-
(a) C. J. Schmidle and P. G. Barnett, J. Am. Chem. Soc., 1956, 78, 3209 CrossRef CAS;
(b) C. Jutz and W. Müller, Chem. Ber., 1967, 100, 1536 CrossRef CAS;
(c) E. M. Beccalli, A. Marchesini and T. Pilati, Tetrahedron, 1989, 45, 7485 CrossRef CAS;
(d) A. R. Katritzky and I. V. Shcherbakova, Can. J. Chem., 1992, 70, 2040 CrossRef CAS.
-
(a) B. T. Lee, T. O. Schrader, B. Martin-Matute, C. R. Kauffman, P. Zhang and M. L. Snapper, Tetrahedron, 2004, 60, 7391 CrossRef CAS; For selected examples on formylation using CHCl3, see:
(b) K. Reimer and F. Tiemann, Berufsbildung, 1876, 9, 824 Search PubMed;
(c) J. Hine and J. M. van der Veen, J. Am. Chem. Soc., 1959, 81, 6446 CrossRef CAS;
(d) H. Wynberg, Chem. Rev., 1960, 60, 169 CrossRef CAS.
-
(a) M. R. Prinshell, D. A. Everson and D. J. Weix, Chem. Commun., 2010, 46, 5743 RSC;
(b) F. Liang, Y. Wang, H. Li, J. Li, D. Zou, Y. Wu and Y. Wu, Chem. Commun., 2013, 49, 10679 RSC;
(c) T. Iwasaki, H. Takagawa, S. P. Singh, H. Kuniyasu and N. Kambe, J. Am. Chem. Soc., 2013, 135, 9604 CrossRef CAS PubMed;
(d) A. C. Wotal, R. D. Ribson and D. J. Weix, Organometallics, 2014, 33, 5874 CrossRef CAS PubMed;
(e) T. Iwasaki, H. Takagawa, K. Okamoto, S. P. Singh, H. Kuniyasu and N. Kambe, Synthesis, 2014, 46, 1583 CrossRef;
(f) M. Zhang, W. Li, Y. Duan, P. Xu, S. Zhang and C. Zhu, Org. Lett., 2016, 18, 3266 CrossRef CAS PubMed;
(g) T. Iwasaki, K. Yamashita, H. Kuniyasu and N. Kambe, Org. Lett., 2017, 19, 3691 CrossRef CAS PubMed . The 1H NMR and GC-MS analysis of the crude reaction system also indicated an in situ-generated ICCl2H (for details, see the ESI†).
-
(a) X. He, K. Ruhlandt-Senge and P. P. Power, J. Am. Chem. Soc., 1994, 116, 6963 CrossRef CAS;
(b) T. Nishikata, Y. Noda, R. Fujimoto and T. Sashikata, J. Am. Chem. Soc., 2013, 135, 16372 CrossRef CAS PubMed;
(c) S. Chiba and H. Chen, Org. Biomol. Chem., 2014, 12, 4051 RSC;
(d) S. Ishikawa, Y. Noda, M. Wada and T. Nishikata, J. Org. Chem., 2015, 80, 755 CrossRef PubMed;
(e) D. J. Fisher, G. L. Burnett, R. Velasco and J. R. de Alaniz, J. Am. Chem. Soc., 2015, 137, 11614 CrossRef CAS PubMed;
(f) X. Chen, X. Liu and J. T. Mohr, J. Am. Chem. Soc., 2016, 138, 6364 CrossRef CAS PubMed;
(g) T. Nishikata, K. Itonaga, N. Yamaguchi and M. Sumimoto, Org. Lett., 2017, 19, 2686 CrossRef CAS PubMed;
(h) X. Wang, S. Zhao, J. Liu, D. Zhu, M. Guo, X. Tang and G. Wang, Org. Lett., 2017, 19, 4187 CrossRef CAS PubMed.
-
(a) L. Legnani and B. Morandi, Angew. Chem., Int. Ed., 2016, 55, 2248 CrossRef CAS PubMed;
(b) S.-E. Wang, Q. He and R. Fan, Org. Lett., 2017, 19, 6478 CrossRef CAS PubMed.
-
(a) A. J. Clark, Chem. Soc. Rev., 2002, 31, 1 RSC;
(b) J. Wang, C. Liu, J. Yuan and A. Lei, Angew. Chem., Int. Ed., 2013, 52, 2256 CrossRef CAS PubMed;
(c) S. Tang, K. Liu, C. Liu and A. Lei, Chem. Soc. Rev., 2015, 44, 1070 RSC;
(d) R. N. Ram, D. K. Gupta and V. K. Soni, J. Org. Chem., 2016, 81, 1665 CrossRef CAS PubMed;
(e) W. Jian, L. Ge, Y. Jiao, B. Qian and H. Bao, Angew. Chem., Int. Ed., 2017, 56, 3650 CrossRef CAS PubMed.
-
(a) M. Schwarz, Synlett, 2000, 1369 CAS;
(b) H. Wissmann and H.-J. Kleiner, Angew. Chem., Int. Ed., 1980, 19, 133 CrossRef;
(c) for the hydrolysis of the dichloromethyl group to an aldehyde, see: ref. 8c.
-
(a) The subjection of 2-phenylpropene to the standard conditions afforded 4,4-dichloro-2-phenyl-1-butene as a yellow liquid in 23% yield, and no conjugated vinyl aldehyde was obtained;
(b) simple alkyl alkenes are not compatible with this reaction system.
Footnote |
† Electronic supplementary information (ESI) available. See DOI: 10.1039/c8sc00210j |
|
This journal is © The Royal Society of Chemistry 2018 |
Click here to see how this site uses Cookies. View our privacy policy here.